Abstract
Objective
Balance difficulties are common in children with sensorineural hearing loss (SNHL). For some of these children, concomitant vestibular deficits may impact postural control. This study aimed to explore vestibular function, functional balance and postural control, and the relationship between these measures in children with SNHL.
Design
Cross-sectional study quantifying peripheral vestibular function (vestibular evoked myogenic potentials [VEMP], video head impulse test), functional balance (Bruininks–Oseretsky Test of Motor Proficiency [BOT]) and postural control (static posturography with modified sensory inputs). The relationship between the degree of vestibular impairment, functional balance and postural control was explored.
Study sample
Eleven with SNHL, and 11 with normal sound detection (NSD) between 5 and 12 years of age.
Results
Children with SNHL had varying degrees of vestibular dysfunction and differences in overall balance performance. Across all children, greater degrees of vestibular impairment were associated with significantly poorer functional balance and postural control performance for complex standing conditions (BOT percentile rank p = 0.001; compliant surface eyes open [EO]: p = 0.027; compliant surface eyes closed: p = 0.048).
Conclusions
Vestibular dysfunction in children with SNHL was variable. Vestibular impairment predicted poorer functional balance performance and postural control abilities, including differences in postural sway patterns.
Introduction
For children with sensory loss affecting the vestibular system, balance problems can be evident in early life, when typical motor milestones of sitting, crawling and walking emerge. Failure to meet motor milestones should trigger onward referral or intervention (Verbecque et al. Citation2017).
As the name implies, mastery of motor milestones is often considered formative elements of childhood development. Sensory dysfunction has developmental consequences for motor milestone mastery. This can further cause delays in integration of the sensory input required for postural control, which is the fundamental requisite for maintaining balance in daily life. For children with sensorineural hearing loss (SNHL), typical milestones of head control, crawling and walking can be significantly delayed (Wiener-Vacher Citation2008; Maes et al. Citation2014a), and there is some evidence to suggest that these delays have repercussions for later childhood, when differences in motor performance persist (Rine et al. Citation2000). Motor delays can also cause an increased risk of falls, clumsiness and longer-term impact on physical participation and activity (Cushing et al. Citation2013; Cushing and Papsin Citation2018).
Children that are most at risk for vestibular dysfunction are those with conditions affecting auditory structures (De Kegel et al. Citation2012; Cushing et al. Citation2013). In children the most common causes of SNHL are related to Connexin 26 mutations, cochleovestibular anomalies occurring in isolation (enlarged vestibular aqueduct), related to a syndrome (Usher, CHARGE syndrome), or acquired (meningitis, cytomegalovirus) (Cushing et al. Citation2013; Martens et al. Citation2022). Greater degrees of SNHL are associated with the increased likelihood of vestibular impairment, and vestibular dysfunction is more closely correlated with conditions related to structural anomalies of the inner ear (Maes et al. Citation2014b; Janky and Givens Citation2015). Conditions affecting the auditory and vestibular structures can have functional and consequential impacts if left undiagnosed.
If underlying mechanisms for motor delay remain unidentified this can affect the success of interventional approaches. In these instances, consequences of undiagnosed vestibular impairment mean that non-specific rehabilitation approaches may cause a plateau of intervention outcomes or prolong intervention unnecessarily (Rine et al. Citation2004). And while this is clearly not life-threatening, it can have a negative impact on quality of life and enjoyment of diverse physical activities at a formative time in development.
One way to ensure that the underlying mechanisms are adquately assessed is to consider all constructs for motor milestone mastery and postural control including sensory integration from the proprioceptive, visual and vestibular systems. This includes postural control measures, which can determine sensory integration processes, functional balance measures to determine motor skill performance on a range of daily activities (Deitz, Kartin, and Kopp Citation2007), and assessment of the peripheral vestibular system to provide quantifying information about vestibular function.
Postural control measures can be particularly useful for establishing postural abilities in children who have motor delay or show differences in motor behaviour. Static posturography documents the amount of postural sway over a specified time period using a force plate and can indicate integration of visual, vestibular and somatosensory systems. Various metrics can be obtained, including centre of pressure (CoP) path length, path velocity or area (Clark et al. Citation2010). Differentiating CoP measurement into discrete frequency bands, known as discrete wavelet transform (DWT) analyses, provides a more nuanced representation of movement patterns not typically observed during traditional measures, and has been valuable for various clinical populations (Rhine, Quatman-Yates, and Clark Citation2017). The wavelet transform involves breaking down postural sway data into various frequency bands that enable greater understanding of physiological constructs of balance control and can be applied across different sensory conditions. Certain frequency components from the DWT analyses are thought to represent different physiological mechanisms used for balance vision (<0.1 Hz) (Friedrich et al. Citation2008), vestibular (0.1–0.39 Hz) (Oppenheim et al. Citation1999), cerebellar (0.39–1.56 Hz) (Paillard et al. Citation2002) and somatosensory information (1.56–6.25 Hz) (Liang et al. Citation2014). These distinct frequency bands represent both sensory and central mechanisms used for postural control and have been used in various contexts (Liang et al. Citation2014). Evaluation of postural control in children with SNHL has shown greater amounts of postural sway in more challenging balance conditions (De Kegel et al. Citation2012). These measures quantify postural control abilities and sensory integration during static activity, but do not capture balance performance during daily activities. Thus, an understanding of daily balance performance is better represented by functional balance measures.
Motor skill performance including fine manual control, body coordination and balance can be determined using functional balance measures (Deitz, Kartin, and Kopp Citation2007). Some measures of functional balance can be used to indicate motor delay and are useful clinical assessments without the need for specialised equipment. Typically, these assessments are well tolerated and have shown to be sensitive to delay (Bruininks Citation2005). However, functional balance tasks must be sufficiently difficult to identify vestibular impairment, as easier tasks may not rely on vestibular inputs (Cushing et al. Citation2008). Additionally, functional balance measures do not clearly delineate underlying mechanisms of motor performance and thus assessment of sensory processes used for balance control should be considered in children who are at risk of vestibular or motor impairments.
Vestibular assessment in children has become an area of interest for many researchers. Assessment of the semicircular canals (SCCs) involves measurement of the vestibulo-ocular reflex (VOR). Various methods of SCC assessment including caloric, rotational chair and video head impulse testing (vHIT) have been performed in children, yet all have some degree of limitation. Caloric testing can be difficult to complete and is not well tolerated even when air calorics are chosen over water calorics (Cushing et al. Citation2008). Rotational chair is limited in providing localising information of the horizontal semicircular canal (HSCC) and is less sensitive to a unilateral deficit in a compensated state. Video head impulse testing uses goggles that are often a poor fit to paediatric patients and fit is critical for accurate assessment. In addition, they have been shown to be insensitive to some pathologies in the adult population (McGarvie et al. Citation2015). However, the benefits of vHIT outweigh the liabilities as vHIT is quick, simple, well tolerated and can measure function for all SCC (Hülse et al. Citation2015; Hamilton, Zhou, and Brodsky Citation2015), and have been shown to be sensitive in quantifying SCC dysfunction in children with SNHL (Janky and Givens Citation2015).
Otolith function is commonly measured using vestibular evoked myogenic potentials (VEMP); cervical VEMP (cVEMP) measures saccule function, and ocular VEMP (oVEMP) measures utricle function, as well as the associated reflex pathways. VEMP are short latency potentials evoked by an auditory or vibratory stimulus, and modulated by myogenic activity (Rosengren et al. Citation2019). A biphasic waveform is typically generated, with two latency measures and a peak-to-peak amplitude calculated to infer otolith function. VEMP assessments have been reported to be well tolerated in the paediatric setting, as both are relatively quick and non-invasive (Fuemmeler et al. Citation2020). VEMP have most commonly been explored in school-aged children, however responses have been recorded in infants (Martens et al. Citation2022). For children with SNHL, VEMP measurements have either been absent (Zhou et al. Citation2009; Cushing et al. Citation2013) or in cases where they are present, have similar latencies but smaller inter-amplitudes, which can be indicative of dysfunction of the relevant structures assessed (Zhou et al. Citation2009). Typically, clinical assessment of the peripheral vestibular system is only considered for children when there are functional balance concerns, or complaints of dizziness or vertigo in children who are able to articulate this accurately.
While the literature has established children with SNHL and vestibular impairment can have delays in motor skill development or postural control differences, these constructs have typically been explored in isolation. Breaking down postural sway data into discrete frequency bands may provide further insight into understanding movement patterns and sensory system reliance that traditional postural control measures do not necessarily provide (Rhine, Quatman-Yates, and Clark Citation2017). Furthermore, comprehensive assessment of peripheral vestibular structures, functional balance and postural control data may be useful adjuncts to inform rehabilitation approaches (Maes et al. Citation2014b). Elements of peripheral vestibular function, functional balance and postural control have been explored in children with SNHL, yet the relationship between these constructs has not been widely considered.
The aim of this study was to explore vestibular function, functional balance and postural control and relationship between these measures in children with SNHL. It was hypothesised that children with SNHL would have differences in peripheral vestibular function, functional balance measures and postural control compared to children with normal sound detection (NSD) thresholds. Furthermore, it was hypothesised that peripheral vestibular dysfunction would negatively impact motor skill performance and postural stability.
Materials and methods
Participants
Two groups of children, one group with SNHL, and one group with NSD, between 5 and 12 years of age were recruited via otolaryngologists, paediatricians and audiologists in community based and private clinical settings in Melbourne, Australia. Guardians provided written consent and children provided verbal assent prior to assessment. All procedures were conducted according to the tenets of the Declaration of Helsinki, and ethical approval was obtained (17-1348H).
Twenty-two children were recruited into the study, n = 11 in each group, with group demographics presented in and hearing loss aetiologies for the SNHL group in . Children in each group were age matched within three months. Those with NSD were characterised by a four-frequency pure tone hearing level average of ≤20 dB bilaterally, and no recent history of middle ear disease (<6 months). Children in the SNHL group had a confirmed diagnosis of bilateral SNHL (characterised by a four-frequency pure tone hearing level average of >20 dB bilaterally), had at least 12 months device experience (hearing aids or cochlear implants), and no recent history of middle ear disease (<6 months). Ten participants had congenital SNHL, one acquired SNHL in infancy. All participants understood simple instructions and had no known neurodevelopmental diagnoses. Children with SNHL had optimised auditory devices at the time of assessment and wore their auditory devices for all assessments.
Table 1. Group demographics for children with normal sound detection and children with sensorineural hearing loss including anthropometric correlates, hearing levels and proportion of normal peripheral vestibular structures (expressed as a percentage).
Table 2. Hearing loss aetiology, proportion of normal vestibular responses, and amplification type for children with sensorineural hearing loss.
Assessment
All children underwent auditory, vestibular and functional balance assessment. Hearing and middle ear status was documented using pure tone audiometry and immittance testing. Vestibular assessments documented peripheral vestibular organ function via the vHIT and VEMP tests. Functional balance measures including the Bruininks–Oseretsky Test of Motor Proficiency (BOT) and static posturography provided an evaluation of body coordination and balance, as well as sensory system integration, involving assessment of standing (static) balance with modified environmental inputs.
Audiometry and immittance
Pure tone audiometry was performed in a sound treated booth using an Interacoustics Affinity PC-based audiometer (Interacoustics, Denmark). Behavioural thresholds were obtained at octave frequencies, and four-frequency pure tone averages (4FA) – calculated from .5 kHz, 1 kHz, 2 kHz and 4 kHz. For the NSD group, the left 4FA HL was 8.4 (5.2) dB HL and right 4FA HL was 7.7 (4.7) dB HL. For the SN group, the left 4FA HL was 106.6 (25) dB HL, and right 4FA HL right ear was 91.9 (35.7) dB HL. Immittance testing using a Grason-Stadler Instruments (GSI) Tympstar included tympanometry and ipsilateral acoustic reflex assessment at 1 kHz; tympanometry findings were classified according to Jerger’s proposed guidelines (Jerger Citation1970). Middle ear status was confirmed prior to advancing to vestibular assessments.
Semicircular canal assessment
Assessment of high frequency SCC function was performed using the GN Otometrics ICS impulse system (GN Otometrics, Taastrup, Denmark). Participants were required to visually fixate on a small static target, located approximately 1 m distance at eye level, directly in front of them. Each coplanar SCC pair was assessed: lateral (left and right HSCC), left anterior/right posterior SCC (LARP) and right anterior/left posterior SCC (RALP). Head impulse magnitude ranged between 15 and 20 degrees, and head impulse peak velocities ranged between 150 and 250 degrees per second for the lateral condition and 100 and 250 degrees per second for the vertical conditions. To minimise recording errors and artefacts, goggles were fit firmly to participants, calibration was performed in between each condition. All efforts were made to ensure there was no eyelid interference (for vertical conditions) and participants remained focused on the visual target thought testing (Mantokoudis et al. Citation2015). A minimum of ten head impulses were performed for each condition. The slow phase eye velocity of the eye movement was compared to the velocity of the head impulse to determine overall VOR gain and was processed and analysed using the GN Otometrics statistical software. Each trial was visually inspected by the examiner, and any artefacts caused by goggle slippage, blinking or pupil tracking difficulties were manually removed (Mantokoudis et al. Citation2015). Head impulses that showed reduced VOR gain and clear, high amplitude corrective saccades with peak eye velocity of 60°/s or greater were retained for analysis (Mantokoudis et al. Citation2015).
Otolith assessment
VEMPs were recorded using Bio-Logic AEP version 7.0.0 software (Natus, Pleasanton, CA) on the Natus Bio-Logic NavPro (Natus, Pleasanton, CA). For cVEMP, a 500 Hz tone burst stimulus of condensing polarity was presented monaurally at 90dBnHL via air conduction (50-ohm ERA insert phones).
Four electrodes were placed on the participant: one common electrode at naison (Nz) on the lower forehead, one non-inverting electrode at the sternoclavicular junction and two inverting electrodes placed on the belly of each sternocleidomastoid muscle (SCM).
Participants lay semi-recumbent with their head and torso positioned approximately 20 degrees from supine. From this position, participants were instructed to lift their head towards their toes and hold this position until the stimulus stopped (Rosengren et al. Citation2019). Real time electromyographic (EMG) rectification was not possible due to device limitations but SCM contraction was visually monitored by the examiner for each trial, and post-stimulus rectification was applied to waveforms. To minimise fatigue effects stimuli were presented alternately between ears and breaks were provided between presentations. Conventional cVEMP labelling (P13 and N23 latencies), and P13-N23 interamplitude were determined for all traces.
For oVEMP a 500 Hz stimulus was presented at 128 dB pFL via bone conduction (B&K minishaker), with the minishaker placed at the midline of the upper forehead (Fz). Four electrodes were placed on the participant: one common electrode at the sternoclavicular junction, one non-inverting electrode on the chin, and two inverting electrodes placed on lower orbits of the eyes (left and right) and aligned with the centre of each pupil. Participants lay semi-recumbent and maintained an upward gaze of approximately 15 degrees for the stimulus duration. Conventional oVEMP labelling (baseline, N10 and P16) and two interamplitude calculations (baseline to N10, and N10-P16) were determined for all traces.
For both VEMP assessments, a minimum of two traces per condition were conducted in all to ensure repeatability. Responses were averaged and labels assigned to the average waveform which was then used for statistical analysis.
Functional balance assessment
Functional balance was measured using two subtests of the BOT 2nd Edition (BOT-2) (Bruininks Citation2005); bilateral coordination and balance. The standardised test battery was used according to BOT-2 guidelines (Bruininks Citation2005); each task was taught to the child via physical demonstration, verbal instruction or instructional pictures (via the BOT task easel). Bilateral coordination tasks included jumping in place and walking in a straight line; these were scored based on the number of responses correct. Balance tasks included standing on one leg and standing on a balance beam; these were scored based on the maximum duration the participant was able to maintain their balance. For each task, a maximum of two trials were administered. Sex-specific norms were used to determine scale scores, percentile ranks, and an overall composite body coordination score for each subtest.
Postural control assessment
The Nintendo Wii Balance Board (WBB) was used to perform computerised static posturography which has been validated against traditional force plate measures (Clark et al. Citation2010). The board was connected to a laptop via Bluetooth and data were recorded at the native frequency (≈40 Hz) using customised software (LabVIEW version 8.5, National Instruments, Austin, TX). System calibration and data processing were conducted using established methods (Clark et al. Citation2010).
Centre of pressure information was measured across four standing balance conditions: standing on a firm surface with eyes open (EO) and eyes closed (EC); and standing on a compliant (foam) surface with eyes open (FEO) and eyes closed (FEC). A 5 cm high-density thermoplastic polyurethane cushion was placed over the WBB platform for compliant conditions (Vitkovic et al. Citation2016).
All participants were instructed to stand barefoot (feet 10 cm apart) on the WBB and remain as still as possible with hands by their sides. For each condition (EO, EC, FEO and FEC), three 30 s trials were completed and the median score of the three trials were used for statistical analyses. Trials were discontinued if the participant lost balance, opened their eyes (for eyes closed conditions), or moved their arms; if this occurred the participant was given one additional trial for the same condition. Height and weight were used as covariates in statistical analyses.
CoP measures included path velocity (cm/s) and DWT analyses, which corresponded to very slow to fast-moving components of the CoP data. The DWT frequency bands comprised of ultralow (<0.10 Hz), very low (0.10–0.39 Hz), low (0.39–1.56 Hz), and moderate frequency bands (1.56–6.25 Hz) and were extracted from the CoP data using the methods described elsewhere (Clark and Pua Citation2018). These are thought to represent different physiological mechanisms: the visual system (ultralow) (Friedrich et al. Citation2008), the vestibular system (very low) (Oppenheim et al. Citation1999), cerebellar system (low) (Paillard et al. Citation2002) and somatosensory system (moderate) (Liang et al. Citation2014). These measures were analysed across all standing balance conditions for the anterior–posterior plane.
Statistical analysis
Path velocity, DWT measures, BOT measures and VEMP latencies (P13, N23, N10 and P16) were assumed to be normally distributed based on the Anderson Darling Test of Normality; VEMP amplitudes (P13-N23, Base-N10 and N10-P16), and vHIT measures (HSCC, ASCC and PSCC) were observed to be not normally distributed.
Outcome measures included latencies and amplitudes (for VEMP), VOR gain (for vHIT), BOT percentile ranks, BOT Balance scale score, BOT bilateral coordination scale score and CoP data (path velocity, DWT analyses for ultralow, very low, low and moderate frequency bands). CoP analyses included height, weight and age as covariates. Group comparisons for each assumed normally distributed outcome measures and were performed using general linear modelling, and Mann–Whitney U tests used for non-parametric data (vHIT gain and VEMP amplitudes). The significance level for post-hoc Tukey analyses was set at p < 0.05. Regression and Pearson correlations were conducted to explore the relationship between clinical vestibular assessments, functional balance and postural sway measures across all children.
Results
All children completed postural control and peripheral vestibular function assessments. Three children (one with NSD and two with SNHL) underwent vHIT for the lateral condition only due to reluctance with completing LARP/RALP conditions. One child with SNHL did not complete VEMP testing due to assessment contraindications.
Peripheral vestibular function between groups
Interpretation of peripheral vestibular function was dependent on the presence or absence of responses for each assessment. For vHIT, all children had measurable responses and thus, all comparisons were included in analysis. Based on normative data obtained from the control group (cut off values for normal VOR gain were based on two standard deviations from the NSD group mean, equating to <0.81 for HSCC, and <0.75 for ASCC/PSCC), five children with SNHL had reduced VOR gain for one or more SCC (19/58, 33%). All impulses with reduced VOR gain were associated with corrective saccades; there were no instances of normal VOR gain with present corrective saccades. A proportion of children with SNHL had absent VEMPs which meant that their findings were removed from comparative analyses. outlines the proportion of normal vestibular responses between the groups for each vestibular assessment, and outlines individual findings for children with SNHL.
Children with SNHL (who had recordable VORs) showed overall lower gain values in all vHIT conditions (). Large variability for the SNHL group was observed with some children demonstrating significantly reduced VOR gain, while others showed clinically normal VOR gain. When absent VEMP responses were excluded from analyses, cVEMP latencies, amplitudes and oVEMP latencies, amplitudes were similar between groups ().
Table 3. Group comparisons for all measures of peripheral vestibular function (vHIT, cVEMP and oVEMP).
Postural stability and functional balance between groups
Decreased postural stability was observed in children with SNHL for conditions requiring greater reliance on the vestibular system, as illustrated by . Faster path velocities indicative of decreased postural stability was observed for children with SNHL when on compliant surfaces (FEO: NSD mean [SD] = 2.40 [0.60] vs. SNHL mean [SD] = 3.84 [2.16], p = 0.046; FEC: NSD mean [SD] = 4.34 [1.22] vs. SNHL mean [SD] = 6.41 [2.84], p = 0.038). Path velocity was similar between groups when standing on a firm surface (EO: NSD mean [SD] = 1.59 [0.55] vs. SNHL mean [SD] = 2.35 [1.36], p = 0.1; EC: NSD mean [SD] = 1.92 [0.49] vs. SNHL mean [SD] = 2.78 [1.54], p = 0.108).
Figure 1. Mean path velocity across the four standing conditions for children with normal sound detection and children with SNHL. Children with SNHL showed reduced postural stability, as indicated by increased path velocities, in conditions where somatosensory information was modified (FEO and FEC conditions). Additionally, large variability in standing balance was observed in children with SNHL across all conditions when compared to children with normal sound detection. *Denotes p < 0.05.
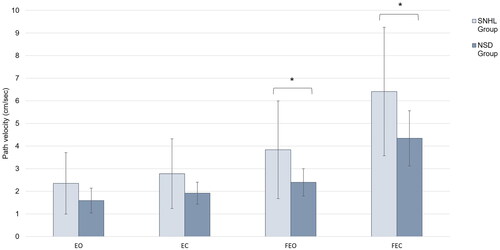
When the postural sway data were broken down into discrete frequency bands, different patterns of postural sway emerged between the groups. For the frequency band thought to correlate to vestibular information (DWT very low), children with SNHL showed overall greater sway across some standing conditions (EO: p = 0.030; EC: p = 0.050; FEO: p = 0.040; FEC: p = 0.267; ). Greater postural sway was also noted in compliant surface conditions (FEO and FEC) across frequency bands thought to be correlated with cerebellar (FEO: p = 0.040; FEC: p = 0.031) and somatosensory (FEO: p = 0.033, FEC: p = 0.038) information () for children with SNHL.
Table 4. Group comparisons for DWT bandwidth velocity across frequency bands for all standing balance conditions.
Relationship between vestibular function, functional balance and postural control
The degree of vestibular loss was categorised based on the number of abnormal responses observed for each vestibular structure (utricle, saccule, HSCC, ASCC and PSCC) in both ears (Janky and Givens Citation2015). A score of 0 indicated abnormal function of all peripheral vestibular structures, and score 10 indicated normal function of all peripheral vestibular structures.
A significant relationship between the degree of peripheral vestibular function and BOT was observed, with a larger number of abnormal peripheral vestibular structures associated with lower percentile ranks, bilateral coordination and balance scale scores (Supplementary Table 1). Peripheral vestibular dysfunction also predicted performance when standing on a compliant surface with FEO and FEC; those with larger postural sway had greater degrees of peripheral vestibular dysfunction.
When eyes were open, peripheral vestibular function predicted DWT performance relating to ultralow, very low and low frequency bands. Those with greater postural sway in these frequency bands had reduced peripheral vestibular function, with a stronger negative correlation noted for more complex standing balance conditions (FEO).
Functional balance and path velocity measures were negatively correlated indicating that children with lower functional balance measures had larger path velocity scores which is overall consistent with reduced postural stability. Lower percentile ranks were significantly associated with larger path velocity measures for all conditions except for when standing with eyes closed on a firm surface (EO path velocity: r (22) = −0.454, p = 0.034; EC path velocity: r (22) = −0.329, p = 0.145; FEO path velocity: r (22) = −0.692, p < 0.001; FEC path velocity: r (22) = −0.661, p < 0.001). This pattern also extended to balance scale scores (EO path velocity: r (22) = −0.478, p = 0.024; EC path velocity: r (22) = −0.338, p = 0.134; FEO path velocity: r (22) = −0.704, p < 0.001; FEC path velocity: r (22) = −0.655, p = 0.001), yet bilateral coordination scale scores were only significantly correlated with more complex standing balance conditions (EO path velocity: r (22) = −0.270, p = 0.225; EC path velocity: r (22) = −0.206, p = 0.371; FEO path velocity: r (22) = −0.492, p = 0.020; FEC path velocity: r (22) = −0.508, p = 0.016).
Discussion
The aim of this study was to explore peripheral vestibular function and postural control in children with SNHL. As such, it is one of few studies to explore the relationship between functional balance, postural control and comprehensive peripheral vestibular function in this population. Most studies in this area have focused on functional balance measures coupled with one to two measures of peripheral vestibular function (Singh et al. Citation2022). Children with SNHL in this study had varying degrees of peripheral vestibular dysfunction, with reduced vestibular function consequently influencing postural control and functional balance ability. There was a significant relationship between peripheral vestibular function and functional balance measures, which has implications for daily activities. Measures of postural control reveal that postural control strategies are different for greater degrees of vestibular loss. This is relevant because these different strategies are used where eyes are open, akin to general daily activity. These findings highlight the importance of routinely performing a range of clinical assessments to measure balance function for all children with SNHL, to understand the likely implications for balance performance and to consider potential remediations.
When considering all peripheral vestibular organs, peripheral vestibular function was significantly different between groups, with large variability in peripheral deficit for children with SNHL. This variability is due to the range of aetiologies, only some of which have known association with vestibular impairment (including Usher syndrome and cytomegalovirus), compared to other aetiologies that show a lower incidence of peripheral deficit, such as Connexin 26 mutations (Cushing et al. Citation2013; Martens et al. Citation2022). Some children with SNHL had partial or complete dysfunction of peripheral vestibular structures, whereas others had similar peripheral vestibular function to children with NSD.
Capturing normative values from age-matched children with NSD is a strength of this study. Previous cut offs for vHIT in children included a fixed value to determine abnormal function (Hamilton, Zhou, and Brodsky Citation2015) or values which differed depending on the plane assessed (Janky and Givens Citation2015). Using the normative VOR range based on children with NSD in this study, children with SNHL showed a large range of variability in SCC function. Children with SNHL and absent saccule function were excluded from the statistical comparison; yet those with saccule function had similar VEMP latencies and amplitudes to children with NSD, in agreement with other findings (Maes et al. Citation2014b).
There is no clear consensus in the literature regarding the degree and extent of vestibular dysfunction in children impacted with SNHL. Few studies have looked at function of all vestibular organs; this study showed similar proportions of SCC and otolith abnormalities consistent with other studies that have assessed all vestibular structures (Janky and Givens Citation2015). The overall incidence of abnormal vestibular responses was lower in this study; 30–40% of vestibular function tests were abnormal in children with SNHL compared to 45–55% (Cushing et al. Citation2008; Maes et al. Citation2014b; Janky and Givens Citation2015), although this estimate can be far greater, up to 90% depending on the vestibular organ assessed (Zhou et al. Citation2009). This difference may also relate to the types of aetiologies and likelihood of associated structural abnormalities.
Overall peripheral vestibular function predicted performance on functional balance measures, with greater degrees of vestibular impairment related to poorer balance. This indicates that functional balance and motor performance are directly influenced by vestibular contributions and this is supported in studies with younger and older children previously published (De Kegel et al. Citation2012; Janky and Givens Citation2015).
Greater degrees of vestibular impairment correlated with more postural sway, most evident for conditions requiring complex sensory system integration, when standing on a compliant surface with EO and closed. This has been reported in other studies where differences in balance performance for people with vestibular impairment occur when conditions become more difficult (Enbom, Magnusson, and Pyykkö Citation1991; Cushing et al. Citation2008). Different patterns emerged when postural sway was broken down into discrete frequency bands. When somatosensory information was modified (standing on a compliant surface with EO and closed), movement patterns of CoP data showed greater postural sway for frequency bands corresponding to cerebellar and somatosensory information. Significantly more postural sway was also noted in the frequency band corresponding to vestibular information in EO conditions (EO on firm and compliant surfaces). This suggests that overall, children with SNHL may be utilising sensory processes effectively, but in cases where peripheral vestibular function is compromised, the incoming sensory information from the vestibular system may be insufficient to effectively contribute to postural control. This may result in greater reliance on somatosensory information. Furthermore, this pattern of responses might be related to the integrative processes required for postural control: this may be related to a motor delay, as motor delays have been widely documented in children with SNHL (De Kegel et al. Citation2012; Maes et al. Citation2014a), or related to the integration of incoming sensory information, which may take longer due to the compensatory strategies (adaptive or substitution) occurring to maintain balance. Specifically, there is evidence to suggest that younger children place greater reliance on visual information for postural control, and the sensory system re-weighting adjusts in later childhood to be more reliant on somatosensory and vestibular information before reaching adult form (Schmid et al. Citation2005).
Postural stability measures were performed in conditions whilst standing stationary. Maintaining postural stability when still should be easier than during dynamic movement as less task demands on the sensory systems are required (Hatzitaki et al. Citation2002). For children with vestibular impairment, postural control challenges may be amplified for scenarios in daily life where dynamic movement is required, which can have a negative impact on physical activity, general mobility and also causing greater falls risk. Moderate to strong correlations were observed between functional balance and postural stability, indicating that those with larger postural sway had decreases in functional balance. Therefore, in the absence of computerised postural stability measures, functional balance measures may serve as an alternative assessment method in the clinical environment.
While not explicitly considered as a factor in the statistical analyses, most children with SNHL in this study had undergone some form of physical therapy in their pre-school years to address motor milestone delays, and regularly participated in sporting activities. Despite physical therapy these group deficits remain, highlighting that children with vestibular impairment are potentially at greater risk of motor delay or falls in future. Cross-sectional comparisons from this study have demonstrated differences in various aspects of balance performance. Yet, longitudinal comparisons may unveil greater differences, with evidence to suggest that motor delay can progress for children with vestibular impairment, particularly if they do not undergo vestibular rehabilitation (Rine et al. Citation2000; Rine et al. Citation2004).
It is known that different aetiologies of hearing loss can present with varying degrees of peripheral vestibular function (Cushing et al. Citation2013). We grouped all aetiologies and degrees of hearing loss into one due to small sample size and as a result this study does not allow statistical comparisons. However, it was observed that for certain conditions (Usher Type I, Cytomegalovirus and Branchio-Oto-Renal), children had vestibular impairment of most or all peripheral vestibular structures which has been noted in other studies (Cushing et al. Citation2013; Verbecque et al. Citation2017)). Additionally, a large proportion of children in this study had at least one cochlear implant. Cochlear implantation has the potential to damage peripheral vestibular structures with an estimated impact of approximately 10% (Jacot et al. Citation2009), however recent studies suggest this incidence is far lower (Dhondt et al. Citation2022). True impacts of cochlear implantation on peripheral vestibular function remain unknown without baseline assessment. Furthermore, age differences for cochlear implantation may contribute to the degree or progression of vestibular deficit, and the time taken to centrally compensate. Age of cochlear implantation may also influence central compensatory processes, auditory mapping and sensory integration. While balance performance could potentially be influenced by the amount of auditory input provided by devices in individuals with vestibular impairment (Vitkovic et al. Citation2016), all children with SNHL in this study had optimised hearing devices worn throughout assessment.
The implications of vestibular impairment can extend beyond balance performance. Depending on the age on the individual, a range of functional implications may be evident, from motor milestone delays in children with congenital vestibular impairment, to activity limitations during sport or other recreational activities. For children with vestibular impairment, there is a body of literature that supports vestibular rehabilitation, including exercises to improve gaze stability, postural control and motor performance (Melo et al. Citation2019). The vestibular rehabilitation approach is largely dependent on the type and severity of the vestibular impairment but may include adaptation/habituation strategies focusing on central mechanisms, or substitution strategies focusing on increasing reliance of other sensory mechanisms, such as vision or somatosensation. Thus, comprehensive vestibular assessment should be considered as a complementary assessment for children with balance difficulties to refine vestibular rehabilitation approaches.
Fundamental motor milestones are achieved by early childhood, yet functional balance deficits can remain if underlying mechanisms for balance control are not investigated thoroughly. While some studies have explored these mechanisms in younger age groups (De Kegel et al. Citation2012) the feasibility of reliable vestibular testing in children <5 years can be challenging, and require specific test protocols, but is important to address the critical need for these assessments to be performed in a timely manner to complement rehabilitation (Martens et al. Citation2022). Furthermore, future research should aim to document the developmental trajectory and longitudinal changes that peripheral vestibular dysfunction has on motor performance and determine whether deficits remain in adolescence and adulthood. While this has been documented in adolescents and cross-sectionally (Melo et al. Citation2015), it is not known how balance performances changes over time in the individual.
Conclusion
Outcomes from this study demonstrate that some children with SNHL can have reduced vestibular function and decreased postural stability, which in turn, can negatively impact functional balance. Furthermore, postural control findings for children with vestibular impairment suggest that strategies to maintain balance in more complex environments may be related to a delay of available sensory integration mechanisms. For any child diagnosed with SNHL, vestibular function and functional balance assessment are imperative to identify vestibular deficit and better understand the functional consequences on motor performance so that appropriate mitigating strategies can be implemented.
Disclosures statement
Professor Rance was supported by the Graeme Clark Chair in Audiology and Spech Science. The authors have no conflicts of interest to declare, financial or otherwise.
Supplemental Material
Download MS Word (19.2 KB)Additional information
Funding
References
- Bruininks, R. H. 2005. Bruininks-Oseretsky Test of Motor Proficiency. Circle Pines, MN: AGS Publishing.
- Clark, R. A., A. L. Bryant, Y. Pua, P. McCrory, K. Bennell, and M. Hunt. 2010. “Validity and Reliability of the Nintendo Wii Balance Board for Assessment of Standing Balance.” Gait & Posture 31 (3):307–310. https://doi.org/10.1016/j.gaitpost.2009.11.012
- Clark, R. A., and Y. H. Pua. 2018. “SeeSway – A free Web-based System for Analysing and Exploring Standing Balance Data.” Computer Methods and Programs in Biomedicine 159:31–36. https://doi.org/10.1016/j.cmpb.2018.02.019
- Cushing, S. L., K. A. Gordon, J. A. Rutka, A. L. James, and B. C. Papsin. 2013. “Vestibular End-organ Dysfunction in Children with Sensorineural Hearing Loss and Cochlear Implants: An Expanded Cohort and Etiologic Assessment.” Otology & Neurotology 34 (3):422–428. https://doi.org/10.1097/MAO.0b013e31827b4ba0
- Cushing, S. L., and B. C. Papsin. 2018. “Cochlear Implants and Children with Vestibular Impairments.” Seminars in Hearing 39 (3):305–320. https://doi.org/10.1055/s-0038-1666820
- Cushing, S. L., B. C. Papsin, J. A. Rutka, A. L. James, and K. A. Gordon. 2008. “Evidence of Vestibular and Balance Dysfunction in Children with Profound Sensorineural Hearing Loss Using Cochlear Implants.” The Laryngoscope 118 (10):1814–1823. https://doi.org/10.1097/MLG.0b013e31817fadfa
- De Kegel, A., L. Maes, T. Baetens, I. Dhooge, and H. Van Waelvelde. 2012. “The Influence of a Vestibular Dysfunction on the Motor Development of Hearing-impaired Children.” The Laryngoscope 122 (12):2837–2843. https://doi.org/10.1002/lary.23529
- Deitz, J. C., D. Kartin, and K. Kopp. 2007. “Review of the Bruininks-Oseretsky Test of Motor Proficiency, (BOT-2).” Physical & Occupational Therapy in Pediatrics 27 (4):87–102. https://doi.org/10.1080/J006v27n04_06
- Dhondt, C., L. Maes, S. Vanaudenaerde, S. Martens, L. Rombaut, R. Van Hecke, R. Valette, F. Swinnen, and I. Dhooge. 2022. “Changes in Vestibular Function Following Pediatric Cochlear Implantation: A Prospective Study.” Ear and Hearing 43 (2):620–630. https://doi.org/10.1097/AUD.000000000000112
- Enbom, H., M. Magnusson, and I. Pyykkö. 1991. “Postural Compensation in Children with Congenital or Early Acquired Bilateral Vestibular Loss.” The Annals of Otology, Rhinology, and Laryngology 100 (6):472–478. https://doi.org/10.1177/000348949110000609
- Friedrich, M., H.-J. Grein, C. Wicher, J. Schuetze, A. Mueller, A. Lauenroth, K. Hottenrott, and R. Schwesig. 2008. “Influence of Pathologic and Simulated Visual Dysfunctions on the Postural System.” Experimental Brain Research 186 (2):305–314. https://doi.org/10.1007/s00221-007-1233-4
- Fuemmeler, E., A. I. Rodriguez, M. Thomas, T. Creutz, D. Fitzpatrick, and K. L. Janky. 2020. “Vestibular Evoked Myogenic Potential (VEMP) Test-retest Reliability in Children.” Otology & Neurotology 41 (8):e1052–e1059. https://doi.org/10.1097/MAO.0000000000002703
- Hamilton, S. S., G. Zhou, and J. R. Brodsky. 2015. “Video Head Impulse Testing (VHIT) in the Pediatric Population.” International Journal of Pediatric Otorhinolaryngology 79 (8):1283–1287. https://doi.org/10.1016/j.ijporl.2015.05.033
- Hatzitaki, V., V. Zisi, I. Kollias, and E. Kioumourtzoglou. 2002. “Perceptual-motor Contributions to Static and Dynamic Balance Control in Children.” Journal of Motor Behavior 34 (2):161–170. https://doi.org/10.1080/00222890209601938
- Hülse, R., K. Hörmann, J. J. Servais, M. Hülse, and A. Wenzel. 2015. “Clinical Experience with Video Head Impulse Test in Children.” International Journal of Pediatric Otorhinolaryngology 79 (8):1288–1293. https://doi.org/10.1016/j.ijporl.2015.05.034
- Jacot, E., T. Van Den Abbeele, H. R. Debre, and S. R. Wiener-Vacher. 2009. “Vestibular Impairments Pre-and Post-cochlear Implant in Children.” International Journal of Pediatric Otorhinolaryngology 73 (2):209–217. https://doi.org/10.1016/j.ijporl.2008.10.024
- Janky, K., and D. Givens. 2015. “Vestibular, Visual Acuity and Balance Outcomes in Children with Cochlear Implants: A Preliminary Report.” Ear and Hearing 36 (6):e364–e372. https://doi.org/10.1097/AUD.0000000000000194
- Jerger, J. 1970. “Clinical Experience with Impedance Audiometry.” Archives of Otolaryngology 92 (4):311–324. https://doi.org/10.1001/archotol.1970.04310040005002
- Liang, Z., R. Clark, A. Bryant, J. Quek, and Y. H. Pua. 2014. “Neck Musculature Fatigue Affects Specific Frequency Bands of Postural Dynamics During Quiet Standing.” Gait & Posture 39 (1):397–403. https://doi.org/10.1016/j.gaitpost.2013.08.007
- Maes, L., A. De Kegel, H. Van Waelvelde, and I. Dhooge. 2014a. “Association between Vestibular Function and Motor Performance in Hearing-impaired Children.” Otology & Neurotology 35 (10):e343–e347. https://doi.org/10.1097/MAO.0000000000000597
- Maes, L., A. De Kegel, H. Van Waelvelde, and I. Dhooge. 2014b. “Rotatory and Collic Vestibular Evoked Myogenic Potential Testing in Normal-hearing and Hearing-impaired Children.” Ear and Hearing 35 (2):e21-32–e32. https://doi.org/10.1097/AUD.0b013e3182a6ca91
- Mantokoudis, G., A. S. Saber Tehrani, J. C. Kattah, K. Eibenberger, C. I. Guede, D. S. Zee, and D. E. Newman-Toker. 2015. “Quantifying the Vestibulo-ocular Reflex with Video-oculography: Nature and Frequency of Artifacts.” Audiology & Neuro-Otology 20 (1):39–50. https://doi.org/10.1159/000362780
- Martens, S., I. Dhooge, C. Dhondt, S. Vanaudenaerde, M. Sucaet, H. Van Hoecke, E. De Leenheer, L. Rombaut, A. Boudewyns, C. Desloovere, et al. 2022. “Three Years of Vestibular Infant Screening in Infants with Sensorineural Hearing Loss.” Pediatrics 150 (1):e2021055340. https://doi.org/10.1542/peds.2021-055340
- McGarvie, L. A., I. S. Curthoys, H. G. MacDougall, and G. M. Halmagyi. 2015. “What Does the Dissociation between the Results of Video Head Impulse Versus Caloric Testing Reveal About the Vestibular Dysfunction in Ménière’s Disease?” Acta Oto-Laryngologica 135 (9):859–865. https://doi.org/10.3109/00016489.2015.1015606
- Melo, R., A. Lemos, C. Macky, M. C. F. Raposo, and K. M. Ferraz. 2015. “Postural Control Assessment in Students with Normal Hearing and Sensorineural Hearing Loss.” Brazilian Journal of Otorhinolaryngology 81 (4):431–438. https://doi.org/10.1016/j.bjorl.2014.08.014
- Melo, R. S., A. Lemos, G. S. Paiva, L. Ithamar, M. C. Lima, S. H. Eickmann, K. M. Ferraz, and R. B. Belian. 2019. “Vestibular Rrehabilitation Exercises Programs to Improve the Postural Control, Balance and Gait of Children with Sensorineural Hearing Loss: A Systematic Review.” International Journal of Pediatric Otorhinolaryngology 127:109650. https://doi.org/10.1016/j.ijporl.2019.109650
- Oppenheim, U., R. Kohen-Raz, D. Alex, A. Kohen-Raz, and M. Azarya. 1999. “Postural Characteristics of Diabetic Neuropathy.” Diabetes Care 22 (2):328–332. https://doi.org/10.2337/diacare.22.2.328
- Paillard, T., C. Costes-Salon, C. Lafont, and P. Dupui. 2002. “Are there Differences in Postural Regulation According to the Level of Competition in Judoists?” British Journal of Sports Medicine 36 (4):304–305. https://doi.org/10.1136/bjsm.36.4.304
- Rhine, T., C. Quatman-Yates, and R. A. Clark. 2017. “A Longitudinal Examination of Postural Impairments in Children with Mild Traumatic Brain Injury: Implications for Acute Testing.” The Journal of Head Trauma Rehabilitation 32 (2):E18–E23. https://doi.org/10.1097/HTR.0000000000000192
- Rine, R. M., J. Braswell, D. Fisher, K. Joyce, K. Kalar, and M. Shaffer. 2004. “Improvement of Motor Development and Postural Control Following Intervention in Children with Sensorineural Hearing Loss and Vestibular Impairment.” International Journal of Pediatric Otorhinolaryngology 68 (9):1141–1148. https://doi.org/10.1016/j.ijporl.2004.04.007
- Rine, R. M., G. Cornwall, K. Gan, C. LoCascio, T. O’Hare, E. Robinson, and M. Rice. 2000. “Evidence of Progressive Delay of Motor Development in Children with Sensorineural Hearing Loss and Concurrent Vestibular Dysfunction.” Perceptual and Motor Skills 90 (3 Pt 2):1101–1112. https://doi.org/10.2466/pms.2000.90.3c.1101
- Rosengren, S. M., J. G. Colebatch, A. S. Young, S. Govender, and M. S. Welgampola. 2019. “Vestibular Evoked Myogenic Potentials in Practice: Methods, Pitfalls and Clinical Applications.” Clinical Neurophysiology Practice 4:47–68. https://doi.org/10.1016/j.cnp.2019.01.005
- Schmid, M., S. Conforto, L. Lopez, P. Renzi, and T. D’Alessio. 2005. “The Development of Postural Strategies in Children: A Factorial Design Study.” Journal of Neuroengineering and Rehabilitation 2 (1):29. https://doi.org/10.1186/1743-0003-2-29
- Singh, A., H. Heet, D. S. Guggenheim, M. Lim, B. Garg, M. Bao, S. L. Smith, D. Garrison, E. M. Raynor, J. W. Lee, et al. 2022. “A Systematic Review on the Association Between Vestibular Dysfunction and Balance Performance in Children with Hearing Loss.” Ear and Hearing 43 (3):712–721. https://doi.org/10.1097/AUD.0000000000001131
- Verbecque, E., T. Marijnissen, N. De Belder, V. Van Rompaey, A. Boudewyns, P. Van de Heyning, L. Vereeck, and A. Hallemans. 2017. “Vestibular (dys) Function in Children with Sensorineural Hearing Loss: A Systematic Review.” International Journal of Audiology 56 (6):361–381. https://doi.org/10.1080/14992027.2017.1281444
- Vitkovic, J., C. Le, S. L. Lee, and R. A. Clark. 2016. “The Contribution of Hearing and Hearing Loss to Balance Control.” Audiology & Neuro-Otology 21 (4):195–202. https://doi.org/10.1159/000445100
- Wiener-Vacher, S. R. 2008. “Vestibular Disorders in Children.” International Journal of Audiology 47 (9):578–583. https://doi.org/10.1080/14992020802334358
- Zhou, G., M. A. Kenna, K. Stevens, and G. Licameli. 2009. “Assessment of Saccular Function in Children with Sensorineural Hearing Loss.” Archives of Otolaryngology-Head & Neck Surgery 135 (1):40–44. https://doi.org/10.1001/archoto.2008.508