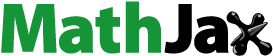
ABSTRACT
This research focused on the different approaches to the transport and internal chelation of metals with amino acids and organic acids in plants. Therefore, in the first phase, the plants studied were identified the characteristics of the bioaccumulation factors. Steria pumila, Echium angustifolium, Typha angustifolia, Sisymbrium austriacum were identified as hyperaccumulators (Cd, Ni), accumulators (Pb, Sn, and Se), excluders (Cr, Hg). On the other hand, the Sisymbrium austriacum only showed the characteristic of the accumulator for Cr. In the second phase, the combined effects of amino acids and organic acids on the chelation of heavy metals in plants were tested by a multi-linear regression model. Related to our hypothesis, Amino acids; Gly and Leu (Cd), Trp and Ile (Pb), Asp, Ser, and Leu (Cr), Ser (Hg), Trp and Glu (Ni), Asp, Thr, and Gly (Sn), Asn and Leu (Se), Organic acids; Malonic and Malic acid (Cd), Malonic acid (Pb), Oxalic and Malic acid (Cr), Oxalic, Succinic, Citric and Butyric acid (Hg), Malonic and Malic acid (Ni), Malonic, Maleic, and Malic acid (Sn), Malonic and Citric acid (Se) were concluded that had combined effect for heavy metal’s phytochelation ability into plants.
Introduction
All plant species that are tolerant or sensitive to the stress of heavy metals have a basic defensive system that acts against the stress of heavy metals. Advances in biological studies and new approaches have begun to understand some of the complex strategies plants use at the cellular and molecular levels to fight against metal stress. The multifunctionality and versatile molecular properties of phytochelates (PCs) and metallothioneins (MTs) are gaining increasing attention because of the detoxification of heavy metals and the maintenance of cellular ionic equilibrium. PCs and MTs have the potential to interact directly or indirectly with the plant’s antioxidant defense system. It also contribute to the transport and distribution of surplus ionized metals between roots and shoots, depending on time or tissueCitation1
Metal hyperaccumulation is a physiological process through which plants store large amounts of metal ions in aboveground tissues without being exposed to toxic effects. For this, the metal ions should be moved from the soil, absorbed by the roots, transmitted to the xylem for delivering them to the aboveground tissues.Citation2 Plant resistance against heavy metals (HM) can be categorized in two: plants either excluder or show toleration for high internal metal concentration. The former is achieved through extracellular precipitation, decreased metal concentration intake through biosorption, or enriched flow. Plants achieve HM tolerance through intracellular chelation of HMs by synthesis of organic acids (OA), amino acids (AA), heavy metal-binding ligands, or glutathione. Antioxidant protection systems and glyoxalase neutralize the harmful effects of Reactive oxygen species (ROS). When methylglyoxal is upregulated,Citation3 the metabolic products get concentrated in plant vacuoles.Citation4 Various root fluid substances, such as OAs and AAs, act as HM ligands to form stable HM complexes in the rhizosphere.Citation5 Considering the mechanisms behind OA-assisted metal chelation, hyperaccumulator plants stabilize HMs in leaf vacuoles using vacuole transporters, while HMs photostabilizing plants retain HMs mostly in root vacuoles.Citation6 Some metal-excluded plants have the ability to grow in heavy metal-contaminated soils and retain HMs in low concentrations above ground through phytostabilizationCitation7 Plants develop various strategies to exclude metals from their tissues through the function of their cell walls, plasma membranes, and mycorrhizae. These plants can protect metabolic sites such as shoots through mechanisms that restrict the movement of toxic metals outside of the rhizosphere or roots.Citation8 OAs in cells inhibit HMs from remaining as free ions in the cytoplasm by complicating them and reducing their bioavailability to plants.Citation9 Stress due to HMs results in the accumulation of certain types of AAs. Limiting their flow to plants by extrinsic binding of HMs to low-weighted molecular compounds excreted by roots comprises the first line of defense against HMs.Citation10 Through this mechanism, strong bonds are formed by chelating metals with the carboxylate groups of citrates, malate, malonate, aconitate, oxalate, or tartrates, which act as electron donors. The roots of the plants also contain hydrated natural pectin polysaccharides, which are cultures that bond toxic metal ions.Citation11
Due to their ability to bind metals, AAs and their by-products can be used to respond to metal toxicity. AAs facilitate chelation of HM ions in cells and xylem juice, thereby resulting in detoxification of HMsCitation12 and increased resistance of plants to toxic metal ion levelsCitation13 However, there is still no clear relationship between the accumulation of metals and the production of these compounds.
Proline is a potential Osmo preservative that has been studied particularly in salinity- and drought-stressed crops. Its role in ROS sweeping and metal chelation in plants exposed to metals has been studied.Citation14 Phytochelatin synthase (PCS) has been reported recently in plants exposed to high metal concentrations. High proline concentration plays a role in regulating the expression of melatonin-2, glutathione reductase, and glutathione synthetase genes.Citation15 Although they are not directly related to the chelation of metals in plants, they regulate the water in proteins. By balancing the protein’s natural state, Osmo protectors help to preserve its structural properties and integrity.Citation16 Generally, the proline concentration is the highest among the AA groups when plants are exposed to HMs.Citation17 However, the concentration changes depending on the metals absorbed by the plants.Citation18 The binding of metals to the constitutively expressed PCS enzyme is facilitated by Proline, as it increases the level of endogenous glutathione (GSH; γ-glutamyl-cysteinyl-glycine) and enables GSH to catalyze the conversion of reduced glutathione to PCS.Citation19 Enzymatic protection is possible via proline-assisted reduction of the activity of free metal ions following the formation of a metal (Zn/Cd) pro-complex.Citation20
This research was planned in two phases. The First phase: The heavy metal contents of four different types of plants collected on farmland and areas polluted by industrial waste were analyzed according to their heavy metal content. From the results of this analysis, four plant species were determined as hyperacumluter, accumulator, and excluder for various heavy metals. The second phase, examined the common effect of amino acids and organic acids on chelation/detoxification of heavy metals in plants with high tolerance to heavy metals.
Material and methods
Study area and preparation for analysis
This study was conducted in the Karabük province of Turkey. Four different species samples of plants Steria pumila (SP), Echium angustifolium (EA), Typha angustifolia (TA), Sisymbrium Austriacum (SA), and soil samples were collected from a steel industry dump site (PA) representing an anthropogenic polluted area (N 41° 10’ 42,60146’ and E 32° 38ʹ42,07808’). As a reference, a few samples (NPA) were collected from the farming areas (N 41° 12’ 45,47’ and E 32° 40’ 38,09”). Each plants species collected from PA and NPA were mixed with each among them according to the area harvested. The plants were washed to remove any surface contaminants and sheared into three parts to separate the root, stem, and leaf. Then, the plant and soil samples were allowed to dry at 24°C for one day. It was determined heavy metals in plant samples, the procedures was followed, about 0.5 g plant samples (in three replicate) were supplemented with 10 ml nitric + perchloric acid mixture. Resultant samples were then wet-digested until having 1 ml sample. Following the wet digestion procedure, resultant solutions were diluted with distilled water and readings were performed in an ICP OES (Inductively Couple Plasma spectrophotometer) (Perkin-Elmer, Optima 4300 DV, ICP/OES, Shelton, CT 06484–4794, USA) for heavy metal contents.Citation21
Determination of amino acids
The determination of AAs is performed using column separation with phenyl isothiocyanate (PITC).Citation22 Standard solutions were prepared. Samples of 10 µl were dissolved in a buffer solution of 100 µl and dried under high pressure for an hour. This was again dissolved in 100 μl of buffer solution by the addition of 5 μl of PITC. The PITC derivatives formed at room temperature 5 min after the reaction timeCitation23 were dissolved for a second time at high pressure. The PITC derivatives of AA formed in 0.05 M sodium acetate at pH 6.8. After dissolving it in a 9:1 (v/v) mixture of 0.1 M sodium acetate and 10% methanol in 40% acetonitrile,Citation24 10–20 µl was analyzed by HPLC.
Determination of organic acids
For analytical measurement of OAs, 15.6 µML oxalic acid, 66.6 µML tartaric acid, 74.6 µML malic acid, 339 µM succinic acid, 96 µM malonic acid, 5.7 µML ascorbic acid, 1.7 µML maleic OAs containing a mixture of acid, 95.1 µML citric acid, and 1.7 µML fumaric acid were used.Citation24 The standards were prepared, and each AA mixture was analyzed by HPLC for the highest points.
Determination of the activity of antioxidant enzymes in plants (POD, CAT, SOD)
For the extraction of enzymes, 0.5 g of fresh plant leaves was taken, placed in a mortar, and ground until they turned into powder by adding liquid nitrogen. Then, 5 ml of cold homogenate buffer (0.1 M KH2PO4, pH 7.0, containing 1% PVP and 1 mm EDTA) was added, and the mixture was transferred to a tube and centrifuged at 15000xg and +4°C for 15 minutes. The supernatant obtained by centrifugation was used as a source for measuring the activity of antioxidant enzymes.Citation25,Citation26
Catalase (CAT) converts H2O2 into O2 and H2O in the activity measurement environment; its activity is monitored based on the resulting decrease in absorbance at 240 nm.Citation27
The peroxidase (POD) activity was determined by monitoring the absorbance increase at 470 nm caused by the colored compoundCitation28 that is the product of the reaction in which fecal H2O2 is the substrate.Citation26
Superoxide dismutase (SOD) activity was analyzed based on the spectrophotometric determination of the inhibition of blue nitro tetrazolium (NBT) photochemical reduction.Citation29,Citation30
Translocation factor (TF) and bioaccumulation factor (BAF)
TF expresses the displacement of metals from roots to shoots by Equationequation (1)(1)
(1) . It was calculated based on the methods suggested byCitation31 and .
BAF explains the amount of metal absorption from the soil into the plant shoots by Equationequation (2)(2)
(2) .
TF > 1 signifies that the plant effectively translocates trace metals from the roots to the shoots . BAF values are categorized as (excluder) 1< (accumulator) <10 (hyperaccumulator) .
Statistical analysis
All data were examined by SPSS 22 software of ANOVA. Duncan’s test was carried out to identify significant differences in the means (p < .05). Based on the analytical results obtained, a multi-linear regression was conducted to model the relationship between amino acids and organic acids in heavy metal uptake in plants.
Results
The TF and BAF provide very important information about the HM accumulation and absorption capacity of plants. Plants either store metals absorbed from the soil in their roots or transfer them to the stem.Citation32 BAF values was calculated plants of SP-EA-TA-SA (Cd 12.33–15.29-21.12–28.69, Cr 0.54–0.71-0.97–1.34, Hg 0.19–0.21-0.23–0.16, Pb 6.27–7.41-6.98–6.42, Ni 25.72–29.29-27.82–23.36, Sn 1.02–1.15-1.10–1.01, Se 1.77–2.62-1.58–1.65. The TF and BAF values of the study samples with HM pollution (PA) were given in S1. In addationaly, the results of the analysis of pH, EC, OM %, and Calcif % of the nutrients and HM content of the soil samples taken from root regions were given in S2, S3, S4.
The nutrient content analysis of the leaf, stem, and root regions of the samples collected from PA showed statistically significant differences (p < .05) (S5). A significant decrease was observed in the nutrient content of the plant samples collected from the PA compared to NPA. This decrease obtained: N 54.35%, P 46.24%, K 40.05%, C 52.58%, Mg 22.77%, Na 53.36%, Zn 60.85%, Fe 16.01%, Mn 22.50%, Cu 25.72%, and B 36.16%. However, there was a 432.44% increase in the concentration of Cl.
HM content analysis in the leaf, stem, and root regions of samples collected from the PA showed statistically significant differences (p < .05). The regions with the highest concentration of HM were leaf > stem > root (). However, there was no statistically significant difference observed in terms of the HM content in samples collected from the NPA. Moreover, the buildup of HMs in plant organs differed based on the plant species. (S6, ). Four plants content means of the PA samples showed higher concentrations of HMs than the NPA. These increases were determined as Cd 1778.30%, Cr 587.46%, Hg 855.23%, Pb 3371.31%, Ni 1040.47%, Sn 598.39%, Se and 21092.15%.
AA metabolism plays an important role in the accumulation of osmolytes as part of the stress tolerance mechanism,Citation33 regulation of intracellular pH, and detoxification of ROS, HMs, and xenobiotics.Citation34 Statistically significant differences between plant organs in AA levels in PA samples (p < .05) were found. The highest AA content was usually on the stem ≥ leaf > root, respectively. But the highest content of AA in plants collected from the NPA was in the order of leaf > stem > root. In the plant samples from the PA, an increase in the AA content was seen in the stem portion. However, there were no statistical differences between the leaves and stems (S7, ). The AA content of the plant samples from the PA showed a significant increase compared to those from the NPA. These increases were calculated as Asp 28.12%, Glu 28.50%, Asn 39.22%, Ser 51.34%, Gln 32.14%, His 19.91%, Gly 37.02%, The 63.59%, Arg 29.02%, Ala 5.12%, Tyr 41.12%, Cys 24.00%, Val% 51.03, Met 43.27%, Trp 38.51%, Phe 28.22%, Ile 32.64%, Leu 41.07%, Lys 14.30%, Sarcosine 39.38%, Hyp 30.60% ().
Analysis of the OA content showed statistically significant differences among the organs (p < .05) (S8). The OA content in PA plant samples was found to be higher than in the NPA plant samples: Oxalic acid 91.02%, Propionic acid 60.70%, Tartaric acid 60.75%, Butyric acid 62.93%, Malonic acid 35.85%, Malic acid 44.64%, Lactic acid 72.43%, Citric acid 55.10%, Maleic acid 77.6%, Fumaric acid 44.77%, and Succinic acid 42.60% ().
Antioxidant and proline levels showed statistically significant differences among the plant regions (p < .05) (S9). It was found that the antioxidant, proline, MDA, and H2O2 content of plant samples from the PA was higher than that of the samples from the NPA. These increases was determineted as CAT 19.53%, POD 15.76%, SOD 22.25%, Proline 39.69%, H2O2 33.61%, and MDA 42.54% (, S10).
Discussion
Plants either store metals absorbed from the soil in their roots or transfer them to the stem. Based on BAF, plants are classified as (excluder) 1< (accumulator) <10 (hyperaccumulator), respectively.Citation35 According to the results of four plants of heavy metals content, Plants (SP, EA, SA and TA) were identified as Cd and Ni hyperaccumulator, Pb, Sn, and Se accumulator. They were also plants Cr and Hg excluder. On the other hand, the SA only showed the characteristic of the accumulator for Cr.
AAs are important metabolites with different functions, such as cell osmotic regulation, absorption of mineral nutrients, detoxification of HMs, and signaling.Citation17,Citation36–38 In this study, a linear, multivariate regression model was used to determine which AA in four different plant samples from PA were effective in the detoxification of different heavy metals in plants. Therefore, the best linear regression model was adjusted as a result of linear correlations of AAs for all plant species (). As a result of Cd amount of four plant species collected from PA in this study, there were differences in terms of the correlation between Cd uptake and AAs. For this reason, a linear multivariate regression model was used to see which AAs showed a higher linear correlation with the Cd content of plant samples. As a result of the adjusted of best linear model, the interaction of Gly, Thr, Tyr, Lys, and Leu in phytochelatin of the Cd is explained at a rate of 73.29% (p < .001) (). It was estimated that 1 unit increase in the amount of Gly and Leu would contribute to the 0.56 and 0.10 units chelation of Cd into plants. Whereas, in a study was reported on lettuce that Glu, Tyr, Lys, Asp play a role in the formation of a cadmium-containing PCS complex and protect it.Citation39 Another study, Try, Lys, and His was observed accumulated more in all tissues of Massai grass exposed to Cd, and reported that these amino acids were probably implicated in Cd accumulation and detoxification in this process.Citation40 Furthermore, a study showed that the AA contents in the root exudates from the rice line with high Cd accumulation, except for Tyr, which increased significantly as the Cd increased. Whereas, in the control group, only Met, Lys and His increased.Citation41
Table 1. Multilinear regression model for heavy metal detoxification by amino acids.
The interaction of Asn, Val, Trp, Ile in phytochelatin of the Pb is explained at a rate of 90.32% (p < .001) (). It was estimated that 1 unit increase in the amount of Trp and Ile would contribute to the 0.20 and 0.08 units chelation of Pb into plants. A statistically significant increase in Vetiver, Asn, Pro, His, Trp, Val, Ile, Thr, Nicotinamide, and Met was observed under Pb stress.Citation42 On the other hand, it was found that higher levels of Pb removed AAs from radish roots.Citation43
The interaction of Asp, Glu, Asn, Ser, Leu in phytochelatin of the Cr is explained at a rate of 82.36% (p < .001) (). It was estimated that a 1 unit increase in the amount of Asp, Ser and Leu would contribute to the 0.011, 0.0009, and 0.033 units chelation of Cr into plants. The underlying events of defense signal transduction against Cr toxicity haven’t been partially elucidated. Glutamic acid, Ser, glutamine, β-alanine, Tyr, Cys, Met, and IleCitation44 content of the samples prepared from the Juncea seedlings grown in Cr solutions decreased in comparison with control seedlings.Citation45 But their Lys and Pro contents increased compared to the control seedlings.Citation44
High Hg intake in plants may cause adverse phytotoxic effects.Citation46 Although largely retained in the roots, small amounts of accumulation can also occur in shoots by displacement of soluble forms or uptake of the gaseous form directly through the leaves.Citation47 Relatively little is known about the molecular mode of action and defense responses of Hg stress. In our research, Tyr and Ser were found responsible for phytochelation of Hg with 92.26%. (p < .001); Notably, Ser alone acted as a phytochelatin at the rate of 92.17%. This regression effect demonstrated that Ser may be an important AA in Hg chelation. It was estimated that a 1 unit increase in the amount of Ser would contribute to the 0.0004 units chelation of Hg into plants. Many researchs of literature refer to Glu-Cys and Gly as phytochelatins of Hg. For this reason, studies on the role of serine in Hg uptake and chelation should be undertaken.Citation48It was reported that inorganic Hg can primarily affect soluble compartments, while methyl Hg primarily affects insoluble compartments, and that levels of Phe, Thr, and Try increased in Elodea nuttallii shoots under stress.Citation49
The interaction of Phe, Trp, Lys, Glu, in phytochelatin of the Ni is explained at a rate of 96.87% (p < .001) (). Ni concentrations of the plants, it was estimated that an increase of 1 unit in the amount of Trp and Glu would contribute to the chelation of 0.05 and 0.031 units of Ni in the plants. In the study by,Citation50 alyssum, a Ni hyperaccumulator, was found to generate His proportional when exposed to nickel. Moreover, they found no uptake of nickel His in the non-Ni hyperaccumulator. In additionally, a study on wheat showed that, compared to control, the highest Ni uptake occurred in the presence of Gln. However, the use of both Gln and Gly significantly increased the root Ni accumulation, while His did not have a significant effect on the Ni accumulation in the roots.Citation51
The interaction of Asp, Thr, Lys, Gly, in phytochelatin of the Sn was explained at a rate of 92.50% (p < .001) (). Sn concentrations of the plants was estimated that an increase of 1 unit in the amount of Asp, Thr and Gly would contribute to the chelation of 0.0004, 0.0007, and 0.002 units of Sn in the plants. In additional, Asn, Arg, Phe, Ile, leu only showed an effect on Se at the rate of 77.50% (p < .001) (). Se concentrations of the plants was estimated that an increase of 1 unit in the amount of Asn and Leu would contribute to the chelation of 0.004 and 0.05 units of Se in the plants. Some of studies showed, the application of Se foliar to potato tubercles has been shown to increase significantly, especially Asp, Glu, Tyr, Thr, and Phe, when compared to controlsCitation52. It has also been reported that Gly reduces the lethal effects of HMs by improving the resistance mechanisms of plants under stress.Citation53
The metabolism of OAs is of fundamental importance on the cellular and the entire plant level. Over the past few years, more attention has been paid to the role of OAs in the modulation of environmental adaptation,Citation54 including the contribution of OAs to the detoxification of HMs. The basis of this is the ability of OAs like citrate, malate, oxalate, malonate, citrate, and tartaric acids to form strong bonds with HM ions by chelation of metals, as well as their effect on the carboxyl groupsCitation55 which transport donor oxygen functions in metal ligands.
In our study, a statistically significant correlation was found between OA levels and HM concentrations in plants (p < .001). Therefore, a linear, multivariate regression model was tested to determine which OA’ amounts in four different plant samples from PA were effective in the detoxification of different heavy metals in plants. Thus, the best linear regression model was adjusted as a result of linear correlations of OAs for all plant species ().
Table 2. Multilinear regression model for heavy metal detoxification by organic acids.
The interaction of Oxalic acid, malonic acid, Malic acid, Lactic acid, Citric in phytochelatin of the Cd is explained at a rate of 67.89% (p < .05) (). Cd concentrations of the plants was estimated that an increase of 1 unit in the amount of Malonic acid and Malic acid would contribute to the chelation of 5.60 and 2.03 units of Cd in the plants. In a study, low concentrations of tartrate or citrate inhibited Cd desorption in soil, while high concentrations of citrate and tartrate promoted Cd desorption.Citation56 Cr concentrations of the plants was estimated that an increase of 1 unit in the amount of Oxalic acid and Malic acid could contribute to the chelation of 0.09 and 0.2 units of Cr in the plants. Hg concentrations of the plants was estimated that an increase of 1 unit in the amount of Oxalic acid, Succinic acid, Citric acid, and Butyric acid could contribute to the chelation of 0.0009, 0.001, 0.01 and 0.0.003 units of Hg in the plants. Pb concentrations of the plants was estimated that an increase of 1 unit in the amount of Malonic acid could contribute to the chelation of 4.41 units of Pb in the plants. Ni concentrations of the plants was estimated that an increase of 1 unit in the amount of Malonic acid and Malic acid could contribute to the chelation of 0.7 and 0.5 units of Ni in the plants. Sn concentrations of the plants was estimated that an increase of 1 unit in the amount of Malonic acid, Maleic acid and Malic acid could contribute to the chelation of 0.009, 0.007 and 0.02 units of Sn in the plants. Se concentrations of the plants was estimated that an increase of 1 unit in the amount of Malonic acid and Citric acid could contribute to the chelation of 0.29 and 0.14 units of Se in the plants.
In a study on the canola plant, the changing levels of OAs showed important effects on all properties, including the accumulation of Pb in roots and stems.Citation57 Cd accumulates in shoots, whereas Cr accumulates in the roots, shoots, leaves, and the dry weight of plants. The interaction with OAs changes the concentrations of the accumulation of Pb in roots and shoots, Cd in shoots, and Cr in roots, shoots, and leaves. It also showed its significance in the dry weight of the plant.Citation57
Plants come up with strategies to counter the negative effects of HMs.Citation58 The toxicity of HMs in a plant can result in excessive production of ROS, which causes the peroxidation of many vital cell components.Citation59 For this reason, plants have an effective defense system consisting of enzymatic and non-enzymatic antioxidantsCitation59 such as SOD, POD, and CAT. They effectively oxidize superoxide radicals to hydrogen peroxide and can directly detoxify ROS while converting non-enzymatic antioxidants of low molecular weight, such as proline, ascorbic acid, and glutathione,Citation60 to water and oxygen.Citation61–63 Enzymatic and non-enzymatic antioxidant groups quench a wide variety of toxic oxygen derivatives to protect cells from oxidative stress. Plants accumulate various metabolic products, including AAs, under HM and abiotic stresses.Citation64
There were differences in the correlation between HMs, such as Ni, Cr, Hg, Pb, Se, Sn, and Cd and antioxidants, proline, MDA, and hydrogen peroxide in plant samples from both the PA and NPA. Although a positive (p < .001) correlation was observed between Cd content and proline in NPA plants, no significant correlation was seen in PA plant samples (p > .05). The interaction between Pb and proline was completely in contrast with that between Cd and proline. Although this interaction showed a positive correlation at p < .001 in PA samples. In conjunction with the increased in Cd and Pb doses in Eruca sativa L., an increase in Proline content was observed.Citation65 No significant correlation was found in the NPA samples (p > .05). On the other hand, the quantity of proline in NPA samples showed a negative correlation with the level of Cr, Ni, Hg, and Sn (p < .001). The PA samples (p < .005) showed a positive correlation with Cr, Ni, Hg, and Sn (p < .001). In a study, There is a correlation between Pro level and heavy metal accumulation, with Cd is being the strongest inducer of Pro content in cells. This association indicated that the accumulation of free Pro corresponded to the absorption of metals by S. amateurs cells.Citation66 While a negative correlation for Se was observed in plant samples collected from the NPA, it showed a positive correlation at p < .001 in plants collected from the PA.
While redox-active (Ni, Fe, and Cu) HMs instantly catalyze the formation of ROS by Fenton reactions, they induce osmotic stress by reducing inactive (Cd, Pb, and Zn) metals and antioxidants.Citation67 HM stresses trigger osmotic stress, oxidative stress, and denaturation of proteins in plants. The rate of ROS clearance and acceleration of the antioxidant system lead to cellular responses such as the activation of the formation of stress proteins.Citation68 In general, plants in reaction to stress produce antioxidant enzymes (SOD, CAT, POD) to prevent the harmful effects of ROS and contribute to the stress response.Citation69,Citation70 Plants produce organ-specific antioxidant defense components depending on the type of stress and developmental stage to cope with oxidative stress.Citation71 As a result of our study, antioxidant enzyme activity showed a positive correlation with Cd content in plant samples from the NPA for CAT, POD, and SOD at p < .001, while it showed a positive correlation at p < .05 in plant samples collected from the PA. It showed similar results for detoxification with proline and H2O2 in samples containing HMs Cr, Ni, Hg, and Sn. In this study, we found that the highest content of all antioxidant enzymes, except proline, was in the leaves. On the other hand, proline was highest in the stem region of the plant (S10). Proline content varied by type of heavy metals and among organs. These results can be a defense mechanism by Proline’s chelating capacity and its binding to metal ions. In a study of Cd applied to mung beans, Proline showed a negatively correlated with antioxidant enzymes. However, Proline content was not significant with MDA. It was also reported similar result byCitation72 that their study conducted with Spirulina platensis-S5, an increase in both proline and MDA contents occurred with increasing metal ion concentration. Moreover, they reported a correlation between free radical formation and proline accumulation.Citation72 In addationaly, all antioxidant enzymes and MDA content were also found to have a significant positive correlation.Citation73 In our studied showed an increase in proline and MDA levels with an increase in the concentration of metallic ions. The fact that this increase was high in the stem portion of the plant was indicative of a correlation between free radical formation and proline accumulation (). The activities of CAT, SOD, and POD showed higher total activity in the leaves.
H2O2 has a long lifespan and is highly permeable.Citation74 It is through the membrane and can quickly diffuse from cell to cell within plants. Therefore, it is reactive, and is easily controlled by antioxidant enzymes as it is quickly produced.Citation74 Based on these results, it is possible to conclude that the increase in H2O2 production in plants with a high heavy metal content may be linked to the high antioxidant activity observed (). H2O2 provides all important criteria for intercellular signals to a high degree. Therefore, it should be considered as an Oxidative signaling molecule.Citation74 Moreover, H2O2 and MDA not only act as signaling molecules under the stress of heavy metals but also play a major role in many physiological events, such as hypersensitive responses and stomatal conductance.Citation74
In plants, HM stress and detoxification of ROS species are associated with proline accumulation. In this way, it protects the membrane integrity of the plant cell and regulates the osmoregulation of the cell, thereby protecting plants against oxidative damage.Citation63,Citation75–77 In this study, the amount of H2O2 in the plant samples showed a parallel correlation with proline.
Conclusion
The exogenous application of different organic or inorganic compounds and their curative effects on HM-induced toxicity in plants are promising studies to clean up polluted land and improve crops. Therefore, it is important to understand the tolerance mechanisms for plant species in the natural environment against such stresses. Mechanisms that strengthen the defense mechanism in the natural environment should be supplied to the plant artificially. Because it’s a need-to-know to try to understand how exposure to HM contributes to the development of crop plants.
This research work aimed at identifying the different approaches to transportation and internal chelation of metals by AAs and OAs in plants. A multiple linear regression model was used to identify the AAs and OAs that jointly affect the chelation and stabilization of HMs in plants in response to metal toxicity and their force of action.
Related to our hypothesis, we can conclude the effect of heavy metals in the plant on phytochelation ability on the increase from one to five (pmol μL−1) of various types of amino acids as follow; Gly and Leu (%19.41, Cd), Trp and Ile (11.32%, Pb), Asp, Ser, and Leu (42.57%, Cr), Trp and Glu (25%, Ni). The effect of heavy metals in the plant on phytochelation ability on the increase from 0.1 to 0.5 (ng/microgram) of various types of Organic acids as follow; Malonic acid and Malic (19.16%, Cd), Malonic acid (10.37%, Pb), Malonic acid and Malic acid (445% Ni). Regression analysis consists of determining the relationship between two or more variables that have a cause and effect relationship. Used this relationship, it is made in order to make an estimation or a prediction on this topic. It is also possible to find a cause and effect relationship with many events in the wild. This estimation can show another aproach to improve heavy metal uptaking by plants and remediation of soils. Therefore, this research needs to in vitro experimental results.
Ser alone acted in Hg phytochelatin at the rate of 92.17%. This regression effect demonstrated that Ser may be an important AA in Hg chelation. For this reason, studies on the role of serine in Hg uptake and chelation should be undertaken
With the increase in the concentration of metal ions, the levels of proline and MDA increased significantly in the stem part of the plant. This increase points to a correlation between free radical formation and proline concentration. The CAT, SOD and POD activities showed a higher total activity in the leaves. It can be concluded that increased production of H2O2 in plants leaves with a high heavy metal content can be associated with high antioxidant activity. In addationally, Proline content varied by type of heavy metals and among organs. Therefore, these results can be a defense mechanism by Proline’s chelating capacity and related its binding to metal ions.
Author contributions
Corresponding Author contributed to the study conception and design. Material preparation, data collection and analysis were performed by Ayhan KOCAMAN.
Supplemental Material
Download MS Word (351.8 KB)Disclosure statement
No potential conflict of interest was reported by the author(s).
Supplementary material
Supplemental data for this article can be accessed on the publisher’s website
Additional information
Funding
References
- Emamverdian A, Ding Y, Mokhberdoran F, Xie Y. Heavy metal stress and some mechanisms of plant defense response. Sci World J. 2015;2015:1–12. doi:10.1155/2015/756120.
- Clemens S, Palmgren MG, Krämer U. A long way ahead: understanding and engineering plant metal accumulation. Trends Plant Sci. 2002;7(7):309–315. doi:10.1016/S1360-1385(02)02295-1.
- Viehweger K. How plants cope with heavy metals. Bot Stud. 2014;55(1):1–12. doi:10.1186/1999-3110-55-35.
- Ahmed E, Holmstrom SJ. Siderophores in environmental research: roles and applications. Microb Biotechnol. 2014;7(3):196–208. doi:10.1111/1751-7915.12117.
- Dalvi AA, Bhalerao SA. Response of plants towards heavy metal toxicity: an overview of avoidance, tolerance and uptake mechanism. Ann Plant Sci. 2013;2:362–368.
- Kosakivska IV, Babenko LM, Romanenko KO, Korotka IY, Potters G. Molecular mechanisms of plant adaptive responses to heavy metals stress. Cell Biol Int. 2021;45(2):258–272. doi:10.1002/cbin.11503.
- Kumar A, Usmani Z, Ahirwal J, Rani P. Phytomanagement of chromium contaminated brown fields. Phytomanage Pollut Sites. 2019;447–469.
- Gavrilescu M. Enhancing phytoremediation of soils polluted with heavy metals. Curr Opin Biotechnol. 2022;74:21–31. doi:10.1016/j.copbio.2021.10.024.
- Yan A, Wang Y, Tan SN, Mohd Yusof ML, Ghosh S, Chen Z. Phytoremediation: a promising approach for revegetation of heavy metal-polluted land. Front Plant Sci. 2020;11:359. doi:10.3389/fpls.2020.00359.
- Kochian LV, Piñeros MA, Liu J, Magalhaes JV. Plant adaptation to acid soils: the molecular basis for crop aluminum resistance. Annu Rev Plant Biol. 2015;66(1):571–598. doi:10.1146/annurev-arplant-043014-114822.
- Titov A, Kaznina N, Talanova V. Tyazhelye mentally i rasteniya [Heavy metals and plants]. Petrozavodsk: KarRC of RAS; 2014.
- Rai V. Role of amino acids in plant responses to stresses. Biol Plant. 2002;45(4):481–487. doi:10.1023/A:1022308229759.
- Manara A. Plant responses to heavy metal toxicity. Plants and heavy metals. Springer; 2012. p. 27–53.
- Gill SS, Gill R, Anjum N. Target osmoprotectants for abiotic stress tolerance in crop plants—glycine betaine and proline. Plant Adapt Environ Change. 2014;97–108.
- Ahmad MA, Gupta M. Exposure of Brassica juncea (L) to arsenic species in hydroponic medium: comparative analysis in accumulation and biochemical and transcriptional alterations. Environ Sci Pollut Res. 2013;20(11):8141–8150. doi:10.1007/s11356-013-1632-y.
- Paleg L, Douglas T, Van Daal A, Keech D. Proline, betaine and other organic solutes protect enzymes against heat inactivation. Funct Plant Biol. 1981;8(1):107–114. doi:10.1071/PP9810107.
- Sharma SS, Dietz K-J. The significance of amino acids and amino acid-derived molecules in plant responses and adaptation to heavy metal stress. J Exp Bot. 2006;57(4):711–726. doi:10.1093/jxb/erj073.
- Theriappan P, Gupta AK, Dhasarrathan P. Accumulation of proline under salinity and heavy metal stress in cauliflower seedlings. J Appl Sci Environ Manage. 2011;15(2). doi:10.4314/jasem.v15i2.68497.
- Zenk MH. Heavy metal detoxification in higher plants-a review. Gene. 1996;179(1):21–30. doi:10.1016/S0378-1119(96)00422-2.
- Sharma S, Vredenburg H. Proactive corporate environmental strategy and the development of competitively valuable organizational capabilities. Strategic Manage J. 1998;19(8):729–753. doi:10.1002/(SICI)1097-0266(199808)19:8<729::AID-SMJ967>3.0.CO;2-4.
- Mertens D. AOAC official method 922.02. Plants preparation of laboratory sample. Off Methods Anal Chapter. 2005;3:20877–22417.
- Heinrikson RL, Meredith SC. Amino acid analysis by reverse-phase high-performance liquid chromatography: precolumn derivatization with phenylisothiocyanate. Anal Biochem. 1984;136(1):65–74. doi:10.1016/0003-2697(84)90307-5.
- Saunders J, Saunders J, Morris S, Wynne S. Amino acid analysis of subcellular fractions by PITC and OPA. Chromatogram. 1988;9:2–4.
- Senden M, Van der Meer A, Limborgh J, Wolterbeek HT. Analysis of major tomato xylem organic acids and PITC-derivatives of amino acids by RP-HPLC and UV detection. Plant Soil. 1992;142(1):81–89. doi:10.1007/BF00010177.
- Angelini R, Federico R. Histochemical evidence of polyamine oxidation and generation of hydrogen peroxide in the cell wall. J Plant Physiol. 1989;135(2):212–217. doi:10.1016/S0176-1617(89)80179-8.
- Angelini R, Manes F, Federico R. Spatial and functional correlation between diamine-oxidase and peroxidase activities and their dependence upon de-etiolation and wounding in chick-pea stems. Planta. 1990;182(1):89–96. doi:10.1007/BF00239989.
- Havir EA, McHale NA. Biochemical and developmental characterization of multiple forms of catalase in tobacco leaves. Plant Physiol. 1987;84(2):450–455. doi:10.1104/pp.84.2.450.
- Yildirim E, Ekinci M, Turan M. Impact of biochar in mitigating the negative effect of drought stress on cabbage seedlings. J Soil Sci Plant Nutr. 2021;21(3):2297–2309. doi:10.1007/s42729-021-00522-z.
- Agarwal S, Pandey V. Antioxidant enzyme responses to NaCl stress in Cassia angustifolia. Biol Plant. 2004;48(4):555–560. doi:10.1023/B:BIOP.0000047152.07878.e7.
- Yordanova RY, Christov KN, Popova LP. Antioxidative enzymes in barley plants subjected to soil flooding. Environ Exp Bot. 2004;51(2):93–101. doi:10.1016/S0098-8472(03)00063-7.
- Malik RN, Husain SZ, Nazir I. Heavy metal contamination and accumulation in soil and wild plant species from industrial area of Islamabad, Pakistan. Pak J Bot. 2010;42:291–301.
- Bhat IUH, Mauris EN, Khanam Z. Phytoremediation of iron from red soil of tropical region by using Centella asiatica. Int J Phytoremediation. 2016;18(9):918–923. doi:10.1080/15226514.2016.1156637.
- Campalans A, Messeguer R, Goday A, Pagès M. Plant responses to drought, from ABA signal transduction events to the action of the induced proteins. Plant Physiol Biochem. 1999;37(5):327–340. doi:10.1016/S0981-9428(99)80039-4.
- Mohanty P, Matysik J, Matysik J. Effect of proline on the production of singlet oxygen. Amino Acids. 2001;21(2):195–200. doi:10.1007/s007260170026.
- Ma LQ, Komar KM, Tu C, Zhang W, Cai Y, Kennelley ED. A fern that hyperaccumulates arsenic. Nature. 2001;409(6820):579. doi:10.1038/35054664.
- Barneix AJ. Physiology and biochemistry of source-regulated protein accumulation in the wheat grain. J Plant Physiol. 2007;164(5):581–590. doi:10.1016/j.jplph.2006.03.009.
- Harding HP, Zhang Y, Zeng H, Novoa I, Lu PD, Calfon M, Sadri N, Yun C, Popko B, Paules R, et al. An integrated stress response regulates amino acid metabolism and resistance to oxidative stress. Mol Cell. 2003;11(3):619–633. doi:10.1016/S1097-2765(03)00105-9.
- Kumar A, Dwivedi S, Singh R, Chakrabarty D, Mallick S, Trivedi P, Adhikari B, Tripathi RD. Evaluation of amino acid profile in contrasting arsenic accumulating rice genotypes under arsenic stress. Biol Plant. 2014;58(4):733–742. doi:10.1007/s10535-014-0435-4.
- Yazdi M, Kolahi M, Kazemi EM, Barnaby AG. Study of the contamination rate and change in growth features of lettuce (Lactuca sativa Linn.) in response to cadmium and a survey of its phytochelatin synthase gene. Ecotoxicol Environ Saf. 2019;180:295–308. doi:10.1016/j.ecoenv.2019.04.071.
- Rabêlo FHS, Fernie AR, Navazas A, Borgo L, Keunen E, da Silva B, Cuypers A, Lavres J. A glimpse into the effect of sulfur supply on metabolite profiling, glutathione and phytochelatins in Panicum maximum cv. Massai exposed to cadmium. Environm Exp Bot. 2018;151:76–88. doi:10.1016/j.envexpbot.2018.04.003.
- Fu H, Yu H, Li T, Zhang X. Influence of cadmium stress on root exudates of high cadmium accumulating rice line (Oryza sativa L.). Ecotoxicol Environ Saf. 2018;150:168–175. doi:10.1016/j.ecoenv.2017.12.014.
- Pidatala VR, Li K, Sarkar D, Wusirika R, Datta R. Comparative metabolic profiling of vetiver (Chrysopogon zizanioides) and maize (Zea mays) under lead stress. Chemosphere. 2018;193:903–911. doi:10.1016/j.chemosphere.2017.11.087.
- Wang Y, Xu L, Shen H, Wang J, Liu W, Zhu X, Wang R, Sun X, Liu L. Metabolomic analysis with GC-MS to reveal potential metabolites and biological pathways involved in Pb & Cd stress response of radish roots. Sci Rep. 2015;5(1):1–13. doi:10.1038/srep18296.
- Handa N, Kohli SK, Thukral AK, Bhardwaj R, Alyemeni MN, Wijaya L, Ahmad P. Protective role of selenium against chromium stress involving metabolites and essential elements in Brassica juncea L. seedlings. 3 Biotech. 2018;8(1):1–14. doi:10.1007/s13205-018-1087-4.
- Berlin M, Barchel D, Brik A, Kohn E, Livne A, Keidar R, Tovbin J, Betser M, Moskovich M, Mandel D. Maternal and newborn thyroid hormone, and the Association With Polychlorinated Biphenyls (PCBs) burden: the EHF (Environmental Health Fund) birth Cohort. Front Pediatr. 2021;9. doi:10.3389/fped.2021.705395.
- Rocha JE, Guedes TA, Bezerra CF, Costa M, Campina FF, de Freitas TS, Souza AK, Sobral Souza CE, Silva MKN, Lobo YM, et al. Mercury chloride phytotoxicity reduction using antioxidative mechanisms evidenced by caffeic acid FTIR. Appl Geochem. 2019;104:109–115. doi:10.1016/j.apgeochem.2019.03.015.
- Ranieri E, Moustakas K, Barbafieri M, Ranieri AC, Herrera‐Melián JA, Petrella A, Tommasi F. Phytoextraction technologies for mercury‐and chromium‐contaminated soil: a review. J Chem Technol Biotechnol. 2020;95(2):317–327. doi:10.1002/jctb.6008.
- Cosio C, Renault D. Effects of cadmium, inorganic mercury and methyl-mercury on the physiology and metabolomic profiles of shoots of the macrophyte Elodea nuttallii. Environ Pollut. 2020;257:113557. doi:10.1016/j.envpol.2019.113557.
- Feng Z, Ji S, Ping J, Cui D. Recent advances in metabolomics for studying heavy metal stress in plants. TrAC Trends Anal Chem. 2021;143:116402. doi:10.1016/j.trac.2021.116402.
- Krämer U, Cotter-Howells JD, Charnock JM, Baker AJ, Smith JAC. Free histidine as a metal chelator in plants that accumulate nickel. Nature. 1996;379(6566):635–638. doi:10.1038/379635a0.
- Sun L, Xu X, Jiang Y, Zhu Q, Yang F, Zhou J, Yang Y, Huang Z, Li A, Chen L. Genetic diversity, rather than cultivar type, determines relative grain Cd accumulation in hybrid rice. Front Plant Sci. 2016;7:1407. doi:10.3389/fpls.2016.01407.
- Etesami H, Maheshwari DK. Use of plant growth promoting rhizobacteria (PGPRs) with multiple plant growth promoting traits in stress agriculture: action mechanisms and future prospects. Ecotoxicol Environ Saf. 2018;156:225–246. doi:10.1016/j.ecoenv.2018.03.013.
- Ježek P, Hlušek J, Lošák T, Jůzl M, Elzner P, Kráčmar S, Buňka F, Martensson A. Effect of foliar application of selenium on the content of selected amino acids in potato tubers (Solanum tuberosum L.). Plant Soil Environ. 2011;57(No. 7):315–320. doi:10.17221/57/2011-PSE.
- Hanushek EA, Woessmann L. Education and economic growth. Econ Edu. 2010;60:67.
- Osmolovskaya N, Viet Vu D, Kuchaeva L. The role of organic acids in heavy metal tolerance in plants. Biol Commun. 2018;63(1):9–16. doi:10.21638/spbu03.2018.103.
- Gao Y, He J, Ling W, Hu H, Liu F. Effects of organic acids on copper and cadmium desorption from contaminated soils. Environ Int. 2003;29(5):613–618. doi:10.1016/S0160-4120(03)00048-5.
- Ebrahimian E, Bybordi A. Effect of organic acids on heavy-metal uptake and growth of canola grown in contaminated soil. Commun Soil Sci Plant Anal. 2014;45(13):1715–1725. doi:10.1080/00103624.2013.875206.
- Hasanuzzaman M. Agronomic crops: volume 3: stress responses and tolerance. Springer Nature; 2020.
- Singh M, Kumar J, Singh S, Singh V, Prasad S, Singh M. Adaptation strategies of plants against heavy metal toxicity: a short review. Biochem Pharmacol. 2015;4:2167–0501.1000161.
- Gupta DK, Palma JM, Corpas FJ. Reactive oxygen species and oxidative damage in plants under stress. Springer; 2015.
- Yadav G, Srivastava PK, Singh VP, Prasad SM. Light intensity alters the extent of arsenic toxicity in Helianthus annuus L. seedlings. Biol Trace Elem Res. 2014;158(3):410–421. doi:10.1007/s12011-014-9950-6.
- Singh S, Prasad SM. Growth, photosynthesis and oxidative responses of Solanum melongena L. seedlings to cadmium stress: mechanism of toxicity amelioration by kinetin. Sci Hortic (Amsterdam). 2014;176:1–10. doi:10.1016/j.scienta.2014.06.022.
- Huang H, Ullah F, Zhou D-X, Yi M, Zhao Y. Mechanisms of ROS regulation of plant development and stress responses. Front Plant Sci. 2019;10:800. doi:10.3389/fpls.2019.00800.
- Shah V, Daverey A. Effects of sophorolipids augmentation on the plant growth and phytoremediation of heavy metal contaminated soil. J Clean Prod. 2021;280:124406. doi:10.1016/j.jclepro.2020.124406.
- Yildirim E, Ekinci M, Turan M, Güleray A, Selda Ö, Dursun A, et al. Impact of cadmium and lead heavy metal stress on plant growth and physiology of rocket (Eruca sativa L.). Kahramanmaras Sütçü Imam Üniversitesi Tarim ve Doga Dergisi. 2019;22:843–850.
- El-Enany A, Issa A. Proline alleviates heavy metal stress inScenedesmus armatus. Folia Microbiol (Praha). 2001;46(3):227–230. doi:10.1007/BF02818538.
- Valko M, Jomova K, Rhodes CJ, Kuča K, Musílek K. Redox-and non-redox-metal-induced formation of free radicals and their role in human disease. Arch Toxicol. 2016;90:1–37. doi:10.1007/s00204-015-1579-5.
- Zhu J-K. Salt and drought stress signal transduction in plants. Annu Rev Plant Biol. 2002;53:247–273. doi:10.1146/annurev.arplant.53.091401.143329.
- Hossain MA, Piyatida P, da Silva JAT, Fujita M. Molecular mechanism of heavy metal toxicity and tolerance in plants: central role of glutathione in detoxification of reactive oxygen species and methylglyoxal and in heavy metal chelation. J Bot. 2012;2012:1–37. doi:10.1155/2012/872875.
- Mishra P, Bhoomika K, Dubey R. Differential responses of antioxidative defense system to prolonged salinity stress in salt-tolerant and salt-sensitive Indica rice (Oryza sativa L.) seedlings. Protoplasma. 2013;250:3–19. doi:10.1007/s00709-011-0365-3.
- AbdElgawad H, Zinta G, Hegab MM, Pandey R, Asard H, Abuelsoud W. High salinity induces different oxidative stress and antioxidant responses in maize seedlings organs. Front Plant Sci. 2016;7:276. doi:10.3389/fpls.2016.00276.
- Choudhary M, Jetley UK, Khan MA, Zutshi S, Fatma T. Effect of heavy metal stress on proline, malondialdehyde, and superoxide dismutase activity in the cyanobacterium Spirulina platensis-S5. Ecotoxicol Environ Saf. 2007;66:204–209. doi:10.1016/j.ecoenv.2006.02.002.
- Hassan M, Israr M, Mansoor S, Hussain SA, Basheer F, Azizullah A. Acclimation of cadmium-induced genotoxicity and oxidative stress in mung bean seedlings by priming effect of phytohormones and proline. Plos one. 2021;16(9):e0257924. doi:10.1371/journal.pone.0257924.
- Cheng Y, Song C. Hydrogen peroxide homeostasis and signaling in plant cells. Sci China Series C Life Sci-English Edition. 2006;49:1.
- Hussain A, Kamran MA, Javed MT, Hayat K, Farooq MA, Ali N, Ali N, Ali M, Manghwar H, Jan F, et al. Individual and combinatorial application of Kocuria rhizophila and citric acid on phytoextraction of multi-metal contaminated soils by Glycine max L. Environ Exp Bot. 2019;159:23–33. doi:10.1016/j.envexpbot.2018.12.006.
- Ling T, Gao Q, Du H, Zhao Q, Ren J. Growing, physiological responses and Cd uptake of Corn (Zea mays L.) under different Cd supply. Chem Speciation Bioavailability. 2017;29:216–221. doi:10.1080/09542299.2017.1400924.
- Su T, Li W, Wang P, Ma C. Dynamics of peroxisome homeostasis and its role in stress response and signaling in plants. Front Plant Sci. 2019;10:705. doi:10.3389/fpls.2019.00705.