Abstract
Background
Respiratory distress is common in neonates admitted to neonatal intensive care units. Additionally, infectious diseases such as intrauterine infections or vertical transmission are important underlying causes of respiratory failure. However, pathogens often cannot be identified in neonates, and there are many cases in which antibacterial drugs are empirically administered. Next-generation sequencing (NGS) is advantageous in that it can detect trace amounts of bacteria that cannot be detected by culturing or bacteria that are difficult to cultivate. However, there are few reports on the diagnosis of infectious diseases using NGS in the neonatal field, especially those targeting respiratory distress.
Objective
The purpose of our study was to investigate the microorganisms associated with neonatal respiratory distress and to determine whether less invasive collection specimens such as plasma and gastric fluid are useful.
Methods
Neonates were prospectively recruited between January and August 2020 from Nagoya University Hospital. The inclusion criteria were as follows: 1) admission to the neonatal intensive care unit; 2) respiratory distress presentation within 48 h of birth; and 3) suspected infection, collection of blood culture, and administration of antibiotics. Plasma samples and blood cultures were simultaneously collected. Gastric fluid samples were also collected if the patient was not started on enteral nutrition. Information on the patients and their mothers were collected from the medical records. DNA was extracted from 140 µL of plasma and gastric fluid samples. DNA sequencing libraries were prepared, and their quality was analyzed. DNA libraries were sequenced using high-throughput NGS. The NGS data of plasma and gastric fluid samples were analyzed using the metagenomic pipeline PATHDET, which calculated the number of reads assigned to microorganisms and their relative abundance. Putative pathogens were listed.
Results
Overall, 30 plasma samples and 25 gastric fluid samples from 30 neonates were analyzed. Microorganism-derived reads of gastric fluid samples were significantly higher than those of plasma samples. Transient tachypnea of the newborn was the most common cause of respiratory distress with 13 cases (43%), followed by respiratory distress syndrome with 7 cases (23%). There were 8 cases (29%) of chorioamnionitis and 7 cases (25%) of funisitis pathologically diagnosed. All blood cultures were negative, and only two gastric fluid cultures were positive for group B Streptococcus (Patient 15) and Candida albicans (Patient 24). Putative pathogens that met the positive criteria for PATHET were detected in four gastric fluid samples, one of which was group B Streptococcus from Patient 15. In the gastric fluid sample of Patient 24, Candida albicans were detected by NGS but did not meet the positive criteria for PATHDET. Cluster analysis of the plasma samples divided them into two study groups, and the indicator genera of each cluster (Phormidium or Toxoplasma) are shown in Figure 1. Clinical findings did not show any significant differences between the two groups. Cluster analysis of the gastric fluid samples divided them into three study groups, and the indicator genera of each cluster (Ureaplasma, Nostoc, and Streptococcus) are shown in Figure 2. The incidence rate of chorioamnionitis was significantly higher in Ureaplasma group than in the other two groups.
Conclusion
Gastric fluid may be useful for assessing neonatal patients with respiratory distress. To the best of our knowledge, this was the first study to reveal that the presence of Ureaplasma in the gastric fluid of neonates with respiratory distress was associated with chorioamnionitis. The early diagnosis of intra-amniotic infections using gastric fluid and its treatment may change the treatment strategy for neonatal respiratory distress. Screening for Ureaplasma in neonates with respiratory distress may reduce the need for empirical antibiotic administration. Further research is required to confirm these findings.
Introduction
Immediately after birth, neonates are in a very unstable clinical state because they start breathing and change their circulatory dynamics to adapt to the external environment. Therefore, many neonates show some form of illness, and 7.8–9.1% of all babies are admitted to the neonatal intensive care unit (NICU) for medical care [Citation1,Citation2]. Respiratory distress is a common symptom of neonates who are admitted to the NICU. Infectious diseases, including intrauterine infections or vertical transmission, are important underlying causes of respiratory failure [Citation3]. Escherichia coli and Group B Streptococcus are the most common causative microorganisms of early-onset infectious diseases in neonates [Citation4]. However, pathogens in neonates often cannot be identified, and there are many cases in which empirical antibacterial treatment is administered [Citation5,Citation6]. Identifying pathogens is important to reduce unnecessary administration of antibiotics, provide appropriate treatment, and improve the prognosis of neonates.
Next-generation sequencing (NGS) can comprehensively analyze nucleic acids within a sample. An advantage of NGS is that it can detect trace amounts of bacteria that cannot be detected by culture or are difficult to cultivate in the laboratory. In clinical microbiology, several studies have shown that NGS can detect putative causative microorganisms that cannot be detected using conventional tests [Citation7,Citation8]. We propose that this technology will be useful in pediatrics, where limited specimens are available for microbiological testing [Citation9,Citation10]. However, in neonatology, reports of NGS-based diagnoses of infectious diseases, especially those that cause respiratory distress, are limited. Furthermore, NGS use requires the identification of the type of specimens that are suitable for identifying the causative microorganisms of neonatal respiratory distress, and this is not clearly known. The gastric fluid of neonates, which can be collected less invasively, maybe a suitable specimen for assessing intra-amniotic infection because it is mainly composed of the amniotic fluid at birth.
This study aimed to investigate the microorganisms associated with neonatal respiratory distress and to determine whether less invasive collection specimens such as plasma and gastric fluid are useful. We used NGS to detect microorganisms that are associated with neonatal respiratory distress from microbial genes identified in plasma and gastric fluid samples and investigated their relationship based on clinical information.
Methods
Patients and samples
Neonates were prospectively recruited from Nagoya University Hospital between January and August 2020 if they met all of the inclusion criteria: 1) admission to the NICU; 2) presenting respiratory distress within 48 h of birth; and 3) neonatologist-suspected infection, collection of blood culture sample, and administration of antibiotics. Plasma and blood culture samples were collected simultaneously. Moreover, gastric fluid samples were collected, and a culture test was performed if the patient had not been started on enteral nutrition. Information of the patient–mother dyads, including gestational age, birth weight, Apgar score, sex, delivery mode, underlying disease, vital signs at onset, laboratory examinations at onset, culture results, premature rupture of membrane, and pathological findings of placenta and umbilical cord, was collected from the medical records. Chorioamnionitis and funisitis were pathologically diagnosed.
Sample preparation and sequencing
The collected plasma and gastric fluid samples were stored at −30 °C until further use. DNA was extracted from 140 µL plasma and gastric fluid samples using a QIAamp UCP Pathogen Mini Kit (Qiagen, Hilden, Germany). DNA concentrations were measured using a Qubit dsDNA HS Assay Kit (Thermo Fisher Scientific, Waltham, MA, USA). DNA sequencing libraries were prepared using the Nextera XT Library Prep Kit (Illumina, San Diego, CA, USA) according to the manufacturer’s instructions, albeit with slight modifications to increase the library concentration as follows: in the clean-up step, DNA libraries were purified twice using AMPure XP beads and the final elution was carried out in 42.5 µL resuspension buffer. Library quality was analyzed using an Agilent 2100 Bioanalyzer (Agilent, Santa Clara, CA, USA) and droplet digital polymerase chain reaction (PCR). DNA libraries were sequenced on the HiSeq system (Illumina) with the 2 × 150 bp paired-end protocol in rapid-run mode.
NGS data processing
The NGS data of plasma and gastric fluid samples were analyzed using the metagenomic pipeline PATHDET, as described previously [Citation11], and the putative pathogens were listed. The PATHDET pipeline calculated the number of reads assigned to microorganisms per million total sequencing reads (RPM), and the percentage of microorganism sequence reads in the total microorganism reads was reported as the relative abundance. The positive criteria for PATHDET were defined as total microorganism reads >650, RPM >200, and a Shannon diversity index <3.0[11].
Statistical analysis
Comparison of microbiota among samples from patients was analyzed as follows using the R package “vegan” and included (1) calculation of Bray–Curtis dissimilarity, (2) hierarchical clustering by Ward method, and (3) extraction of indicator genus of each cluster by the IndVal method as described previously[Citation11]. The results were compared between two groups using Fisher’s exact test for categorical variables and the Mann–Whitney U test for continuous variables. Meanwhile, the results were compared among three groups using Fisher’s exact test, residual analysis for categorical variables, and the Kruskal–Wallis test for continuous variables. SPSS version 27.0 (IBM, Chicago, IL, USA) was used for the statistical analyses, and p-values <.05 were considered statistically significant.
Results
Sequence reads by NGS
A total of 31 plasma and 27 gastric fluid samples were collected from 31 neonates. One plasma sample and two gastric fluid samples did not produce sequence output owing to poor library quality; in total, 30 plasma samples and 25 gastric fluid samples from 30 neonates were analyzed. We obtained an average of 15 and 27 million reads per plasma and gastric fluid sample, respectively; 0.08% of the total reads in the plasma samples and 0.20% in the gastric fluid samples were identified as non-human reads. The RPM of the gastric fluid samples was significantly higher than that of the plasma samples (plasma: 111 ± 110 vs. gastric fluid: 1277 ± 3,835, p = .01).
Clinical characteristics of neonates with respiratory distress
The median gestational age was 33.5 weeks (range, 24–41 weeks). Transient tachypnea of the newborn was the most common cause of respiratory distress in 13 cases (43%), followed by respiratory distress syndrome in 7 cases (23%). There were 8 cases (29%) of chorioamnionitis and 7 cases (25%) of funisitis. All blood cultures were negative, and only two gastric fluid cultures were positive for Group B Streptococcus (Patient 15) and Candida albicans (Patient 24). Supplementary Table summarizes the clinical characteristics of the 30 neonates.
NGS results in plasma samples
shows the relative abundance of microorganisms at the genus level in plasma samples. In the cluster analysis, the plasma samples were divided into two groups, and the representative microorganisms in each group (indicator genera) were identified. The group with Nostoc, Phormidium, Microcoleus, and Sphingomonas as the indicator genera was called the Phormidium group, and the group with Toxoplasma, Mycobacterium, and Bacillus as the indicator genera were called the Toxoplasma group. The clinical characteristics of the two groups were compared (), and no significant intergroup differences were found.
Figure 1. Relative abundance and cluster analysis of the microbiota in plasma samples. One bar graph represents one plasma sample and shows the relative abundance at which each microorganism is detected. The Bray–Curtis dissimilarity among plasma samples is calculated, and the samples are clustered into two clusters by using the Ward method. The indicator genera of each cluster extracted by the IndVal method are indicated by * and †.
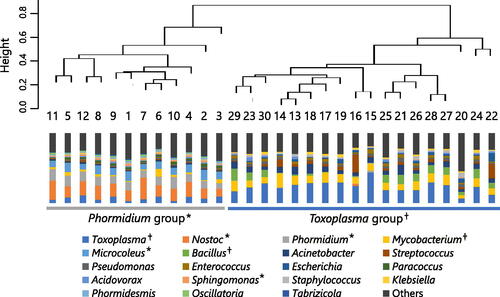
Table 1. Comparison of patient characteristics according to plasma sample group.
NGS results in gastric fluid samples
shows the relative abundance of microorganisms at the genus level in gastric fluid samples. Putative pathogens that met the positive criteria for PATHDET were detected in four gastric fluid samples as follows: Nostocaceae from Patients 2 and 14, Streptococcus anginosus group and E. coli from patient 24; and Group B Streptococcus from Patient 15. In the gastric fluid sample of Patient 24, Candida albicans were detected by NGS but did not meet the positive criteria for PATHDET. For the cluster analysis, the gastric fluid samples were divided into three groups, and the indicator genera could be identified. The group with Ureaplasma as the indicator genus was named the Ureaplasma group; the group with Nostoc, Phormidium, and Microcoleus as the indicator genera were named the Nostoc group; and the group with Streptococcus and Escherichia as the indicator genera were named the Streptococcus group.
Figure 2. Relative abundance and cluster analysis of the microbiota in gastric fluid samples. The bar graph represents one gastric fluid sample and shows the relative abundance at which each microorganism is detected. The Bray–Curtis dissimilarity among gastric fluid samples is calculated, and the samples are grouped into three clusters by using the Ward method. The indicator genera of each cluster extracted by the IndVal method are indicated by *, †, and ‡.
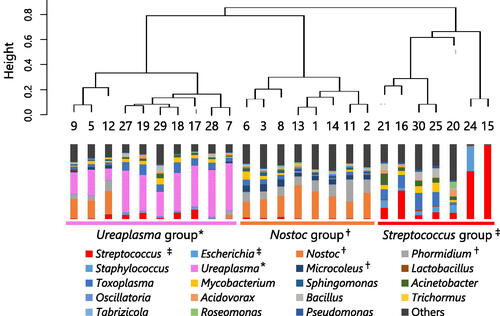
The comparison of the clinical characteristics of the three groups is shown in . The rate of chorioamnionitis was significantly higher in the Ureaplasma group. Although the rates of premature rupture of membranes and funisitis tended to be higher in the Ureaplasma group, the differences were not significant. In addition, we focused on Ureaplasma and compared the clinical characteristics between the Ureaplasma group and the non-Ureaplasma group (). The rates of premature rupture of membrane and chorioamnionitis were significantly higher in the Ureaplasma group.
Table 2. Comparison of patient characteristics according to gastric fluid sample group.
Table 3. Comparison of patient characteristics according to the detection of Ureaplasma in gastric fluid samples.
Discussion
In the present analysis of gastric fluid by NGS, Ureaplasma was detected in 10 of the 25 (40%) gastric fluid samples. To our best knowledge, this is the first study to determine that the presence of Ureaplasma in gastric fluid is associated with chorioamnionitis. Ureaplasma, an asymptomatic genitourinary normal inhabitant, is detected in 72.3%–80% of cervical and vaginal discharge cultures from pregnant women[Citation12,Citation13]. Some studies have reported no association between the vaginal carriage and preterm birth [Citation14–16]. In addition, Ureaplasma was detected in 22%–30% of gastric fluid samples from preterm infants by PCR and 16S rRNA gene sequencing in previous studies [Citation17,Citation18], and a similar detection rate was observed in the current study. The detection of Ureaplasma in amniotic fluid and the placenta in preterm infants is associated with chorioamnionitis [Citation19,Citation20], but no association between Ureaplasma in gastric fluid and chorioamnionitis has been found.
As the gastric fluid of neonates mainly consists of amniotic fluid, the detection of bacteria in the gastric fluid indicates a high likelihood of detection of those bacteria in the amniotic fluid [Citation19,Citation21]. Thus, examination of the gastric fluid of neonates facilitates the assessment of intra-amniotic infection. Although amniotic fluid cannot be collected in all cases, gastric fluid can be collected less invasively and is useful as a test sample. In this study, NGS was used to detect Ureaplasma spp. and Streptococcus spp. Ureaplasma is difficult to detect in culture tests [Citation22] but can be detected by NGS. However, NGS has low fungal detection sensitivity, and C. albicans did not meet the positive criteria for PATHDET in this study despite having the highest number of reads in the fungal genomes in the analytical data. Extracting DNA from some fungal species is difficult because of the presence of several cell wall compounds that interfere with the DNA extraction process [Citation23]. Therefore, the sequence readings for fungi in clinical samples tend to be lower than those for other microorganisms [Citation24]. To improve the detection of fungal pathogens, it is necessary to set lower fungal detection thresholds and to develop more effective fungal DNA extraction methods for clinical samples.
Only 4 cases in the current study met the positive criteria for PATHDET, and the detection of Group B Streptococcus in Patient 5 was consistent with the culture results. In Patients 2 and 14 in whom Nostocaceae was detected, the amount of DNA extracted was very low, and contamination from the kit during library preparation was suspected. Patient 24, in whom the S. anginosus group and E. coli were detected, had been delivered via a vacuum extraction after prolonged labor and was diagnosed with meconium aspiration syndrome. Streptococcus anginosus is a vaginal indigenous bacterium. In Patient 24, it is possible that the amniotic fluid is already contaminated during childbirth and that the patient has swallowed the contaminated amniotic fluid.
The early diagnosis of Ureaplasma using gastric fluid samples and the option of individualized treatment may change the treatment strategy in neonatal respiratory distress. In some studies of attempts to improve prognosis by preemptively targeting Ureaplasma, which is associated with intrauterine infection, the administration of macrolide antibiotics to neonates without confirmation of the presence of Ureaplasma does not affect prognosis [Citation25,Citation26]. The review study by Chang et al. showed that azithromycin was effective at Ureaplasma eradication, but most studies did not find a significant preventive effect of azithromycin for bronchopulmonary dysplasia [Citation27]. However, several meta-analyses reported a significant association between Ureaplasma respiratory colonization and bronchopulmonary dysplasia [Citation28]. Therefore, the detection of Ureaplasma has prognostic value for bronchopulmonary dysplasia. In addition, the finding that additional pathogenic agents are unlikely to be found by comprehensive analysis using NGS has important implications for clinical treatment decisions. Under the present condition, the assay used in this study takes more than 40 h to complete and several hours for data analysis. The purpose of using NGS was not only for rapid detection of Ureaplasma, but also for detecting the microbiome. When targeting Ureaplasma in the clinical setting, the PCR method for Ureaplasma can be used to diagnose it more quickly. However, improvement of the NGS methods will enable its utility for rapid diagnosis. In the future, the gastric fluid of neonates may be examined using NGS for early diagnosis of Ureaplasma and improve prognosis. In addition, screening neonates for respiratory distress may reduce empirical antibiotic administration.
NGS could not identify the microorganisms that were responsible for neonatal respiratory distress in plasma samples, and no microorganism was associated with clinical information. Blood cultures were negative for all samples, and the number of reads that could be analyzed was small. When the number of reads was small, bacteria such as Nostoc and Phormidium, which seemed to be contaminants that were introduced during the library preparation, accounted for a large proportion of microorganisms and were identified as indicator genera. Toxoplasma gondii, a clinically important protozoan that causes congenital toxoplasmosis, is a representative bioinformatics contaminant [Citation11]. Plasma was unsuitable as the sample because sufficient amounts of DNA could not be detected.
This study had several limitations. First, the sample size was small. Furthermore, the participants were heterogeneous, including extremely low birth weight and term infants. Second, as only DNA was analyzed, RNA viruses could not be detected. RNA analysis was not performed because we believed that the previously known causative microorganisms of neonatal respiratory distress could be almost completely covered by DNA analysis. Third, we could not perform a comparison with the group without respiratory distress. It is unclear how often Ureaplasma was detected in neonates without respiratory distress.
In conclusion, the plasma and gastric fluid of neonates with respiratory distress were analyzed using NGS, and gastric fluid was more useful as a test sample. Ureaplasma that was detected in the gastric fluid was associated with chorioamnionitis. No other microorganism was associated with the clinical course. Early diagnosis of intra-amniotic infections due to Ureaplasma in gastric fluid samples and specific treatment of Ureaplasma infection may help improve prognosis in neonates with respiratory distress; however, further research is needed to confirm the clinical applicability and relevance of this finding.
Ethics approval and consent to participate
The study was approved by the Institutional Review Board of Nagoya University Graduate School of Medicine (approval no. 2019-0373). This study was carried out in compliance with the Declaration of Helsinki guidelines. Written consent was obtained from the parents of the neonates who participated in this study.
Authors’ contributions
T.Ok., K.Ho. M.H., and Y.I. designed the study. Y.Sa., K.Ha., and M.Y. collected clinical samples. T.O., N.T. Y.Su., and J.K reviewed the medical records for the clinical assessment of patients. T.Ok., K.Ho., M.Y., T.S., and Y.T. conducted experiments. T.Ok., K.Ho., and T.Og. performed the bioinformatics analysis. T.O., K.H., N.T., Y.S. and Y.I. drafted the manuscript. All authors have read and approved the final manuscript.
Supplemental Material
Download MS Word (108.6 KB)Acknowledgements
The authors acknowledge all the children and parents who participated in this study.
Disclosure statement
The authors report there are no competing interests to declare.
Data availability statement
Full datasets supporting the findings of this study are available from the corresponding author upon reasonable request. Sequencing data generated and/or analyzed during the current study are available in the DDBJ Sequence Read Archive under experiment number DRR402161-DRR402215.
Additional information
Funding
References
- Harrison W, Goodman D. Epidemiologic trends in neonatal intensive care, 2007-2012. JAMA Pediatr. 2015;169(9):855–862.
- Kim Y, Ganduglia-Cazaban C, Chan W, et al. Trends in neonatal intensive care unit admissions by race/ethnicity in the United States, 2008–2018. Sci Rep. 2021;11(1):23795.
- Reuter S, Moser C, Baack M. Respiratory distress in the newborn. Pediatr Rev. 2014;35(10):417–428; quiz 429.
- Shane AL, Sánchez PJ, Stoll BJ. Neonatal sepsis. Lancet. 2017;390(10104):1770–1780.
- Ramasethu J, Kawakita T. Antibiotic stewardship in perinatal and neonatal care. Semin Fetal Neonatal Med. 2017;22(5):278–283.
- Schulman J, Dimand RJ, Lee HC, et al. Neonatal intensive care unit antibiotic use. Pediatrics. 2015;135(5):826–833.
- Wilson MR, Sample HA, Zorn KC, et al. Clinical metagenomic sequencing for diagnosis of meningitis and encephalitis. N Engl J Med. 2019;380(24):2327–2340.
- Blauwkamp TA, Thair S, Rosen MJ, et al. Analytical and clinical validation of a microbial cell-free DNA sequencing test for infectious disease. Nat Microbiol. 2019;4(4):663–674.
- Takeuchi S, Kawada J, Horiba K, et al. Metagenomic analysis using next-generation sequencing of pathogens in bronchoalveolar lavage fluid from pediatric patients with respiratory failure. Sci Rep. 2019;9(1):12909.
- Suzuki T, Kawada J, Okuno Y, et al. Comprehensive detection of viruses in pediatric patients with acute liver failure using next-generation sequencing. J Clin Virol. 2017;96:67–72.
- Horiba K, Torii Y, Okumura T, et al. Next-generation sequencing to detect pathogens in pediatric febrile neutropenia: a single-center retrospective study of 112 cases. Open Forum Infect Dis. 2021;8:ofab223.
- Wang QY, Li RH, Zheng LQ, et al. Prevalence and antimicrobial susceptibility of ureaplasma urealyticum and mycoplasma hominis in female outpatients, 2009–2013. J Microbiol Immunol Infect. 2016;49(3):359–362.
- Capoccia R, Greub G, Baud D. Ureaplasma urealyticum, mycoplasma hominis and adverse pregnancy outcomes. Curr Opin Infect Dis. 2013;26(3):231–240.
- Klein LL, Gibbs RS. Use of microbial cultures and antibiotics in the prevention of infection-associated preterm birth. Am J Obstet Gynecol. 2004;190(6):1493–1502.
- Bartkeviciene D, Opolskiene G, Bartkeviciute A, et al. The impact of ureaplasma infections on pregnancy complications. Libyan J Med. 2020;15(1):1812821.
- Carey JC, Blackwelder WC, Nugent RP, et al. Antepartum cultures for ureaplasma urealyticum are not useful in predicting pregnancy outcome. The vaginal infections and prematurity study group. Am J Obstet Gynecol. 1991;164(3):728–733.
- Oue S, Hiroi M, Ogawa S, et al. Association of gastric fluid microbes at birth with severe bronchopulmonary dysplasia. Arch Dis Child Fetal Neonatal Ed. 2009;94(1):F17–F22.
- Bajorek S, Parker L, Li N, et al. Initial microbial community of the neonatal stomach immediately after birth. Gut Microbes. 2019;10(3):289–297.
- Kim SM, Romero R, Lee JH, et al. Gastric fluid versus amniotic fluid analysis for the identification of intra-amniotic infection due to ureaplasma species. J Matern Fetal Neonatal Med. 2016;29:2579–2587.
- Namba F, Hasegawa T, Nakayama M, et al. Placental features of chorioamnionitis colonized with ureaplasma species in preterm delivery. Pediatr Res. 2010;67(2):166–172.
- Miralles R, Hodge R, Mcparland PC, et al. Relationship between antenatal inflammation and antenatal infection identified by detection of microbial genes by polymerase chain reaction. Pediatr Res. 2005;57(4):570–577.
- Colaizy TT, Kuforiji T, Sklar RS, et al. PCR methods in clinical investigations of human ureaplasmas: a minireview. Mol Genet Metab. 2003;80(4):389–397.
- Frau A, Kenny JG, Lenzi L, et al. DNA extraction and amplicon production strategies deeply influence the outcome of gut mycobiome studies. Sci Rep. 2019;9(1):9328.
- Miller S, Naccache SN, Samayoa E, et al. Laboratory validation of a clinical metagenomic sequencing assay for pathogen detection in cerebrospinal fluid. Genome Res. 2019;29(5):831–842.
- Ballard HO, Shook LA, Bernard P, et al. Use of azithromycin for the prevention of bronchopulmonary dysplasia in preterm infants: a randomized, double-blind, placebo controlled trial. Pediatr Pulmonol. 2011;46(2):111–118.
- Viscardi RM, Terrin ML, Magder LS, et al. Randomized trial of azithromycin to eradicate ureaplasma respiratory colonization in preterm infants: 2-year outcomes. Pediatr Res. 2022;91(1):178–187.
- Chang E, Ballard KE, Johnson PN, et al. Azithromycin for eradication of ureaplasma and prevention of bronchopulmonary dysplasia in preterm neonates in the neonatal intensive care unit. J Pediatr Pharmacol Ther. 2023;28(1):10–19.
- Viscardi RM, Kallapur SG. Role of ureaplasma respiratory tract colonization in bronchopulmonary dysplasia pathogenesis: current concepts and update. Clin Perinatol. 2015;42(4):719–738.