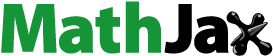
ABSTRACT
The use of zeolites as fillers in mixed matrix membranes (MMM) in gas separation is a well-studied subject. The difficulties to produce a membrane with high selectivity and low defects in the zeolite/polymer interface are well documented. Many researchers have reported that the functionalization of zeolite surface led to membranes with higher compatibility with zeolite/polymer via using silane groups, amine groups, or ionic liquids in the particles surface to improve the gas permeability and separation efficiency. This article aims to describe, summarize and discuss the use of amines, silanes and ionic liquids as surface modifiers. Those modifications lead to an increase in selectivity and in polymer/filler compatibility by increasing the dispersion of the particles in the polymeric matrix, which represents the most recent subjects in the development of MMMs. The performance results surpass by many times the efficiency of regular MMM synthesis. The work is focused specifically on MMMs using the silico-aluminophosphate SAPO-34 due its potential, diverging from general reviews, and focusing on a specific case discussing recent works and new ways to develop this theme through a deeper study. All possible SAPO-34 surface modifications are compared, and new possible membrane modifications and treatments are proposed.
Introduction
Zeolites have often been used as adsorbents for gas purification and as ion exchangers in detergents and are extremely useful as catalysts in petrochemicals (for example, in petroleum refining), in the synthesis of organic products, and also in pharmaceutical and fine chemical processes.[Citation1–4] These versatile applications are possible because zeolites have a high surface area and adsorption capacity, with properties varying in a wide range of polarity. Also, the zeolite structure allows for the creation of active sites, such as acidic sites. The strength and concentration of the active sites can be controlled according to the desired application. The size of zeolite channels and cavities are compatible with most molecules of raw materials used in industry and their complex network gives produces different types of selectivity. These properties can be used to drive a reaction according to the desired product, due to the selectivity of the reagent, product and transition state.[Citation5] Zeolites have long been widely used as solid acid catalysts in industry. There are already commercial processes and plants using these catalysts because of the advantages they show over traditional homogeneous acid catalysts[Citation1,Citation4,Citation6–8]
The silicoaluminophosphates (SAPOs) are zeolites in the form TO4 (T= Si, Al or P) widely used in catalysis and separation processes because their pore size is in dimensions near to most common molecules. Varied three-dimensional arrangements of the basic SAPO building units result in frameworks containing channels of molecular dimensions that characterize the molecular sieves. Zeolites are classified according to size: small (less than 0.4 nm or 4 Å), medium, (0.4–0.6 nm or 4–6 Å), large, (0.6–0.8 nm or 6–8 Å), or even super large pore (bigger than 0.8 nm). Molecular sieves with pores larger than 2 nm (20 Å), with amorphous walls, are classified as mesoporous.[Citation9] SAPOs can be prepared basically through different processes. The most common processes are hydrothermal[Citation10,Citation11] and dry-gel[Citation12,Citation13] methods. These methodologies can influence the crystal size and characteristics of the final material.[Citation14,Citation15]
SAPO-34 appeared as one of the main materials used in mixed matrix membranes (MMM) used for post-combustion gas separation in comparison with other zeolites mainly due to its pore size (0.38 nm) near to the kinetic diameter of gases like He (0.26 nm), H2 (0.29 nm), CO2 (0.33 nm), N2 (0.36 nm), CO (0.37 nm), or CH4 (0.38 nm).[Citation16–20] This means that the pore size of SAPO-34 is big enough to allow H2, He, CO2 to permeate, but small enough to block the permeation of the other gases. In comparison, SAPO-11 has elliptical pore of 0.39 × 0.63 nm; SAPO-31 has a 0.54 nm main pore size; SAPO-41 has elliptical pore of 0.43 × 0.70 nm, and other pore sizes are as follows: SAPO-44 (0.45 nm), Zeolite A (0.41 nm), and ZSM-5 (0.54 nmx0:56 nm). The possible role of SAPO-34 in CO2/N2 gas separation is presented in ,[Citation21,Citation22] It could be used in methanol to olefins conversion reactions.[Citation9,Citation10,Citation23–26]
Figure 1. Mixed matrix membrane with SAPO-34 CO2/N2 separation.[Citation21,Citation22] .
![Figure 1. Mixed matrix membrane with SAPO-34 CO2/N2 separation.[Citation21,Citation22] .](/cms/asset/3e695d2e-26c7-4769-9dc7-eaa271cb65f7/lspr_a_2204080_f0001_oc.jpg)
This work aims to discuss recent works on MMMs using SAPO-34 as filler taking those studies as a base to debate recent methodologies that deal with the matrix/filler incompatibilities reported by many authors due to an organic matrix and inorganic filler interaction, studying and proposing strategies that could mitigate those interfacial voids and, therefore, promote an increase in selectivity. The work is focused specifically on MMMs using SAPO-34 due its potential, ease of production, possible internal (doping), and external modifications (surface modifications). SAPO-34 MMMs diverge from the general MMM reviews presented elsewhere.[Citation22,Citation24–36] The study allows us to focus on a specific case, although many strategies presented for these MMMs could also be extended for other fillers.
SYNTHESIS AND CHARACTERIZATION OF SAPO-34
Structure Directing Agent (SDA) or Template
The synthesis of SAPO-34 can be performed in various ways, leading to the formation of the solid with a chabazite structure (CHA). Crystallization process in the synthesis of SAPO-34 crystals depends significantly on the type and concentration of template because both the nucleation and crystal-growth rates related to the alkalinity of the solution and the interaction between the template and the inorganic species, influencing in surface area and crystal size.[Citation37]
More than 20 types of organic SDAs have been utilized to synthesize SAPO-34 catalysts. Among these templates, tetraethylammonium hydroxide (TEAOH), morpholine (MOR), triethylamine (TEA) and diethylamine (DEA) are the most used templates. The choice and concentration of SDA significantly affects the resulting structure, particle size and physicochemical properties of synthesized zeolites. In general, compared to other templates, TEAOH tends to direct the synthesis of smaller SAPO-34 crystals using less SDA when compared to other SDAs in the same molar composition. However, the high cost of TEAOH may limit the practical catalyst of large-scale production in industry.[Citation12,Citation15,Citation37–40]
Mixing several templates into the synthesis gel to direct the formation of zeolites has proven to be an effectively low-cost way to synthesize SAPO-34 catalysts with small crystal size.[Citation41] Many authors[Citation19,Citation23,Citation37,Citation42,Citation43] have obtained spherical aggregation of nanosized SAPO-34 crystals using TEAOH and morpholine (MOR) as mixed templates, when compared with using MOR alone. The use of mixed template reduces particle size and cost.[Citation26]
Synthesis Processes
Hydrothermal Synthesis
Hydrothermal synthesis consists in a direct synthesis preparing a gel with the Si, Al, and P sources under autogenous pressure using a Teflon autoclave, which is the main and simpler process used to obtain SAPO-34.[Citation10,Citation11,Citation23]
Dry-Gel Synthesis
Dry-gel synthesis follows hydrothermal synthesis, although prior to transfer the freshly prepared gel, it is dried. The resultant material is then placed in the Teflon autoclave, with a water amount following a pre-established ratio. The heat treatment generates the final solid. This technique tends to promote smaller crystals than the hydrothermal process due to a higher gel concentration during the heat treatment, which favors nucleation instead of crystal growth producing small crystals with high purity.[Citation12,Citation13,Citation44]
Microwave Synthesis
Microwave synthesis follows the same procedure as the hydrothermal synthesis, differing only in the heat source. However, the heating rate used shortens the crystallization time of nanoporous molecular sieves, and results in a more homogeneous heating, avoiding the temperature gradient encountered during the conventional hydrothermal synthesis. Therefore, the microwave heating technique has been regarded as an effective way to synthesize defect-free, uniform and small-sized crystals due to the high concentration of nuclei and accelerated homogenous nucleation through rapid and uniform heating.[Citation19,Citation45,Citation46]
Crystal Growth Inhibitor-Assisted Synthesis
Crystal growth inhibitor-assisted synthesis consists of using one or more crystal growth inhibitors, such as β-cyclodextrin or Tween-20, the most common, to control and to favor nucleation in detriment of crystal growth, resulting in small crystal size that can be tuned according to the desired size.[Citation47,Citation48]
Post-Synthesis Treatment
The SAPO-34 microporous structure presents serious diffusion limitations and results in rapid catalyst deactivation due to carbon deposits. Creating meso- and/or macro-pores into the microporous SAPO-34 structure produce a hierarchical SAPO-34. This is considered as an effective way to suppress catalyst deactivation. The use of post-synthesis treatment can generate a secondary porosity by depriving the micropores using etching processes by acid, base and/or other chemical reagents.[Citation49–53]
The obtained solids are characterized by x-ray diffraction (XRD), as presented in by the typical diffraction peaks of the CHA structure, where 2θ at 9.5°, 12.5°, 16.6°, 20.5°, 26° and 31.2°Correspond to the (101), (110), (021), (211), (220) and (401) planes, respectively, as related to the interplanar spacing (dhkl). The N2 adsorption and desorption for the textural properties (surface area, pore size, pore volume) thought to be isotherms, is presented in showing a type 1 isotherm characteristic of microporous materials. Temperature programmed desorption of NH3 was also studied. It consists in determining the total surface acidity by injecting a known amount (volume and concentration) of NH3 to be adsorbed by the zeolite, which is related to the acid sieves. Static laser light scattering (SLS), also called laser diffraction or dynamic light scattering (DLS), is used to determine the average particle size, and scanning electron microscopy (SEM) allows to visualize these particles and how they are present dispersed in the membrane.[Citation9,Citation54–60]
Figure 2. Crystallographic axes of SAPO-34 (CHA)[Citation54] and microscopy presenting the shape of chabazite SAPO-34.
![Figure 2. Crystallographic axes of SAPO-34 (CHA)[Citation54] and microscopy presenting the shape of chabazite SAPO-34.](/cms/asset/c494917f-77ca-4341-911f-239d02f6092b/lspr_a_2204080_f0002_oc.jpg)
Figure 3. Chabazite (CHA) XRD pattern characteristic of the SAPO-34 zeolite. Adapted from.[Citation9,Citation55] .
![Figure 3. Chabazite (CHA) XRD pattern characteristic of the SAPO-34 zeolite. Adapted from.[Citation9,Citation55] .](/cms/asset/cbc622f3-4e87-44cf-96ad-9267ac9b9839/lspr_a_2204080_f0003_oc.jpg)
SAPO-34 as Filler of Mixed Matrix Membranes for Gas Separation
The permeability coefficient of a gas i (Pi) is the product of the gas flux Ji and membrane thickness, l, divided by the pressure difference across the membrane, Δpi, as presented in Eq. 1. For MMMs, the main influences are the molecular sieving of a gas through the filler pores, according to the connecting diameter, and its solubility in the polymeric matrix.[Citation1,Citation61]
While the selectivity, α, is the ratio of the permeability coefficients of the two gases, where Pa is the permeability of the more permeable gas and Pb is the permeability of the less permeable gas in a binary mixture as presented in Eq. 2.[Citation61–64]
When a gas mixture is brought to a membrane surface by any driving force, there is an accumulation of the less permeable species and a depletion of the more permeable components in the boundary layer adjacent to the membrane and this causes a concentration gradient building up in the boundary layer, as shown in . This phenomenon is referred to as concentration polarization. It exists in all membrane separation processes because of the selectivity of the membrane. This has serious adverse effects on membrane separation processes, leading to a decrease in the available driving force for the more permeable species across the membrane and an increase for the less permeable species, reducing the overall efficiency of separation and raising the costs of capital and operation.[Citation61–63]
Figure 5. Permeation effect for the (a) more permeable gas and (b) less permeable gas at the membrane surface.

Since zeolites have a well-controlled pore diameter, which is the main requirement of using them as fillers in membrane production, they can effectively separate gases even with small difference of kinetic diameter as presented in . The use of zeolites and other silicate derivatives as constituents of MMMs in the separation of gases, such as H2, CO2, CH4, N2, CO, O2, H2O, among others, did lower the energy consumption, being tunable and compatible with traditional processes. The addition of a filler in these membranes promotes a better separation at the molecular level according to the kinetic diameter of the gases, and gives permeability and selectivity above the Robeson upper limit. The Robeson upper bound limit was a study performed by Robeson, who reunited permeability references for polymeric membranes, compiled and plotted the limits for a series of gases serving as reference for further efficient separation processes of a binary mixture using different types of membranes. The limit was revised in 2008 and 2019. The Robeson upper bound limits are presented in and compared with some MMMs using SAPO-34 as filler.[Citation21,Citation62–64,Citation69–71]
Figure 7. Selectivity comparison between mixed matrix polymer membranes doped with SAPO-34 and the 2008[Citation63,Citation65–67] and 2019[Citation68] upper limits proposed by Robeson for left CO2 and CH4, and right CO2 and N2.
![Figure 7. Selectivity comparison between mixed matrix polymer membranes doped with SAPO-34 and the 2008[Citation63,Citation65–67] and 2019[Citation68] upper limits proposed by Robeson for left CO2 and CH4, and right CO2 and N2.](/cms/asset/cdd4bb17-5662-4f73-a38a-81878b691f55/lspr_a_2204080_f0007_oc.jpg)
Although several studies in the production of MMM using SAPO-34 as the dispersed solid presented excellent separation results, the main problem in these membranes is the compatibility between particles, as inorganic components and the polymer matrix, as an organic component. Priming prior to the preparation of the polymeric solution, which consists in adding a small quantity of the polymer that will be used to promote a better dispersion without a sudden change in the solution density and viscosity, is performed by many authors. However, defects in the solid-polymer interface still remain present.[Citation21,Citation65–76] Therefore, modifications of the zeolite surface could lead to a better dispersion, better interaction filler/matrix and reduction of defects in the obtained MMM.
Wettability is another important factor in membranes that depends on their structural characteristics, operating conditions, the nature and adsorbent properties. In fact, the membrane pore size has a direct effect on the membrane wettability as reported in the Laplace equation. Thus, membranes with small pore size may have higher wetting resistance.[Citation77–80] On the other hand, water may play a very important role in reversing the MMM selectivity in CO2/N2 separation due to a more stable interaction between CO2 and H2O which causes slower diffusion than N2 in a wet membrane.[Citation81]
Surface Modifications
Surface functionalization of zeolites improves the zeolite-polymer matrix interaction. The functional groups act as a bound that promotes a better link and diminishes interfacial problems. Amine groups, silane groups and ionic liquids (ILs) are proposed to improve the interaction between these components.[Citation65,Citation66,Citation82–85] The superficial modification of SAPO-34 intends to increase the separation performance of MMMs containing SAPO-34 in gas separation.[Citation72,Citation74,Citation86]
Amino Groups
Adding amine groups to the zeolite surface can increase the interaction between dispersed particles and the polymeric matrix while also improving the CO2 solubility due a preferential adsorption mechanism. Venna and Carreon[Citation87] used different amino groups, ethylenediamine (EDA), hexylamine (HA), and octylamine (OA) to modify the SAPO-34 surface in an MMM on tubular porous stainless-steel supports to tolerate high pressure and temperature. EDA showed a better result in the modification compared to other amino groups in this study, promoting a 40% and 167% increase in the separation performance of CO2/CH4 and CO2/N2, respectively. The increases in separation performance are related mainly to preferential adsorption of CO2 on a basic SAPO-34 surface as presented in .
Figure 8. Amino group grafting. Adapted from.[Citation28] .
![Figure 8. Amino group grafting. Adapted from.[Citation28] .](/cms/asset/44811944-1b1c-4f82-bba2-9c216932b350/lspr_a_2204080_f0008_oc.jpg)
Ahmad et al.[Citation75,Citation88] studied the effect of ethylenediamine (EDA) and hexylamine (HA) functionalized SAPO-34 in polyethersulfone, reporting that the use of EDA promotes a higher amino group grafting on the SAPO-34 surface when compared to HA. The use of EDA showed a better compatibility between filler and polymer that resulted in better separation performance for CO2/CH4 when compared to a nonfunctionalized membrane. However, HA has not shown an improvement in separation. These works demonstrated that the modification of the surface of SAPO-34 can effectively improve the separation performance, according to the basicity developed by the amine group on the surface of the solid.
Silane Groups
The use of silane groups chemically modifies the surface of the zeolites, which changes the surface properties for a better compatibility and preferential CO2 adsorption. This modification usually leads the zeolite surface to a hydrophobic characteristic,[Citation70] improving the stability in wet conditions to separate CO2 from other gases in a mix stream. Although, some of the silane modifications are reported to rise the CO2 adsorption characteristics according to the silane group used, mainly those with a primary or secondary nitrogen in the carbon chain acting as preferential CO2 adsorbents like amino groups, but chemically adhered to the zeolite surface.[Citation28,Citation89–93]
Junaidi et al.[Citation71] studied the effects of 3-aminopropyltrimethoxysilane (APMS) modified SAPO-34 on the synthesis of a polysulfone (PSf) MMM, showing that the use of ethanol as solvent promoted a higher grafting to incorporate the silane group on SAPO-34 zeolites and gave higher CO2/N2 and CO2/CH4 selectivities of 135% and 124%, respectively, when compared to the pure PSf membrane, PSf/SAPO-34 membranes without APMS, and the use of isopropyl alcohol (IPA) as solvent. However, the use of ethanol also promotes a reduction in surface area.
Zhao et al.[Citation92] investigated the use of 3-(2-aminoethylamino)propyl-dimethoxy-methylsilane (APDS) as a surface modifier to increase the compatibility on sheet-like SAPO-34 zeolite filler in a PEBAX MH 1657 polymer, showing how important is the balance between the strength of the interactions between the filler and matrix. A weak interaction leads to a low compatibility and interfacial voids while an extremely strong interaction could block the pores of the zeolites drastically reducing the flux through the MMM.
Although the use of silane groups is a viable way to promote CO2 separation, silane coupling agents with two or three alkoxy groups may cause the formation of a massive number of coupling points on the external surface of zeolitic fillers, resulting in pore blockage. For example, Ismail et al.[Citation94] modified a zeolite 4A using aminopropyl-triethoxysilane (APTES) obtaining good compatibility between the modified zeolite and PES membrane resulting in an improvement in CO2/CH4 selectivity. However, the CO2 permeability was reduced by almost 80% when compared to a pure PES membrane. Therefore, it is very important to choose a suitable silane coupling agent for surface modification of zeolites to favorably improve the interfacial adhesion, as well as the separation performances of the MMM.
Ionic Liquids
Ionic liquids (IL) are liquid organic salts composed of an organic cation and an inorganic polyatomic anion.[Citation95–99] The use of ILs with high affinity and selectivity to CO2 onto the particle surface and then dispersing the modified particles in the polymer membrane may enhance the separation characteristics since ILs can promote a higher CO2 solubility due to a preferential CO2 adsorption in the zeolite surface, resulting in better permeation and selectivity properties. As intermediates, ILs can improve the zeolite–polymer interaction (), as studied by many researchers. Ahmad et al.[Citation100] reported modifications of PSf MMMs incorporated with SAPO-34 using [emim][Tf2N], an IL highly selective to CO2, which increased the CO2/CH4 selectivity of the PSf/SAPO-34 MMM by more than 400% compared to the unmodified membrane, determining that the use of the IL surface modifiers could affect the textural properties of the SAPO-34. In addition, the IL could interact with the zeolite surface in the same way as the amine groups due to electrostatic interactions.
Figure 9. Zeolite-Ionic liquid interaction.[Citation74,Citation95] .
![Figure 9. Zeolite-Ionic liquid interaction.[Citation74,Citation95] .](/cms/asset/cec7c78c-1afb-42bd-9d49-7633cd6f7065/lspr_a_2204080_f0009_oc.jpg)
shows SEM observations of PES/SAPO-34 and PES/SAPO-34 modified with [emim][Tf2N]. The PES/SAPO-34 presents voids between the interface of the polymer and zeolite particles. Although it is possible to assume that an increase in selectivity after a surface modification could be caused by that modification, it cannot be stated that one surface modification is better than the others based only in the post-treatment selectivity since the membrane obtained can vary by many factors. It is interesting to study all different superficial modifications in the same MMM with similar initial values of permeabilities and selectivities.[Citation74] Since the Ahmad et al.[Citation100] work only contemplates a small case-study, several other factors that affect the physical properties of IL-modified zeolite, such as IL selection and loading, could be studied in future works. Moreover, smaller particle size and zeolite crystalline modifications could also be included in the proposed future works, since it could affect the dispersion and the interactions with ILs.
Nasir et al.[Citation74] examined the effects of the inclusion of two different surface modifiers, [emim][Tf2N] and amine groups (EDA and HA), in the functionalization process of SAPO-34 to increase the CO2/CH4 selectivity in MMM of PES/SAPO-34. They noticed a decrease in CO2 permeation due to a blocking effect.[Citation74] This blockage resulted from a reduction in the availability of the pores in the zeolite caused by the presence of large molecules on the zeolite surface. This phenomenon is also present in other works, for example, the use of IL after membrane synthesis for an interfacial sealing as proposed by Ahmad et al.[Citation86] using [bmim][Ac] in PSf/SAPO-34 membrane resulted in an increase of more than 800% in CO2/N2 selectivity compared to unmodified MMM. However, this work also showed that the pore volume of SAPO-34 can be affected after being modified by large molecules, narrowing the pore of SAPO-34 zeolite, and restricting the diffusion of gases. For example, the N2 permeance in a membrane post-sealed with 0.4 M [bmim][Ac] reduced by 98% when compared to an unmodified membrane. Even though the use of zeolite surface modifiers (amino groups, silane groups and ILs) increases the separation properties, an in-depth study needs to be performed to optimize the compatibility as stated by many authors.[Citation65,Citation66,Citation74,Citation82,Citation86,Citation100–104] presents some studies using different zeolite surface modifiers and the obtained results for CO2/CH4 and CO2/N2 separations.
Table 1. Different MMM studies with CO2 permeability, CO2/CH4 and CO2/N2 separations using SAPO-34 with different surface modifiers.
Table 2. Different MMM studies with CO2 permeance, CO2/CH4 and CO2/N2 separations using SAPO-34 with different surface modifiers.
CURRENT DEVELOPMENT PERSPECTIVES OF MMMS FUNCTIONALIZED WITH SAPO-34
The use of zeolites remains important in MMM development since it shows a high thermal and chemical stability, commercial availability and is capable of a well-controlled pore structure. The properties of zeolite, such as polarity, are dependent on its chemical composition. SAPO-34, zeolite 4A and ZSM-5 are examples of common zeolite in MMM fabrication presenting more polarity due to a higher Si/Al ratio and having a stronger interaction with CO2.[Citation22,Citation69]
The use of a glassy matrix polymer, such as polyhexafluoropropylene (PHFP) with SAPO-34 nanoparticles, is a recent study and represents a novelty in this line. The polymeric matrix characteristics of the PHFP displayed a higher permeation in comparison with the PHFP membrane without SAPO-34 filling, which indicated how SAPO-34 can improve the separation properties in a material.[Citation111] The use of a denser polymeric material forces the gas flux to pass through the zeolite pores, which resulted in reduction in permeation, although it can increase the selectivity for a specific gas. On the other hand, an increase in permeation that leads to a reduction in selectivity could indicate the presence of interfacial voids.[Citation22]
Wu et al.[Citation105] studied the use of nanosized SAPO-34 crystals (100 and 200 nm) in PES to prepare MMMs. The use of nanosized SAPO-34 can increase the separation performance since it leads to a better dispersion. However, they[Citation105] determined that the use of crystals smaller than 200 nm exhibits a high level of agglomeration causing nonselective defects at the interface between particles, leading to a decrease in the separation performance verified by SEM and permeation analyses.
Liu et al.[Citation112] investigated the gas diffusivity and solubility in PES MMM containing commercial SAPO-34. The results revealed that commercial SAPO-34 presented a better separation performance because of less defects than laboratory-grade SAPO-34, concluding that commercial SAPO-34 with an appropriate surface modification could improve the performance in MMMs. However, those solids could not be submitted to doping processes or other procedures to change the zeolite characteristics.
Dense polyimide is used as a polymer matrix that can promote an interaction with a high electron density structure that could lead to a higher CO2 solubility. Wu et al.[Citation113] used 4,4′-(hexafluoroisopropylidene) diphthalic anhydride (6FDA) combined with 1,3-diamino-2,4,6-trimethylbenzene (TrMPD) as the polymeric matrix doped with 30% w/w SAPO-34 to obtain a new MMM. The results obtained showed a 121% increase in CO2 permeation when compared to the neat membrane. However, the separation performance of CO2/CH4 and CO2/N2 was under the 2008 and 2019 Robeson upper bounds.
As presented, the use of silane groups can be a major modification of the SAPO-34 surface promoting a better interaction with the polymer matrix. Zhao et al.[Citation92] used 3-(2-aminoethylamino)propyl-dimethoxymethylsilane (APDS) to modify the SAPO-34 surface for a better compatibility with the Pebax MH 1657 polymer matrix, using 8% w/w loading of the modified zeolite in the MMM. The results presented a highly stable separation of CO2/CH4. The CO2 permeation increased by 49% and the selectivity CO2/CH4 increased by 96% when compared to the neat polymer, which is between Robeson upper bound of 2019 and 2008.
The use of ILs to enhance CO2 separation in membranes, as a polymer/IL composite membrane, is due to the high solubility of CO2 in selected ILs. In that way, the IL, when casted with the polymer, is trapped inside the polymer chain. Several studies have shown that the use of ILs can increase the permeation of CO2 in different polymer matrixes.[Citation22,Citation85,Citation114–119] Jansen et al.[Citation118] studied the effects of high contents (20–80%w/w) of 1-ethyl-3-methylimidazolium bis(trifluoromethylsulfonyl)imide ([emim][Tf2N]) in poly(vinylidene fluorideco-hexafluoropropylene) (p(VDF-HFP)) and stated that increasing the IL content led to a more flexible sample and this favored the transport of methane, N2 and CO2. The increase of IL content promoted a slight decrease in CO2/N2 and CO2/CH4 selectivities while the H2/N2 selectivity strongly decreased. The separation mechanism changed from a diffusion-controlled process to a solubility-controlled process. The same fact was reported by Uchytil et al..[Citation119,Citation120] However, this change in the separation mechanism led Jansen et al.[Citation118] to obtain a highly effective separation of CO2/H2 in high IL content (above 40% w/w) due to the preferential permeance of CO2 rather than H2.
Following these works, Rabiee et al.[Citation85] used poly(ether-b-amide6) (Pebax1657) as polymer matrix with [emim][BF4] IL content 20–100%w/w, obtaining similar results as observed by Jansen et al..[Citation118] They reported that the addition of IL, as a low molecular weight additive, caused a plasticization effect on the membrane body, thus leading to greater chain mobility and, consequently, penetrant gases diffused faster, and the permeation increased for all IL levels tested.[Citation85] Estahbanati et al.[Citation114] obtained similar results when using the same polymer matrix (Pebax1657) and IL (emim][BF4]), showing a high increase in CO2 permeability, however, a low increase in CO2/CH4 and CO2/N2 selectivities of 17% and 34%, respectively, for a 50% w/w IL in Pebax1657.
Mohshim et al.[Citation102] studied the effects of [emim][Tf2N] additions in polyethersulfone (PES) membrane on CO2/CH4 separation. The results showed a dense structure due to a high compatibility of [emim][Tf2N] over PES with a high increase in CO2 permeation with the addition of 5–15% w/w IL when compared with the neat membrane. However, the CH4 permeation also increased for the PES/[emim][Tf2N]. Similar results were obtained by Mannan et al.[Citation120] who also stated that more than 20% w/w IL in PES caused a small increase in CO2 permeability and CO2/CH4 selectivity.
The addition of SAPO-34 in the casting process, to prepare MMMs, presents remarkable separation performance due to the combination of a higher interaction of the membrane with CO2 and a higher CO2 solubility related to the presence of IL, as it is reported by several recent works using different matrix polymer and different ILs to evaluate the best combination.[Citation22,Citation36] ILs can also be used in post-sealing in MMM to remove void imperfections and increase CO2 permeation, as described by different researchers.[Citation86,Citation93,Citation121,Citation122] Mohshim et al.[Citation103] studied the incorporation of 5–20% w/w of [emim][Tf2N] in PES MMM containing 20% w/w of SAPO-34. The results showed that the range of 5–20% w/w of [emim][Tf2N] increased CO2 permeance in the range of 170–253% at 30 bar, also the selectivity of CO2/CH4 increases in the range of 130–210%. The results have demonstrated that ILs increased the separation performance by acting as a wetting agent to improve the interfacial adhesion between SAPO-34 and polymer, producing defect-free membranes. This wetting agent promotes a better dispersion of the SAPO-34 crystals in the polymeric matrix, which reduces the agglomeration of small crystals, the main responsible for the presence of voids in the membrane. Dong et al.[Citation123] showed that the use of mesoporous materials and surface modification using silane coupling groups can also act as wetting agents and promote a better zeolite dispersion.
Similar results were obtained by Nasir et al. (2018)[Citation74] in studying the same PES/SAPO-34/IL membrane with 4% w/w IL load and comparing the results obtained with PES/SAPO-34/IL with SAPO-34 doped with EDA and HA. The results showed that the inclusion of 4% w/w IL reduced the CO2 permeation due to a plasticization effect, a rigidified area around SAPO-34 particles; however, the CO2/CH4 selectivity was increased by up to 111%. The addition of EDA and HA increased that plasticization effect, with a lower CO2 permeability and an increase in CO2/CH4 selectivity from 26.4 to 37.2, although this selectivity is still lower than that reported by Mohshim et al.,[Citation103] who obtained a CO2/CH4 selectivity of 46.2 for a 5% w/w IL loading.
At the same time, Ahmad et al.[Citation100] studied the influence of SAPO-34 doped with [emim][TF2N] in PSf polymer matrix. However, these authors used a different approach to modify the SAPO-34 surface with IL. Instead of only mixing the SAPO-34 and IL. The zeolite was mixed with ethanol, and then the IL was added, centrifuged, washed with ethanol and dried at 60ºC. Five percent w/w of the modified zeolites were dispersed in PSf polymeric matrix. The results demonstrated that the inclusion of unmodified SAPO-34 in PSf enhanced the CO2 permeability but reduced the CO2/N2 selectivity due to defects in the interface polymer/zeolite. Despite that, the modified SAPO-34 showed a 14% decrease in CO2 permeance, CO2/CH4 and CO2/N2 selectivity increased 50% and 31%, respectively, as compared to the neat PSf membrane, possibly attributed to the improved interfacial adhesion between the polymer and particles.
Recently, Shafie et al.[Citation124] used 1%, 2%, and 3% w/w of modified silica particles with [emim][Tf2N] in polycarbonate (PC) MMM, using a similar surface modification procedure as Ahmad et al..[Citation95] The results showed an increase in CO2 permeability and CO2/CH4 selectivity compared to the pure PC membrane at below 6 bar. PC-Sil-IL with 3% w/w IL modified silica particles showed the most promising result with 400% higher CO2/CH4 selectivity, surpassing the 2008 Robeson upper bound but staying below the 2019 redefined upper bound.
Since deep eutectic solvents (DESs) have emerged as a low-cost alternative for ILs, the use of those solvents in MMMs shows a new route that can be studied. Jiang et al.[Citation125] used tetra-butylammonium chloride/imidazole, a DES, to functionalize PES membranes for bovine serum albumin (BSA) ultrafiltration. The results showed that the addition of DES to the casting solutions improved the membrane porous structure, contributing to a remarkably enhanced permeability and a high selectivity of the resultant membranes with a maximum water flux of 6.45 times higher than the additive-free membrane and a high BSA rejection of 97.7% at 2 bar. Craveiro et al.[Citation126] prepared a PTFE membrane to support different DESs. The supported liquid membranes exhibited higher permeability toward CO2, especially using choline chloride and urea in ratio 1:2. The membrane containing this DES also showed high selectivity for CO2/CH4, with values above the 2008 Robeson upper bound. Thus, the use of DESs as post-sealing agent can be studied in future work.
Conclusions
It has been stated by many researchers that MMMs present a great potential for further investigation and development of the separation performance. The use of fillers like SAPO-34 to improve the separation characteristics is a well-studied case and can greatly increase membrane performance in gas separation. The development of surface modification of fillers using amine groups, silane groups and ionic liquids leads to a decrease in permeation, despite an increase in selectivity, and most important, an increase in polymer/filler compatibility and in filler dispersion in the polymeric matrix. However, the number of polymers and fillers that can be matched shows the lack of studies in this subject, especially in the field of particle/polymer interaction to mitigate interfacial voids, since the combination of the three parts, filler, surface modifier and polymer, will define the final characteristics of the membrane according to the strength of the interaction. This strength must be just enough to mitigate the presence of voids, since the use of stronger interaction between zeolite and surface modifier can potentially block the zeolite pores, leading to a reduction in permeation and selectivity.
A more extensive work regarding which of the modification could result in better final performance separation, using the same MMM with similar initial permeabilities and selectivities and promoting different superficial modifications to the filler, obtaining the final performance in separation should also be considered since permeability and selectivity differ in many papers making impossible to determine, at this point, the best surface modification.
The use of deep eutectic solvents can be an alternative to ILs in a more economical and environmentally-friendly synthesis. These solvents could be used during the solution preparation or as a post-sealing impregnation to improve the PES/SAPO-34 separation performance. Post-impregnation is a way to heal MMM with poor compatibility after synthesis. Few studies have reported the use of ILs for that procedure in MMM, and some works have used DESs in simple polymeric membranes. Thus, no studies have tried the same procedure in MMM, which shows that this area is still under development.
Abbreviation list
[bmim][Ac] | = | 1-Butyl-3-methylimidazolium acetate |
[emim][BF4] | = | 1-Ethyl 3 methyl imidazolium tetrafluoroborate |
[emim][Tf2N] | = | 1-Ethyl-3-methylimidazolium bis(trifluoromethylsulfonyl)imide |
6FDA | = | 4,4′-(Hexafluoroisopropylidene) diphthalic anhydride |
APDS | = | Dimethoxymethylsilane |
APMS | = | 3-aminopropyltrimethoxysilane |
APTES | = | Aminopropyltriethxysilanes |
BSA | = | Bovine serum albumin |
CHA | = | Chabazite structure |
DEA | = | Diethylamine |
DES | = | Deep eutectic solvent |
DLS | = | Dynamic light scattering |
EDA | = | Ethylenediamine |
HA | = | Hexylamine |
IL | = | Ionic Liquid |
IPA | = | Isopropyl alcohol |
MMM | = | Mixed Matrix Membranes |
MOR | = | Morpholine |
OA | = | Octylamine |
p(VDF-HFP) | = | Poly(vinylidene fluoride-co-hexafluoropropylene) |
PC | = | Polycarbonate |
PES | = | Polyethersulfone |
PHFP | = | Polyhexafluoropropylene |
PSf | = | Polysulfone |
PTFE | = | Polytetrafluoroetilene |
SAPO | = | Silicoaluminophosphate |
SDA | = | Structure Directing Agent |
SEM | = | Scanning Electron Microscopy |
SLS | = | Static laser light scattering |
TEA | = | Triethylamine |
TEAOH | = | Tetraethylammonium hydroxide |
TrMPD | = | 1,3-Diamino-2,4,6-trimethylbenzene |
XRD | = | X-Ray diffraction |
Disclosure statement
No potential conflict of interest was reported by the authors.
Additional information
Funding
References
- Amaral, R. A.; Habert, A. C.; Borges, C. P. Performance Evaluation of Composite and Microporous Gas–Liquid Membrane Contactors for CO2 Removal from a Gas Mixture. Chem. Eng. Process. 2016, 102, 202–209. DOI: 10.1016/j.cep.2016.01.018.
- Primo, A.; Garcia, H. Zeolites as Catalysts in Oil Refining. Chem. Soc. Rev. 2014, 43(22), 7548–7561. DOI: 10.1039/C3CS60394F.
- Hölderich, W. F.; Röseler, J.; Heitmann, G.; Liebens, A. T. The Use of Zeolites in the Synthesis of Fine and Intermediate Chemicals. Chem. Eng. Process. 1997, 37(4), 353–366. DOI: 10.1016/S0920-5861(97)81094-2.
- Zimmerman, C. M.; Singh, A.; Koros, W. J. Tailoring Mixed Matrix Composite Membranes for Gas Separations. J. Memb. Sci. 1997, 137(1–2), 145–154. DOI: 10.1016/S0376-7388(97)00194-4.
- Davis, M. E.; Lobo, R. F. Zeolite and Molecular Sieve Synthesis. Chem. Mater. 1992, 4(4), 756–768. DOI: 10.1021/cm00022a005.
- Chang, C. D.; Lang, W. H.; Smith, R. L. The Conversion of Methanol and Other O-Compounds to Hydrocarbons Over Zeolite Catalysts. J. Catal. 1977, 47(2), 249–259. DOI: 10.1002/chin.197918119.
- Twaiq, F. A.; Zabidi, N. A. M.; Bhatia, S. Catalytic Conversion of Palm Oil to Hydrocarbons: Performance of Various Zeolite Catalysts. Ind. Eng. Chem. Res. 1999, 38(9), 3230–3237. DOI: 10.1021/ie980758f.
- Tan, X.; Robijns, S.; Thür, R.; Ke, Q.; De Witte, N.; Lamaire, A.; Li, Y.; Aslam, I.; Havere, D. V.; Donckels, T., et al. Truly Combining the Advantages of Polymeric and Zeolite Membranes for Gas Separations. Science. 2022, 378(6625), 1189–1194. DOI: 10.1126/science.ade1411.
- Sena, F. C.; De Souza, B. F.; De Almeida, N. C.; Cardoso, J. S.; Fernandes, L. D. Influence of Framework Composition Over SAPO-34 and MeAPSO-34 Acidity. Appl. Catal. A. 2011, 406(1–2), 59–62. DOI: 10.1016/j.apcata.2011.08.010.
- Liu, G.; Tian, P.; Li, J.; Zhang, D.; Zhou, F.; Liu, Z. Synthesis, Characterization and Catalytic Properties of SAPO-34 Synthesized Using Diethylamine as a Template. Microporous. Mesoporous. Mater. 2008, 111(1–3), 143–149. DOI: 10.1016/j.micromeso.2007.07.023.
- Liu, G.; Tian, P.; Liu, Z. Synthesis of SAPO-34 Molecular Sieves Templated with Diethylamine and Their Properties Compared with Other Templates. Chin. J. Catal. 2012, 33(1), 174–182. DOI: 10.1016/S1872-2067(10)60325-2.
- Li, J.; Li, Z.; Han, D.; Wu, J. Facile Synthesis of SAPO-34 with Small Crystal Size for Conversion of Methanol to Olefins. Powder Technol. 2014, 262, 177–182. DOI: 10.1016/j.powtec.2014.04.082.
- Hirota, Y.; Murata, K.; Tanaka, S.; Nishiyama, N.; Egashira, Y.; Ueyama, K. Dry Gel Conversion Synthesis of SAPO-34 Nanocrystals. Mater. Chem. Phys. 2010, 123(2–3), 507–509. DOI: 10.1016/j.matchemphys.2010.05.005.
- Askari, S.; Halladj, R.; Sohrabi, M. An Overview of the Effects of Crystallization Time, Template and Silicon Sources on Hydrothermal Synthesis of SAPO-34 Molecular Sieve with Small Crystals. Rev. Adv. Mater. Sci. 2012, 32(2), 83–93. https://www.ipme.ru/e-journals/RAMS/no_23212/01_23212_askari.html
- Askari, S.; Sedighi, Z.; Halladj, R. Rapid Synthesis of SAPO-34 Nanocatalyst by Dry Gel Conversion Method Templated with Morpholine: Investigating the Effects of Experimental Parameters. Microporous. Mesoporous. Mater. 2014, 197, 229–236. DOI: 10.1016/j.micromeso.2014.06.028.
- Prakash, A. M.; Unnikrirhnan, S. Synthesis of SAPO-34: High Silicon Incorporation in the Presence of Morpholine as Template. J. Chem. Soc. Faraday Trans. 1994, 90(15), 2291–2296. DOI: 10.1039/FT9949002291.
- Sun, Q.; Wang, N.; Guo, G.; Yu, J. Ultrafast Synthesis of Nano-Sized Zeolite SAPO-34 with Excellent MTO Catalytic Performance. Chem. Commun. 2015, 51(91), 16397–16400. DOI: 10.1039/c5cc07343j.
- Gao, B.; Yang, M.; Qiao, Y.; Li, J.; Xiang, X.; Wu, P.; Wei, Y.; Xu, S.; Tian, P.; Liu, Z. A Low-Temperature Approach to Synthesize Low-Silica SAPO-34 Nanocrystals and Their Application in the Methanol-To-Olefins (MTO) Reaction. Catal. Sci. Technol. 2016, 6(20), 7569–7578. DOI: 10.1039/c6cy01461e.
- Zhong, J.; Han, J.; Wei, Y.; Tian, P.; Guo, X.; Song, C.; Liu, Z. Recent Advances of the Nano-Hierarchical SAPO-34 in the Methanol-To-Olefin (MTO) Reaction and Other Applications. Catal. Sci. Technol. 2017, 7(21), 4905–4923. DOI: 10.1039/c7cy01466j.
- Luna, F. J.; Schuchardt, U. Modificação de zeólitas para uso em catálise. Quim. Nova. 2001, 24(6), 885–892. DOI: 10.1590/S0100-40422001000600027.
- Li, S.; Falconer, J. L.; Noble, R. D. Improved SAPO-34 Membranes for CO2/CH4 Separations. Adv. Mater. 2006, 18(19), 2601–2603. DOI: 10.1002/adma.200601147.
- Usman, M. Recent Progress of SAPO-34 Zeolite Membranes for CO2 Separation: A Review. Membranes. 2022, 12(5), 507. DOI: 10.3390/membranes12050507.
- Salmasi, M.; Fatemi, S.; Hashemi, S. J. MTO Reaction Over SAPO-34 Catalysts Synthesized by Combination of TEAOH and Morpholine Templates and Different Silica Sources. Sci. Iran. 2012, 19(6), 1632–1637. DOI: 10.1016/j.scient.2012.04.019.
- Rimaz, S.; Kosari, M.; Zarinejad, M.; Ramakrishna, S. A Comprehensive Review on Sustainability-Motivated Applications of SAPO-34 Molecular Sieve. J. Mater. Sci. 2022, 57(2), 848–886. DOI: 10.1007/s10853-021-06643-1.
- Pacheco, M. J.; Vences, L. J.; Moreno, H.; Pacheco, J. O.; Valdivia, R.; Hernández, C. Review: Mixed-Matrix Membranes with CNT for CO2 Separation Processes. Membranes. 2021, 11(6), 457. DOI: 10.3390/membranes11060457.
- Sun, Q.; Xie, Z.; Yu, J. The State-Of-The-Art Synthetic Strategies for SAPO-34 Zeolite Catalysts in Methanol-To-Olefin Conversion. Natl. Sci. Rev. 2018, 5(4), 542–558. DOI: 10.1093/nsr/nwx103.
- Xu, J.; Haw, K. G.; Li, Z.; Pati, S.; Wang, Z.; Kawi, S. A Mini-Review on Recent Developments in SAPO-34 Zeolite Membranes and Membrane Reactors. Reaction Chem. Eng. 2021, 6(1), 52–66. DOI: 10.1039/d0re00349b.
- Makertihartha, I. G. B. N.; Kencana, K. S.; Dwiputra, T. R.; Khoiruddin, K.; Lugito, G.; Mukti, R. R.; Wenten, I. G. SAPO-34 Zeotype Membrane for Gas Sweetening. Rev. Chem. Eng, De Gruyter Open Ltd. 2020. DOI: 10.1515/revce-2019-0086.
- Yang, H.; Xu, Z.; Fan, M.; Gupta, R.; Slimane, R. B.; Bland, A. E.; Wright, I. Progress in Carbon Dioxide Separation and Capture: A Review. J. Environ. Sci. 2008, 20(1), 14–27. DOI: 10.1016/S1001-0742(08)60002-9.
- Bernardo, P.; Drioli, E.; Golemme, G. Membrane Gas Separation: A Review/State of the Art. Ind. Eng. Chem. Res. 2009, 48, 4638–4663. DOI: 10.1021/ie8019032.
- Khalilpour, R.; Mumford, K.; Zhai, H.; Abbas, A.; Stevens, G.; Rubin, E. S. Membrane-Based Carbon Capture from Flue Gas: A Review. J. Clean. Prod. 2015, 103, 286–300. DOI: 10.1016/j.jclepro.2014.10.050.
- Ismail, A. F.; David, L. I. B. A Review on the Latest Development of Carbon Membranes for Gas Separation. J. Memb. Sci. 2001, 193(1), 1–18. DOI: 10.1016/S0376-7388(01)00510-5.
- Aroon, M. A.; Ismail, A. F.; Matsuura, T.; Montazer-Rahmati, M. M. Performance Studies of Mixed Matrix Membranes for Gas Separation: A Review. Sep. Purif. Technol. 2010, 75(3), 229–242. DOI: 10.1016/j.seppur.2010.08.023.
- Zhang, Y.; Sunarso, J.; Liu, S.; Wang, R. Current Status and Development of Membranes for CO2/CH4 Separation: A Review. Int. J. Greenhouse Gas Control. 2013, 12, 84–107. DOI: 10.1016/j.ijggc.2012.10.009.
- Vinoba, M.; Bhagiyalakshmi, M.; Alqaheem, Y.; Alomair, A. A.; Pérez, A.; Rana, M. S. Recent Progress of Fillers in Mixed Matrix Membranes for CO2 Separation: A Review. Sep. Purif. Technol. 2017, 188, 431–450. DOI: 10.1016/j.seppur.2017.07.051.
- Ahmad, N. N. R.; Leo, C. P.; Mohammad, A. W.; Shaari, N.; Ang, W. L. Recent Progress in the Development of Ionic Liquid-Based Mixed Matrix Membrane for CO2 Separation: A Review. Int. J. Energy Res. 2021, 45(7), 9800–9830. DOI: 10.1002/er.6518.
- Zhang, Y.; Ren, Z.; Wang, Y.; Deng, Y.; Li, J. Synthesis of Small-Sized SAPO-34 Crystals with Varying Template Combinations for the Conversion of Methanol to Olefin. Catalysts. 2018, 8(12), 570. DOI: 10.3390/catal8120570.
- Najafi, N.; Askari, S.; Halladj, R. Hydrothermal Synthesis of Nanosized SAPO-34 Molecular Sieves by Different Combinations of Multi Templates. Powder Technol. 2014, 254, 324–330. DOI: 10.1016/j.powtec.2014.01.037.
- Li, M.; Wang, Y.; Bai, L.; Chang, N.; Nan, G.; Hu, D.; Zhang, Y.; Wei, W. Solvent-Free Synthesis of SAPO-34 Nanocrystals with Reduced Template Consumption for Methanol-To-Olefins Process. Appl. Catal. A. 2017, 531, 203–211. DOI: 10.1016/j.apcata.2016.11.005.
- Li, Z.; Martínez-Triguero, J.; Yu, J.; Corma, A. Conversion of Methanol to Olefins: Stabilization of Nanosized SAPO-34 by Hydrothermal Treatment. J. Catal. 2015, 329, 379–388. DOI: 10.1016/j.jcat.2015.05.025.
- Ye, L.; Cao, F.; Ying, W.; Fang, D.; Sun, Q. Methanol Conversion on SAPO-34 Catalysts Synthesized by Tri-Templates. Mater. Res. Soc. Symp. Proc. 2010, 1279, 205–213. DOI: 10.1557/proc-1279-22.
- Lee, Y. J.; Baek, S. C.; Jun, K. W. Methanol Conversion on SAPO-34 Catalysts Prepared by Mixed Template Method. Appl. Catal. A. 2007, 329, 130–136. DOI: 10.1016/j.apcata.2007.06.034.
- Chae, H. J.; Park, I. J.; Song, Y. H.; Jeong, K. E.; Kim, C. U.; Shin, C. H.; Jeong, S. Y. Physicochemical Characteristics of SAPO-34 Molecular Sieves Synthesized with Mixed Templates as MTO Catalysts. J. Nanosci. Nanotechnol. 2010, 10(1), 195–202. DOI: 10.1166/jnn.2010.1487.
- Li, M.; Zhang, J.; Liu, X.; Wang, Y.; Liu, C.; Hu, D.; Zeng, G.; Zhang, Y.; Wei, W.; Sun, Y. Synthesis of High Performance SAPO-34 Zeolite Membrane by a Novel Two-Step Hydrothermal Synthesis + Dry Gel Conversion Method. Microporous. Mesoporous. Mater. 2016, 225, 261–271. DOI: 10.1016/j.micromeso.2015.11.056.
- Bing, L.; Wang, G.; Wang, F.; Liu, X.; Zhang, B. Preparation of a Preferentially Oriented SAPO-34 Membrane by Secondary Growth Under Microwave Irradiation. R.S.C. Adv. 2016, 6(61), 56170–56173. DOI: 10.1039/c6ra09018d.
- Zong, Z.; Carreon, M. A. Thin SAPO-34 Membranes Synthesized in Stainless Steel Autoclaves for N2/CH4 Separation. J. Memb. Sci. 2017, 524, 117–123. DOI: 10.1016/j.memsci.2016.11.011.
- Venna, S. R.; Carreon, M. A. Synthesis of SAPO-34 Crystals in the Presence of Crystal Growth Inhibitors. J. Phys. Chem B. 2008, 112(51), 16261–16265. DOI: 10.1021/jp809316s.
- Shi, H. Synthesis of SAPO-34 Zeolite Membranes with the Aid of Crystal Growth Inhibitors for CO2/CH4 Separation. New. J. Chem. 2014, 38(11), 5276–5278. DOI: 10.1039/c4nj01405g.
- Chen, X.; Vicente, A.; Qin, Z.; Ruaux, V.; Gilson, J. P.; Valtchev, V. The Preparation of Hierarchical SAPO-34 Crystals via Post-Synthesis Fluoride Etching. Chem. Commun. 2016, 52(17), 3512–3515. DOI: 10.1039/c5cc09498d.
- Yang, G.; Han, J.; Huang, Y.; Chen, X.; Valtchev, V. Busting the Efficiency of SAPO-34 Catalysts for the Methanol-To-Olefin Conversion by Post-Synthesis Methods. Chin. J. Chem. Eng. 2020, 28(8), 2022–2027. DOI: 10.1016/j.cjche.2020.05.028.
- Liu, G.; Tian, P.; Xia, Q.; Liu, Z. An Effective Route to Improve the Catalytic Performance of SAPO-34 in the Methanol-To-Olefin Reaction. J. Nat. Gas. Chem. 2012, 21(4), 431–434. DOI: 10.1016/S1003-9953(11)60387-3.
- Zheng, T.; Liu, H.; He, P.; Zhang, R.; Meng, X.; Xu, C.; Liu, H.; Yue, Y.; Liu, Z. Post Synthesis of Hierarchical SAPO-34 via Citric Acid Etching: Mechanism of Selective Desilication. Microporous. Mesoporous. Mater. 2022, 335, 111798. DOI: 10.1016/j.micromeso.2022.111798.
- Jadav, D.; Bandyopadhyay, R.; Bandyopadhyay, M. Synthesis of Hierarchical SAPO-5 & SAPO-34 Materials by Post-Synthetic Alkali Treatment and Their Enhanced Catalytic Activity in Transesterification. Eur. J. Inorg. Chem. 2020, 2020(10), 847–853. DOI: 10.1002/ejic.201901250.
- Shen, B.; Chen, X.; Fan, X.; Xiong, H.; Wang, H.; Qian, W.; Wang, Y.; We, F. Resolving Atomic SAPO-34/18 Intergrowth Architectures for Methanol Conversion by Identifying Light Atoms and Bonds. Nat. Commun. 2021, 12(1), 2212. DOI: 10.1038/s41467-021-22438-z.
- Doan, T.; Nguyen, K.; Dam, P.; Vuong, T. H.; Le, M. T.; Thanh, H. P. Synthesis of SAPO-34 Using Different Combinations of Organic Structure-Directing Agents. J. Chem. 2019, 2019, 6197527. DOI: 10.1155/2019/6197527.
- Gao, F.; Walter, E. D.; Washton, N. M.; Szanyi, J.; Peden, C. H. Synthesis and Evaluation of Cu/SAPO-34 Catalysts for NH 3-SCR 2: Solid-State Ion Exchange and One-Pot Synthesis. Appl. Catal. B. 2014, 162, 501–514. DOI: 10.1016/j.apcatb.2014.07.029.
- Wu, Z.; Ran, R.; Ma, Y.; Wu, X.; Si, Z.; Weng, D. Quantitative Control and Identification of Copper Species in Cu–SAPO-34: A Combined UV–Vis Spectroscopic and H2 -TPR Analysis. Res. Chem. Inter. 2019, 45(3), 1309–1325. DOI: 10.1007/s11164-018-3680-x.
- Chen, H.; Wang, M.; Yang, M.; Shang, W.; Yang, C.; Liu, B.; Hao, Q.; Zhang, J.; Ma, X. Organosilane Surfactant-Directed Synthesis of Nanosheet-Assembled SAPO-34 Zeolites with Improved MTO Catalytic Performance. J. Mater. Sci. 2018, 54(11), 8202–8215. DOI: 10.1007/s10853-019-03485-w.
- Li, G.; Wang, B.; Sun, Q.; Xu, W. Q.; Ma, Z.; Wang, H.; Zhang, D.; Zhou, J. Novel Synthesis of Fly-Ash-Derived Cu-Loaded SAPO-34 Catalysts and Their Use in Selective Catalytic Reduction of NO with NH3. Green Energy Environ. 2019, 4, 470–482. DOI: 10.1016/j.gee.2019.03.003.
- Shamanaev, I. V.; Deliy, I. V.; Gerasimov, E. Y.; Pakharukova, V. P.; Kodenev, E. G.; Aleksandrov, P. V.; Bukhtiyarova, G. A. Synergetic Effect of Ni2P/SiO2 and γ-Al2O3 Physical Mixture in Hydrodeoxygenation of Methyl Palmitate. Catalysts. 2017, 7(11), 329. DOI: 10.3390/catal7110329.
- Freeman, B. D. Basis of Permeability/Selectivity Trade-Off Relations in Polymeric Gas Separation Membranes. Macromolecules. 1999, 32(2), 375–380. DOI: 10.1021/ma9814548.
- Robeson, L. M. Correlation of Separation Factor versus Permeability for Polymeric Membranes. J. Memb. Sci. 1991, 62, 165–185. DOI: 10.1016/0376-7388(91)80060-J.
- Robeson, L. M. The Upper Bound Revisited. J. Memb. Sci. 2008, 320(1–2), 390–400. DOI: 10.1016/j.memsci.2008.04.030.
- Zhao, D.; Ren, J.; Li, H.; Hua, K.; Deng, M. Poly(amide-6-B-Ethylene Oxide)/SAPO-34 Mixed Matrix Membrane for CO2 Separation. J. Energy Chem. 2014, 23(2), 227–234. DOI: 10.1016/S2095-4956(14)60140-6.
- Hudiono, Y. C.; Carlisle, T. K.; Bara, J. E.; Zhang, Y.; Gin, D. L.; Noble, R. D. A Three-Component Mixed-Matrix Membrane with Enhanced CO2 Separation Properties Based on Zeolites and Ionic Liquid Materials. J. Memb. Sci. 2010, 350(1–2), 117–123. DOI: 10.1016/j.memsci.2009.12.018.
- Hudiono, Y. C.; Carlisle, T. K.; LaFrate, A. L.; Gin, D. L.; Noble, R. D. Novel Mixed Matrix Membranes Based on Polymerizable Room-Temperature Ionic Liquids and SAPO-34 Particles to Improve CO2 Separation. J. Memb. Sci. 2011, 370(1–2), 141–148. DOI: 10.1016/j.memsci.2011.01.012.
- Hu, L.; Cheng, J.; Li, Y.; Liu, J.; Zhang, L.; Zhou, J.; Cen, K. Composites of Ionic Liquid and Amine-Modified SAPO 34 Improve CO2 Separation of CO2-selective Polymer Membranes. Appl. Surf. Sci. 2017, 410, 249–258. DOI: 10.1016/j.apsusc.2017.03.045.
- Comesaña-Gándara, B.; Chen, J.; Bezzu, C. G.; Carta, M.; Rose, I.; Ferrari, M. C.; Esposito, E.; Fuoco, A.; Jansen, J. C.; McKeown, N. B. Redefining the Robeson Upper Bounds for CO2/CH4 and CO2/N2 Separations Using a Series of Ultra Permeable Benzotriptycene-Based Polymers of Intrinsic Microporosity. Energy Environ. Sci. 2019, 12(9), 2733–2740. DOI: 10.1039/c9ee01384a.
- Peydayesh, M.; Asarehpour, S.; Mohammadi, T.; Bakhtiari, O. Preparation and Characterization of SAPO-34 - Matrimid® Mixed Matrix Membranes for CO2/CH4 Separation. Chem. Eng. Res. Des. 2013, 91(7), 1335–1342. DOI: 10.1016/j.cherd.2013.01.022.
- Junaidi, M. U. M.; Leo, C. P.; Ahmad, A. L.; Ahmad, N. A. Fluorocarbon Functionalized SAPO-34 Zeolite Incorporated in Asymmetric Mixed Matrix Membranes for Carbon Dioxide Separation in Wet Gases. Microporous. Mesoporous. Mater. 2015, 206, 23–33. DOI: 10.1016/j.micromeso.2014.12.013.
- Junaidi, M. U. M.; Khoo, C. P.; Leo, C. P.; Ahmad, A. L. The Effects of Solvents on the Modification of SAPO-34 Zeolite Using 3-Aminopropyl Trimethoxy Silane for the Preparation of Asymmetric Polysulfone Mixed Matrix Membrane in the Application of CO2 Separation. Microporous. Mesoporous. Mater. 2014, 192, 52–59. DOI: 10.1016/j.micromeso.2013.10.006.
- Ahmad, N. A.; Noh, A. N. M.; Leo, C. P.; Ahmad, A. L. CO2 Removal Using Membrane Gas Absorption with PVDF Membrane Incorporated with POSS and SAPO-34 Zeolite. Chem. Eng. Res. Des. 2017, 118, 238–247. DOI: 10.1016/j.cherd.2016.12.019.
- Fakult, D. T.; Hussain, M. Mixed Matrix Membranes for Gas Separation (Mixed-Matrix-Membranen Zur Gastrennung) Als Dissertation genehmigt von der Technischen Fakultät der Universität Erlangen-Nürnberg; Universität Erlangen-Nürnberg: Erlangen, 2013.
- Nasir, R.; Ahmad, N. N. R.; Mukhtar, H.; Mohshim, D. F. Effect of Ionic Liquid Inclusion and Amino-Functionalized SAPO-34 on the Performance of Mixed Matrix Membranes for CO2/CH4 Separation. J. Environ. Chem. Eng. 2018, 6(2), 2363–2368. DOI: 10.1016/j.jece.2018.03.032.
- Ahmad, N. N. R.; Mukhtar, H.; Mohshim, D. F.; Nasir, R.; Man, Z. Effect of Different Organic Amino Cations on SAPO-34 for PES/SAPO-34 Mixed Matrix Membranes Toward CO2/CH4 Separation. J. Appl. Polym. Sci. 2016, 133(18), 1–6. DOI: 10.1002/app.43387.
- Li, S.; Carreon, M. A.; Zhang, Y.; Funke, H. H.; Noble, R. D.; Falconer, J. L. Scale-Up of SAPO-34 Membranes for CO2/CH4 Separation. J. Memb. Sci. 2010, 352(1–2), 7–13. DOI: 10.1016/j.memsci.2010.01.037.
- Miao, S.; Xiong, Z.; Zhang, J.; Wu, Y.; Gong, X. Polydopamine/SiO2 Hybrid Structured Superamphiphobic Fabrics with Good Photothermal Behavior. Langmuir. 2022, 38(30), 9431–9440. DOI: 10.1021/acs.langmuir.2c01629.
- Han, X.; Gong, X. In Situ, One-Pot Method to Prepare Robust Superamphiphobic Cotton Fabrics for High Buoyancy and Good Antifouling. ACS Appl. Mater. Interfaces. 2021, 13(26), 31298–31309. DOI: 10.1021/acsami.1c08844.
- Rezaei-DashtArzhandi, M.; Ismail, A. F.; Goh, P. S.; Wan Azelee, I.; Abbasgholipourghadim, M.; Rehman, G. U.; Matsuura, T. Zeolite ZSM5-Filled PVDF Hollow Fiber Mixed Matrix Membranes for Efficient Carbon Dioxide Removal via Membrane Contactor. Ind. Eng. Chem. Res. 2016, 55, 12632–12643. DOI: 10.1021/acs.iecr.6b03117.
- Rezaeia, M.; Ismai, A. F.; Hashemifard, S. A.; Bakeric, G.; Matsuura, T. Experimental Study on the Performance and Long-Term Stability of PVDF/Montmorillonite Hollow Fiber Mixed Matrix Membranes for CO2 Separation Process. Int. J. Greenhouse Gas Control. 2014, 26, 147–157. DOI: 10.1016/j.ijggc.2014.04.021.
- Kumar, P.; Kim, S.; Ida, J.; Guliants, V. V. Comments on the Origins of N2/CO2 Selectivity of Gas Separation Membranes. Ind. Eng. Chem. Res. 2009, 48, 3702. DOI: 10.1021/ie9001487.
- Hu, L.; Zhou, J.; Li, Y.; Cheng, J.; Cen, K.; Liu, J. CO2 Absorption and Diffusion in Ionic Liquid [P66614][Triz] Modified Molecular Sieves SBA-15 with Various Pore Lengths. Fuel Processing Technol. 2017, 172, 216–224. DOI: 10.1016/j.fuproc.2017.12.022.
- Asghari, M.; Dashti, A.; Rezakazemi, M.; Raji, M.; Sodeifian, G. Polyurethane-SAPO-34 Mixed Matrix Membrane for CO2/CH4 and CO2/N2 Separation. Chin. J. Chem. Eng. 2018, 27, 322–334. DOI: 10.1016/j.cjche.2018.03.012.
- Huang, Y.; Zong, Z.; Zhou, S. J.; Huang, Y.; Song, Z.; Feng, X.; Zhou, R.; Meyer, H. S.; Yu, M.; Carreon, M. A. SAPO-34 Membranes for N2/CH4 Separation: Preparation, Characterization, Separation Performance and Economic Evaluation. J. Memb. Sci. 2015, 487, 141–151. DOI: 10.1016/j.memsci.2015.03.078.
- Rabiee, H.; Ghadimi, A.; Mohammadi, T. Gas Transport Properties of Reverse-Selective poly(ether-b-amide6)/[Emim][BF4] Gel Membranes for CO2/light Gases Separation. J. Memb. Sci. 2015, 476, 286–302. DOI: 10.1016/j.memsci.2014.11.037.
- Ahmad, N. N. R.; Leo, C. P.; Mohammad, A. W.; Ahmad, A. L. Interfacial Sealing and Functionalization of Polysulfone/SAPO-34 Mixed Matrix Membrane Using Acetate-Based Ionic Liquid in Post-Impregnation for CO2 Capture. Sep. Purif. Technol. 2018, 197, 439–448. DOI: 10.1016/j.seppur.2017.12.054.
- Venna, S. R.; Carreon, M. A. Amino-Functionalized SAPO-34 Membranes for CO2/CH4 and CO2/N2 Separation. Langmuir. 2011, 27, 2888–2894. DOI: 10.1021/la105037n.
- Ahmad, N. N. R.; Mukhtar, H.; Man, Z.; Mohshim, D. F. Synthesis and Characterization of EDA-Functionalized Polyethersulfone/SAPO-34 Mixed Matrix Membrane. ARPN J. Eng. Appl. Sci. 2015, 10(15), 6192–6195. http://scholars.utp.edu.my/id/eprint/22064
- Cai, W.; Xie, J.; Luo, J.; Chen, X.; Wang, M.; Wang, Y.; Li, J. N-Octyltrichlorosilane Modified SAPO-34/PDMS Mixed Matrix Membranes for Propane/Nitrogen Mixture Separation. Separat. 2022, 9(3), 64. DOI: 10.3390/separations9030064.
- Mees, F. D. P.; Voort, P. V. D.; Cool, P.; Martens, L. R. M.; Janssen, M. J. G.; Verberckmoes, A. A.; Kennedy, G. J.; Hall, R. B.; Wang, K.; Vansant, E. F. Controlled Reduction of the Acid Site Density of SAPO-34 Molecular Sieve by Means of Silanation and Disilanation. J. Phys. Chem B. 2003, 107(14), 3161–3167. DOI: 10.1021/jp0276045.
- Ahmad, N. A.; Leo, C. P.; Junaidi, M. U. M.; Ahmad, A. L. PVDF/PBI Membrane Incorporated with SAPO-34 Zeolite for Membrane Gas Absorption. J. Taiwan Inst. Chem. Eng. 2016, 63, 143–150. DOI: 10.1016/j.jtice.2016.02.023.
- Zhao, X.; Liu, W.; Liu, X.; Zhang, B. Mixed Matrix Membranes Incorporated with Aminosilane-Functionalized SAPO-34 for Upgrading CO2/CH4 Separation Performances. Ind. Eng. Chem. Res. 2021, 60(38), 13927–13937. DOI: 10.1021/acs.iecr.1c01522.
- Mu, Y.; Chen, H.; Xiang, H.; Lan, L.; Shao, Y.; Fan, X.; Hardacre, C. Defects-Healing of SAPO-34 Membrane by Post-Synthesis Modification Using Organosilica for Selective CO2 Separation. J. Memb. Sci. 2019, 575, 80–88. DOI: 10.1016/j.memsci.2019.01.004.
- Ismail, A. F.; Kusworo, T. D.; Mustafa, A. Enhanced Gas Permeation Performance of Polyethersulfone Mixed Matrix Hollow Fiber Membranes Using Novel Dynasylan Ameo Silane Agent. J. Memb. Sci. 2008, 319(1–2), 306–312. DOI: 10.1016/j.memsci.2008.03.067.
- Rogers, R. D.; Seddon, K. R. Ionic Liquids - Solvents of the Future? Science. 2003, 302(5646), 792–793. DOI: 10.1126/science.1090313.
- Holbrey, J. D.; Seddon, K. R. Ionic Liquids. In Clean Products and Processes, Springer-Verlag, 1999; Vol. 1, pp. 223–236. http://www.ch.qub.ac.uk/staff/personal/krs
- Wikes, J. S.; Wassescheid, P.; Welton, T. Ionic Liquids in Synthesis; Weinheim: WILEY-VCH Verlags GmbH & Co, 2008; Vol. 2, pp. 1–2, 3-527-60070-1.
- Forsyth, S. A.; Pringle, J. M.; MacFarlane, D. R. Ionic Liquids - an Overview. Aust. J. Chem. 2004, 57(2), 113–119. DOI: 10.1071/CH03231.
- Wilkes, J. S.; Wasserscheid, P.; Welton, T. Ionic Liquids in Synthesis, 2nd. Ed; Wiley-VCH: Weinheim, 2007; Vol. 1, pp. 1–6. DOI: 10.1002/9783527621194.ch1.
- Ahmad, N. N. R.; Leo, C. P.; Mohammad, A. W.; Ahmad, A. L. Modification of Gas Selective SAPO Zeolites Using Imidazolium Ionic Liquid to Develop Polysulfone Mixed Matrix Membrane for CO2 Gas Separation. Microporous. Mesoporous. Mater. 2017, 244, 21–30. DOI: 10.1016/j.micromeso.2016.10.001.
- Cheng, J.; Li, Y.; Hu, L.; Zhou, J.; Cen, K. CO2 Adsorption Performance of Ionic Liquid [P66614][2-Op] Loaded Onto Molecular Sieve MCM-41 Compared to Pure Ionic Liquid in Biohythane/Pure CO2 Atmospheres. Energy Fuels. 2016, 30(4), 3251–3256. DOI: 10.1021/acs.energyfuels.5b02857.
- Mohshim, D. F.; Mukhtar, H.; Man, Z. Ionic Liquid Polymeric Membrane: Synthesis, Characterization & Performance Evaluation. Key Eng. Mater. 2014, 594–595, 18–23. DOI: 10.4028/0000www.scientific.net/KEM.594-595.18.
- Mohshim, D. F.; Mukhtar, H.; Man, Z. The Effect of Incorporating Ionic Liquid into Polyethersulfone-SAPO34 Based Mixed Matrix Membrane on CO2 Gas Separation Performance. Sep. Purif. Technol. 2014, 135, 252–258. DOI: 10.1016/j.seppur.2014.08.019.
- Ilyas, A.; Muhammad, N.; Gilani, M. A.; Vankelecom, I. F. J.; Khan, A. L. Effect of Zeolite Surface Modification with Ionic Liquid [Aptms][ac] on Gas Separation Performance of Mixed Matrix Membranes. Sep. Purif. Technol. 2018, 205, 176–183. DOI: 10.1016/j.seppur.2018.05.040.
- Wu, T.; Liu, Y.; Kumakiri, I.; Tanaka, K.; Chen, X.; Kita, H. Preparation and Permeation Properties of PeSu-Based Mixed Matrix Membranes with Nano-Sized CHA Zeolites. J. Chem. Eng. Japan. 2019, 52(6), 514–520. DOI: 10.1252/jcej.18we241.
- Cakal, U.; Yilmaz, L.; Kalipcilar, H. Effect of Feed Gas Composition on the Separation of CO2/CH4 Mixtures by PES-SAPO 34-HMA Mixed Matrix Membranes. J. Memb. Sci. 2012, 417–418, 45–5. DOI:10.1016/j.memsci.2012.06.011.
- Oral, E. E.; Yilmaz, L.; Kalipcilar, H. Effect of Gas Permeation Temperature and Annealing Procedure on the Performance of Binary and Ternary Mixed Matrix Membranes of Polyethersulfone, SAPO-34, and 2-Hydroxy 5-Methyl Aniline. J. Appl. Polym. Sci. 2014, 131(17), 8498–8505. DOI: 10.1002/app.40679.
- Carter, D.; Tezel, F. H.; Kruczek, B.; Kalipcilar, H. Investigation and Comparison of Mixed Matrix Membranes Composed of Polyimide Matrimid with ZIF – 8, Silicalite, and SAPO-34. J. Memb. Sci. 2017, 544, 35–46. DOI: 10.1016/j.memsci.2017.08.068.
- Junaidi, M. U. M.; Leo, C. P.; Ahmad, A. L.; Kamal, S. N. M.; Chew, T. L. Carbon Dioxide Separation Using Asymmetric Polysulfone Mixed Matrix Membranes Incorporated with SAPO-34 Zeolite. Fuel Process. Technol. 2014, 118, 125–132. DOI: 10.1016/j.fuproc.2013.08.009.
- Mohshim, D. F.; Mukhtar, H.; Dutta, B. K.; Man, Z. Predicting CO2 Permeation Through an Enhanced Ionic Liquid Mixed Matrix Membrane (IL3M). Int. J. Chem. Eng. 2019, 9525783, 10. DOI: 10.1155/2019/9525783.
- Santaniello, A.; Di Renzo, A.; Maio, F. D.; Belov, N. A.; Yampolskii, Y. P.; Golemme, G. Competing Non Ideal Behaviour of SAPO-34 and Poly(hexafluoropropylene) in Mixed Matrix Membranes. Microporous. Mesoporous. Mater. 2020, 303. DOI: 10.1016/j.micromeso.2020.110241.
- Liu, Y.; Takata, K.; Kita, H.; Tanaka, K. Investigation of Gas Diffusivity and Solubility of PES Based Mixed Matrix Membranes Using Commercial SAPO-34 Zeolite. Trans. Mat. Res. Soc. Jpn. 2021, 46(1), 39–43. DOI: 10.14723/tmrsj.46.39.
- Wu, T.; Shi, Y.; Liu, Y.; Kumakiri, I.; Tanaka, K.; Chen, X.; Kita, H. Improved Gas Permeation Properties of 6FDA-Trmpd Mixed-Matrix Membrane with SAPO-34 Crystals Toward CO2 Separation. Energy Fuels. 2021, 35(13), 10680–10688. DOI: 10.1021/acs.energyfuels.1c00925.
- Estahbanati, E. G.; Omidkhah, M.; Amooghin, A. E. Preparation and Characterization of Novel Ionic Liquid/Pebax Membranes for Efficient CO2/light Gases Separation. J. Ind. Eng. Chem. 2017, 51, 77–89. DOI: 10.1016/j.jiec.2017.02.017.
- Lu, S. C.; Khan, A. L.; Vankelecom, I. F. J. Polysulfone-Ionic Liquid-Based Membranes for CO2/N2 Separation with Tunable Porous Surface Features. J. Memb. Sci. 2016, 518, 10–20. DOI: 10.1016/j.memsci.2016.06.031.
- Huang, G.; Isfahani, A. P.; Muchtar, A.; Sakurai, K.; Shrestha, B. B.; Qin, D.; Yamaguchi, D.; Sivaniah, E.; Ghalei, B. Pebax/Ionic Liquid Modified Graphene Oxide Mixed Matrix Membranes for Enhanced CO2 Capture. J. Memb. Sci. 2018, 565, 370–379. DOI: 10.1016/j.memsci.2018.08.026.
- Bernardo, P.; Jansen, J. C.; Bazzarelli, F.; Tasselli, F.; Fuoco, A.; Friess, K.; Izák, P.; Jarmarová, V.; Kacírková, M.; Clarizia, G. Gas Transport Properties of Pebax®/room Temperature Ionic Liquid Gel Membranes. Sep. Purif. Technol. 2012, 97, 73–82. DOI: 10.1016/j.seppur.2012.02.041.
- Jansen, J. C.; Friess, K.; Clarizia, G.; Schauer, J.; Izák, P. High Ionic Liquid Content Polymeric Gel Membranes: Preparation and Performance. Macromolecul. 2011, 44(1), 39–45. DOI: 10.1021/ma102438k.
- Uchytil, P.; Schauer, J.; Petrychkovych, R.; Setnickova, K.; Suen, S. Y. Ionic Liquid Membranes for Carbon Dioxide-Methane Separation. J. Memb. Sci. 2011, 383(1–2), 262–271. DOI: 10.1016/j.memsci.2011.08.061.
- Mannan, H. A.; Mukhtar, H.; Shahrun, M. S.; Bustam, M. A.; Man, Z.; Bakar, M. Z. A. Effect of [EMIM][Tf2N] Ionic Liquid on Ionic Liquid-Polymeric Membrane (ILPM) for CO2/CH4 Separation. Procedia. Eng. 2016, 148, 25–29. DOI: 10.1016/j.proeng.2016.06.477.
- Hong, S.; Kim, D.; Jeong, Y.; Kim, E.; Jung, J. C.; Choi, N.; Nam, J.; Yip, A. C. K.; Choi, J. Healing of Microdefects in SSZ-13 Membranes via Filling with Dye Molecules and Its Effect on Dry and Wet CO2 Separations. Chem. Mater. 2018, 30, 3346–3358. DOI: 10.1021/acs.chemmater.8b00757.
- Mannan, H. A.; Nasir, R.; Mukhtar, H.; Mohshim, D. F.; Shaharun, M. S. Role of Ionic Liquids in Eliminating Interfacial Defects in Mixed Matrix Membranes. In Interfaces in Particle and Fibre Reinforced Composites: Current Perspectives on Polymer, Ceramic, Metal and Extracellular Matrices; Woodhead Publishing, 2020 Ed. Elsevier, 2019; pp. 269–309. DOI: 10.1016/B978-0-08-102665-6.00011-X.
- Dong, G.; Li, H.; Chen, V. Challenges and Opportunities for Mixed-Matrix Membranes for Gas Separation. J. Mater. Chem. A. 2013, 1, 4610. DOI: 10.1039/c3ta00927k.
- Shafie, S. N. A.; Nordin, N. A. H. M.; Bilad, M. R.; Misdan, N.; Sazali, N.; Putra, Z. A.; Wirzal, M. D. H.; Idris, A.; Jaafar, J.; Man, Z. [emim][Tf2N]-modified Silica as Filler in Mixed Matrix Membrane for Carbon Dioxide Separation. Membranes. 2021, 11(5), 371. DOI: 10.3390/membranes11050371.
- Jiang, B.; Zhang, N.; Wang, B.; Yang, N.; Huang, Z.; Yang, H.; Shu, Z. Deep Eutectic Solvent as Novel Additive for PES Membrane with Improved Performance. Sep. Purif. Technol. 2018, 194, 239–248. DOI: 10.1016/j.seppur.2017.11.036.
- Craveiro, R.; Neves, L. A.; Duarte, A. R. C.; Paiva, A. Supported Liquid Membranes Based on Deep Eutectic Solvents for Gas Separation Processes. Sep. Purif. Technol. 2021, 254. DOI: 10.1016/j.seppur.2020.117593.