ABSTRACT
In eukaryotes, EPSINs are Epsin N-terminal Homology (ENTH) domain-containing proteins that serve as monomeric clathrin adaptors at the plasma membrane (PM) or the trans-Golgi Network (TGN)/early endosomes (EE). The model plant Arabidopsis thaliana encodes for seven ENTH proteins, of which so far, only AtEPSIN1 (AtEPS1) and MODIFIED TRANSPORT TO THE VACUOLE1 (AtMTV1) localize to the TGN/EE and contribute to cargo trafficking to both the cell surface and the vacuole. However, relatively little is known about role(s) of any plant EPSIN in governing physiological responses. We have recently shown that AtEPS1 is a positive modulator of plant immune signaling and pattern-triggered immunity against flagellated Pseudomonas syringae pv. tomato (Pto) DC3000 bacteria. In eps1 mutants, impaired immune responses correlate with reduced accumulation of the receptor FLAGELLIN SENSING2 (AtFLS2) and the convergent immune co-receptor BRASSINOSTEROID INSENTIVE1-ASSOCIATED RECEPTOR KINASE1 (AtBAK1) in the PM. Here, we report that in contrast to AtEPS1, the TGN/EE-localized AtMTV1 did not contribute significantly to immunity against pathogenic Pto DC3000 bacteria. We also compared the amino acid sequences, peptide motif structures and in silico tertiary structures of the ENTH domains of AtEPS1 and AtMTV1 in more detail. We conclude that despite sharing the classical tertiary alpha helical ENTH-domain structure and clathrin-binding motifs, the overall low amino acid identity and differences in peptide motifs may explain their role(s) in trafficking of some of the same as well as distinct cargo components to their site of function, with the latter potentially contributing to differences in physiological responses.
The trans-Golgi network (TGN) serves as an important sorting station to ensure that cargo proteins and other components reach their correct subcellular location for appropriate function. In plants, the TGN functionally overlaps with the early endosome (EE) and is thus referred to as the TGN/EE.Citation1–3 As such, this cellular compartment is critical for post-Golgi trafficking of newly synthesized proteins to the plasma membrane (PM) or the vacuole; recycling of endocytosed proteins to the PM; and vacuolar trafficking of endocytosed PM proteins for degradation. Increasing evidence highlights the importance of clathrin-coated vesicle (CCV) components in cargo transport from the TGN/EE to their destination organelle. CCV formation occurs at both the PM and TGN/EE as a complex and highly coordinated process that involves distinct CCV core, adaptor, and accessory components.Citation3,Citation4 While the field is making inroads into identifying CCV components functioning at the TGN/EE and their cellular roles, their impact on physiological responses beyond plant growth and development remains largely elusive.Citation1–4
We have recently advanced this limited understanding by identifying Arabidopsis thaliana EPSIN1 (AtEPS1) as a positive modulator of plant immune responses and immunity against bacterial pathogens.Citation5 AtEPS1 is one of seven Epsin N-terminal Homology (ENTH)-domain proteins in ArabidopsisCitation6,Citation7 that are implicated as monomeric clathrin adaptors to link clathrin core components with other vesicular trafficking proteins and yet unknown lipid components to the site of CCV formation.Citation8,Citation9 Consistent with its localization to the TGN/EE,Citation8,Citation9 AtEPS1 functions in trafficking of endogenous and ectopically expressed proteins to the PM or the vacuole.Citation5,Citation9 Notably, our work identifies AtEPS1 as a critical component for the correct PM abundance of the plant immune receptor FLAGELLIN SENSING2 (AtFLS2), the convergent co-receptor BRASSINOSTEROID INSENTIVE1-ASSOCIATED RECEP-TOR KINASE1 (AtBAK1) and a small subset of structurally diverse PM proteins.Citation5 We found that loss of AtEPS1 does not affect ligand-induced endocytosis of AtFLS2Citation5 and constitutive bulk membrane endocytosis.Citation9 Consistent with decreased PM accumulation of FLS2 and BAK1, eps1 mutants display impaired immune responses to bacterial flg22, the active 22-amino acid derivative of bacterial flagellin, and other pathogen- or damage-associated molecular patternsCitation5 that require PM-localized cognate host immune receptors and BAK1 for immune signaling. We also discovered that loss of AtEPS1 results in increased susceptibility to flagellated Pseudomonas syringae pv. tomato (Pto) DC3000 bacteria, thus linking decreased PM accumulation of AtFLS2 and AtBAK1 to impaired immunity against bacterial leaf pathogens.Citation5
So far, potential roles of the other six Arabidopsis ENTH-proteins in plant immunity have not been explored. Here, we focused on Arabidopsis MODIFIED TRANSPORT TO THE VACOULE1 (AtMTV1) because so far, it is the only other Arabidopsis ENTH-domain containing protein that localizes to the TGN/EE and serves as a monomeric clathrin adaptor in cargo trafficking to the PM and the vacuole.Citation9,Citation10 To test whether similar to AtEPS1,Citation5 AtMTV1 is required for effective resistance to virulent pathogenic Pto DC3000 bacteria, we infected leaves from 5-week-old plants Col-0 (wild-type), eps1-2 (SAIL_394_G02) null mutant,Citation5 and mtv1-2 (Salk_061811) null mutantCitation10 with the leaf bacterial pathogen Pto DC3000 (OD600 = 0.0005). Plant growth, plant infection by syringe-infiltration of Pto DC3000, leaf tissue processing and bacterial dilution plating were performed as described.Citation5 No difference in bacterial growth was observed at 0-day post-infection (dpi) between either single mutant compared to Col-0 (; p > 0.05, n = 14), confirming that similar number of bacteria were initially infiltrated into leaves of each genotype. Consistent with our previous study,Citation5 eps1-2 null mutant plants showed enhanced susceptibility as seen by the increased number of Pto DC3000 compared to Col-0 at 3 dpi (; p = 0.0038, n = 33–34). In addition, eps1-2 plants supported a significantly increased amount of Pto DC3000 bacterial colonization compared to mtv1-2 plants (; p = 0.0416, n = 33–34). However, in contrast to eps1-2 and Col-0, no statistically significant difference was observed between mtv1-2 mutant and Col-0 plants at 3 dpi (; p > 0.05, n = 33–34). Similar results were obtained in each of the four independent experiments that were combined for , indicating that unlike AtEPS1, AtMTV1 does not play any significant role in defense against Pto DC3000 bacterial infection.
Figure 1. Loss of AtEPS1 but not AtMTV1 results in significant increased susceptibility to Pto DC3000 pathogenic bacteria. (a) Using syringe infiltration of Pto DC3000 (OD600 = 0.0005) in 5-week-old leaves, eps1-2 but not mtv1-2 null mutants displayed increased susceptibility at 3 days post-infiltration (dpi) compared to wild-type Col-0. cfu/cm2 = colony forming units per area. n=14 for 0 dpi and n=33-34 for 3 dpi with n being an individual leaf tissue sample from 8 and 16 different plants, respectively. Data are shown using LOG10 scale for y-axis. Values are mean ± SEM. Statistics were conducted using Student’s t test; same letter represents no significant difference; different letter represents a significant difference. Graph combines data from four independent experiments, with each individual experiment showing similar results. (b) Different domain and peptide motif architecture between AtEPS1 and AtMTV1 are indicative of distinct physiological functions. For individual motifs, letters indicate amino acids using their single letter codes. (c) Predicted structural fold of AtEPS1 ENTH domain (green) is similar to that of resolved NMR structure for AtMTV1 ENTH-domain (ref 14) (cyan).
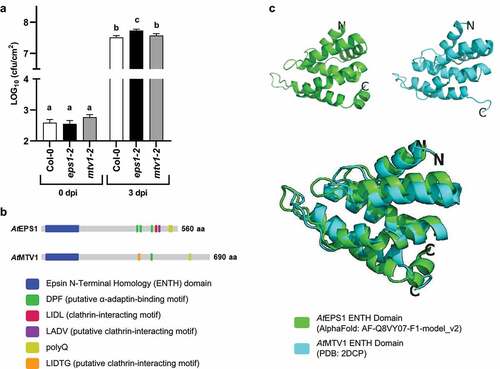
The difference in function of AtEPS1 but not AtMTV1 in contributing significantly to resistance against PtoDC3000 is unlikely due to differences in their gene expression in Arabidopsis rosette leaves, the relevant tissue used for pathogen infection assays () as Pto DC3000 is a leaf pathogen.Citation4,Citation5 Using Plant eFP Viewer public transcriptome repository (http://bar.utoronto.ca/eplant/)Citation11 to visualize the gene expression ratio of AtMTV1 (AT3G16270) to AtEPS1 (AT5G11710) showed that both ENTH genes are expressed in rosette leaves with AtMTV1 gene expression being only slightly decreased compared to AtEPS1 with a Log2 ratio between −0.45 to 0 (Supplemental Figure S1). These are relatively minor expression differences compared to the high gene expression differences of AtMTV1 to AtEPS1 in other aerial tissues, namely mature pollen (Log2 ratio = 0.89), dry seed (Log2 ratio = 2.23) and late stages of embryo development (Log2 ratio = 0.89 to 2.23; see also ref 9) (Supplemental Figure S1). In fact, when we used ePLANT eFP viewerCitation11 to visualize the relative gene expression levels of these two ENTH genes after syringe infiltration of rosette leaves with Pto DC3000 relative to its mock treatment at the corresponding hours post-infiltration, it was AtMTV1 expression that was higher (Supplemental Figure S2A; Log2 ratio = 0.67) than AtEPS1 (Supplemental Figure S2B; Log2 ratio = 0.15) at 2 hr-post-infiltration with this virulent pathogenic bacteria.
AtEPS1 and AtMTV1 are reported to function in trafficking of overlapping but also distinct cargo to the vacuole and the cell surface.Citation9 Thus, it is tempting to speculate that AtEPS1 but not AtMTV1 may govern transport of proteins with immune function to their site of function. Because for eukaryotic EPSINs, differences in protein sequences, domain and peptide motif structure dictate their interaction with distinct vesicular trafficking proteins and cargo proteins,Citation6,Citation7 we performed a more detailed analysis comparing AtEPS1 and AtMTV1 proteins. Using EMBOSS Needle pairwise alignment (https://www.ebi.ac.uk/Tools/psa/emboss_needle/) that was visualized using JalView (https://www.jalview.org/) to compare full length protein sequences (Supplemental Figure S3), we observed that these two ENTH-proteins shared overall low amino acid sequence identity (17.7%) and similarities (29.6%) including within their ENTH-domains (Supplemental Figure S3). AtEPS1 and AtMTV1 also showed differences in their peptide motif architecture in the unstructured middle and C-terminal regions (; Supplemental Figure S3). The number of putative adaptor-binding peptide motifs differ, with AtEPS1 possessing three but AtMTV1 only one DPF peptide motif (; Supplemental Figure S3). AtEPS1 and AtMTV1 have distinct clathrin binding motifs in their unstructured C-terminus, with AtMTV1 containing the putative clathrin-interacting peptide motif LIDTG (; Supplemental Figure S3) and AtEPS1 containing the confirmed LIDLCitation8 and the potential LADV clathrin-interacting motifs (, Supplemental Figure S3). Both AtEPS1 and AtMTV1 contain poly-glutamine (polyQ) stretches; but that of AtEPS1 is longer consisting of eleven glutamines compared to the four glutamines in AtMTV1. So far, roles of polyQs remain elusive for plant EPSINs; but polyQ stretches can facilitate protein-protein interactions and form cytoplasmic condensates in plants.Citation12
Due to its low primary sequence similarity to other EPSIN paralogs (including AtEPS1, Supplemental Figure S3), AtMTV1 is annotated as a phylogenetically distant ENTH-protein.Citation6,Citation9 In some cases, its ENTH domain has been referred to as a VHS domain (www.uniprot.org; Q9C5H4), an N-terminal domain named after its occurrence in Vps27, Hrs and STAM proteins and that shares extremely similar α-helical folds with ENTH-domains.Citation13 To assess whether the low primary sequence identity for their ENTH-domain may result in different tertiary structures, we downloaded the resolved Nuclear Magnetic Resonance (NMR) structure of AtMTV1 ENTH domain as published encompassing amino acids 9–135Citation14 from the Protein Data Bank (PDB) (2DCP). For comparison, we downloaded the predicted structure of AtEPS1 ENTH domain (amino acids 27–152) (this study) from Alphafold (AF-Q8VY07-F1-model_v2), an open access protein structure prediction database.Citation15,Citation16 For molecular visualization, the two ENTH structures were aligned and analyzed using the open-source program PYMOL (https://pymol.org/2/). Notably, despite their relatively low amino acid sequence similarity, the ENTH-domains from AtEPS1 and AtMTV1 were predicted to share a compact globular structure composed of seven α-helical superhelix fold that were connected by loops of varying lengths (; Supplemental Movie 1). As such, our findings are consistent with reports for other eukaryotic ENTH-proteins.Citation6,Citation13
In conclusion, we provide evidence that AtEPS1 but not AtMTV1 plays an apparent role in effective immunity against Pto DC3000 (this study), thus demonstrating differences in the physiological roles of these two TGN/EE-localized Arabidopsis ENTH-proteins in planta. Diverse biological function is consistent with AtEPS1 and AtMTV1 a) showing low sequence identity and differences in peptide motif architectures (this study); b) localizing to different regions of the TGN/EE and preferentially bind to β-subunits of distinct AP complexes;Citation9 and c) functioning in trafficking of overlapping as well as distinct cargo to the vacuole and the cell surface.Citation9 In future studies, it will be interesting to delineate whether different peptide motifs and solvent-exposed amino acids in the ENTH-domains of AtEPS1 or AtMTV1 provide binding specificity for lipids and/or proteins necessary for trafficking of distinct cellular components to their subcellular site of function to confer physiological responses.
Supplemental Material
Download Zip (3.9 MB)Acknowledgments
The authors thank the Heese lab [University of Missouri (MU)] for discussions. This work was supported by grants and fellowship from the National Science Foundation (NSF)-Plant Biotic Interactions under grant number 1758843 (to AH); NSF-Graduate Research Fellowship under grant number 1443129 (to ELM); Deutsche Forschungsgemeinschaft (DFG) SA2427/2-1 (to MS); the MU Life Science Fellowship (to KM) and the MU Wayne L. Ryan Fellowship (to KM).
Disclosure statement
No potential conflict of interest was reported by the author(s).
Supplementary material
Supplemental data for this article can be accessed online at https://doi.org/10.1080/15592324.2022.2163337
Additional information
Funding
References
- LaMontagne ED, Heese A. Trans-Golgi network/early endosome: a central sorting station for cargo proteins in plant immunity. Curr Opin Plant Biol. 2017;40:114–4. doi:10.1016/j.pbi.2017.08.012.
- Rosquete MR, Drakakaki G. Plant TGN in the stress response: a compartmentalized overview. Curr Opin Plant Biol. 2018;46:122–129. doi:10.1016/j.pbi.2018.09.003.
- Law KC, Chung KK, Zhuang X. An update on coat protein complexes for vesicle formation in plant post-golgi trafficking. Front Plant Sci. 2022;13:826007. doi:10.3389/fpls.2022.826007.
- Ekanayake G, LaMontagne ED, Heese A. Never walk alone: clathrin-coated vesicle (CCV) components in plant immunity. Annu Rev Phytopathol. 2019;57:387–409. doi:10.1146/annurev-phyto-080417-045841.
- Collins CA, LaMontagne ED, Anderson JC, Ekanayake G, Clarke AS, Bond LN, Salamango DJ, Cornish PV, Peck SC, Heese A. EPSIN1 modulates the plasma membrane abundance of FLAGELLIN SENSING2 for effective immune responses. Plant Physiol. 2020;182(4):1762–1775. doi:10.1104/pp.19.01172.
- Zouhar J, Sauer M. Helping hands for budding prospects: ENTH/ANTH/VHS accessory proteins in endocytosis, vacuolar transport, and secretion. Plant Cell. 2014;26(11):4232–4244. doi:10.1105/tpc.114.131680.
- Feng Y, Hiwatashi T, Minamino N, Ebine K, Ueda T. Membrane trafficking functions of the ANTH/ENTH/VHS domain-containing proteins in plants. FEBS Lett. 2022;596(17):2256–2268. doi:10.1002/1873-3468.14368.
- Song J, Lee MH, Lee GJ, Yoo CM, Hwang I. Arabidopsis EPSIN1 plays an important role in vacuolar trafficking of soluble cargo proteins in plant cells via interactions with clathrin, AP-1, VTI11, and VSR1. Plant Cell. 2006;18(9):2258–2274. doi:10.1105/tpc.105.039123.
- Heinze L, Freimuth N, Rößling AK, Hahnke R, Riebschläger S, Fröhlich A, Sampathkumar A, McFarlane HE, Sauer M. EPSIN1 and MTV1 define functionally overlapping but molecularly distinct trans-Golgi network subdomains in Arabidopsis. Proc Natl Acad Sci U S A. 2020;117(41):25880–25889. doi:10.1073/pnas.2004822117.
- Sauer M, Delgadillo MO, Zouhar J, Reynolds GD, Pennington JG, Jiang L, Liljegren SJ, Stierhof YD, De Jaeger G, Otegui MS, et al. MTV1 and MTV4 encode plant-specific ENTH and ARF GAP proteins that mediate clathrin-dependent trafficking of vacuolar cargo from the trans-Golgi network. Plant Cell. 2013;25(6):2217–2235. doi:10.1105/tpc.113.111724.
- Waese J, Fan J, Pasha A, Yu H, Fucile G, Shi R, Cumming M, Kelley LA, Sternberg MJ, Krishnakumar V, et al. ePlant: visualizing and exploring multiple levels of data for hypothesis generation in plant biology. Plant Cell. 2017;29(8):1806–1821. doi:10.1105/tpc.17.00073.
- Emenecker RJ, Holehouse AS, Strader LC. Sequence determinants of in cell condensate morphology, dynamics, and oligomerization as measured by number and brightness analysis. Cell Commun Signal. 2021;19(1):65. doi:10.1186/s12964-021-00744-9.
- De Camilli P, Chen H, Hyman J, Panepucci E, Bateman A, Brunger AT. The ENTH domain. FEBS Lett. 2002;513(1):11–18. doi:10.1016/s0014-5793(01)03306-3.
- López-Méndez B, Güntert P. Automated protein structure determination from NMR spectra. J Am Chem Soc. 2006;128(40):13112–13122. doi:10.1021/ja061136l.
- Jumper J, Evans R, Pritzel A, Green T, Figurnov M, Ronneberger O, Tunyasuvunakool K, Bates R, Žídek A, Potapenko A, et al. Highly accurate protein structure prediction with AlphaFold. Nature. 2021;596(7873):583–589. doi:10.1038/s41586-021-03819-2.
- Varadi M, Anyango S, Deshpande M, Nair S, Natassia C, Yordanova G, Yuan D, Stroe O, Wood G, Laydon A, et al. AlphaFold protein structure database: massively expanding the structural coverage of protein-sequence space with high-accuracy models. Nucleic Acids Res. 2022;50(D1):D439–D444. doi:10.1093/nar/gkab1061.