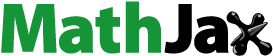
Abstract
Megalospheric specimens of Lepidocyclina from seven localities in western and central Cuba were morphometrically investigated using 11 growth-independent characters for equatorial sections of nepionts and 19 growth-independent and invariant characters describing equatorial sections of neanic chamberlets. This provides a complete geometric reconstruction of the equatorial morphology for lepidocyclinids. Specimens were objectively (to be precise, intersubjectively) classified by clustering and ordination methods resulting in four morphologically homogenous groups. These unprejudiced groups are then subjectively identified as Lepidocyclina macdonaldi, L. pustulosa, L. ariana and L. ocalana following the taxonomic rules and checked for significant differences. The D/P-ratio (deuteroconch/protoconch) separates L. ocalana (D/P 1) from L. pustulosa (D/P
1), L. macdonaldi and L. ariana (D/P
1). The form of neanic chamberlets differentiates both L. macdonaldi and L. pustulosa possessing arcuate chamberlets from L. ariana and L. ocalana with spatulate chamberlets. The smaller sized characters isolate L. pustulosa from the other species. Lepidocyclina macdonaldi has the longest stratigraphical range occurring from ABZ 9 to ABZ 16 (American larger Benthic foraminifera Zonation). Lepidocyclina ariana is restricted to ABZ 12, while L. pustulosa and L. ocalana range from ABZ 13 to ABZ 16 and ABZ 15 to ABZ 16, respectively. An evolutionary trend could be detected in nepionts of L. macdonaldi starting from slightly smaller nepionts at the first appearance to significantly larger nepionts at the end of the Priabonian. Additionally, the arcuate chamberlets arranged in rows transform from not connected to connected. Differences within L. ocalana can be explained by environmental influences due to the palaeogeographical position of samples.
Introduction
After the virtual extinction of larger benthic foraminifera (LBF) at the Cretaceous/Paleogene boundary, enhanced oligotrophy in the Late Paleocene favoured a larger benthic foraminiferal turnover (LFT). A rapid increase in species number and shell size is expressed in strong dimorphism of generations (Hottinger, Citation1997, Citation1998; Orue-Etxebarria et al., Citation2001; Scheibner et al., Citation2005). LBF then dominated the Paleogene neritic biota around the Tethys, Indo-Pacific and American-Caribbean faunal provinces, optimally documenting the maturity and evolution of benthic communities (Adams, Citation1983; Hottinger, Citation1997, Citation1998; Serra-Kiel et al., Citation1998). This applies in particular to the family Lepidocyclinidae that originated at the base of the Eocene in the Americas and became one of the most dominant components in LBF communities throughout the Cenozoic. The high evolutionary rates exhibited by members of this family have been routinely used in biostratigraphy (e.g. Adams, Citation1987; Benedetti & Pignatti, Citation2013; Benedetti & Schiavinotto, Citation2023; Butterlin, Citation1987; Caudri, Citation1996; Chaproniere, Citation1980; Cole, Citation1960; de Mello e Sousa et al., Citation2003; Drooger, Citation1993; Frost & Langenheim, Citation1974; Mitchell et al., Citation2022; Robinson, Citation1996, Citation2004; Robinson & Wright, Citation1993; Saraswati, Citation1994, Citation1995; Sirotti, Citation1982).
The Lepidocyclinidae is a group of Cenozoic three-layered LBF with an initial embryonal part consisting of a protoconch and deuteroconch. An equatorial layer of numerous, radially to concentrically arranged neanic chamberlets and two variably developed layers of lateral chamberlets signifies further growth. Regarding phylogeny, the major radiation of Eocene and Oligocene Lepidocyclina lineages starts in the latest Paleocene or earliest Eocene from an Amphistegina-like ancestor in the Gulf of Mexico and North Caribbean (BouDagher-Fadel & Price, Citation2010; Robinson, Citation1996). Transitional forms belonging to genera Helicostegina, Eulinderina and Polylepidina led to Lepidocyclina (BouDagher-Fadel & Price, Citation2010; Butterlin, Citation1987; Grimsdale, Citation1959; Robinson, Citation1996). Evolution was accomplished through various modifications in morphology, including development of the two lateral chamber layers, a reduction in the length of the nepionic spire of chambers, and increasing radial symmetry of the test. The appearance of lepidocyclinids in the early Oligocene of the Tethys and in the late early Oligocene of the Indo-Pacific confirms eastward migration (BouDagher-Fadel & Price, Citation2010).
The extreme morphological complexity of lepidocyclinids was based on the embryonic apparatus and adjacent chambers as the only reliable tool for species identification (e.g. Adams, Citation1987; Benedetti et al., Citation2010; Benedetti & Pignatti, Citation2013; Drooger, Citation1993; Robinson, Citation1996; Mitchell et al., Citation2022; Van der Vlerk, Citation1959, Citation1963). In order to decipher their morphology and the subtle morphological changes that occur with time, a great number of parameters, indices and criteria have been developed to describe the equatorial morphology of the megalospheric generation (e.g. Benedetti & Pignatti, Citation2013; Benedetti & Schiavinotto, Citation2023; Chaproniere, Citation1980; Hohenegger & Torres-Silva, Citation2020; Robinson, Citation1996; Saraswati, Citation1994, Citation1995; Schiavinotto, Citation1994a, Citation1994b, Citation2010, Citation2016; Schiavinotto & Benedetti, Citation2022). Evolutionary trends include the reduction in the nepionic spire of chambers (Tan, Citation1936) and the increase of the deuteroconch embracement of the protoconch, becoming a globular embryo (Drooger & Freudenthal, Citation1964). The parameter R for the position of the first retrovert chambers of Eulinderina was proposed by Robinson (Citation1996) to express the phylogenetic stage. The progressive reduction of the nepionic spire in Eulinderina occurred through planktic foraminiferal zones P11/P12, and NP 15/NP 16 for calcareous nannoplankton zones, respectively (Robinson, Citation1996). Directed variability in the embryonal parameters of the Mediterranean Nephrolepidina marginata from a deepening upwards succession pointed to a link to palaeo-ecological factors and not to evolution (Benedetti & Pignatti, Citation2013). Therefore, LBF species delimitation based on morphology has to be treated simultaneously by a multitude of morphological characters to explain test shape dependent on niches and evolutionary tendencies (Hohenegger, Citation2011, Citation2014).
Based on extant nummulitids from the West Pacific, Hohenegger (Citation2011) introduced the use of growth-independent and growth-invariant characters as a tool to explain the complete change in test shape during ontogeny as well as their value in clarifying phylogenetic relations and for defining biological species in fossil forms. Growth-dependent characters, which change at each growth step, were described as functions depending on time represented by chamber number (e.g. backbend angle, perimeter ratio of chambers). Constants of growth functions were then used as growth-invariant characters and allow the complete growth changes of the specific character during ontogeny to be determined.
Quantification of test morphology in the fossil record using growth-independent and growth-invariant characters was carried out on Heterostegina, Nummulites, Palaeonummulites and Operculinoides from the Cuban Eocene (Hohenegger & Torres-Silva, Citation2017; Torres-Silva et al., Citation2017, Citation2019). These studies allowed enhanced species recognition and better interpretation of evolutionary trends separated from environmental and palaeogeographical diversification. Therefore, the use of growth-independent and growth-invariant characters seems to be the strongest tool to explore the lepidocyclinid phylogeny.
The present investigation focuses on the morphology of the nepiont and equatorial chamberlets of Eocene Lepidocyclina from Cuba using growth-independent and -invariant characters that provide a complete geometric reconstruction of equatorial sections. We use the set of measurements proposed by Hohenegger and Torres-Silva (Citation2020) to describe the shape of neanic chamberlets and introduce a set of growth-independent characters for the nepiont. An intersubjective (= shared meanings constructed by people in their interactions with each other; incorrectly defined as ‘objective’) grouping based on these characters leads to a delimitation of morphologically homogenous groups within the Cuban Lepidocyclina, which can be interpreted as species. They will be correlated to American Benthic Zones (ABZ; Mitchell et al., Citation2022) and phylogenetic connections between the investigated Lepidocyclina species are discussed.
Geological setting
Shallow marine deposits bearing LBF in Cuba reflect, starting from the middle Eocene, the post-orogenic history of piggy-back basins formed to the south of the major faults on the allochthonous thrust units of the extinct the Cretaceous volcanic arc and ophiolites (Iturralde-Vinent, Citation1994, Citation1995) (). These sediments were deposited in a variety of settings within the outer margins of the carbonate platforms or can frequently be found as penecontemporaneous re-sedimented components of turbidites in hemipelagic sequences related to arc/subduction tectonic situations in the Caribbean (Brönnimann & Rigassi, Citation1963; García-Delgado & Torres-Silva, Citation1997; Iturralde-Vinent, Citation1995; Molina et al., Citation2016; Torres-Silva et al., Citation2017, Citation2019).
Figure 1. A, schematic tectonic map of western and central Cuba (after Iturralde-Vinent, Citation1994) with locations of the stratigraphical sections and samples. B, stratigraphical relations of the Eocene units in western and central Cuba slightly modified from García-Delgado & Torres-Silva (Citation1997). Stratigraphical ranges of the studied sections, A (98LC-1), B (LM-52), C (LM-23), D (P-559, P-562), E (CA-215), F (CA-216).

This work includes stratigraphical sections of Loma Candela, Jabaco, Hatillo, Jicotea, Arroyo Blanco and Blanco formations, which are in western and central Cuba, part of the post-orogenic history of these strike-slip basins during middle to late Eocene (). Torres-Silva et al. (Citation2017, Citation2019) have described sections in detail along with a morphometric approach of the family Nummulitidae. Spot samples collected as part of the systematic geological mapping of Cuba (Kantshev et al., Citation1976) are also included. A short description of the sections is presented below and the locations, site name, site label, formations and age are shown in .
Table 1. Sampling stations with Lepidocyclina.
Western Cuba
Bartonian to early Priabonian deposits of the Loma Candela Formation (Torres-Silva et al., Citation2017, Citation2019) are located at Loma Candelaria (site label 98LC-1) along the roadside to San Diego de los Baños (22°35′48ʺ N, 83°23′10ʺ W; ). At this locality, the formation is partially exposed as a nearly 20 m thick sequence of conglomerates, interbedded with limestones and marls bearing mainly nummulitids (). One spot sample from the Jabaco Formation was collected at Loma Jabaco (site label LM-52), from a barely exposed Late Priabonian sequence of hemipelagic marls with intercalated argillaceous limestones (Torres-Silva et al., Citation2017, Citation2019). It cropped out near the intersection of the road from Guanajay to El Mariel, 4.5 km W-NW of Guanajay (Havana province, western Cuba; 22°56′46ʺ N, 82°44′30ʺ W, ). One spot sample (LM-23) was collected from a Middle Eocene succession of marls and limestones of Hatillo Formation, located 2 km south of San Miguel de los Baños town in Matanzas province (22°51′9ʺ N, 81°19′60ʺ W, ).
Figure 2. Distribution of the samples and LBF assemblages at Loma Candelaria section, western Cuba (modified from Torres-Silva et al., Citation2019).

Central Cuba
Isolated megalospheric specimens of Lepidocyclina were investigated from two spot samples (P-559 and P-562) of Jicotea Formation. Samples were collected from a Late Eocene succession of polymictic sandstones, conglomerates and bio-calcarenites poorly exposed at the north-west of La Esperanza town in Villa Clara Province (22°30′0ʺ N, 79°46′0ʺ W; ). The lower part of Arroyo Blanco Formation is represented at the Loma El Santo section (site label CA-215). A Lutetian sequence of about 20 m of hemipelagic marls and argillaceous limestones with interbedded re-sedimented sandstones are exposed 3 km east of Sancti Spiritus, central Cuba (21°58′0ʺ N, 79°25′59ʺ W; ).
Figure 3. Distribution of samples and assemblages LBF in Loma El Santo section, central Cuba (modified from Torres-Silva et al., Citation2019).

Megalospheric specimens of Lepidocyclina were studied from a nearly 60 m thick Priabonian succession of limestones, marly limestones and marls with abundant LBF, bivalves and gastropods of Blanco Formation (). This sequence outcrops are exposed at the Loma Vigía locality (site label CA-216) in a quarry near Siguaney in Sancti Spiritus province (21°59′0ʺ N, 79°16′36ʺ W; ).
Figure 4. Distribution of samples and LBF assemblages at Loma Vigía section, central Cuba (modified from Torres-Silva et al., Citation2019).

Material and methods
A total of 59 samples were collected from the above-mentioned three sections and four spot samples. From these localities, 58 isolated megalospheric specimens of Lepidocyclina were thin-sectioned through the equatorial plane, to investigate diagnostic inner characters. Each sectioned specimen was photographed with a digital microscope (Leica MC 170 HD) and the morphological characters were measured in equatorial section using the image-processing program ImageJ, version 1.50e. The sometimes abundant microspheres were not investigated using growth-independent and -invariant characters. Distribution of the lepidocylinid specimens at the investigated sections and sampling spots is presented in Supplemental material Table 1.
The associated LBF assemblages were studied based on 42 petrological thin sections and more than 400 oriented individual thin-sections including nummulitids, heterosteginids, orthophragminids, amphisteginids and agglutinated conical forms. Planktic foraminifera and calcareous nannofossils that co-occur with LBF assemblages were studied and assigned to their biozones according to Berggren et al. (Citation1995), Pearson et al. (Citation2006), Martini (Citation1971) and Agnini et al. (Citation2014). The Lepidocyclina occurrences were assigned to American Benthic Zones (ABZ) according to Mitchell et al. (Citation2022). The material is stored at the Department of Palaeontology, University of Vienna under the sample numbers: 98LC-1, LM-52, LM-23, CA-215, CA-216, P-559 and P-562.
To obtain growth-independent and growth-invariant characters in equatorial sections of Lepidocyclinidae, two steps in data processing are necessary. Based on direct measurements of chamberlets in equatorial sections (), growth-independent and -invariant characters can be calculated using constants of growth functions. describes equations and characters of growth functions for neanic chamberlets () following the detailed description in Hohenegger and Torres Silva (2020), while describes the newly developed characters for the nepiont ().
Figure 5. Measurements in equatorial sections: A, spatulate chamberlet; B, arcuate chamberlet; C, nepiont.

Figure 6. Lepidocyclina specimens with measurements of chamberlets (in sectors). 1070 measurements were taken for first and 1163 measurements for the second specimen demonstrating the high time and effort in measuring a single individual. A, 98C-1h(616) and B, P562(496).

Table 2. Growth functions and derived growth-invariant characters for neanic chamberlets.
Table 3. Growth-invariant characters for the nepiont.
Neanic chamberlets
According to Hohenegger & Torres Silva (Citation2020), characters of neanic chamberlets are measured using sectors of the test, where chamberlet rows are preserved from the nepiont to the test margin (). The first row (j = 1) starts after the row with auxiliar and spiral chambers surrounding the proto- and deuteroconch. Sector angles
were kept small to reduce the number of measurements. Therefore, sector angles change slightly between succeeding rows j as does the number i of measured chamberlets within row j. This leads to a large number of approximately 1000 measurements per individual ().
With the exception of the characters ‘total row area’ and ‘chamberlet number’ (), the arithmetic means of measured traits in each row represents the character state for the complete row j, while for the characters ‘total row area’ and ‘chamberlets number’ the transformations
were used.
Growth functions based on row number were fitted for each character and their constants could be used as growth-invariant characters (). Character acronyms follow Hohenegger &Torres Silva (Citation2020) and two representatives of Lepidocylina exemplify the fitting of growth functions ().
Figure 7. Growth functions of specimens pictured in , left for L. group 1, specimen 98C-1h(616), right for L. group 5, specimen P562(496).

Figure 8. Growth functions of specimens pictured in , left for L. group 1, specimen 98C-1h(616), right for L. group 5, specimen P562(496).

Growth of the characters ‘total-, distal- and proximal chamberlet height’ and ‘chamberlet width’ could be fitted by exponential functions (, ). The function values for the first row were used as the initial growth-invariant character, while the constant b was used as the second growth-invariant character determining the function’s increase.
For the character ‘distance to centre’ fitted by a linear function, only function values at the first row were used as the initial growth-invariant character, because constant b completely correlates with cumulative frequencies of the character ‘proximal chamberlet height’, thus becoming redundant.
Linear functions fitting the character ‘chamberlets number’ are approximations to stepwise functions demonstrating the growth program, which is caused by the constancy of chamberlet numbers in a series of consecutive rows (). Without significant increase in chamberlet shape and area, the insertion of additional chamberlets in row j based on the number of ‘dividing chamberlets’ in row j–1 allows the instantaneous enrichment of chamberlet numbers (Hohenegger & Torres Silva, Citation2020, fig. 12). Besides the multiplicative constant b used as the second growth-invariant character, function values of the 6th row determine the initial growth-invariant characters, because after an initial linear increase in chamberlet number, the stepwise functions start in almost all investigated specimens at the 6th row ().
The character ‘chamberlet area’ could be fitted by a 2nd order polynomial function (; ). Function values of the first row act as the initial invariant character. Function extremes – almost minima – and their row positions work as further invariant characters approximating growth of chamberlet area ().
Exponential functions fit the character ‘total row area’ (), again with function values of the first chamberlets acting as initial growth-invariant characters, while constant b characterizes the intensity of increase ().
The characters ‘frontal roundness’ and ‘frontal angle’ are growth-independent, thus can be characterized by arithmetic means due to their equal frequency distributions (; ). The character ‘basal chamberlet width’ is better characterized by the median () because of significant unequal frequency distributions or the complete lack of this character in some specimens (e.g. L. group 5; ).
Nepiont
Here, the nepiont is reduced to measurements of the proto- and deuteroconch. All investigated specimens possess primary auxiliary chambers that do not function as differentiators between groups. As single measurements, all characters of the nepiont are growth-independent. Measurements did not follow the commonly used procedures, which are based on Eurasian lepidocyclinids possessing a larger deuteroconch compared to the protoconch, like in Nephrolepidina (e.g. Benedetti et al., Citation2010; Coletti et al., Citation2019; Drooger, Citation1993; Less et al., Citation2018; Van Wessen, Citation1978). These measurements are difficult to adopt when the deuteroconch is smaller than the protoconch (e.g. L. group 1 of ). Therefore, nepiont measurements comparable to those of neanic chamberlets were executed, allowing the unbiased comparison between proto- and deuteroconch (; ).
Grouping
Eleven growth-independent characters of the nepiont and 19 growth-invariant characters describing neanic chamberlets () were used for positioning the 56 investigated specimens (Supplemental material Table 2) into homogeneous groups. Homogeneities in specimens were obtained by cluster analysis using standardized data (Supplemental material Table 3). Ward’s cluster analysis, based on the ‘Minimum Variance Criterion’, which exclusively depends on Euclidean distances as dissimilarity measures (Ward, Citation1963), could be used to find homogeneous groups and subgroups interpreted as species or subspecies. Principal component analysis (PCA; Hotelling, Citation1933) was applied for specimens grouped by samples on the one hand and for morphologically homogeneous groups, possibly interpreted as species obtained by cluster analysis, on the other. Separate PCAs were performed for neanic chamberlets and nepionts to characterize their influence on homogeneous groups. After describing character frequency distributions of homogeneous groups, their differences were obtained using the parameter-free Kruskal-Wallis test and the post-hoc Dunn’s test for pairwise comparison (Dunn, Citation1964). A final discriminant analysis (Fisher, Citation1936) points to the striking differences between homogeneous groups. Simple statistical analyses were performed in Excel, while complex analyses were completed using the statistical program package PAST 4.10 (Hammer, Citation2021).
Results
Classification
The hierarchical cluster analysis resulted in two clusters, both with a ‘within cluster distance’ (WCD) as a measure for homogeneity between members of <22.6 ().
Specimens of samples CA-216-E3, CA-216-F3, P-559 (exclusive individual 514) and P-562 (exclusive individual 494) belong to a group formerly designated as Lepidocyclina ocalana, objectively named ‘L. group 5’. All individuals of samples 98LC-1h, 98LC-1i, CA-215-17, LM-23 and LM 56 belong to a group formerly designated as L. macdonaldi ().
Three significant subgroups can be found within this L. macdonaldi group. All specimens of sample LM-23 (WCD < 5.1) belong to a distinct cluster designated as ‘L. group 3’. Specimens 98LC-1i(640) and 98LC-1i(642) form a separate cluster with a WCD of < 3.7 and are the only representatives of the formerly designated L. macdonaldi in the investigated samples (Hohenegger & Torres Silva, 2020), now classified as ‘L. group 2’. All specimens of sample LM-52 together with individuals 98LC-1i(853) and 98LC-1i(854) constitute a separate cluster (WCD < 6.0) named ‘L. group 4’.
Grouping by Ward’s method is one way to detect and explain the underlying cluster structure, but like other cluster analyses, it is insufficient for finding the underlying natural cluster structure as in other clustering methods. This explains differences to groupings obtained by ordination methods.
The two main axes of PCA representing 68.7% of total variance () demonstrate a clearer grouping, separating subgroups in cluster analysis as distinct groups. Samples 98LC-1h, 98LC-1i and CA-215-17 cover the same region with increasing dispersion along the second axis, thus strengthening the classification as ‘L. group 1’ obtained by cluster analysis, while samples LM-23 (exclusively individual 429) and LM-52 are clearly separated from the former sample group (), designated as ‘L. group 3’ and ‘L. group 4’. Individuals 853 and 854 of sample 98LC-1i named ‘L. group 2’ were included in ‘L. group 1’ as was recovered by cluster analysis. These specimens were not differentiated from the ‘L. group 1’ by the first and second PC axes, different to the separation in cluster analysis. The low number of specimens causes this. Differentiation with respect to the other specimens of ‘L. group 1’ is documented in factor scores of the 5th PCA for the two specimens (4.70 and 3.17 against the range for the other cluster members between 1.35 and 1.44). With 4.38% variance proportion, the 5th component shows high factor loadings of the characters frtRD (frontal roundness), frtAng (frontal angle), RAr increase (total row area increase) and ChN increase (chamberlet number increase).
Figure 10. Principal component analysis based on growth-independent and growth-invariant characters of neanic chamberlets and nepionts. Grouping by samples (point colours) and groups (background colour of convex hulls). Points with yellow margins represent the position of ‘L. group 2’ within ‘L. group 1’.

Specimens of samples CA-216-E3, CA-216-F3, P-559 (exclusive individual 514) and P-562 (exclusive individual 494) representing ‘L. group 5’ cover the same region in both PCA axes with an increasing dispersion of the younger sample along the first axis (). As in the cluster analysis, the outliers P-559(514) and P-562(494) belong to the ‘L. group 1’ ().
Component loadings explain the position of specimens in the axes system (). Positive values along the first axis determine high rates in the initial values of ‘total chamberlet height’, ‘distal chamberlet height’, ‘proximal chamberlet height’, and ‘chamberlet width’. Negative values along the first axis can be found in the increase of characters mentioned above, which documents a positive increase at low axis values versus a negative increase at high axis values (). Negative values in the first axis determine the intensity of chamberlet basal width decreasing to 0 at positive values (). Except for the characters ‘frontal roundness’, ‘frontal angle’ and the increase of the ‘total row area’, all remaining characters, especially those characterizing the nepiont, mark high character states at positive axis values ().
Figure 11. Loadings of the first and second axes in principal component analysis based on growth-independent and growth-invariant characters of neanic chamberlets and nepionts.

Loadings of the second principal component are less important (8.86% of total variance), but positive values indicate a stronger increase in chamberlet height characters and the basal width. The main consequence can be found in all protoconch characters, indicating higher values on the positive axis side ().
Calculating PCA restricted to neanic chamberlets characters changes the positions of groups (). The first and second component represent 66.9% of total variance. While ‘L. group 4’ comes in contact with ‘L. group 1’ at the second component, but does not overlap, ‘L. group 3’ approaches ‘L. group 5’ overlapping with the latter (). Therefore, L. group 3 is closer in neanic chamberlets to ‘L. group 5’ than to ‘L. group 1’ expressed in component 1, while ‘L. group 4’ is similar in the form of neanic chamberlets to ‘L. group 1’, but smaller as expressed in component 2.
Figure 12. Principal component analysis based on growth-invariant characters of neanic chamberlets. Grouping by samples (point colours) and groups (background colour of convex hulls). Points with yellow margins represent the position of ‘L. group 2’.

Restriction of PCA to nepiont characters leads to a dominance of the first axis (82.6%) compared to the second axis (12%). Both axes place ‘L. group 2’ within ‘L. group 1’, indicating similar size and proportion between proto- and deuteroconch (). Smaller size and similar proportions between proto- and deuteroconch result in the position of ‘L. group 3’ at negative values of component 2. The transition from the relation ‘larger protoconch/smaller deuteroconch’ to ‘smaller protoconch/larger deuteroconch’ is remarkable on the first component, with a clear transition zone between nepionts of ‘L. group 1’ and ‘L. group 5’ at low positive component values ().
Figure 13. Principal component analysis based on growth-independent characters of nepionts. Grouping by samples (point colours) and groups (background colour of convex hulls). Points with yellow margins represent the position of ‘L. group 2’.

Groups are clearly separated by discriminant analyses, where the first and second axes explain 88% of total variance (). The dominant first axis (67.6%) is significant positively loaded by all characters describing the nepiont, in particular the ‘total height of the protoconch’ (Hprot) and ‘heights at the largest widths’ (HW) of the proto- and deuteroconch (). For neanic chamberlet characters, the first axis clearly differentiates arcuate chamberlets with negative axis values (especially ‘basal chamberlet width’ basChW and ‘proximal chamberlet heights’ proxChH) from spatulate chamberlets possessing positive values (). ‘Lepidocylina group 5’ is positioned with positive values along the first axis, while ‘L. group 3’, located at the axis origin, overlaps, using the 95% confidence limits, with ‘L. group 1’, positioned at low negative axis values (). The second discriminant axis (20.3% total variance) is positively loaded by the neanic chamberlet characters ‘frontal roundness’ (frtRD), ‘frontal angle’ (frtAng) and the increase of the ‘distal chamberlet height’ (distChHincrease). High negative loadings of neanic chamberlet characters refers to the size of chamberlets (), while negative loadings of protoconch characters indicate large nepiont size. Lepidocyclina group 4 with small nepionts is thus located at high positive values of the second axis and does not overlap by 95% confidence limits neither with ‘L. group 1’ nor with ‘L. group 3’ (). Both specimens of L. group 2’ are clearly separated from the other groups in both discriminant axes (), mainly based on negative loadings of the characters ‘frontal roundness’ and ‘frontal angle’ (). This is caused by the small size of specimens that enforce the differentiation in initial chambers. In all PCA groupings () both specimens belong to ‘L. group 1’.
Figure 14. Discriminant analysis separating species. Ellipses point to the 95% confidence limits. Axis values of characters shown in .

Table 4. Loading of characters in discriminant axes 1 and 2. Checking for significance of non-correlation (H0) by t-test (bold characters).
Identification and differentiation
Growth-independent and growth-invariant characters were compared between groups that will be identified as species. Boxplots, the Kruskal-Wallis and the post-hoc Dunn test investigated differences between species (Supplemental material 4). The two specimens of ‘L. group 2’, identified as small specimens possibly belonging to L. macdonaldi are not significant for dispersion analysis, thus their character states must be regarded with caution or should be removed in the following analyses.
Regarding the character ‘total chamberlet height’, ‘L. group 5’, identified as L. ocalana (cf. Cushman, Citation1920; pl. 27, figs 1–4; pl. 28, figs 1–3), shows the highest values for the character state ‘initial heights’ (totalChHinit), not significantly different to ‘L. group 3’, identified as L. ariana (cf. Cole & Ponton, Citation1934; pl. 2, figs 1–5). The remaining ‘L. group 1’ = L. macdonaldi (cf. Cushman, 1919; pl. 40, figs 1–6) and ‘L. group 4’ = L. pustulosa (cf. Mitchell et al., Citation2022, figs 108, 109) demonstrate similar and significant lower values (). Similar differences can be detected by the ‘increase in total chamberlet height’ (totalChHincr), where a (positive) increase characterizes L. macdonaldi and L. pustulosa, in contrast to the significant decrease found in L. ocalana and L. ariana (, Supplemental material 4). Differences in the character ‘chamberlet height’ are shown in .
Figure 15. Box plots of neanic character states for the Lepidocyclina groups (species). Significant differences checked by the Kruskal-Wallis test. L. group 1 = L. macdonaldi, L. group 2 = L. ?macdonaldi (small specimens), L. group 3 = L. ariana, L. group 4 = L. pustulosa, L. group 5 = L. ocalana.

The character ‘distal chamberlet height’ demonstrates similar relations. Again, L. ocalana and L. ariana show the highest values for the character state ‘initial height’ (distChHinit), while L. macdonaldi possess significantly lower values. Lepidocyclina pustulosa differs from the other species in having the lowest values (, Supplemental material 4). In the character state ‘increase in distal chamberlet height’ (distChHincr), L. macdonaldi and L. pustulosa show a similar increase, while L. ariana and L. ocalana demonstrate a significant decrease (). Differences among species in the character ‘distal chamberlet height’ are shown in .
The character ‘proximal chamberlet height’ shows a trend consistent with the previous relationships between groups and species. Again, significant differences exist between L. ocalana and L. ariana with high values, and L. macdonaldi with medium values, while L. pustulosa shows the significantly lowest values in the character state ‘initial height’ (proxChHinit). The character state ‘height increase’ (proxChHincr) behaves quite similarly to the state for the distal chamberlet height, with an increase for L. macdonaldi (inclusive small individuals of group 2) and L. pustulosa and a decrease for L. ocalana and L. ariana (, Supplemental material 4). Differences among species in the character ‘proximal chamberlet height’ are shown in .
Differences between the character states distChHinit–proxChHinit and distChHincr–proxChHincr were tested for every species using Student’s t-tests (Supplemental material 5). Only in L. ocalana are the differences between distChHinit and proxChHinit significant, reflecting the presence of spatulate chamberlets, but differences in the character states distChHincr–proxChHincr are insignificant as in all other species (Supplemental material 5).
For the character ‘chamberlet width’, differences in the character state ‘initial width’ (ChWinit) are significantly higher for L. ocalana, similar values for L. macdonaldi and L. ariana, but significantly lower for L. pustulosa (, Supplemental material 4). The ‘increase in chamberlet width’ (ChWincr) follows the same trends as in all chamberlet height character states: significant increases in L. macdonaldi and L. pustulosa, and significant decreases in L. ariana and L. ocalana (, Supplemental material 4). Differences between species in the character ‘chamberlet width’ are shown in .
The growth-independent character ‘basal chamberlet width’ (basChW) separates subspecies L. macdonaldi and L. pustulosa, possessing arcuate chamberlets, from L. ariana and L. ocalana with spatulate chamberlets, where basal width is not present (, Supplemental material 4). From the two small L. macdonaldi (group 2) specimens, only individual 98LC-1i(642) has rhombic chamberlets (Adams, Citation1987) without basal width starting from the first row. Differences between species in the character ‘basal chamberlet width’ are shown in .
The strongest dissimilarity of both specimens of group 2 to the other species is expressed in the character ‘frontal roundness’ (frtRD) with the lowest values close to 1.0 (). Of the species with much higher values, indicating increasing roundness, L. ariana possesses the significantly highest values (, Supplemental material 4).
Flatness of the peripheral chamberlet part is expressed in the character ‘frontal angle’ (frtAng). Lepidocyclina pustulosa and L. ariana show the highest values, significantly different from the flat rounding in L. macdonaldi and L. ocalana (, Supplemental material 4).
The mean values of ‘chamberlet area’ follow a 2nd order polynomial regression that is characterized by three parameters (). Here the character states ‘initial chamberlet area’ (ChAr init) – the function values at the first row– the ‘extreme value of chamberlet area’ (ChAr extreme) and its ‘position along the rows’ (rowChAr extreme) are used. In the ‘initial chamberlet area’, Lepidocyclina pustulosa demonstrates the lowest values, while L. ariana and L. ocalana possess similar high values (, Supplemental material 4). The ‘extremes in chamberlet area’ particularly show the lowest values for Lepidocyclina pustulosa, while the remaining species do not differ in this character state (, Supplemental material 4). Differences between the ‘initial chamberlet area’ and the ‘extremes in chamberlet area’ are always negative, insignificant for Lepidocyclina pustulosa and extremely significant for L. ocalana (Supplemental material 4). There are no clear differences between species in the ‘row positions of the extremes’; only L. ocalana with the highest values differs from L. macdonaldi, characterized by the lowest values (, Supplemental material 4).
Figure 17. Box plots of neanic character states for the Lepidocyclina groups (species). Significant differences checked by the Kruskal-Wallis test. L. group 1 = L. macdonaldi, L. group 2 = L. ?macdonaldi (small specimens), L. group 3 = L. ariana, L. group 4 = L. pustulosa, L. group 5 = L. ocalana.

Similar relations between species can be shown in the character ‘total row area’. Lepidocyclina ocalana and L. ariana have similar high values in the character state ‘initial row area’ (RwAr init), which are significantly different from the other species (, Supplemental material 4). Similar values in the character state ‘increase in row areas’ (RwAr incr) can be found in all species except L. ocalana with significant lower values (, Supplemental material 4). Differences between species in the character ‘total row area’ are shown in .
The character ‘chamberlet numbers’ follows a linear regression (). Other than L. ariana and L. ocalana with similar highest values, the remaining species differ significantly among each other in the character state ‘function value at the 6th row’ (ChN row6), (, Supplemental material 4). These differences are also present in the character state ‘increase in chamberlet number’ (ChN incr). Differences between species in the character ‘chamberlet numbers’ are shown in .
The character ‘initial distance’ (initDist) correlates with the size of the nepiont. Lepidocyclina macdonaldi and L. ariana are of similar size, L. pustulosa is significantly smaller and L. ocalana is significantly larger (, Supplemental material 4). Differences between species in the character ‘distance to centre’ are shown in .
The nepiont behaves in a similar manner in all growth-independent characters. Differences in size between species are expressed by the character ‘central width’ (NWcentral). L. macdonaldi and L. ariana are not significantly different in this character. Lepidocyclina ocalana shows significantly higher values and L. pustulosa has the lowest values (, Supplemental material 4).
Figure 19. Box plots of nepiont character states for the Lepidocyclina groups (species). Significant differences checked by the Kruskal-Wallis test. L. group 1 = L. macdonaldi, L. group 2 = L. ?macdonaldi (small specimens), L. group 3 = L. ariana, L. group 4 = L. pustulosa, L. group 5 = L. ocalana.

Similar relations between species to those found in the character ‘central width’ are represented in all other nepiont characters (, Supplemental material 4). Beside the homogeneous characters in L. macdonaldi and L. ariana, L. pustulosa always demonstrates significant lower values, contrary to L. ocalana that is distinguished by the significant highest values in all characters describing the nepiont (, Supplemental material 4).
Because nepiont characters are not significantly different between species, differences between protoconch and deuteroconch characters are of interest. Comparing the heights HProt and HDeut, the latter is significantly smaller in L. macdonaldi and L. ariana; it is the same in L. pustulosa, and larger, but not significantly, in L. ocalana (Supplemental material 6). Comparing widths WProt and WDeut, only in L. ocalana the WDeut is significantly larger (Supplemental material 6). The ‘heights at widths’ character HWDeut is significantly smaller in L. macdonaldi and L. ariana, the same in L. pustulosa and larger, but not significantly, in L. ocalana (Supplemental material 6). Regarding hypotenuses, DHypo is significantly smaller than PHypo in L. macdonaldi and L. ariana, the same in L. pustulosa, and significantly larger in L. ocalana (Supplemental material 6). The same relationships can be found comparing areas PAr and DAr in groups and subgroups (Supplemental material 6).
Taxonomy
Naming the four morphologically homogeneous groups of the Cuban Eocene is based on a typological approach, where the original descriptions often lack or weakly represent the important characters of the equatorial sections, especially the nepionts. In addition to the differential diagnoses of the species, tables are presented here for each species with all character means and standard deviations combined with statistically significant differences between the identified species. Differences between species in chamberlet shape, size and arrangement are demonstrated by geometric constructions using the arithmetic means of character states ().
Figure 20. Geometric reconstruction of chamberlets based on the means of character states for the species ().

Genus Lepidocyclina Gümbel, Citation1868, emend. Adams (Citation1987)
Type species
Nummulites mantelli Morton, Citation1833.
Diagnosis
Lamellar-perforate, lenticular, orbitoidiform tests where the megalospheric generation is characterized by a two-chambered nepiont of variable size, consisting of a proto- and deuteroconch that are separated by a straight or slightly curved common wall. Two principal auxiliary chambers (PAC) positioned at both ends of the common nepiont wall, each one with two stolons, produce up to four spires with variable numbers of adauxiliary chambers and/or periembryonic chambers. An equatorial layer of numerous, radially to concentrically arranged chamberlets, which can be arcuate, rhombic, spatulate or hexagonal in shape, follows the nepionic stage. Two variable layers of lateral chamberlets are developed on each side of the equatorial plane.
Lepidocyclina macdonaldi Cushman, Citation1918
(, , 22A–G)
Figure 21. A–G, Lepidocyclina macdonaldi Cushman. Equatorial sections of megalospheric individuals. A, 98LC-1(640); B, 98LC-1(642); C, 98LC-1(616); D, 98LC-1(617); E, CA-215 (66); F, CA-215 (74); G, LM-23 (429); H, I, Lepidocyclina ariana Cole & Ponton. Equatorial sections of megalospheric individuals; H, LM-23(431); I, LM-23 (433). Loma Candela Formation (98LC-1), Arroyo Blanco Formation (CA-215) and Hatillo Formation (LM-23).

Table 5. L. group 1 = Lepidocyclina macdonaldi. Statistical parameters of characters and character states and comparison with related species: + averages significantly higher, – averages significantly lower. Symbols: +, – 0.05 > p(same) > 0.01; + +, – – 0.01 > p(same) > 0.00001; + + +, – – – 0.00001 > p(same).
1918 Lepidocyclina macdonaldi Cushman: 94, pl. 40, figs 1–6.
1920 Lepidocyclina macdonaldi Cushman; Cushman: 77, pl. 34, figs 1–3.
1952 Lepidocyclina (Pliolepidina) macdonaldi (Cushman); Cole: 16, pl. 7, figs 1–19, pl. 8, figs 1–4, pl. 14, fig. 11.
2022 Lepidocyclina macdonaldi Cushman; Mitchell et al.: 517, figs 86, 87.
Material
Twenty-five oriented equatorial sections of megalospheric specimens as follows: seventeen from Loma Candelaria section (98LC-1), one from San Miguel de los Baños (LM-23), five from Loma El Santo section (CA-215), and two from La Esperanza (P-559 and P-562).
Description
Lamellar-perforate, lenticular, orbitoidiform tests. Bilocular embryo characterized by a spherical protoconch and a deuteroconch; the common wall between the two chambers is straight (mean: D/P 0.575, Hprot 331 μm, Wprot 430 μm; Hdeut 216 μm, Wdeut 387 μm). Two large principal auxiliary chambers of similar size, semicircular in shape; each generates two spires of numerous periembryonic chambers of irregular size and shape. Equatorial chamberlets rhombic, missing contacts between row chamberlets documented by significant values for ‘basal chamber widths’ (, ), coming in contact in specimens from Jicotea Formation. After the cycle of larger periembryonic chambers, chamberlets are arranged in annular rows keeping heights constant during ontogeny, but with decreasing chamberlet widths (). Medium increase in row areas and distance to the centre, weak increase in chamberlet numbers. Numerous lateral chamberlets in axial sections. Parameters of characters and character states presented in with comparison to L. ariana, L. ocalana and L. pustulosa.
Occurrences
Middle to late Lutetian, Arroyo Blanco Formation; middle Eocene, Hatillo Formation; Bartonian to lower Priabonian, Loma Candela Formation; Priabonian, Jicotea Formation. ABZ 9 to ABZ 16.
Lepidocyclina ariana Cole & Ponton, Citation1934
(, )
Figure 22. Nepionts. A–G, Lepidocyclina macdonaldi Cushman. Equatorial sections of megalospheric individuals. A, 98LC-1(640); B, 98LC-1(642); C, 98LC-1(616); D, 98LC-1(617); E, CA-215 (66); F, CA-215 (74); G, LM-23 (429). H, I, Lepidocyclina ariana Cole & Ponton. Equatorial sections of megalospheric individuals; H, LM-23(431); I, LM-23 (433). Loma Candela Formation (98LC-1), Arroyo Blanco Formation (CA-215) and Hatillo Formation (LM-23).

Table 6. L. group 3 = Lepidocyclina ariana. Statistical parameters of characters and character states and comparison with related species: + averages significantly higher, – averages significantly lower. Symbols: +, – 0.05 > p(same) > 0.01; + +, – – 0.01 > p(same) > 0.00001; + + +, – – – 0.00001 > p(same).
1934 Lepidocyclina (Lepidocyclina) ariana Cole & Ponton: 142–143, pl. 2, figs 1–5.
1944 Lepidocyclina (Pliolepidina) ariana (Cole & Ponton); Cole: 61–62, pl. 1, fig. 14; pl. 13, fig. 3; pl. 14, figs 1–7; pl. 16, figs 11–13; pl. 17, fig. 13.
1945 Lepidocyclina (Pliolepidina) ariana (Cole & Ponton); Cole: 111–115, pl. 15, figs 1–3; pl. 17, figs 1–6; pl. 22, figs 9–14.
1974 Lepidocyclina macdonaldi Cushman; Frost & Langenheim: 147, pl. 40, figs 1–3, 7–12.
1981 Lepidocyclina (Neolepidina) ariana (Cole & Ponton); Butterlin: 210–211, pl. XLVII, figs 3–5.
2022 Lepidocyclina ariana Cole & Ponton; Mitchell et al.: 513–516, figs 81–84.
Material
Four oriented equatorial sections of megalospheric specimens from the locality San Miguel de los Baños (LM-23).
Description
Lamellar-perforate, lenticular, orbitoidiform tests. The nepiont distinguished by an ovate protoconch larger than the slightly reniform deuteroconch (mean D/P: 0.554, Hprot 358 μm, Wprot 481 μm; Hdeut 231 μm, Wdeut 445 μm); both chambers separated by an almost straight wall. Two spires of relatively large periembryonic chambers follow large PACs embracing the protoconch and deuteroconch. The equatorial plane is then characterized by approximately two cycles of irregular and large, sometimes undivided chamberlets, prior to a transition to cycles of equatorial chamberlets arranged in alternating annular rows. Short-spatulate equatorial chamberlets with decreasing mean distances between proximal and distal chamberlet height ranging from 5.1 μm (row 1) to 3.1 μm (row 50), additionally marked by the lack of basal chamberlet widths (); chamberlets varying little in size through ontogeny (). Rows strongly increase in area, chamberlet numbers and distance to the centre (). Axial sections similar to Eolepidina antillea with few and thick lateral chamberlets. Parameters of characters and character states presented in with comparison to L. macdonaldi, L. ocalana and L. pustulosa.
Occurrences
Middle Eocene, Hatillo Formation. ABZ 12.
Remarks
The middle Eocene species L. ariana and L. macdonaldi have often been regarded as synonymous since their equatorial morphologies are similar (Cole, Citation1944, Citation1945; Frost & Langenheim, Citation1974). Cole (Citation1956); Butterlin (Citation1981) and Mitchell et al. (Citation2022) separated these two species based on the difference in their lateral chamberlet shape; until then L. ariana had been separated from L. macdonaldi solely based on differences in lateral layers (Butterlin, Citation1981; Mitchell et al., Citation2022). Growth-independent characters of the nepiont show similar size and proportions between L. ariana and L. macdonaldi (). However, growth-independent and -invariant characters of neanic chamberlets, such as ‘distal chamberlet height increase’, ‘chamberlet width increase’, ‘basal chamberlet width’, ‘frontal roundness’, ‘frontal angle’ and ‘chamber number increase’, demonstrate significant differences between both species especially in the development of spatial chamberlets (, ).
Lepidocyclina ocalana Cushman, Citation1920
(, )
Figure 23. A–G, Lepidocyclina ocalana Cushman. Nepionts of megalospheric individuals. A, CA-216-E3(7a); B, CA-216-E3(7b); C, CA-216-F3(3b); D, P-559 (513); E, CA-216-F3(3a); F,CA-216-E3(12); G, CA-216-F3(3c). Blanco Formation (CA-216); Jicotea Formation (P-559).

Figure 24. A–D, Lepidocyclina ocalana Cushman. A, B, equatorial sections of megalospheric individuals; C, D, axial sections of megalospheric individuals. A, P-562(491); B, P-562(481); C, P-562(499); D, P-562(498). Jicotea Formation (P-562).

Figure 25. Lepidocyclina ocalana Cushman. Nepionts of megalospheric individuals. A, CA-216-E3(7a); B, CA-216-E3(7b); C, CA-216-E3(12); D, CA-216-F3(3a); E, CA-216-F3(3b); F, CA-216-F3(3c); G, P-559 (513); H, P-559 (509); I, P-562 (491). Blanco Formation (CA-216); Jicotea Formation (P-559; P-562).

Table 7. L. group 5 = Lepidocyclina ocalana. Statistical parameters of characters and character states and comparison with related species: + averages significantly higher, – averages significantly lower. Symbols: +, – 0.05 > p(same) > 0.01; + +, – – 0.01 > p(same) > 0.00001; + + +, – – – 0.00001 > p(same).
1920 Lepidocyclina ocalana Cushman: 70–71, pl. 27, figs 1–4; pl. 28, figs 1–3.
1941 Lepidocyclina ocalana Cushman; Cole: 41–43, pl. 13, figs 1–7; pl. 16, figs 1–4, 6–10.
2016 Lepidocyclina chaperi (Cushman); Molina et al.: 34, pl. 1, fig. 5.
2020 Lepidocyclina chaperi (Cushman); Hohenegger & Torres-Silva: 184, fig. 2.
2022 Lepidocyclina ocalana Cushman, Citation1920; Mitchell et al.: 519–521, figs 87–89.
Material
Nineteen oriented equatorial sections of megalospheric specimens were measured from the following localities: 11 from Loma Vigía (CA-216), three from La Esperanza (P-559) and five from La Esperanza (P-562).
Description
Lamellar-perforate, lenticular, orbitoidiform tests with a slightly umbonal swelling and thin margins, often broken off. Piles small, numerous, evenly distributed over the surface and of similar size. Pronounced dimorphism: microspheric forms up to 1.6 cm in equatorial and about 5 mm in axial diameter with a slight selliform deformation of the equatorial chamberlet layer. Megalospheric specimens with a sharp margin, often with a faint axial swelling and a stronger selliform deformation as compared to the microspheres. Megalospheres with large, two-chambered embryonic apparatus, with thin walls. Protoconch and deuteroconch almost equal in volume, the deuteroconch significantly larger in the equatorial plane, but smaller in the axial direction, sometimes compressed in the equatorial axis of the embryo (mean D/P: 1.394, Hprot 371 μm, Wprot 648 μm; Hdeut 423 μm, Wdeut 733 μm). Irregular arrangement of auxiliary and adauxiliary chamberlets. In particular, both auxiliary chamberlets are located at 90 degrees to the equatorial plane and may become very long, extending over a quarter of the circumference of the nepiont. Because of the irregular arrangement of apertures in the deuteroconch, the real number of adauxiliary chamberlets cannot be recognized in equatorial section. Neanic chamberlets spatulate, with decreasing mean distances between proximal and distal chamber heights from 21.8 μm (row 1) to 6.2 μm (row 5; ). Size of chamberlets decreases strongly during ontogeny, documented in both heights and widths (). The height of the chamberlets in the axial direction, determining the thickness of the equatorial chamberlet layer, does not increase in megalospheric, only slightly in microspheric forms (). Lateral chamberlets low, with round, sometimes vermicular outline as seen in sections tangential to the shell’s lateral surface, with thick coarsely perforated walls. Piles connected at the base of the lateral chamberlet layer, where the lumen of the latter is particularly low. In microspheric nepionts, 8–10 tiny spiral chambers are followed by about three cycles of conspicuously low chamberlets prior to a transition to ordinary cycles of spatulate neanic chamberlets. Statistical parameters of characters and character states of megalospheres are presented in with comparison to L. macdonaldi, L. ariana and L. pustulosa.
Occurrences
Priabonian, Blanco and Jicotea formations. ABZ 15 to ABZ 16.
Remarks
L. ocalana is clearly separated from the species L. pustulosa and L. macdonaldi by almost all characters describing the equatorial morphology ( and ). Neanic chamberlet affinities between L. ocalana and L. ariana are expressed by the characters ‘total’, ‘distal’ and ‘proximal chamberlet height’, ‘basal chamberlet width’, ‘chamberlet area’, ‘total row area’ and ‘chamberlet numbers’ that reflect in both species the spatulate shape of neanic chamberlets.
Table 8. L. group 4 = Lepidocyclina pustulosa. Statistical parameters of characters and character states and comparison with related species: + averages significantly higher, – averages significantly lower. Symbols: +, – 0.05 > p(same) > 0.01; + +, – – 0.01 > p(same) > 0.00001; + + +, – – – 0.00001 > p(same).
Lepidocyclina pustulosa (Douvillé, Citation1917)
(, )
Figure 26. Lepidocyclina pustulosa (H. Douvillé). A–C, equatorial sections of megalospheric individuals; D–F, axial sections of megalospheric individuals; G–I, nepiont enlargement. A, G, LM-52(734); B, I, LM-52(738); C, H, LM-52(735); D, LM-52(742); E, LM-52(744); F, LM-52(751). Jabaco Formation (LM-52).

1917 Isolepidina pustulosa Douvillé: 844, figs 1–4.
1974 Lepidocyclina (Lepidocyclina) pustulosa (Douvillé); Frost & Langenheim: 131, pl. 33, figs 1–6.
1981 Lepidocyclina (Neolepidina) pustulosa (Douvillé); Butterlin: 213, pl. 48, figs 5, 6.
1993 Lepidocyclina pustulosa Douvillé; Robinson &Wright: 317, figs 23.1, 23.3.
2022 Orbitoina wrighti Mitchell et al.: 542–543, figs 108, 109.
Material
Eight equatorial sections of megalospheric specimens were measured, six from Loma Jabaco (LM-52) and two from Loma Candelaria section (98LC-1).
Description
Lamellar-perforate, lenticular, strongly biconvex, orbitoidiform tests with the surface centrum covered by coarse pustules. Pronounced dimorphism, microspheric generation up to three times larger than the megalospheres. Megalospheric specimens with a small to medium-sized embryonic apparatus; protoconch and deuteroconch roughly equal in size (isolepidine, mean D/P ratio: 0.998, Hprot 204 μm, Wprot 272 μm; Hdeut 187 μm, Wdeut 295 μm), hemispherical in shape and joined by an almost straight common wall. Two large PAC of similar size and semicircular in shape followed by four spires of numerous periembryonic chamberlets of irregular size and shape. Equatorial chamberlets arcuate, unconnected within rows as documented by significant ‘basal chamberlet widths’ (); arranged in intersecting curves with about 2 cycles of irregular and larger chamberlets prior to a transition to ordinary cycles of arcuate chamberlets. Equatorial chamberlets slightly increasing in height and width (), clearly observable in axial sections, where the equatorial layer height increases to the test periphery (). Rows increase weakly in areas and distances to the centre, stronger in chamberlet numbers (). Lateral chamberlets open, lenticular, with floors and roofs slightly arched and arranged in well-defined tiers with up to 11 chamberlets per tier. Central part of the test marked by large and conical pillars, which extend from the nepiont up to the test surface. Statistical parameters of characters and character states presented in with comparison to L. macdonaldi, L. ocalana and L. ariana.
Occurrences
Bartonian to Priabonian, Loma Candela and Jabaco formations. ABZ 12 to ABZ 16.
Remarks
The name L. pustulosa is used to describe morphotypes with small isolepidine nepionts and arcuate neanic chamberlets. Lepidocyclina pustulosa is probably the most reported Lepidocyclina species in the American region (Butterlin, Citation1981; Cole, Citation1963; Eames et al., Citation1962; Frost & Langenheim, Citation1974; Robinson & Wright, Citation1993; Vaughan & Cole, Citation1941) because of its uncertain status, mainly caused by the incomplete type description. It has further been referred to as synonyms of L. peruviana, L. cedarkeyensis, L. trinitatis, L. rdouvillei (Cole, Citation1963; Vaughan & Cole, Citation1941). Characters and character states of the equatorial morphology showed by far the strongest differences with each of the species investigated in this study (, ). This may strengthen the classification of the ‘pustulosa morphotype’ into the genus Orbitoina as has been recently proposed by Mitchell et al. (Citation2022). Our specimens closely match Jamaican specimens described by Mitchell et al. (Citation2022) under Orbitoina wrighti.
Biostratigraphy
To improve the correlation of Eocene shallow-marine deposits across sections from Cuba, we correlate the Lepidocyclina occurrences with the larger benthic foraminiferal zonation for the Eocene of Caribbean and Central America (ABZ) recently proposed by Mitchell et al. (Citation2022).
Lepidocyclina ariana occurs at San Miguel de los Baños locality, site label LM-23 () associated with Operculinoides floridensis, Eoconuloides parvulus, Pseudophragmina sp. and Fallotella sp. This assemblage encompasses the planktic foraminifer Morozovelloides lehneri indicating Zone P 12, which is in agreement with ABZ 12 ().
Figure 27. Stratigraphical ranges of the lepidocyclinids species at the Cuban sections and their correlation with the American Larger Foraminifera Zones (ABZ) proposed by Mitchel et al. (2022). ABZ zonation scheme calibrated against planktic foraminifera and calcareous nannofossils zonations along with Shallow Benthic Zonation (Mitchell et al., Citation2022).

Lepidocyclina macdonaldi occurs in Loma El Santo section, site label CA-215 (). The LBF assemblages consist of Nummulites striatoreticulatus, Operculinoides floridensis, O. soldadensis, Cushmania americana, Fallotella floridana, Amphistegina parvula, Asterocyclina havanensis, Discocyclina marginata and Fabiania cassis (Torres-Silva et al., Citation2019) (). The most characteristic planktic foraminiferal species are Hantkenina alabamensis, Morozovella aragonensis, Globigerinatheka mexicana, Igorina brodermani, Pseudohastigerina micra and Acarinina sp. Following Pearson et al. (Citation2006), zones E10/E11 (Lutetian, middle Eocene) were identified. These biozones correlate with the P12 zone (Berggren et al., Citation1995). The most characteristic species of calcareous nannofossils are Sphenolithus furcatolithoides, S. cuniculus and Reticulofenestra umbilicus. Stratigraphical attribution to NP16 Zone (Martini, Citation1971) is based on the absence of Blackites gladius (Locker, Citation1967; Varol, Citation1989) and the presence of R. umbilicus. More precise biostratigraphical attribution allows the zonation defined by Agnini et al. (Citation2014). The section can be attributed to Zone CNE13 (Reticulofenestra umbilicus Base Zone) with an estimated age from 43.06 Ma to 42.37 Ma (Torres-Silva et al., Citation2019). Based on these results, L. macdonaldi can be assigned to ABZ 9.
Lepidocyclina macdonaldi occurs also at Loma Candelaria section (site label 98LC-1). The larger foraminiferal assemblage consists of Nummulites striatoreticulatus, Palaeonummulites trinitatensis, Operculinoides floridensis, O. willcoxi, Asterocyclina minima and Heterostegina cubana (). According to the calcareous nanofossil assemblage, this section can be assigned to NP 16-17 based on the occurrences of Discoaster barbadensis, Calusiococcus fenestratus, Braarudosphaera bigelowii, Sphenolitus radians, Reticulofenestra wadae, R. dictyoda, Cyclicargolithus floridanus, Cocolithus eopelagicus, Chiasmolithus nitudus and Blackites spinosus.
A few specimens of L. macdonaldi could be detected at the localities P-559 and P-562. Taking into consideration the occurrences of L. macdonadi with Heterostegina cubana, the latter restricted to the upper Bartonian to early Priabonian, we propose an age comprised within ABZ 13 to ABZ 15. Mitchell et al. (Citation2022) established an ABZ 14 to ABZ 16 stratigraphical range for L. macdonaldi, but we here extend the range from ABZ 9 to ABZ 16 ().
Lepidocyclina ocalana occurs at Loma Vigia section (Site label CA-216) associated with Heterostegina ocalana, Operculinoides floridensis, Asterocyclina minima, Amphistegina cubensis and Fallotella sp. (). The stratigraphical attribution of calcareous nannofossils to NP 17 to NP 19 (Martini, Citation1971) is based on the presence of species Cyclicargolithus floridanus, Braarudosphaera bigelowi, Reticulofenestra dictyoda, Pontosphaera multipora, Cibrocentrum reticulaum, Coronocyclus nitescens and Cocolithus formosus.
Lepidocyclina ocalana was also recognized at the localities P-559 and P-562, both associated with Heterostegina ocalana and L. pustulosa. According to Mitchell et al. (Citation2022), L. ocalana ranges from ABZ 13 to ABZ 16. At the Cuban sections this species can be attributed to ABZ 15 to ABZ 16 ().
Lepidocyclina pustulosa occurs as a few specimens at the Loma Candelaria section (site label 98LC-1) and dominates at the type locality of Jabaco Formation (site label LM-52) from ABZ 15 to ABZ 16 (). This is supported by the NP19-20/CP 15 calcareous nanofossils assemblage reported by Torres-Silva et al. (Citation2017) (). L. pustulosa is associated with Heterostegina ocalana, Operculinoides floridensis, Asterocyclina nassauensis, Fallotella cookei and Amphistegina cubensis (Torres-Silva et al., Citation2017). At the Cuban sections this species ranges from ABZ 12 to ABZ 16.
Phylogenetic relationships
After determining species based on growth-independent and growth-invariant characters from the investigated sections and locations, significant differences within species were tested. Discriminant analysis strengthens the differences within species and allows distinction between evolutionary and environmental effects in fossil forms, as have been seen in the fossil nummulitid from the Eocene of Cuba (Hohenegger et al., Citation2022; Torres-Silva et al., Citation2017, Citation2019). Benedetti et al. (Citation2010) and Benedetti and Pignatti (Citation2013) suggested a variation of embryo size with depth in the tethyan Nephrolepidina. Schiavinotto and Benedetti (Citation2022) and Benedetti and Schiavinotto (Citation2023) validated the importance of the degree of stolonic distalization (Factor FD5) for the evolutionary steps in the genus Nephrolepidina. However, related extant LBF for testing lepidocyclinid test morphology variations along environmental gradients are absent. Therefore, the causes of morphologic variations are sometimes more difficult to explain than species delimitation itself.
Significant character differences within L. macdonaldi were found between localities 98LC-1 and CA- 215 that represent different stratigraphical levels within the Eocene (). Significant character differences of L. macdonaldi cannot be found between samples 98LC-1h and 98LC-1i (same locality), while specimens in sample CA-215-17 are significantly larger in the characters ‘basal chamber width’, ‘chamber area’, ‘chamber number of the 6th row of neanic chambers, and the ‘central width of the nepiont’ (). Specimens from localities P-559 and P-562 are differentiated from the older specimens by arcuated chamberlets that maintain contact in rows and an increase in central chamberlet width in contrast to a decrease of this character state in the older specimens. The main differences with respect to the other samples are the significantly higher values in almost all nepiont measurements (). These differences can be changes in morphology through time, or environmental changes. The increase in nepiont measurements () suggests a stratigraphical dependence, thus an evolutionary effect.
Table 9. Lepidocyclina macdonaldi. Statistical parameters of characters and character states between samples. Bold numbers document significant differences with < 5% error probability.
At localities CA-216, P-559 and P-562 significant character differences of L. ocalana are documented between the homogeneous CA-216-E3 with CA-216-F3 and the homogeneous P-559 with P-562 (). In neanic chamberlets, the latter group shows larger chamberlets combined with chamberlets and row areas and smoother final angles (). Regarding measurements of the nepiont, samples P-559 and P-562 are larger in the central and protoconch widths, also in height and width of the deuteroconch. Specimens of sample P-559 possess the largest deuteroconchs (). All localities with L. ocalana are similar in age and range from ABZ15 to ABZ 16 (Priabonian), implying that the sources of the morphologic differences are more likely environmental changes. Strong affinities of almost all characters and characters states of the neanic chamberlets between L. ariana and L. ocalana may strengthen their possible phylogenetic connections. Strong differences between L. ariana and L. macdonaldi in the characters and characters states of neanic chamberlets signal their importance in discerning species with similar nepiont morphology.
Table 10. Lepidocyclina ocalana. Statistical parameters of characters and character states between samples. Bold numbers document significant differences with < 5% error probability.
Conclusions
The morphometric investigation of equatorial sections in lepidocyclinid tests presented here led to groupings based on similarities. Eleven growth-independent characters for the nepiont and 19 growth-independent and growth-invariant characters describing neanic chamberlets provide a complete geometric reconstruction of the lepidocyclinid equatorial morphology. The four homogenous groups were identified as Lepidocyclina macdonaldi, L. ariana, L. ocalana and L. pustulosa. Differentiation into arcuate and short spatulate neanic chamberlets were relevant to separate the species L. macdonaldi from L. ariana possessing similar nepiont morphologies. A phylogenetic connection between L. ariana and L. ocalana is suggested by similarities in almost all characters and characters states characterizing neanic chamberlets, but the D/P ratio strongly differ between both species (D/P 1 in L. ariana, D/P
1 in L. ocalana). Subgroups within L. macdonaldi on the one side and L. ocalana on the other describing nepiont and neanic chamberlets explain the influence of environmental factors, therefore reaffirming the ecological species concept.
At Cuban localities, the middle Eocene is characterized by the species L. macdonaldi, L. ariana and L. pustulosa. The occurrence of L. macdonaldi at ABZ 9 marked the first appearance of Lepidocyclina in Cuba. This species has the longest stratigraphical range occurring from ABZ 9 to ABZ 16 and is by far the most abundant species. Lepidocyclina ariana is restricted to ABZ 12, while L. pustulosa occurs from ABZ 12 to ABZ 16. L. ocalana is typical for the Priabonian ranging from ABZ 15 to ABZ 16. The integration of our biostratigraphical results into the Eocene American Larger Benthic Foraminifera Zonation (ABZ) proposed by Mitchell et al. (Citation2022) could improve the correlation of Eocene deposits across America and allows further standardization between regions and discrimination between local, regional and global trends.
Associate Editor: Vincent Perrichot
Supplemental Material 1
Download MS Excel (9.5 KB)Supplemental Material 2
Download MS Excel (33.4 KB)Supplemental Material 3
Download MS Excel (34.9 KB)Supplemental Material 4
Download MS Excel (18.8 KB)Supplemental Material 5
Download MS Excel (13.5 KB)Supplemental Material 6
Download MS Excel (20.7 KB)Acknowledgements
This paper was funded by the Austrian Science Fund (FWF) project P 32722-B: ‘Phylogenetic and Palaeo-biogeographical Tendencies of Lepidocyclinids (Foraminifera) in the Caribbean Realm based on Morphometric Characters’ and by the North-South Dialogue scholarship of the Austrian Exchange Service (OeAD). We thank Consuelo Dıaz Otero, Dora Garcıa Delgado and Manuel Iturralde Vinent (Cuba) for the help in sampling the Cuban sections. We thank our former working group on larger foraminifera at the Institute of Paleontology, University of Vienna, consisting of Antonino Briguglio (Genova, Italy), Wolfgang Eder (Vienna), Shunichi Kinoshita (Tsukuba, Japan) and Julia Wöger (Vienna) for many discussions and helpful hints. Further, we thank Stjepan Ćorić (Geological Survey of Austria) for his assistance in species and biozone recognition of calcareous nannofossils and Christian Baal (Vienna University) for his help with the specimen photographs.
Disclosure statement
No potential conflict of interest was reported by the authors.
Supplemental material
Supplemental material for this article can be accessed here: https://doi.org/10.1080/14772019.2023.2287728.
References
- Adams, C. G. (1983). Speciation, phylogenesis, tectonism, climate and eustasy: factors in the evolution of Cenozoic larger foraminiferal bioprovinces. The Emergence of the Biosphere: Systematic Association, 23, 255–289.
- Adams, C. G. (1987). On the classification of the Lepidocyclinidae (Foraminiferida) with redescriptions of the unrelated Paleocene genera Actinosiphon and Orbitosiphon. Micropaleontology, 33, 289–317. https://doi.org/10.2307/1485571
- Agnini, C., Fornaciari, E., Raffi, I., Catanzariti, R., Pälike, H., Backman, J., & Rio, D. (2014). Biozonation and biochronology of Paleogene calcareous nannofossils from low and middle latitudes. Newsletters on Stratigraphy, 47(2), 131–181. https://doi.org/10.1127/0078-0421/2014/0042
- Benedetti, A., Di Carlo, M., & Pignatti, J. (2010). Embryo size variation in larger foraminiferal lineages: stratigraphy versus paleoecology in Nephrolepidina praemarginata (R. Douvillé, 1908) from the Majella Mt. (Central Apennines). Journal of Mediterranean Earth Sciences, 2, 19–29.
- Benedetti, A. & Pignatti, J. (2013). Conflicting evolutionary and biostratigraphical trends in Nephrolepidina praemarginata (Douvillé, 1908) (Foraminiferida). Historical Biology, 25, 363–383. https://doi.org/10.1080/08912963.2012.713949
- Benedetti, A. & Schiavinotto, F. (2023). Evolutionary trends in the Mediterranean Nephrolepidina: new chronosubspecies and biostratigraphic constraints. Historical Biology, 35(4), 518–536. https://doi.org/10.1080/08912963.2022.2054711
- Berggren, W. A., Kent, D. V., Swisher III, C. C., & Aubry, M. P. (1995). A revised Cenozoic geochronology and chronostratigraphy. Geocronology Timescales and Global Stratigraphic Correlation, SEPM Special Publication, 54, 129–212.
- BouDagher-Fadel M. K., & Price, G. D. (2010). Evolution and paleogeographic distribution of the lepidocyclinids. Journal of Foraminiferal Research, 40, 79–108. https://doi.org/10.2113/gsjfr.40.1.79
- Brönnimann, P., & Rigassi, D. (1963). Contribution to the geology and paleontology of the area of the city of La Habana, Cuba, and its surroundings. Eclogae Geologicae Helvetiae, 56(1), 193–480.
- Butterlin, J. (1981). Claves para la determinación de macroforaminíferos de México y del Caribe, del Cretácico Superior al Mioceno Medio. Instituto Mexicano del Petróleo.
- Butterlin, J. (1987). Origine et évolution des Lépidocyclines de la région des Caraıbes. Comparaisons et relations avec les Lépidocyclines des autres regions du Monde. Revue de Micropaléontologie, 29, 203–219.
- Caudri, C. M. B. (1996). The larger Foraminifera of Trinidad (West Indies). Eclogae Geologicae Helvetiae, 89(3), 1137–1310.
- Chaproniere, G. C. H. (1980). Biometrical studies of early Neogene larger Foraminiferida from Australia and New Zealand. Alcheringa, 4, 153–181. https://doi.org/10.1080/03115518008618929
- Cole, W. S. (1941). Stratigraphic and paleontologic studies of wells in Florida. Florida Geological Survey Bulletin, 19, 1–53.
- Cole, W. S. (1944). Stratigraphic and paleontologic studies of wells in Florida No. 3. Florida Geological Survey Bulletin, 29, 1–168.
- Cole, W. S. (1945). Stratigraphic and paleontologic studies of wells in Florida No. 4. Florida Geological Survey Bulletin, 28, 1–160.
- Cole, W. S. (1952). Eocene and Oligocene larger foraminifera from the Panama Canal Zone and vicinity. US Geological Survey Professional Paper, 244, 1–41.
- Cole, W. S. (1956). Jamaican larger Foraminifera. Bulletins of American Paleontology, 36, 203–233.
- Cole, W. S. (1960). Variability in embryonic chambers of Lepidocyclina. Micropaleontology, 6, 133–144. https://doi.org/10.2307/1484465
- Cole, W. S. (1963). Illustrations of the conflicting interpretations of the biology and classification of certain larger Foraminifera. Bulletins of American Paleontology, 46, 6–63.
- Cole, W. S., & Ponton, G. M. (1934). New species of Fabularia, Asterocyclina, and Lepidocyclina from the Florida Eocene. American Midland Naturalist, 15(2), 138–147. https://doi.org/10.2307/2420242
- Coletti, G., Bosio, G., Collareta, A., Malinverno, E., Bracchi, V. A., Di Celma, C., Basso, D., Stainbank, S., Spezzaferri, S., Cannings, T. & Bianucci, G. (2019). Biostratigraphic, evolutionary, and paleoenvironmental significance of the southernmost lepidocyclinids of the Pacific coast of South America (East Pisco Basin, southern Peru). Journal of South American Earth Sciences, 96, 102372. https://doi.org/10.1016/j.jsames.2019.102372
- Cushman, J. A. (1918). The larger fossil foraminifera of the Panama Canal Zone. Bulletin of the US National Museum, 103, 89–102.
- Cushman, J. A. (1920). The American species of Orthophragmina and Lepidocyclina. USGS Professional Paper, 125-D, 39–105.
- de Mello e Sousa, S. H., Fairchild, T. R., & Tibana, P. (2003). Cenozoic biostratigraphy of larger foraminifera from the Foz do Amazonas basin, Brazil. Micropaleontology, 49(3), 253–266. https://doi.org/10.2113/49.3.253
- Douvillé, H. (1917). Les Orbitoides de l'Ile de la Trinité. Comptes Rendus hebdomadaires des Séances de l'Académie des Sciences, 164, 841–849.
- Drooger, C. W. (1993). Radial Foraminifera; morphometrics and evolution: Verhandelingen der Koninklijke Akademie van Wetenschappen. Afdeeling Natuurkunde. Eerste Reeks, 41, 1–242.
- Drooger, C. W., & Freudenthal, T. (1964). Associations of Miogypsina and Lepidocyclina at some European localities. Eclogae geologica helvetica, 57, 509–528.
- Dunn, O. J. (1964). Multiple comparisons using rank sums. Technometrics, 6, 241–252. https://doi.org/10.1080/00401706.1964.10490181
- Eames, F. E., Banner, F. T., Blow, W. H., & Clarke, W. J. (1962). Fundamentals of mid-Tertiary stratigraphical correlation. Cambridge University Press.
- Fisher, R. A. (1936). The use of multiple measurements in taxonomic problems. Annals of Eugenics, 7(2), 179–188. https://doi.org/10.1111/j.1469-1809.1936.tb02137.x
- Frost, S. H., & Langenheim, R. L. (1974). Cenozoic reef biofacies. Tertiary larger foraminifera and scleractinian corals from Chiapas, Mexico. Northern Illinois University Press, DeKalb.
- García-Delgado, D., & Torres-Silva, A. I. (1997). Sistema Paleógeno. In K. E. Nuñez & G. F. Furrazola-Bermúdez (Eds.), Estudios sobre Geología de Cuba (pp. 115–140). Centro Nacional de Información Geológica.
- Grimsdale, T. F. (1959). Evolution in the American Lepidocyclinidae (Cainozoic Foraminifera): An interim view. Proceedings Koninklijke Nederlandse Akademie van Wetenschappen, Amsterdam, Series B, 62, 8–33.
- Gümbel, C. W. (1868). Beiträge zur Foraminiferenfauna der Nordalpinen Eocängebilde. Abhandlungen der Mathematisch-Physicalischen Classe der Koniglich Bayerischen Akademie der Wissenschaften, 10, 581–730.
- Hammer, Ø. (2021). PAST. Paleontological Statistics (Version 4.06). Natural History Museum, University Oslo.
- Hohenegger, J. (2011). Growth-invariant meristic characters tools to reveal phylogenetic relationships in Nummulitidae (Foraminifera). Turkish Journal of Earth Sciences, 20(6), 655–681. https://doi.org/10.3906/yer-0910-43
- Hohenegger, J. (2014). Species as the basic units in evolution and biodiversity: Recognition of species in the Recent and geological past as exemplified by larger foraminifera. Gondwana Research, 25(2), 707–728. https://doi.org/10.1016/j.gr.2013.09.009
- Hohenegger, J., & Torres-Silva, A. I. (2017). Growth-invariant and growth-independent characters in equatorial sections of Heterostegina shells relieve phylogenetic and paleobiogeographic interpretations. Palaios, 32(1), 30–43. https://doi.org/10.2110/palo.2015.092
- Hohenegger, J., & Torres-Silva, A. I. (2020). Methods for testing ontogenetic changes of neanic chamberlets in lepidocyclinids. Journal of Foraminiferal Research, 50(2), 182–194. https://doi.org/10.2113/gsjfr.50.2.182
- Hohenegger, J., Torres-Silva, A. I., & Eder, W. (2022). Interpreting morphologically homogeneous (Paleo-)populations as ecological species enables comparison of living and fossil organism groups, exemplified by nummulitid foraminifera. Journal of Earth Science, 33, 1362–1377. https://doi.org/10.1007/s12583-021-1567-z
- Hotelling, H. (1933). Analysis of a complex of statistical variables into principal components. Journal of Educational Psychology, 24, 417–441, 498–520. https://doi.org/10.1037/h0070888
- Hottinger, L. (1997). Shallow benthic foraminiferal assemblages as signals for depth of their deposition and their limitations. Bulletin de la Société géologique de France, 168(4), 491–505.
- Hottinger, L. (1998). Shallow benthic foraminifera at the Paleocene-Eocene boundary. Strata, 9, 61–64.
- Iturralde‐Vinent, M. A. (1994). Cuban geology: a new plate‐tectonic synthesis. Journal of Petroleum Geology, 17(1), 39–69. https://doi.org/10.1111/j.1747-5457.1994.tb00113.x
- Iturralde‐Vinent, M. A. (1995). Cuencas sedimentarias del Paleoceno-Eoceno de Cuba. Boletín de la Sociedad Venezolana de Geología, 20(1–2), 75–80.
- Kantshev, I., Boyanov, I., Popov, N., Cabrera, R., Goranov, A., Iolkicev, I., Kanszirski, M., & Stancheva, M. (1976). Geología de la provincia de Las Villas. Resultado de las investigaciones y levantamiento geológico a escala, 1:250 000 [Unpublished report]. Academia de Ciencias de Cuba y Bulgaria, Instituto de Geología y Paleontología.
- Less, G., Frijia, G., Özcan, E., Saraswati, P. K., Parente, M., & Kumar, P. (2018). Nummulitids, lepidocyclinids and Sr-isotope data from the Oligocene of Kutch (western India) with chronostratigraphic and paleobiogeographic evaluations. Geodinamica Acta, 30, 183–211. https://doi.org/10.1080/09853111.2018.1465214
- Locker, S. (1967). Neue Coccolithophoriden (Flagellata) aus dem Alttertiär Norddeutschlands. Geologie, 16, 361–364.
- Martini, E. (1971). Standard Tertiary and Quaternary calcareous nannoplankton zonation. In A. Farinacci (Ed.), Proceedings of the Second Planktonic Conference, Roma 1970 (pp. 739–785). Tecnoscienza.
- Mitchell, S. F., Robinson, E., Özcan, E., Jiang, M. M., & Robinson, N. (2022). A larger benthic foraminiferal zonation for the Eocene of the Caribbean and Central American region. Carnets de Géologie, 22(11), 409–565. https://doi.org/10.2110/carnets.2022.2211
- Molina, E., Torres-Silva, A. I., Ćorić, S., & Briguglio, A. (2016). Integrated biostratigraphy across the Eocene/Oligocene boundary at Noroña, Cuba, and the question of the extinction of orthophragminids. Newsletters on Stratigraphy, 49(1), 27–40. https://doi.org/10.1127/nos/2015/0069
- Morton S. G. (1833). Supplement to the "Synopsis of the organic remains of the ferruginous sand formation of the United States," contained in vols. XVII and XVIII of this journal. American Journal of Science and Arts, 23, 288–294.
- Orue-Etxebarria, X., Pujalte, V., Bernola, G., Apellaniz, E., Baceta, J. I., Payros, A., & Tosquella, J. (2001). Did the Late Paleocene thermal maximum affect the evolution of larger foraminifers? Evidence from calcareous plankton of the Campo Section (Pyrenees, Spain). Marine Micropaleontology, 41(1), 45–71. https://doi.org/10.1016/S0377-8398(00)00052-9
- Pearson, P. N., Olsson, R. K., Huber, B. T., Hemleben C., & Breggren, W. A. (Eds.). (2006). Atlas of Eocene planktonic Foraminifera. Cushman Foundation for Foraminiferal Research, Special Publication, 41, 513 pp.
- Robinson, E. (1996). Using larger foraminifers in high resolution biostratigraphy: an example from the Eocene of the Gulf of Mexico and northern Caribbean. Palaios, 11(3), 220–229. https://doi.org/10.2307/3515231
- Robinson, E. (2004). Zoning the White Limestone Group of Jamaica using larger foraminiferal genera: a review and proposal. In S. K. Donovan (Ed.), The Mid-Cainozoic White Limestone Group of Jamaica (pp. 39–75) Cainozoic Research, 3(1–2).
- Robinson, E., & Wright, R. M. (1993). Jamaican Paleogene larger foraminifera. In R. M. Wright & E. Robinson (Eds.), Biostratigraphy of Jamaica (pp. 283–345). Geological Society of America Memoir.
- Saraswati, P. K. (1994). Biometric study of Lepidocyclina (Nephrolepidina) from Kutch, Saurashtra and Quilon (India). Geological Society of India, 44, 79–90.
- Saraswati, P. K. (1995). Biometry of early Oligocene Lepidocyclina from Kutch, India. Marine Micropaleontology, 26, 303–311. https://doi.org/10.1016/0377-8398(95)00018-6
- Scheibner, C., Speijer, R. P., & Marzouk, A. M. (2005). Turnover of larger foraminifera during the Paleocene-Eocene Thermal Maximum and paleoclimatic control on the evolution of platform ecosystems. Geology, 33, 493–496. https://doi.org/10.1130/G21237.1
- Schiavinotto, F. (1994a). Biometry of the neanic stage of Upper Chattian Nephrolepidina morgani (Lemoine & R. Douvillé). Geologica Romana, 29, 291–306.
- Schiavinotto, F. (1994b). Neanic state biometry in Nephrolepidina praemarginata (R. Douvillé, 908). Bollettino della Società Geologica Italiana, 112, 805–824.
- Schiavinotto, F. (2010). Neanic stage biometry in Nephrolepidina from the upper Oligocene of lonedo (lugo di Vicenza–northern Italy). Bollettino della Società Paleontologica Italiana, 49, 173–194.
- Schiavinotto, F. (2016). Neanic acceleration in Nephrolepidina from the Oligo-Miocene Mt. Torretta section (L’Aquila, central Apennines): biometric results and evolutionary, taxonomic and biostratigraphic remarks. Journal of Mediterranean Earth Sciences, 8, 63–87.
- Schiavinotto, F., & Benedetti, A. (2022). Nephrolepidina and unispiralled Miogypsinidae from the Oligo-Miocene toe-of-slope succession of Gran Sasso (L'Aquila, Central Apennines-Italy): biometric and evolutionary remarks. Micropaleontology, 67(5), 483–514. https://doi.org/10.47894/mpal.67.5.04
- Serra-Kiel, J., Hottinger, L., Caus, E., Drobne, K., Ferrández, C., Jauhri, A. K., Less, G., Pavlovec, R., Pignatti, J., Samso, J. M., Schaub, H., Sirel, E., Strougo, A., Tambareau, Y., Tosquella, J., & Zakrevskaya, E. (1998). Larger foraminiferal biostratigraphy of the Tethyan Paleocene and Eocene. Bulletin de la Société géologique de France, 169(2), 281–299.
- Sirotti, A. (1982). Phylogenetic classification of Lepidocyclinidae: a proposal. Bollettino della Societa Paleontologica Italiana, 21, 99–112.
- Tan, S. H. (1936). Lepidocyclina zeijlmansi nov. sp., eine poly-lepidine Orbitoidide von Zentral-Borneo, nebst Bemerkungen über die verschiedenen Einteilungsweisen der Lepidocyclinen. Ingenieur in Nederlandsch-Indie, Mijnbouw en Geologie, 1, 7–14.
- Torres-Silva, A. I., Hohenegger, J., Ćorić, S., Briguglio, A., & Eder, W. (2017). Biostratigraphy and evolutionary tendencies of Eocene heterostegines in Western and Central Cuba based on morphometric analyses. Palaios, 32(1), 44–60. https://doi.org/10.2110/palo.2016.004
- Torres-Silva, A. I., Eder, W., Hohenegger, J., & Briguglio, A. (2019). Morphometric analysis of Eocene nummulitids in western and central Cuba: taxonomy, biostratigraphy and evolutionary. Journal of Systematic Palaeontology, 17(7), 557–595. https://doi.org/10.1080/14772019.2018.1446462
- Van der Vlerk, I. M. (1959). Problems and principles of Tertiary and Quaternary stratigraphy: Quarterly Journal of the Geological Society, 115, 49–64. https://doi.org/10.1144/GSL.JGS.1959.115.01.04
- Van der Vlerk, I. M. (1963). Biometric research on Lepidocyclina. Micropaleontology, 9, 425–426. https://doi.org/10.2307/1484502
- Van Wessen, E. J. (1978). Study of Lepidocyclinidae from South-East Asia, particularly from Java and Borneo. Utrecht Micropalaeontological Bullettin, 19, 1–163.
- Varol, O. (1989). Palaeocene calcareous nannofossil biostratigraphy. In J. Crux & S. van Veck (Eds.), Nannofossils and their applications (pp. 267–310). Ellis Horwood.
- Vaughan, T. W., & Cole, W. S. (1941). Preliminary report on the Cretaceous and Tertiary larger foraminifera of Trinidad British West Indies. Geological Society of America Special Papers, 30, 1–137.
- Ward Jr., J. H. (1963). Hierarchical grouping to optimize an objective function. Journal of the American Statistical Association, 58, 236–244. https://doi.org/10.1080/01621459.1963.10500845