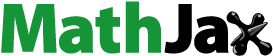
Abstract
Objectives
We sought to investigate the effect of maternal hypothyroidism during pregnancy on fetal cardiac structural and functional remodeling using fetal echocardiography.
Methods
A total of 59 pregnant women with history of hypothyroidism were prospectively enrolled as the study group, and 74 normal fetuses as the control group. Fetal echocardiography was performed on each subject. Demographic, clinical, and fetal echocardiographic variables were measured, including left ventricular (LV) and right ventricular (RV) free wall and ventricular septal thickness, fractional shortening (FS), stroke volume (SV), cardiac output (CO), combined cardiac output (CCO), cardiac index (CI), combined cardiac index (CCI), aortic and pulmonary artery velocity, ductus venosus (DV) and pulmonary vein (PV) spectral Doppler, and Tei index.
Results
The incidence of echogenic intracardiac foci (EIF) was higher in the study group than that in the control group (18.6% vs. 6.8%, p = .036). The thickness of LV free wall and interventricular septum was reduced, the pulmonary velocities and CCI, RV FS, CO, and CI were lower, the S, D, S/A, and pulsatility index (PI) of DV were higher, and LV Tei index was higher in the study group compared with the control group. There was no significant difference in other variables between the two groups.
Conclusions
There is cardiac remodeling, and systolic, diastolic functional alterations in fetuses with maternal hypothyroidism. Further investigation is warranted to develop strategies to optimize the outcome of these fetuses.
Introduction
The thyroid hormone can regulate the metabolism of carbohydrates, protein, and fat, and affect the functions of nervous, endocrine, cardiovascular, and reproductive systems. After pregnancy, the maternal thyroid will undergo a series of physiological changes, such as the increase of thyroid volume, the level of thyroid hormones and the thyroid autoimmune state. Thyroid diseases are common in women of childbearing age. The reported prevalence of hypothyroidism during pregnancy in China is about 1% [Citation1]. Due to the close relationship between maternal and fetal thyroid function, hypothyroidism during pregnancy can cause serious consequences. Previous studies have shown that maternal hypothyroidism is associated with significant increase in miscarriage, preterm birth, placental abruption, gestational hypertension, and intrauterine growth restriction [Citation2,Citation3]. The neurointellectual development of offspring of women with hypothyroidism but without adequate treatment may also be affected [Citation4]. Dong et al.’s research shows a higher FT4 concentration was associated with an increased risk of congenital heart defects [Citation5]. A study based on the follow up of a Danish birth cohort shows maternal thyroid function during third trimester of pregnancy may affect long-term blood pressure in the offspring [Citation6]. At present, there are few data on the effect of hypothyroidism on fetal cardiovascular structure and function.
The objective of this study is to investigate the effect of maternal hypothyroidism during pregnancy on fetal cardiac structure and function by fetal echocardiography.
Materials and methods
Study population
A total of 90 singleton fetuses with maternal hypothyroidism were recruited as the experimental group, and 90 normal singleton fetuses were recruited as the control group in Shijiazhuang Fourth Hospital from November 2018 to November 2019. According to the guideline of Endocrinology Society of Chinese Medical Association, the criterion for hypothyroidism is maternal serum TSH >4.00 mIU/L and free T4 is within or below the reference level.
Exclusion criteria: (1) Suboptimal fetal echocardiographic images due to maternal obesity, fetal position, and other reasons. (2) Other pregnancy complications; (3) the fetus has a congenital cardiovascular disease or other abnormality. (4) Exit during testing. Finally, 59 fetuses were included in the experimental group, 74 fetuses were included in the control group. See flowchart for details (). The research protocol was approved by the Ethics Committee of the Fourth Hospital of Shijiazhuang. Written informed consent was obtained before enrollment.
Laboratory examination
Examination of mother
The thyroid function tests were obtained, including the serum thyroid stimulating hormone (TSH), and free thyroxine (FT4). In addition, the age, height, weight, gestational age, and maternal history of the women were recorded. Medical therapy data with levothyroxine (LT4) were also recorded.
Examination of fetus
All fetal echocardiographic studies were performed using a GE Voluson E10 ultrasonic system with a 2.0–5.0 MHz probe. All fetuses were examined by routine prenatal ultrasound to ascertain fetal cardiac and extracardiac abnormalities, and fetal weight and gestational weeks estimated. The fetal echocardiographic study was performed according to the standards by A Practical Guide to Fetal Echocardiography for Normal and Abnormal Hearts (3rd edition) [Citation7] and Guidelines for Ultrasonic Examination of Fetal Heart in China [Citation8]. All fetal echocardiographic imaging (2D and M-mode) and color and spectral Doppler views and images were obtained, recorded digitally, and measured off-line.
The ventricular fractional shortening (FS) was calculated according to the formula: FS = (Dd – Ds)/Dd, where s and d represent systolic and diastolic dimension (D), respectively (). The dimension, peak velocity (VAO), velocity time integration (VTI), and heart rate (HR) were measured using 2D and spectral Doppler at the left ventricular (LV) and right ventricular (RV) outflow tracts, aortic and pulmonary artery. Stroke volume (SV), cardiac output (CO), and cardiac index (CI) were determined using the following formula: SV = D2 × π × VTI/4, CO = SV × HR, combined cardiac output (CCO) = LVCO + RVCO, combined cardiac index (CCI) = CCO/estimated fetal weight (EFW).
Figure 2. The diastolic and systolic diameters of left and right ventricleswere measured by M-mode echocardiography and FS were calculated. A is a 25-weeks normalfetus and B is a 25-weeks fetus in a pregnant woman with hypothyroidism. The RV FS of Bfetus was lower than that of A fetus.
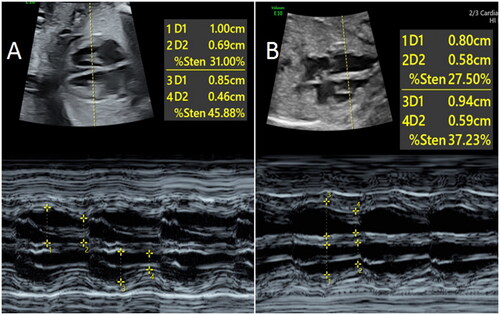
The method of measuring Tei index is as follows: in the view of LV outflow tract, the pulsed Doppler sample volume was placed between LV inflow tract and outflow tract, and the Doppler spectrum of LV inflow tract and outflow tract was obtained. The time (a) between the end of mitral A wave and the beginning of E wave in the next cycle was measured and the duration (b) of aortic wave was also measured (). The LV Tei index was calculated according to the formula: Tei index = (a – b)/b. The Doppler spectra of tricuspid valve and pulmonary valve were obtained in the RV inflow and outflow tract, respectively, and the RV Tei index was determined after correcting the averaged HR [Citation9].
Figure 3. Schematic diagram of calculating Tei index. The time (a) between the end of mitral A wave to the beginning of E wave in the next cardiac cycle, and the duration (b) of aortic ejection were measured after the Doppler spectra of LV inflow and outflowtract were obtained. Tei index = (a–b)/b. LVOT: Left ventricular outflow tract.
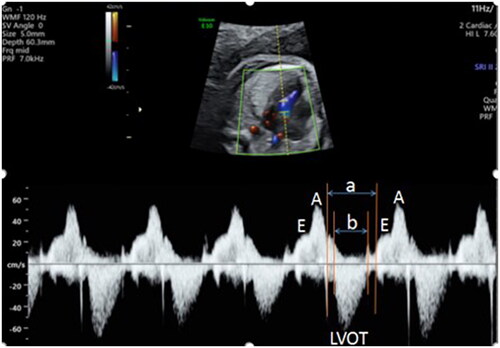
For venous flow Doppler, the Doppler sampling volume was placed in the ductus venosus (DV) in the fetal upper abdomen views, and in the right lower pulmonary vein (PV) in the four-chamber views. The peak velocities of the S wave, D wave and A wave, S/A, pulsatile index (PI) and preload index (PLI) of DV and PV were measured. All the above Doppler flow measurements were optimized for an angle of incidence of <20° between the Doppler ultrasound and the direction of blood flow.
All the studies were performed by the same experienced operator (YZ).
Statistical analysis
All the analyses were performed using SPSS version 26.0 software (SPSS Inc., Chicago, IL).
Sample size calculation
For case-control studies, Schlesselman’s recommends the following formula:
When p0 = 0.25, OR = 10, α = 0.05, β = 0.1, M ≈ 40, that is, the minimum logarithm of case control satisfying the design requirements of this experiment is 40 pairs.
The Shapiro–Wilk test was applied to ascertain normal distribution of the variables. Data from continuous variables were expressed as mean ± standard deviation. Data of homogeneity and heterogeneity of variance were compared between groups using t-test and corrected t-test, respectively. Categorical data were presented as the number of subjects or percentage, and comparison between groups was performed by chi-square test. Statistical tests were two sided, and a p value <.05 was considered statistically significant.
In order to improve the reproducibility of our study results, we performed three repeated measurements of cardiac function parameters in some patients and conducted consistency tests to show that the measurement error of a single examination in our study was within the error range (Table S1).
We constructed Bootstrap test with 1000 iterations for all the results in this paper to perform multiple correction. Multiple linear models were constructed to estimate association of maternal hypothyroidism with fetal cardiac function. Adjusted models were further performed to exclude the impact of maternal age, body mass index (BMI), gestational week, history of gestation, and fetal weight (Table S2). The adjusted results are roughly in line with the previous results.
Results
Fetal cardiac structural abnormality and remodeling
There was no statistical difference in maternal age, gestational age, number of gestations, and maternal height and weight between the study (hypothyroidism) and control groups (p > .05). The serum TSH in the study group was all >4.0 mU/L, with or without free thyroxine (FT4) less than the lower limit of the reference range. The 59 pregnant women in hypothyroidism group were treated with levothyroxine (LT4) with an average daily dose of 52.2 ± 34.5 μg. The TSH of the pregnant women was less than 2.5 mU/L after treatment. The TSH was within normal reference range and no subject was treated with levothyroxine in the control group ().
Table 1. Clinical data for hypothyroidism and control groups.
Echogenic intracardiac focus (EIF) was present in 11 of the 59 fetuses (18.6%) in study group, and five of 74 (6.8%) in the control group (p = .036) ().
Table 2. Comparison of fetal EIF.
There was no statistical difference in cardiothoracic ratio between the study and the control group (p = .66). The thickness of LV free wall (0.16 ± 0.03 cm vs. 0.17 ± 0.03 cm, p = .004) and interventricular septum (0.17 ± 0.02 cm vs. 0.18 ± 0.03 cm, p = .004) was reduced in the study group compared with the control group. There was no significant difference in the thickness of RV free wall between the study and the control group (0.17 ± 0.02 cm vs. 0.18 ± 0.03 cm, p = .052). There was no statistical difference in the LV FS between the two groups (31.83 ± 8.27% vs. 33.68 ± 6.41%, p = .104); but the RV FS was significantly reduced in the study group compared with the control group (24.34 ± 8.28% vs. 29.49 ± 5.41%, p = .002) ().
Table 3. Comparison of fetal myocardial thickness between hypothyroidism and control group.
Fetal cardiovascular flow changes
There was no statistical difference in aortic velocities, CCO, LV SV, LV CO, LV CI, RV SV, DV A wave velocities and PLI, and pulmonary venous S, D, and A wave velocities, S/A ratio, PI and PLI (p > .05, respectively). However, the pulmonary velocities and RV CO, RV CI, and CCI in the study group were lower than the control. DV S, D wave, S/A ratio, and PI were higher in the study group compared with the control group (p < .05, respectively) ().
Table 4. Comparison of fetal heart systolic function, ductus venosus, and pulmonary vein Doppler variables.
Fetal cardiac functional changes
There was no significant difference in RV Tei index (0.32 ± 0.10 vs. 0.29 ± 0.11, p = .104), but higher LV Tei index (0.37 ± 0.07 vs. 0.32 ± 0.08, p < .001) in study group compared with the control group ().
Table 5. Comparison of left and right ventricular Tei index.
Discussion
The cardiovascular system is one of the important target organs of thyroid hormone [Citation10–13]. Many studies have demonstrated that hypothyroidism affects the structure and function of cardiovascular system in adults. Animal studies have also shown that maternal hypothyroidism is associated with lower HR, LV development pressure and tolerance to ischemia in both male and female off-springs as adults [Citation14]. However, there are few reports on the effect of hypothyroidism on fetal heart structure and function during pregnancy.
Among the recruited cases of this study, one fetus in the hypothyroidism group had a vagal right subclavian artery, and one fetus in the control group had a permembranous ventricular septal defect with a diameter of 0.12 cm. These data do not show significant differences in the presence of congenital heart defect between the two groups for the small sample size. It was consistent with the results of Khoury et al. [Citation15], but different from those of Su et al. and Robert et al. [Citation2,Citation16]. In our study, the incidence of EIF in hypothyroidism group was higher than that in the control group, which may be related to fetal metabolic disorder caused by hypothyroidism in pregnant women [Citation17].
Cardiac remodeling refers to a series of changes in ventricular size, shape, wall thickness, and tissue structure caused by myocardial injury or increased load. Studies have shown that cardiac embryogenic gene reactivation is an important mechanism of cardiac remodeling, and thyroxine can interfere with the reactivation of embryogenic gene [Citation18]. Liu [Citation19] showed that the ventricular weight, cardiomyocytes, and vascular abnormalities of the offspring of pregnant rats with hypothyroidism were decreased, and the cardiac development of the offspring improved after L-T4 replacement therapy. Liu and Gerdes [Citation20] found that the cross-sectional area and volume of cardiac myocytes in all cardiac regions of adult rats with hypothyroidism decreased compared with normal ones, and the cross-sectional area and volume of cardiac myocytes could return to normal when thyroid function recovered. Our study showed that the thickness of LV free wall and ventricular septum was reduced in the hypothyroidism group than that in the control group, which may be related to the decrease in the cross-sectional area and volume of fetal cardiomyocytes caused by hypothyroidism in pregnant women.
Fetal cardiac function includes systolic, diastolic function, and overall myocardial function. The LV and RV FS, SV, CO, CI and combined CCO, CCI, are commonly used variables of cardiac contractile function, which represent myocardial contractility and are widely used in evaluating cardiac contractile function and the occurrence, development and prognosis of fetal cardiovascular diseases [Citation21–23]. DV, connecting umbilical vein and inferior vena cava, plays an important role in ensuring adequate supply of oxygen-rich blood in umbilical vein to the fetal brain and myocardium. The increase in positive flow resistance of DV reflected the increase in RV end-diastolic pressure. DV flow parameter is an indirect measure of right diastolic dysfunction [Citation24]. Hofstaetter et al. and Yang et al. applied DV blood flow to the study of chronic hypoxia and hypertensive disorder complicating pregnancy, which showed that DV blood flow parameters would change when fetal hypoxia and intrauterine blood supply were poor [Citation25,Citation26]. DeVore and Horenstein [Citation27] showed that the increase of DV-PLI is associated with diminished intrauterine oxygen supply. Carvalho et al. [Citation28] found that the values of DV-PI and DV-S/A in fetuses with chronic hypoxia were higher than those in normal fetuses. Fetal PV Doppler pattern is related to the pressure of the left atrium, which reflects the changes of the diastolic function of the left ventricle. Myocardial Performance Index (MPI), i.e. Tei index, is not dependent on cardiac structure, HR, blood flow, and other measures, and is mainly used to evaluate the overall global function of the heart, including cardiac systolic function and diastolic function and the fetal cardiac function in congenital heart disease and twin-twin transfusion syndrome [Citation29]. In this study, CCI and RV FS, CO, CI and the pulmonary velocities were lower; S, D wave, S/A and PI of DV were higher; and Tei index of LV was higher in the hypothyroidism group than those in the control group. Pulmonary vein Doppler and other cardiac structural and functional variables showed no significant differences between the two groups. Therefore, our study suggests that maternal hypothyroidism may cause cardiac remodeling and affect cardiac systolic and diastolic function.
There are many reasons that hypothyroidism during pregnancy may change fetal heart structure and function. First, thyroid hormone can enhance myocardial contractility by promoting the release of calcium from cardiomyocytes through its receptors. It can also regulate the electrochemical and mechanical effects of myocardium by regulating Na/K-ATP enzymes, Na/Ca exchangers, and voltage-gated K channels, thus enhance myocardial contractility [Citation30]. Second, thyroid hormone can also act on the related receptors on cardiomyocytes and increase the sensitivity of myocardium to catecholamines [Citation31]. In addition, the effect of thyroid hormone on cardiomyocyte function may be related to anti-apoptosis, as well as promotion of cell proliferation, and cardiomyocyte development and maturation [Citation19]. In hypothyroidism, the release and reuptake of calcium decrease, the role of thyroid hormone in regulating electrochemical channels is weakened, the sensitivity of myocardium to catecholamines is decreased, and the development and maturation of cardiomyocytes are also affected, which may lead to the ventricular remodeling and decreased left and RV systolic and diastolic function. A study based on animal models suggests that developmental hypothyroidism may represent a risk factor for cardiovascular disorders [Citation32]. Pedaran et al.’s findings demonstrate that maternal thyroxine is of particular relevance during the second half of pregnancy for establishing cardiac properties [Citation33].
There are limitations associated with this study. First, this is a single center study. The sample size of this study is relatively small. Second, there were no data on those with clinical hypothyroidism. Third, this study only measured at one time point and did not do longitudinal follow-up. Finally, most of the mothers were with subclinical hypothyroidism during pregnancy likely due to thyroid hormone replacement, which may explain our findings in somewhat more mild fetal cardiac remodeling and functional alterations.
Conclusions
There is cardiac remodeling and systolic, diastolic, and overall functional alterations in fetuses with maternal hypothyroidism compared to euthyroidism. Our data advocate routinely monitoring thyroxine levels during pregnancy to avoid adverse cardiac effects in the offspring.
Supplemental Material
Download MS Word (18.6 KB)Acknowledgements
We are grateful to Ms. Yucui Meng for her assistance with statistical analysis and Dr. Yuan Li for his assistance in enrolling the subjects in this study.
Disclosure statement
No potential conflict of interest was reported by the author(s).
Additional information
Funding
References
- Shan ZY, Chen YY, Teng WP, et al. A study for maternal thyroid hormone deficiency during the first half of pregnancy in China. Eur J Clin Invest. 2009;39(1):37–42.
- Su PY, Huang K, Hao JH, et al. Maternal thyroid function in the first twenty weeks of pregnancy and subsequent fetal and infant development: a prospective population-based cohort study in China. J Clin Endocrinol Metab. 2011;96(10):3234–3241.
- Léger J, dos Santos S, Larroque B, et al. Pregnancy outcomes and relationship to treatment adequacy in women treated early for congenital hypothyroidism: a longitudinal population-based study. J Clin Endocrinol Metab. 2015;100(3):860–869.
- Alexander E, Abalovich M, Stagnaro Green A, et al. Guidelines of the American Thyroid Association for the diagnosis and management of thyroid disease during pregnancy and postpartum. Thyroid. 2011;21(10):1081–1125.
- Dong J, Peng T, Li M-Q, et al. Association between maternal thyroxine and risk of fetal congenital heart defects: a hospital-based cohort study. Int J Endocrinol. 2022;2022:3859388.
- Rytter D, Andersen SL, Bech BH, et al. Maternal thyroid function in pregnancy may program offspring blood pressure, but not adiposity at 20 y of age. Pediatr Res. 2016;80(1):7–13.
- Abuhamad AZ, Chaoui R. A practical guide to fetal echocardiography normal and abnormal hearts. 3rd ed. USA: Wolters Kluwer Health Inc.; 2015. p. 66–252.
- Dong FQ. Guidelines for ultrasonic examination of fetal heart in China. 1st ed. Beijing: People’s Health Publishing House; 2018. p. 33–108.
- Zhang YH, Chen GH, Li WJ, et al. The value of Tei index in evaluating fetal cardiac function after average heart rate correction. J Appl Med. 2013;29(22):3655–3657.
- Chaker L, Bianco AC, Jonklaas J, et al. Hypothyroidism. Lancet. 2017;390(10101):1550–1562.
- Sun T, Guan AN, Zhang XY. Diagnosis and treatment of 21 cases of hypothyroidism heart disease. Chin J Pract Intern Med. 2001;21(5):300–301.
- Kahaly GJ, Dillmann WH. Thyroid hormone action in the heart. Endocr Rev. 2005;26(5):704–728.
- Kahaly G, Mohr-Kahaly S, Beyer J, et al. Left ventricular function analyzed by Doppler and echocardiographic methods in short-term hypothyroidism. Am J Cardiol. 1995;75(8):645–648.
- Ghanbari M, Jeddi S, Bagheripuor F, et al. The effect of maternal hypothyroidism on cardiac function and tolerance to ischemia–reperfusion injury in offspring male and female rats. J Endocrinol Invest. 2015;38(8):915–922.
- Khoury MJ, Becerra JE, d‘Almada PJ. Maternal thyroid disease and risk of birth defects in offspring: a population-based case-control study. Paediatr Perinat Epidemiol. 1989;3(4):402–420.
- Robert E, Vollset SE, Botto L, et al. Malformation surveillance and maternal drug exposure: the MADRE project. Int J Risk Saf Med. 1994;6(2):75–118.
- Wang LZ, Xue L. Clinical follow-up and mechanism of fetal intracardiac hyperechoic foci. Journal of Knowledge of prevention and treatment of cardiovascular diseases (second half); 2015;(2):82–84.
- Zhong DL, Wu HC. Research progress of thyroid hormone on myocardial remodeling. Guangdong Med J. 2016;37(10):1573–1575.
- Liu JL. Effect of congenital hypothyroidism on cardiac development [D]. Tianjin, China: Tianjin Medical University; 2017.
- Liu Z, Gerdes AM. Influence of hypothyroidism and the reversal of hypothyroidism on hemodynamics and cell size in the adult rat heart. J Mol Cell Cardiol. 1990;22(12):1339–1348.
- Meta-analysis Global Group in Chronic Heart Failure (MAGGIC). The survival of patients with heart failure with preserved or reduced left ventricular ejection fraction: an individual patient data meta-analysis. Eur Heart J. 2012;33(14):1750–1757.
- Drazner MH, Rame JE, Marino EK, et al. Increased left ventricular mass is a risk factor for the development of a depressed left ventricular ejection fraction within five years: the cardiovascular health study. J Am Coll Cardiol. 2004;43(12):2207–2215.
- Pieri M, Belletti A, Monaco F, et al. Outcome of cardiac surgery in patients with low preoperative ejection fraction. BMC Anesthesiol. 2016;16(1):97.
- Berkley E, Chauhan SP, Abuhamad A, et al. Doppler assessment of the fetus with intrauterine growth restriction. Am J Obstet Gynecol. 2012;206(4):300–308.
- Hofstaetter C, Gudmundsson S, Hansmann M. Venous Doppler velocimetry in the surveillance of severely compromised fetuses. Ultrasound Obstet Gynecol. 2002;20(3):233–239.
- Yang X, Wang YC, Liu XY. Tei index combined with pulmonary venous and ductal blood flow parameters to evaluate fetal cardiac function in patients with gestational hypertension. Chin J Ultrasound Med. 2017;33(9):809–812.
- DeVore GR, Horenstein J. Ductus venosus index: a method for evaluating right ventricular preload in the second-trimester fetus. Ultrasound Obstet Gynecol. 1993;3(5):338–342.
- Carvalho FH, Moron AF, Mattar R, et al. Ductus venosus Doppler velocimetry to predict acidemia at birth in pregnancies with placental insufficiency. Rev Assoc Med Bras (1992). 2005;51(4):221–227.
- Jiang ZJ, Su FL. Tei index evaluation of right ventricular function in fetus with hypertensive disease during pregnancy. J Guangxi Med Univ. 2017;34(6):890–891.
- Luo BY, Zhao YJ. Effects of thyroid hormone on cardiovascular system. Int J Endocrinol Metab. 2008;28(4):246–248.
- Lazarus JH, Othman S. Thyroid disease in relation to pregnancy. Clin Endocrinol. 1991;34(1):91–98.
- Mittag J, Lyons DJ, Sällström J, et al. Thyroid hormone is required for hypothalamic neurons regulating cardiovascular functions. J Clin Invest. 2013;123(1):509–516.
- Pedaran M, Oelkrug R, Sun Q, et al. Maternal thyroid hormone programs cardiovascular functions in the offspring. Thyroid. 2021;31(9):1424–1435.