ABSTRACT
This study assessed whether symptoms of muscle damage could be reduced by a combination of therapeutic ultrasound and electrical stimulations, and whether this could be enhanced by blood flow restriction. Before and 48 h after performing eccentric elbow flexion exercises, individuals completed assessments of muscle damage. A 10-min therapeutic ultrasound and electrical stimulation treatment was then applied with and without blood flow restriction to assess short (5 min) and long-term (24 h) improvements. Twenty-three individuals completed the study (11 females). Data were analysed using Bayesian repeated measures ANOVAs. The damaging exercise increased discomfort (BF10 = 2.93e14) and relaxed joint angle (BF10 = 2425.90) while decreasing pain pressure threshold (BF10 = 289.71). Each of these variables was acutely improved with the combination treatment protocol (all BF10 ≥ 74) with no added effect of blood flow restriction. A combination of therapeutic ultrasound and interferential electrical stimulations appeared effective at acutely alleviating symptoms of muscle damage with no additive effect of blood flow restriction.
Introduction
The application of blood flow restriction (BFR) involves placing pneumatic cuffs at the most proximal part of the arms or legs in attempt to reduce arterial inflow to the muscle while preventing venous return back to the heart. Applying BFR during aerobic exercise (Abe et al., Citation2006), low load resistance exercise (Laurentino et al., Citation2012), or even electrical stimulations (Natsume et al., Citation2015) has been shown to augment increases in both muscle size and strength. While most research examining BFR has focused on outcomes of muscle size and strength, recent research suggests that applying BFR during low load resistance exercise (Hughes & Patterson, Citation2020), or in the absence of exercise (Page et al., Citation2017), may induce a hypoalgesic (i.e. pain-inhibiting) effect. The exact mechanisms as to how BFR induces a hypoalgesic effect are unknown but are most commonly hypothesized to be related to the production of endogenous opioids which increase dopamine release in the brain and prevent pain transmission in the peripheral nervous system (Song et al., Citation2021). Furthermore, BFR has been shown to enhance the recovery of force following damaging exercise (Page et al., Citation2017) suggesting that BFR may be effective at alleviating symptoms of muscle damage when used alone or in conjunction with other treatment modalities.
Another modality that has been shown effective for alleviating symptoms of muscle damage involves the use of therapeutic ultrasound which has been shown to decrease pain and enhance the recovery of muscle strength following damaging eccentric exercises (Aaron et al., Citation2017; Hasson et al., Citation1990). The exact mechanisms whereby therapeutic ultrasound may be working to reduce symptoms associated with muscle damage are unknown but may be related to increased blood flow to the damaged tissue which assists in tissue repair and reduces the presence of noxious stimuli that activate pain receptors (Aaron et al., Citation2017). Other hypotheses have been centred on increases in vascular permeability resulting in reductions in tissue pressure (Hasson et al., Citation1990). While it is plausible that alleviating sensations of discomfort would serve to directly assist in the recovery of force production, this does not appear to be the case as treatment protocols enhancing recovery from muscle damage often demonstrate reduced discomfort without any augmentation in force production (Denegar and Perrin, Citation1992).
Electrical stimulations have also been used to treat symptoms of muscle damage. Electrical stimulations appear to be the most effective at alleviating sensations of discomfort as opposed to enhancing recovery of force (Babault et al., Citation2011). The mechanisms responsible for reducing discomfort are likely dependent upon the type of electrical stimulations used. Transcutaneous electrical nerve stimulation (TENS) is likely to reduce discomfort by increasing endogenous opioid release and/or blocking transmission of nociceptive fibres (Babault et al., Citation2011) similar to that previously mentioned for BFR. Neuromuscular electrical stimulation (NMES) is thought to assist with alleviating symptoms of muscle damage by increasing blood flow and clearing metabolites (Babault et al., Citation2011). Our laboratory recently experimented with electrical stimulations combined with BFR as a means of preventing (Cintron et al., Citation2022) or treating (Brown et al., Citation2022) symptoms of muscle damage. We observed no additive effect above electrical stimulations performed alone, hypothesizing that these modalities may be working through similar redundant mechanisms.
Studies have compared the effectiveness of electrical stimulations to that of therapeutic ultrasound, noting similar benefits present among individuals with chronic low back pain (Durmus et al., Citation2010). Others have examined the combined use of therapeutic ultrasound and electrical stimulations, demonstrating reduced discomfort and improved range of motion in people with fibromyalgia (Almeida et al., Citation2003). As the combined use of therapeutic ultrasound and neuromuscular electrical stimulations may be working through different mechanisms to alleviate symptoms of muscle damage compared to that of BFR, it would seem plausible that these treatment modalities could have additive effects. It remains unknown; however, whether the effects of BFR and combined ultrasound and electrical stimulations are additive. Thus, the purpose of the present study was to determine if the effectiveness of therapeutic ultrasound and electrical stimulations could be further enhanced if performed under BFR.
Materials & methods
Participants
To be eligible for the study, individuals needed to be between the ages of 18 and 64 years of age, they could not have an implanted electrical device, and they could not be classified as hypertensive. Additionally, individuals could not have more than one of the following risk factors for thromboembolism (Motykie et al., Citation2000): 1) body mass index (BMI) ≥30; 2) diagnosed with Crohn’s or Inflammatory Bowel Disease; 3) past fracture of the hip, pelvis, or femur; 4) major surgery within the last 6 months; 5) diagnosed with varicose veins; 6) family history of deep vein thrombosis; 7) family history of pulmonary embolism; 8) using oral contraceptives; 9) using tobacco. Participants that were enrolled in the study were also instructed to avoid doing any upper-body exercise for the remainder of the study and to avoid performing any outside treatments such as stretching or using non-steroidal anti-inflammatory drugs intended to alleviate discomfort in their arms.
Study overview
Individuals came to the laboratory for a total of three visits (). On the first visit, individuals had their discomfort, relaxed joint angle, pain pressure threshold, muscle thickness, and isometric strength measured on both arms. Changes in each of these variables were used as indirect indicators of muscle damage. Individuals were then seated on an isokinetic dynamometer (Biodex Systems 4; Shirley, NY; USA) where they completed a damaging bout of eccentric exercises on both arms to conclude visit 1. Individuals returned to the laboratory 48 h later to complete their second visit which began with assessing relaxed joint angle, discomfort, pain pressure threshold, muscle thickness, and isometric strength to determine the effectiveness of the damaging exercise bout. Individuals then completed the treatment protocol on each of their arms simultaneously, with the only difference being that one arm had BFR applied during the treatment. Five minutes after the treatment was completed, individuals again had their discomfort, relaxed joint angle, pain pressure threshold, muscle thickness, and isometric strength assessed to determine the acute effects of the treatment protocols. This concluded visit 2. Visit 3 took place 24 h after visit 2 and consisted of measuring discomfort, relaxed joint angle, pain pressure threshold, muscle thickness, and isometric strength to determine the chronic effects of the treatment protocols.
Discomfort
The Borg CR10+ scale was used to assess ratings of discomfort (Borg, Citation1990). All individuals were given the same instructions that the scale ranges from 0 indicating no discomfort up to 10 which indicates the worst discomfort the individual ever experienced before. Individuals were allowed to choose decimals and could also choose a number exceeding 10 if they were experiencing more discomfort than they ever felt before. The discomfort rating was asked to the individual after having them flex and extend their elbow multiple times through the full range of motion.
Relaxed joint angle
Relaxed joint angle at the elbow was measured with the individual standing with their arms in a relaxed position. A goniometer was used with the axis placed on the lateral epicondyle of the humerus. The stationary arm was pointed towards the acromion process, and the mobile arm was pointed towards the styloid process of the ulna. The relaxed joint angle was recorded as the degrees of elbow flexion by subtracting the recorded angle from 180°. Therefore, 0° indicated complete elbow extension, and muscle damage would be expected to increase the relaxed joint angle resulting in a greater amount of elbow flexion at rest.
Muscle thickness
The individual’s upper arm was measured as the distance between the acromion process and the lateral epicondyle of the humerus. Two images were taken at 60% of this distance (more distally) using an ultrasound machine (Logiq P9, General Electric, Fairfield, CT, USA) with the probe placed over the muscle belly of the anterior upper arm. If the two images differed by more than 0.1 cm, subsequent images were taken until obtaining consistent results. The average of the two measurements were then used for analysis. The muscle thickness was recorded as the distance between the muscle-fat and muscle-bone interfaces. Changes in muscle thickness were used as an indicator of muscle swelling which is an indirect marker of muscle damage.
Pain pressure threshold
A digital force transducer (Mxmoonfree ZMF-50N; Zhengzhou, China) was pressed against the elbow flexor muscles using the same location used for the muscle thickness measure. The pressure was applied at a standardized rate of 0.32 kg/cm2/sec which was done using a metronome. Individuals were instructed to indicate the first point at which the pressure being applied was perceived as painful. This measurement was used as an indicator of pain sensitivity.
Isometric strength
Individuals were seated on an isokinetic dynamometer (Biodex Systems 4; Shirley, NY; USA) with the lever arm locked into 60° of elbow flexion. Individuals were asked to pull towards them as hard as possible using the force of their elbow flexors. Two attempts were given each separated by 60 s of rest. Each of the attempts lasted approximately 3–5 s and was terminated once there was a plateau or drop in isometric torque. Individuals were given verbal encouragements and visual feedback of their torque produced throughout the test. The maximal amount of torque that was generated across the two attempts was recorded as the individual’s peak torque for analysis.
Damaging exercise
Individuals were seated on an isokinetic dynamometer (Biodex Systems 4; Shirley, NY; USA) where they performed six sets of maximal eccentric elbow flexion exercises. Each of these sets consisted of 10 repetitions that were performed isokinetically at a speed of 90°/sec. This protocol of 60 maximal repetitions was chosen at it has been previously shown to successfully induce muscle damage (McKinnon et al., Citation2012). Individuals were asked to pull towards them as hard as possible while attempting to prevent the lever arm from lowering to full elbow extension. The lever arm was then automatically returned to 90° of elbow flexion before beginning the next repetition. Each of the sets was separated by 30 s of rest, and strong verbal encouragement was given throughout.
Combination protocol
The combination treatment protocol was administered to both arms using a tabletop device (Intellect Legend XT, Dallas, TX, USA). The individual was asked to point to the location where they felt the most discomfort in their elbow flexors, and a circle with a 12 cm diameter was drawn around this area. Three circular electrodes (3.2 cm diameter) were placed around the affected area, and pulsed ultrasound was administered (1 MHz; 0.8W/cm2) with a 50% duty cycle. The interferential current was administered with a carrier frequency of 4 kHz and an amplitude-modulated frequency of 100 Hz. The current amplitude was increased until a visible twitch was present. The treatment protocol was administered continuously for a 10-min period as this duration has previously shown to be effective at reducing sensations of pain (Çıtak-Karakaya et al., Citation2006).
Blood flow restriction protocol
A 5-cm-wide nylon cuff (Hokanson inc.; Bellevue, WA; USA) was placed at the most proximal portion of the individual’s arm that was assigned to complete the combination protocol under BFR. A Doppler probe (Arjo Inc.; Addison, IL; USA) was then placed at the distal radial artery until an auditory pulse was present. A rapid cuff inflator (Hokanson Inc.; Bellevue, WA; USA) was then used to inflate the pressure cuff in 1 mmHg increments until a pulse was no longer present. The lowest pressure at which a pulse was not present was recorded as the individual’s arterial occlusion pressure. The pressure that was applied during the treatment protocol was 80% of the individual’s arterial occlusion pressure. The pressure cuff was inflated immediately before the combination protocol on the appropriate arm and was deflated after the treatment was finished. The opposite arm that was assigned to complete the combination therapy without BFR had a restrictive cuff placed on the top of the arm but it remained uninflated throughout the protocol. The arm assigned to complete the BFR protocol was counterbalanced for limb dominance to ensure an equal number of participants received BFR on the dominant and non-dominant limbs.
Statistics
All statistics were computed using JASP version 0.16 (JASP Team; Netherlands). A 2 (within factor of condition) × 4 (within factor of time) Bayesian repeated measures ANOVA was used to assess changes in discomfort, relaxed joint angle, pain pressure threshold, muscle thickness, and isometric strength. All Bayesian statistics were computed using uninformed priors, and the interaction and main effects were compared using Bayes Factors (BF10). A BF10 of >3 or >10 was used as moderate and strong support for the alternate hypothesis, respectively. A BF10 of <0.33 or <0.10 was used as moderate and strong support for the null, respectively (van Doorn et al., Citation2021). Post-hoc comparisons were adjusted using Holm’s procedure (Holm, Citation1979).
Results
Descriptive statistics
A total of 23 individuals completed the study (12 males, 11 females). One individual dropped out of the study due to discomfort specifically during the treatment completed with BFR. Means and standard deviations were as follows: age: 21 (2) years, height 169.2 (12.1) cm, and weight: 70.6 (14.9) kg.
Discomfort
There was no interaction (BF10 = 0.06) or main effect of condition (BF10 = 0.26), but there was a main effect of time (BF10 = 8.30e13). Discomfort increased as a result of the damaging exercise (BF10 = 2.93e14) but decreased in response to the treatments (BF10 = 50846.64) and further decreased 24 h post-treatment (BF10 = 19300.58; ).
Figure 2. Discomfort.
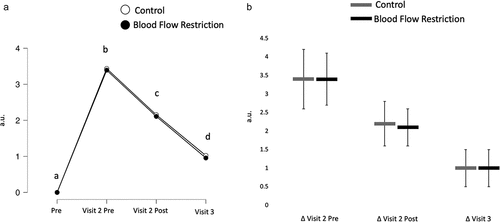
Relaxed joint angle
There was no interaction (BF10 = 0.06) nor was there a main effect of condition (BF10 = 0.25). There was a main effect of time (BF10 = 483.92) with relaxed joint angle increasing as a result of the damaging exercise (BF10 = 2425.90) and decreasing in response to the treatment protocols (BF10 = 74.57). Resting joint angle was still above baseline at the 72 h post measure when compared to before the damaging exercise (BF10 = 3.65; ).
Figure 3. Relaxed joint angle.
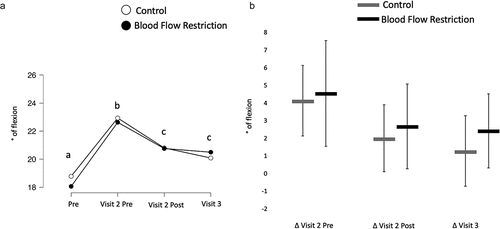
Muscle thickness
There was an interaction for muscle thickness (BF10 = 8.98) with greater increases in muscle thickness present in the BFR condition at both the post-treatment (BF10 = 12.43) and 24 h post-treatment time points (BF10 = 2.79e11; ).
Figure 4. Muscle thickness.
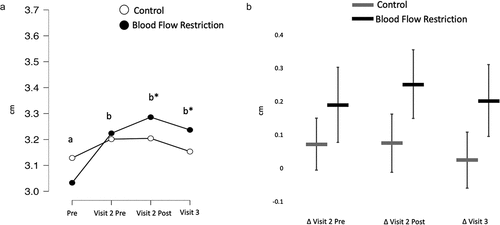
Pain pressure threshold
There was no interaction (BF10 = 0.55) nor was there a main effect of condition (BF10 = 0.22), but there was a main effect of time (BF10 = 29.65). Pain pressure threshold decreased as a result of the damaging exercise (BF10 = 289.71) but increased back to baseline after the treatment was administered (BF10 = 167.21) and remained unchanged at the 24 h post-treatment time point (BF10 = 0.16; ).
Figure 5. Pain-pressure threshold.
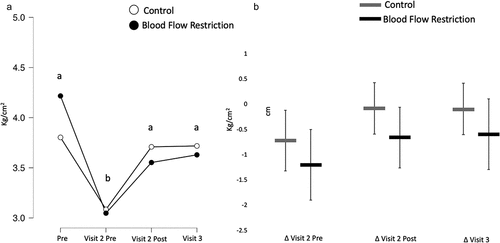
Isometric strength
There was no interaction (BF10 = 0.27) nor was there a main effect of condition (BF10 = 0.26). There was a main effect of time (BF10 = 27006.80) with isometric strength decreasing as a result of the damaging exercise (BF10 = 2262.99). It was inconclusive what effect the acute treatment had on isometric strength recovery (BF10 = 1.11), but isometric strength was increased with another 24 h of recovery (BF10 = 112.55; ).
Figure 6. Isometric strength.
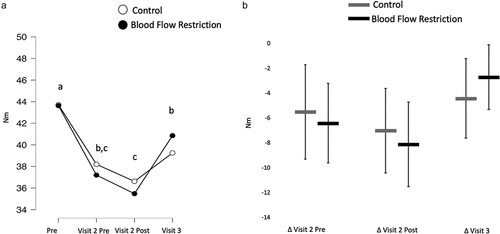
Discussion
The primary finding of the present study was that, while the combination of ultrasound and electrical stimulation therapy was effective at acutely alleviating symptoms of muscle damage, the effectiveness was not enhanced when performed under BFR. It was previously shown that applying BFR in the absence of exercise was able to attenuate muscle damage when administered immediately after the bout of damaging exercise (Page et al., Citation2017). A similar study assessed the efficacy of BFR in conjunction with unloaded elbow flexion exercises and found no preventative effect of BFR on attenuating symptoms of muscle damage (Wong et al., Citation2020). The authors also noted that the use of BFR was unable to assist in the treatment of muscle damage when administered 24 h after the damaging exercise bout (Wong et al., Citation2020). The findings of the present study are also in line with previous studies from our laboratory suggesting that the use of BFR could not assist with the prevention (Cintron et al., Citation2022) or treatment (Brown et al., Citation2022) of muscle damage when combined with electrical stimulations. Our results extend these findings and suggest that BFR also appears to be ineffective at enhancing the acute effectiveness of therapeutic ultrasound and electrical stimulations at mitigating symptoms of muscle damage. The lack of effectiveness could be due to BFR working through mechanisms that are redundant with electrical stimulations and therapeutic ultrasound (e.g. increased blood flow post-treatment).
While BFR did not have any additive effect, the use of combined ultrasound and electrical stimulations did appear to acutely mitigate symptoms of muscle damage. The effectiveness of the therapeutic ultrasound and electrical stimulations has been previously shown to be beneficial for individuals experiencing chronic pain conditions (Almeida et al., Citation2003; Durmus et al., Citation2010), and these results demonstrate that it may also be effective at alleviating discomfort associated with exercise. Previous studies have supported the effectiveness of electrical stimulations to reduce discomfort associated with muscle damage (Babault et al., Citation2011), but the effectiveness of therapeutic ultrasound has been questioned (Holmes & Rudland, Citation1991). In the present study, it is unclear whether therapeutic ultrasound had any additive effect when administered in conjunction with electrical stimulations. Pulsed ultrasound has been suggested to work via increasing membrane permeability, diffusion rates, and protein synthesis to assist with reduced inflammation (Xu et al., Citation2021). In the present study, there was no reduction in muscle swelling when measured 5-min post-treatment or 24 h post-treatment. It is possible that the muscle contraction occurring via electrical stimulations may have resulted in a fluid shift into the muscle and this may have countered any effectiveness of the therapeutic ultrasound at reducing muscle thickness.
The only difference between the BFR and control conditions was that BFR resulted in an acute increase in muscle thickness that was also present 24 h later. Anecdotally, several individuals reported discomfort, numbness, and cramping sensations in their arm when completing the BFR treatment condition. These sensations of discomfort, combined with the one aforementioned individual who dropped out from the study due to discomfort associated with BFR, may deter individuals from completing this treatment. It is currently unclear whether the use of BFR enhances muscle damage when combined with low load resistance exercise (de Queiros et al., Citation2021; Loenneke et al., Citation2014; Wernbom et al., Citation2020), but the present protocol consisted of a longer duration of BFR than what is commonly employed in conjunction with resistance exercise (10-min vs. ~4-min). While, it is possible that BFR may have enhanced (as opposed to the goal of alleviating) muscle damage, this was not evident in any other indirect markers of muscle damage. Furthermore, it is possible that the prolonged muscle swelling could be beneficial as it has been a proposed mechanism as to how BFR enhances muscle hypertrophy (Fry et al., Citation2010).
This study is not without limitations. First, it is possible that the BFR protocol may have induced a systemic effect whereby reducing discomfort on the opposite limb. We elected to use a within-subject design to account for differences in the magnitude of discomfort that would be present from the damaging exercise, and differences in pain sensitivity across individuals. Furthermore, the present study does not allow us to assess if there were any chronic effects of the combination therapy as we did not have a true control group capturing the natural recovery process of the damaging exercise without any treatments performed. While a previous study would suggest little improvement in soreness, range of motion, or torque production spanning 48 h to 72 h following damaging exercise (Nosaka & Sakamoto, Citation2001), we still cannot be confident the combination treatment was effective. We do feel that we have captured the acute effectiveness as natural recovery would not be expected to occur appreciably in the 15-min period spanning the pre and post-treatment measures. While we would have liked to include a true control group, the primary focus on the present study was to determine whether BFR could enhance the effectiveness of therapeutic ultrasound and electrical stimulations.
Conclusion
The combined use of electrical stimulations and therapeutic ultrasound was effective at acutely reducing discomfort and pain sensitivity associated with damaging eccentric exercises. The effectiveness of this treatment was not enhanced when performed in combination with BFR. The results of our study may not generalize to all BFR, electrical stimulation, and therapeutic ultrasound studies as the specific parameters chosen for each may impact the outcomes.
Disclosure statement
No potential conflict of interest was reported by the author(s).
Data availability statement
Data are available upon request of the corresponding author.
Additional information
Funding
References
- Aaron, S. E., Delgado-Diaz, D. C., & Kostek, M. C. (2017). Continuous ultrasound decreases pain perception and increases pain threshold in damaged skeletal muscle. Clinical Journal of Sport Medicine: Official Journal of the Canadian Academy of Sport Medicine, 27(3), 271–277. https://doi.org/10.1097/jsm.0000000000000343
- Abe, T., Kearns, C. F., & Sato, Y. (2006). Muscle size and strength are increased following walk training with restricted venous blood flow from the leg muscle, kaatsu-walk training. Journal of Applied Physiology, 100(5), 1460–1466. (1985). https://doi.org/10.1152/japplphysiol.01267.2005
- Almeida, T. F., Roizenblatt, S., Benedito-Silva, A. A., & Tufik, S. (2003). The effect of combined therapy (ultrasound and interferential current) on pain and sleep in fibromyalgia. Pain, 104(3), 665–672. https://doi.org/10.1016/s0304-3959(03)00139-8
- Babault, N., Cometti, C., Maffiuletti, N. A., & Deley, G. (2011). Does electrical stimulation enhance post-exercise performance recovery? European Journal of Applied Physiology, 111(10), 2501–2507. https://doi.org/10.1007/s00421-011-2117-7
- Borg, G. (1990). Psychophysical scaling with applications in physical work and the perception of exertion. Scandinavian Journal of Work, Environment & Health, 16(Suppl 1), 55–58. https://doi.org/10.5271/sjweh.1815
- Brown, L. J., Gasser, A. N., Sterner, R. L., & Dankel, S. J. (2022). The impact of blood flow restricted electrical stimulations on recovery from muscle damage. Clinical Physiology and Functional Imaging, 43(2), 103–108. https://doi.org/10.1111/cpf.12797
- Cintron, H. E., Heyburn, J. J., Sterner, R. L., & Dankel, S. J. (2022). Blood flow restricted electrical stimulations to prevent or attenuate symptoms of muscle damage. Research in Sports Medicine, 32(1), 1–12. https://doi.org/10.1080/15438627.2022.2132862
- Çıtak-Karakaya, İ., Akbayrak, T., Demirtürk, F., Ekici, G., & Bakar, Y. (2006). Short and long-term results of connective tissue manipulation and combined ultrasound therapy in patients with fibromyalgia. Journal of Manipulative and Physiological Therapeutics, 29(7), 524–528. https://doi.org/10.1016/j.jmpt.2006.06.019
- de Queiros, V. S., Dos Santos, Í. K., Almeida-Neto, P. F., Dantas, M., de França, I. M., Vieira, W. H. B., Neto, G. R., Dantas, P. M. S., & Cabral, B. (2021). Effect of resistance training with blood flow restriction on muscle damage markers in adults: A systematic review. Public Library of Science ONE, 16(6), e0253521. https://doi.org/10.1371/journal.pone.0253521
- Denegar, C. R., & Perrin, D. H. (1992). Effect of transcutaneous electrical nerve stimulation, cold, and a combination treatment on pain, decreased range of motion, and strength loss associated with delayed onset muscle soreness. Journal of Athletic Training, 27(3), 200–206. https://pubmed.ncbi.nlm.nih.gov/16558162
- Durmus, D., Durmaz, Y., & Canturk, F. (2010). Effects of therapeutic ultrasound and electrical stimulation program on pain, trunk muscle strength, disability, walking performance, quality of life, and depression in patients with low back pain: A randomized-controlled trial. Rheumatology International, 30(7), 901–910. https://doi.org/10.1007/s00296-009-1072-7
- Fry, C. S., Glynn, E. L., Drummond, M. J., Timmerman, K. L., Fujita, S., Abe, T., Dhanani, S., Volpi, E., & Rasmussen, B. B. (2010). Blood flow restriction exercise stimulates mTORC1 signaling and muscle protein synthesis in older men. Journal of Applied Physiology, 108(5), 1199–1209. (1985). https://doi.org/10.1152/japplphysiol.01266.2009
- Hasson, S., Mundorf, R., Barnes, W., Williams, J., & Fujii, M. (1990). Effect of pulsed ultrasound versus placebo on muscle soreness perception and muscular performance. Scandinavian Journal of Rehabilitation Medicine, 22(4), 199–205. https://doi.org/10.2340/165019779022199205
- Holm, S. (1979). A simple sequentially rejective multiple test procedure. Scandinavian Journal of Statistics, 6(2), 65–70. http://www.jstor.org/stable/4615733
- Holmes, M. A. M., & Rudland, J. R. (1991). Clinical trials of ultrasound treatment in soft tissue injury: A review and critique. Physiotherapy Theory and Practice, 7(3), 163–175. https://doi.org/10.3109/09593989109106968
- Hughes, L., & Patterson, S. D. (2020). The effect of blood flow restriction exercise on exercise-induced hypoalgesia and endogenous opioid and endocannabinoid mechanisms of pain modulation. Journal of Applied Physiology, 128(4), 914–924. (1985). https://doi.org/10.1152/japplphysiol.00768.2019
- Laurentino, G. C., Ugrinowitsch, C., Roschel, H., Aoki, M. S., Soares, A. G., Neves, M., Jr., Aihara, A. Y., Fernandes Ada, R., & Tricoli, V. (2012). Strength training with blood flow restriction diminishes myostatin gene expression. Medicine and Science in Sports and Exercise, 44(3), 406–412. https://doi.org/10.1249/MSS.0b013e318233b4bc
- Loenneke, J. P., Thiebaud, R. S., & Abe, T. (2014). Does blood flow restriction result in skeletal muscle damage? A critical review of available evidence. Scandinavian Journal of Medicine & Science in Sports, 24(6), e415–422. https://doi.org/10.1111/sms.12210
- McKinnon, N. B., Graham, M. T., & Tiidus, P. M. (2012). Effect of creatine supplementation on muscle damage and repair following eccentrically-induced damage to the elbow flexor muscles. Journal of Sports Science and Medicine, 11(4), 653–659.
- Motykie, G. D., Zebala, L. P., Caprini, J. A., Lee, C. E., Arcelus, J. I., Reyna, J. J., & Cohen, E. B. (2000). A guide to venous thromboembolism risk factor assessment. Journal of Thrombosis and Thrombolysis, 9(3), 253–262. https://doi.org/10.1023/a:1018770712660
- Natsume, T., Ozaki, H., Saito, A. I., Abe, T., & Naito, H. (2015). Effects of electrostimulation with blood flow restriction on muscle size and strength. Medicine and Science in Sports and Exercise, 47(12), 2621–2627. https://doi.org/10.1249/MSS.0000000000000722
- Nosaka, K., & Sakamoto, K. (2001). Effect of elbow joint angle on the magnitude of muscle damage to the elbow flexors. Medicine and Science in Sports and Exercise, 33(1), 22–29. https://doi.org/10.1097/00005768-200101000-00005
- Page, W., Swan, R., & Patterson, S. D. (2017). The effect of intermittent lower limb occlusion on recovery following exercise-induced muscle damage: A randomized controlled trial. Journal of Science & Medicine in Sport / Sports Medicine Australia, 20(8), 729–733. https://doi.org/10.1016/j.jsams.2016.11.015
- Song, J. S., Spitz, R. W., Yamada, Y., Bell, Z. W., Wong, V., Abe, T., & Loenneke, J. P. (2021). Exercise-induced hypoalgesia and pain reduction following blood flow restriction: A brief review. Physical Therapy in Sport, 50, 89–96. https://doi.org/10.1016/j.ptsp.2021.04.005
- van Doorn, J., van den Bergh, D., Böhm, U., Dablander, F., Derks, K., Draws, T., Etz, A., Evans, N. J., Gronau, Q. F., Haaf, J. M., Hinne, M., Kucharský, Š., Ly, A., Marsman, M., Matzke, D., Gupta, A., Sarafoglou, A., Stefan, A., Voelkel, J. G., & Wagenmakers, E. J. (2021). The JASP guidelines for conducting and reporting a bayesian analysis. Psychonomic Bulletin & Review, 28(3), 813–826. https://doi.org/10.3758/s13423-020-01798-5
- Wernbom, M., Schoenfeld, B. J., Paulsen, G., Bjørnsen, T., Cumming, K. T., Aagaard, P., Clark, B. C., & Raastad, T. (2020). Commentary: Can blood flow restricted exercise cause muscle damage? Commentary on blood flow restriction exercise: Considerations of methodology, application, and safety. Frontiers in Physiology, 11, 243. https://doi.org/10.3389/fphys.2020.00243
- Wong, V., Dankel, S. J., Spitz, R. W., Bell, Z. W., Viana, R. B., Chatakondi, R. N., Abe, T., & Loenneke, J. P. (2020). The effect of blood flow restriction therapy on recovery after experimentally induced muscle weakness and pain. Journal of Strength & Conditioning Research, 36(4), 1147–1152. https://doi.org/10.1519/jsc.0000000000003692
- Xu, M., Wang, L., Wu, S., Dong, Y., Chen, X., Wang, S., Li, X., & Zou, C. (2021). Review on experimental study and clinical application of low-intensity pulsed ultrasound in inflammation. Quantitative Imaging in Medicine and Surgery, 11(1), 443–462. https://doi.org/10.21037/qims-20-680