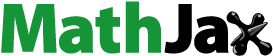
ABSTRACT
Allelopathy is the main chemical means in the invasion process of exotic plants and one of the key factors in grassland degradation. In this experiment, we investigated the effects of ethyl acetate phase extract (EAE), n-butanol phase extract (BE) and aqueous phase extract (AE) from the aboveground (stems and leaves) and roots of Ligularia sagitta on seed germination and seedling growth of four Gramineae forages (Poa pratensis L. Festuca ovina L. Elymus nutans Griseb. Agropyron cristatum (L.) Gaertn.) in their sympatric domains and one Legosuminae forage (Medicago sativa L.). The chemical components in each phase extract of L. sagitta were determined with UHPLC-MS/MS non-targeted metabolomics, and the differential compounds were screened using Orthogonal Partial Least Squares-Discriminant Analysis (OPLS-DA). Within a set concentration range, EAE significantly inhibited seed germination and seedling growth of four Gramineae forages. BE and AE acted mainly in the seedling growth stage and did not significantly inhibit forage seed germination. P. pratensis was most sensitive to L. sagitta extracts; at 2.0 mg/mL of EAE from roots, germination energy and germination rate of P. pratensis seeds were 0. L. sagitta extracts inhibited the growth of M. sativa seedlings and did not inhibit its seed germination. A total of 904 compounds were identified with UHPLC-MS/MS, among which 31, 64, 81 and 66 metabolites displayed different accumulation patterns in the four comparison groups (R.EAE vs. R.BE, R.EAE vs. R.AE, SL.EAE vs. SL.BE, SL.EAE vs. SL.AE), respectively. In particular, 9 compounds were found to be common up-regulated differential metabolites in the four comparison groups and were enriched in EAE. Additionally, N,N-dimethylaniline, Caffeic acid, 4-Hydroxybenzoic acid, 4-Hydroxybenzaldehyde and cis-9-Octadecenoic acid as potential allelochemicals in L. sagitta. The results of this study support efforts at finding alternative control plants for the restoration of poisonous grass-type degraded grasslands.
1. Introduction
In the past few decades, Plant invasions have intensified globally as a result of human activities, ongoing climate change and the destruction of natural habitats.Citation1,Citation2 Although several invasive plant species bring some benefits to the invaded sites, they also have many negative impacts on local biodiversity and ecosystems.Citation3,Citation4 The ability of invasive plant species to deplete native biodiversity through competition or the secretion of secondary metabolites and to alter ecological succession in natural habitats by altering the soil microbial environment and physicochemical properties has been demonstrated in many studies.Citation5,Citation6 It has been shown that most invasive plant species invade grassland ecosystems.Citation7 Grassland ecosystems are vital for the development of animal husbandry and sustainability of specific ecological functions in environmental protection and biodiversity conservation.Citation8 However, grassland ecosystems are fragile and vulnerable to climate change and anthropogenic disturbance, and they can easily become new habitats for invasive alien species.Citation9 An invasion of grasslands by exotic organisms leads to degradation, including a change in dominant species from native to invasive and associated reduction of good forage and decrease in biodiversity.Citation10 Grassland invasions by exotic species are directly associated with livestock production losses and indirectly associated with economic losses for the prevention and control of invasive alien species.Citation11
Most invasive plant species exhibit allelopathic effects, indicating that the role of allelopathy in promoting species invasions may be widespread.Citation12 Plant allelopathy refers to the release of allelochemicals into the environment through root secretions and subsequent rainwater leaching, which directly or indirectly affects the germination and growth of other plants.Citation13 The release of allelochemicals is a survival strategy evolved by species to obtain ecological advantages in special habitats, which may affect the growth of other plants and the succession of plant communities in the same habitat, and even affect the distribution pattern and biodiversity of plant populations in a region.Citation14,Citation15 When allelochemicals contact or approach the target plant, they directly interfere with normal plant processes such as photosynthesis and respiration, or indirectly affect the target species by changing the surrounding environment, especially soil physical, chemical and microbial properties.Citation16–18
A number of studies have shown that the plant tissue and root soil extracts of poisonous exotic weeds can reduce seed germination rate and seedling biomass of tested plants,Citation19,Citation20 and that the allelopathic effects differed significantly among the sources, extract concentrations and plant species tested. In general, allelochemicals of invasive plants have little effect in their native environment on the adjacent species due to long-term mutual adaptation; however, they exert a strong allelopathic interference effect as new substances in the invaded habitat.Citation21
Ligularia sagitta is widely distributed in alpine shrublands, riverside swamps, and meadows in western China.Citation22 The roots and leaves of L. sagitta can be used as medicine to relieve fever, cough, and nausea.Citation23,Citation24 However, L. sagitta also has certain toxicity. The pyrrolizidine alkaloids contained in L. sagitta are the main toxic components. Ingestion by livestock can cause poisoning or even death, severely inhibiting the development of animal husbandry.Citation25,Citation26 L. sagitta has recently become a dominant species in natural grasslands in the Qinghai-Tibet Plateau, and is one of the poisonous invasive weeds in degraded grasslands.Citation27–29 Studies have found that a variety of plants of the genus Ligularia such as Ligularia virgaurea and Ligularia cymbulifera, have shown allelopathic effects. The terpenoids contained in the plants of the genus are one of the sources of allelopathic activity.Citation30,Citation31
The purpose of this study was to examine the allelopathic activity of aboveground and roots extracts of L. sagitta on five forages, and its effects on germination and growth of forage seeds. The results provide a theoretical basis for future grassland restoration, and control of L. sagitta.
2. Materials and methods
2.1. Source of plant material
The whole plant of L. sagitta in vigorous growth period was collected from Maqu County, Gannan Tibetan Autonomous Prefecture, Gansu Province (E: 100°45′45′′-102°29′00′′, N: 33°06′30′′-34°30′15′′) in August 2021, and a total of 80 kg of fresh samples were collected. It was identified as L. sagitta by Professor Guo Yehong of Gansu Agricultural University. The soil on the plant was brushed clean and the plant was divided into two parts: aboveground (stems-leaves) and roots, which were air-dried and pulverized for use.
2.2. Production of extracts
Dried stems-leaves (7.6 kg) and roots (6.1 kg) samples of L. sagitta were soaked in 95% ethanol and extracted three times after 7, 5, and 3 d. The extracts obtained were combined and filtered through filter paper. The filtrate was concentrated on a rotary evaporator (40 °C) to yield the residue of stems-leaves (432 g) and roots (339 g) of L. sagitta. The resulting stems-leaves extracts and roots extracts were suspended in warm water (40 °C), respectively. And sequentially subjected to graded extraction with equal volumes of two solvents of different polarity, ethyl acetate and n-butanol. Each solvent extraction was repeated three times. The extracts obtained were combined and concentrated to dryness using a rotary evaporator along with the aqueous phase left after extraction to obtain the roots ethyl acetate extract (R.EAE, 189 g), stems-leaves ethyl acetate extract (SL.EAE, 216 g), roots n-butanol extract (R.BE, 56 g), stems-leaves n-butanol extract (SL.BE, 67 g), roots aqueous extract (R.AE, 35 g) and stems-leaves aqueous extract (SL.AE, 42 g). All extracts were stored in a refrigerator at 4°C.
2.3. Forage seeds
Seeds of four Gramineae forages (Poa pratensis L., Festuca ovina L., Elymus nutans Griseb., Agropyron cristatum (L.) Gaertn.) were collected in September 2021 in Maqu County, Gannan Tibetan Autonomous Prefecture, China (E: 100°45′45′′-102°29′00′′, N: 33°06′30′′-34°30′15′′). And one Legosuminae forage (Medicago sativa L.) were provided by the Hengyou Ecological Company in Lanzhou City, Gansu Province (September 2021). The four Gramineae species tested in this study were native to the L. sagitta invasion site, and M. sativa was a planted forage. Full and healthy seeds were selected for seed germination experiment.
2.4. Allelopathic activity determination of L. sagitta extracts
The allelopathic activity was determined according to a previously reported method with some modifications.Citation32,Citation33 The extracts of each phase (extracts prepared with different solvents) were accurately weighed, dissolved in a small amount of methanol and diluted with distilled water (working concentration ranges of 0.25, 0.50, 0.75, 1.0, and 2.0 mg/mL). The final concentration of methanol never exceeded 1.6%. Seeds were sterilized by immersion in 75% ethanol for 3 min, followed by several rinses with sterilized distilled water. Petri dishes (90 × 15 mm) were pre-lined with two layers of filter paper and moistened with 4 mL of the previously configured solution; the seeds were evenly placed in the Petri dishes with 30 seeds in each dish. The control group was placed in the same concentration of methanol aqueous solution as the experimental groups. Three replicates were set for each treatment.
Petri dishes were placed in an incubator for 12 days (light: 12 h 10,000 lux, 180 μmol m−2 s−1/dark: 12 h, 0 lux; 23 ± 0.5 °C). Distilled water was added regularly to maintain the extract concentration during culture. Germination energy (GE) is defined as the number of seeds germinated during the first 1/3 of the specified period of the germination test as a percentage of the number of seeds tested. Germination rate (GR) means the number of seeds germinated within a specified germination period as a percentage of the total number of seeds tested. GE was counted on the 4th day, and the GR was counted at the end of the experiment on the 12th day, and the calculations are shown in Equationequations (1)(1)
(1) and (Equation2
(2)
(2) ), respectively. Ten seedlings were randomly selected from each Petri dish, and root length and shoot length of the seedlings were measured with a digital Vernier caliper (0-150 mm, Harbin Measuring & Cutting Tool Group Co.,Ltd, China); fresh weight of the seedlings was determined with an analytical balance with precision to ten-thousandth of a gram. (If the number of germinated seeds was less than 10, growth parameters of all seedlings in the Petri dish were measured and the average value was calculated).
2.5. UHPLC-MS/MS analysis
Chromatographic (Vanquish UHPLC, Thermo Fisher, Germany) conditions: Hypersil Gold column (100 × 2.1 mm, 1.9 μm, Thermo Fisher, USA), Gradient elution was performed at a flow rate of 0.2 mL/min and a column temperature of 40°C. For the positive ion mode, mobile phase A was 0.1% formic acid aqueous solution and mobile phase B was methanol. For the negative ion mode, mobile phase A was 5 mM ammonium acetate solution (pH = 9.0), and mobile phase B was methanol. The gradient elution procedure was 0–1.5 min with 2% B/A, and kept for 1.5 min; 1.5–3 min with 2–85% B/A; 3–10 min with 85–100% B/A; 10–10.1 min with 100–2% B/A; 10.1–12 min with 2% B/A.
Mass spectrometry (Q ExactiveTM HF, Thermo Fisher, Germany) conditions: an electron spray ionization (ESI) instrument was used with a scanning range m/z of 100–1500; ESI was set to spray voltage of 3.5 Kv, sheath gas flow rate of 35 psi, auxiliary gas flow rate of 10 L/min, capillary temperature of 320°C, and polarity: positive, negative; MS/MS secondary scanning was data-dependent.
2.6. Data processing and multivariate analysis
Chemical analysis was based on secondary profiling information obtained from public databases of metabolite information mzCloud (https://www.mzcloud.org/), mzVault, and Masslist. After obtaining the spectral analysis data of metabolites in different samples, peak identification, integration, retention time correction, peak alignment, and mass spectrometry fragment attribution analysis were performed. The qualitative results and relative contents of the compounds were obtained after standardization of the data.
Multivariate statistical analysis of metabolomics data was performed using SIMCA 14.0 (Umetrics, Umeå, Sweden) software for hierarchical cluster analysis (HCA), principal component analysis (PCA), and orthogonal partial least squares discrimination analysis (OPLS-DA). OPLS-DA is a multivariate statistical analysis method with supervised pattern recognition that can effectively screen for differential metabolites by excluding study-irrelevant effects. Allelopathic data were tested for normality, homogeneity of variance and single factor analysis of variance (ANOVA) using SPSS 26.0 (Armonk, NY, USA). Correlations between the independent (extract concentration) and dependent variables (germination energy, germination rate, root length, shoot length and fresh weight) were tested separately using ANOVA and significance was set to (p < .05). Finally, Origin 2021 (Hampton, Massachusetts, USA) is used for graphing the figures.
3. Results
3.1. Effects of L. sagitta extracts on seed germination
Previous experiments showed that low concentration of methanol had no effect on the germination of grass seeds. Therefore, solvent interference was ignored.Citation32 The results showed that BE and AE had no significant inhibitory effect on germination of the tested forages, but EAE significantly inhibited germination of the four Gramineae forages, and the degree of inhibition gradually increased with the increase in extract concentration. The extracts of L. sagitta had no effect on the germination of M. sativa seeds.
3.1.1. Effects on germination energy of forage seeds
L. sagitta extracts significantly inhibited germination energy (GE) of P. pratensis seeds and delayed germination of P. pratensis seeds (). At 2.0 mg/mL of EAE, germination energy of P. pratensis seeds decreased to 0, and germination energy of E. nutans, A. cristatum, and F. ovina seeds decreased to 2.2, 15.6, and 23.3%, respectively (). The inhibitory effect of R.EAE on germination energy of F. ovina and E. nutans was greater than that of SL.EAE, but that on A. cristatum was less than that of SL.EAE. The extracts of L. sagitta had no significant effect on germination energy of M. sativa seeds ().
Figure 1. Effects of L. sagitta extracts on seed germination of P. pratensis. a: roots ethyl acetate extract (R.EAE), b: roots n-butanol extract (R.BE), c: roots aqueous extract (R.AE), d: stems-leaves ethyl acetate extract (SL.EAE), e: stems-leaves n-butanol extract (SL.BE), f: stems-leaves aqueous extract (SL.AE). In each graph, different letters indicate significant differences between concentration treatments, p < .05. Concentration units for treatment groups are mg/mL. Error bars indicate standard deviation (SD). (GE) germination energy; (GR) germination rate; (CK) control group.
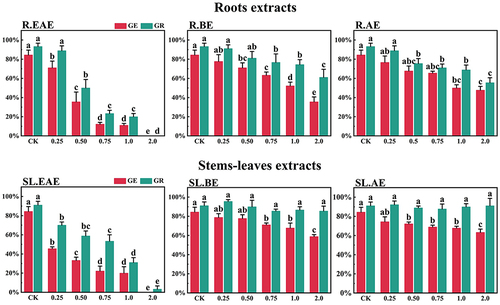
Figure 2. Effects of L. sagitta extracts on seed germination of F. ovina. a: roots ethyl acetate extract (R.EAE), b: roots n-butanol extract (R.BE), c: roots aqueous extract (R.AE), d: stems-leaves ethyl acetate extract (SL.EAE), e: stems-leaves n-butanol extract (SL.BE), f: stems-leaves aqueous extract (SL.AE). In each graph, different letters indicate significant differences between concentration treatments, p < .05. Concentration units for treatment groups are mg/mL. Error bars indicate standard deviation (SD). (GE) germination energy; (GR) germination rate; (CK) control group.
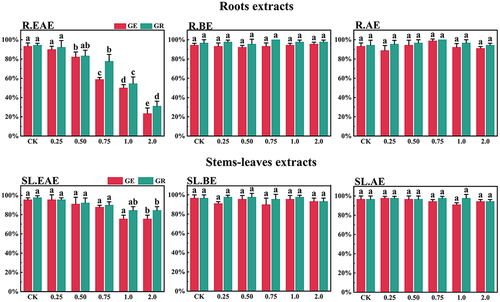
Figure 3. Effect of L. sagitta extracts on seed germination of E. nutans. a: roots ethyl acetate extract (R.EAE), b: roots n-butanol extract (R.BE), c: roots aqueous extract (R.AE), d: stems-leaves ethyl acetate extract (SL.EAE), e: stems-leaves n-butanol extract (SL.BE), f: stems-leaves aqueous extract (SL.AE). In each graph, different letters indicate significant differences between concentration treatments, p < .05. Concentration units for treatment groups are mg/mL. Error bars indicate standard deviation (SD). (GE) germination energy; (GR) germination rate; (CK) control group.
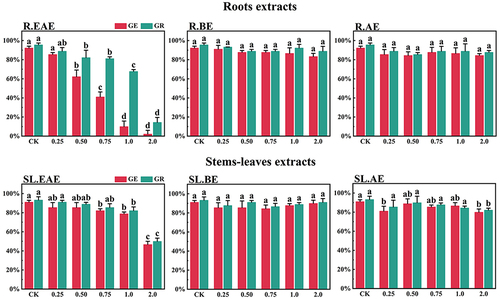
Figure 4. Effect of L. sagitta extracts on seed germination of A. cristatum. a: roots ethyl acetate extract (R.EAE), b: roots n-butanol extract (R.BE), c: roots aqueous extract (R.AE), d: stems-leaves ethyl acetate extract (SL.EAE), e: stems-leaves n-butanol extract (SL.BE), f: stems-leaves aqueous extract (SL.AE). In each graph, different letters indicate significant differences between concentration treatments, p < .05. Concentration units for treatment groups are mg/mL. Error bars indicate standard deviation (SD). (GE) germination energy; (GR) germination rate; (CK) control group.
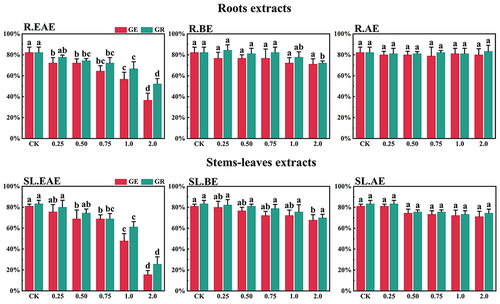
Figure 5. Effects of L. sagitta extracts on seed germination of M. sativa. a: roots ethyl acetate extract (R.EAE), b: roots n-butanol extract (R.BE), c: roots aqueous extract (R.AE), d: stems-leaves ethyl acetate extract (SL.EAE), e: stems-leaves n-butanol extract (SL.BE), f: stems-leaves aqueous extract (SL.AE). In each graph, different letters indicate significant differences between concentration treatments, p < .05. Concentration units for treatment groups are mg/mL. Error bars indicate standard deviation (SD). (GE) germination energy; (GR) germination rate; (CK) control group.
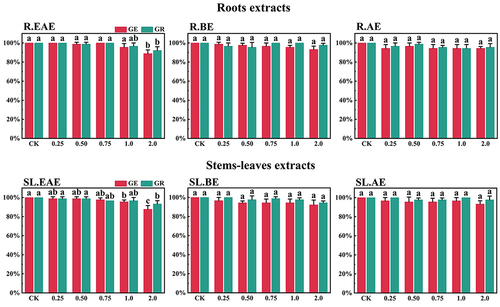
3.1.2. Effects on germination rate of forage seeds
The inhibition effect of L. sagitta extracts on the germination rate (GR) of four Gramineae forage seeds was weaker than that on the germination energy. At 0.75 mg/mL of R.EAE, the germination rate of P. pratensis seeds was 23.3% and it decreased to 0 at 2.0 mg/mL of R.EAE (). Seed germination of E. nutans and F. ovina exhibited similar results, and the inhibition effect was greatest at 2.0 mg/mL of R.EAE. The seed germination rate for E. nutans was 14.4% (), and that for F. ovina was 31.1% (), which was significantly different from the control. The effect of stems-leaves extracts on seed germination of F. ovina was not significant. For A. cristatum, the inhibitory effect of stems-leaves extracts was greater than that of roots extracts (). Germination rate of M. sativa seeds was above 90.0%, which was not significantly different from that of the control, and its germination was not affected by L. sagitta extract ().
3.2. Effects of L. sagitta extracts on the growth of forage seedlings
EAE had the most significant inhibitory effect on shoot length of forage grass seedlings, and severely inhibited shoot length of four Gramineae species at concentrations >0.50 mg/mL; at the highest concentration (2.0 mg/mL), the inhibition of shoot length of P. pratensis was 87.7% (), and that of E. nutans was 87.0% (Table S2). BE and AE significantly inhibited shoot growth of P. pratensis seedlings, but had no significant inhibitory effect on shoot length of E. nutans seedlings. SL.BE and SL.AE did not significantly inhibit shoot length of F. ovina or A. cristatum. All extracts inhibited shoot length of M. sativa seedlings to the same extent, and the degree of inhibition increased with increasing extract concentration. Overall, roots extracts inhibited shoot length more than stems-leaves extracts.
Table 1. Effects of L. sagitta extracts on P. pratensis seedling growth.
Extract treatments significantly reduced root length of five forage seedlings, and root length inhibition rate gradually increased with the increase in extract concentration. At the highest concentration of each phase of the extract (2.0 mg/mL), root length inhibition of P. pratensis seedlings ranged from 69.7 to 100.0% (). F. ovina seedlings exhibited the maximum inhibition rate of 87.2% in the SL.EAE treatment, followed by R.AE with 73.1% (Table S1). EAE had the greatest inhibitory effect on root length of E. nutans and A. cristatum. (S.Table S2, S.Table S3). Results for M. sativa differed from those for the other forages, with the highest inhibition of root length ranging from 63.0 to 84.0 at 2.0 mg/mL of AE (S.Table S4) and roots turning brown in color, and with twisted and distorted morphology after the AE treatment.
Different extracts of L. sagitta varied in the degree of inhibition of fresh weight of the five forages. Similarly, EAE inhibited fresh weight of the forages most, with the greatest inhibition of P. pratensis seedlings. At 2.0 mg/mL, inhibition of fresh weight of A. cristatum seedlings ranged from 65.6 to 72.1% (), followed by E. nutans with inhibition ranging from 66.5 to 66.7%, and lowest inhibition of M. sativa. In addition, R.BE and R.AE significantly inhibited fresh weight of P. pratensis and F. ovina seedlings.
3.3. Overall analysis of UPLC-MS/MS results of L. sagitta extracts
A total of 904 components were identified from L. sagitta stems-leaves and roots extracts, including 154 organic acids, 102 lipids, 102 alkaloids, 86 terpenoids, 82 amino acids and their derivatives, 63 phenols, 63 heterocyclic rings, 56 carbohydrates, 41 flavonoids, 30 esters, 29 nucleotides and their derivatives, 20 coumarins, 14 lignans, 14 quinones, 14 aldehydes, and 34 kinds of other compounds.
3.3.1. PCA and HCA analysis
To gain a preliminary understanding of the overall metabolite differences between the samples in each group (extracts prepared with different solvents) and the magnitude of variability between the samples within the groups, the samples were subjected to principal component analysis (PCA) and hierarchical cluster analysis (HCA). The first two principal components in the PCA () elaborated about 75.3% of total variance (PC1 = 50.8%, PC2 = 24.5%, respectively). The samples within each group were gathered together, and the separation trend between groups was obvious, indicating that there were significant differences in metabolites between stems-leaves and roots and different extraction phases of L. sagitta. HCA () showed that the six extract phases were clustered into two major groups, and SL.EAE and R.EAE were clustered into one group, which was different from BE and AE, while the metabolite types and contents of BE and AE were closer and clustered into another group. The combined results of PCA and HCA indicate that the extracts of different parts and different extraction phases of L. sagitta were significantly different.
Figure 6. A, PCA score plot. b, HCA. Three biological repeat sequences were set up for each extraction phase (indicated by the numbers 1,2,3 at the end of the group name). Roots ethyl acetate extract (R.EAE), roots n-butanol extract (R.BE), roots aqueous extract (R.AE), stems-leaves ethyl acetate extract (SL.EAE), stems-leaves n-butanol extract (SL.BE), stems-leaves aqueous extract (SL.AE).
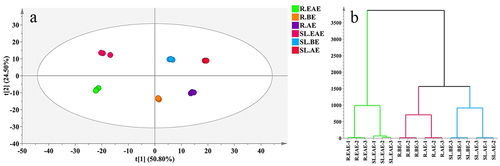
3.3.2. OPLS-DA analysis
The results of the allelopathy tests of the extracts in subsections 3.1 and 3.2 showed that the strongest allelopathic activity in the extract of L. sagitta was EAE. Therefore, by comparing the differences between EAE, and BE, and EAE and AE, potential allelochemicals of L. sagitta were screened. Pairwise comparative was analyzed using OPLS-DA models for the different phases of stems-leaves and roots extracts (R.EAE vs. R.BE; R.EAE vs. R.AE; SL.EAE vs. SL.BE; SL.EAE vs. SL.AE). The OPLS-DA score scatter plots for each group were depicted in . To validate the OPLS-DA model, 200 permutation tests were performed in pairwise comparison groups(Fig. S1). The predictability (Q2) and goodness-of-fit (R2X, R2Y) exceeded 0.9 in all pairwise comparisons (R.EAE vs. R.BE, R2X = 0.958, R2Y = 1, Q2 = 1; R.EAE vs. R.AE, R2X = 0.972, R2Y = 1, Q2 = 1; SL.EAE vs. SL.BE, R2X = 0.954, R2Y = 1, Q2 = 0.999; SL.EAE vs. SL.AE, R2X = 0.970, R2Y = 1, Q2 = 1), indicating that the OPLS-DA model was sufficiently predictable and was not overfitted (). Therefore, it can be used for further screening of potential allelochemicals.The OPLS-DA score scatter plots also showed that the different extraction phases indicated a clear trend toward segregation, which proved that the compounds contained in different extraction phases were quite different.
Figure 7. OPLS-DA score scatter plots. a: R.EAE vs. R.BE; b: R.EAE vs. R.AE; c: SL.EAE vs. SL.BE; d: SL.EAE vs. SL.AE. Roots ethyl acetate extract (R.EAE), roots n-butanol extract (R.BE), roots aqueous extract (R.AE), stems-leaves ethyl acetate extract (SL.EAE), stems-leaves n-butanol extract (SL.BE), stems-leaves aqueous extract (SL.AE). Three biological repeat sequences were set up for each extraction phase.
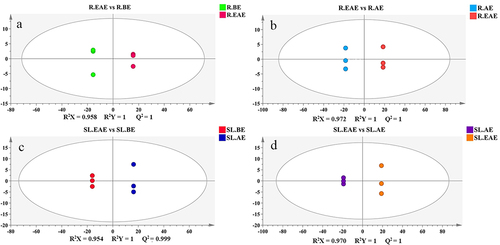
3.3.3. Screening of differential metabolites in different extraction phases
To gain more insight into the differences between R.EAE and R.BE, R.EAE and R.AE, and SL.EAE and SL.BE, and SL.EAE and SL.AE, respectively. Significantly different metabolites were screened by VIP value, p value, and FC (Fold Change) value. p value < .05, FC value ≥ 2 or ≤ 0.5 among the metabolites with a VIP value ≥ 1.5 were used as an identification criterion. A volcano diagram () was used to show the screening results. Differential metabolites of different extracts were shown in Supplementary Table S6–9. There were 31 differential metabolites (19 up-regulated and 12 down-regulated) between R.EAE and R.BE (, Table S5, Table S6), 64 (21 up-regulated and 43 down-regulated) between R.EAE and R.AE (, Table S5, Table S7), 81 (31 up-regulated and 50 down-regulated) between SL.EAE and SL.BE (, Table S5, Table 8), and 66 (27 up-regulated and 39 down-regulated) between SL.EAE and SL.AE (, Table S5, Table S9). Attention worthy, the up-regulated differential metabolites of different extracts of L. sagitta roots were mainly terpenoids, lipids, alkaloids, and organic acids, whereas the up-regulated differential metabolites of different extracts of stems-leaves were mainly terpenoids, organic acids, and flavonoids.
Figure 8. Volcano map of differential metabolites. a: R.EAE vs. R.BE; b: R.EAE vs. R.AE; c: SL.EAE vs. SL.BE; d: SL.EAE vs. SL.AE. Roots ethyl acetate extract (R.EAE), roots n-butanol extract (R.BE), roots aqueous extract (R.AE), stems-leaves ethyl acetate extract (SL.EAE), stems-leaves n-butanol extract (SL.BE), stems-leaves aqueous extract (SL.AE). Red, green and gray points indicate up-regulated, down-regulated and non-significant differential metabolites, respectively. Symbol size indicates VIP-value.
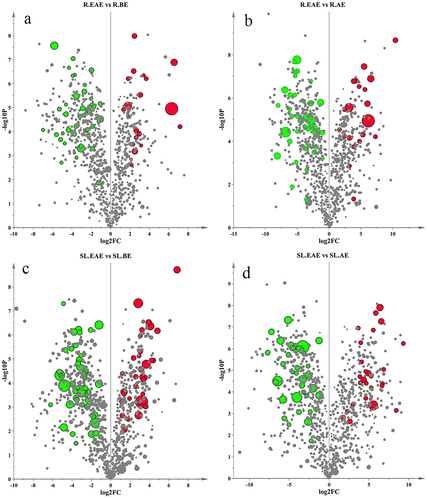
Analysis of the up-regulated compounds in the above four sets of comparisons using Venn diagrams () showed that nine compounds were up-regulated differential metabolites common to R.EAE vs. R.BE, R.EAE vs. R.AE, SL.EAE vs. SL.BE, and SL.EAE vs. SL.AE, which were N,N-Dimethylaniline, Caffeic acid, 2-Phenylethylamine, 4-Hydroxybenzoic acid, Eupatilin, 4-Hydroxybenzaldehyde, cis-9-Octadecenoic, 1-Monolinolein, and Schizandrol A. Their relative contents in different extracts were shown in .
Figure 9. Venn diagram showing the number of overlapping and specific differential metabolites in the four comparison groups (R.EAE vs. R.BE, R.EAE vs. R.AE, SL.EAE vs. SL.BE, SL.EAE vs. SL.AE). Roots ethyl acetate extract (R.EAE), roots n-butanol extract (R.BE), roots aqueous extract (R.AE), stems-leaves ethyl acetate extract (SL.EAE), stems-leaves n-butanol extract (SL.BE), stems-leaves aqueous extract (SL.AE).
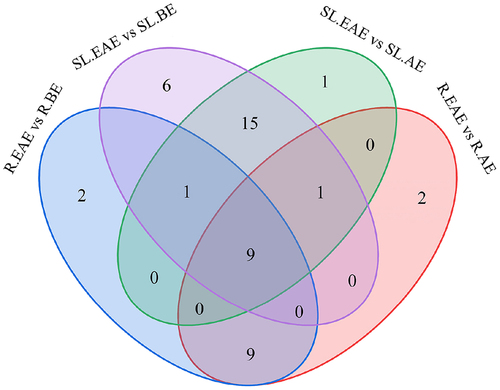
Table 2. Relative content of 9 common differential metabolites.
4. Discussion
A variety of plants invade grasslands through allelopathy mechanisms, in which allelochemicals confer a competitive advantage to the invasive plant.Citation34 Toxic invasive plants can reduce density of the native plant community by decreasing seed germination rate, and reducing access to light, water, and nutrients by inhibiting shoot or root growth in seedlings; this, in turn, affects the distribution pattern and biodiversity of plant populations in the region.Citation35–37
Our experimental results showed that EAE of L. sagitta had a significant inhibitory effect on germination energy, germination rate, shoot length, root length and fresh weight of the four Gramineae forages, and the inhibitory effect showed concentration dependence. Germination energy reflects the homogeneity and vitality of seed germination, and the seed germination rate indicates the amount of seed germination. On the one hand, L. sagitta reduced the germination energy and germination rate of seeds of four Gramineae species and delayed seed germination, which may reduce the abundance of native species in the regionCitation38; On the other hand, L. sagitta also inhibits the root growth of forage seedlings, diminishing nutrient uptake and utilization and depriving forages of early competitiveness.Citation39 This directly affects the status and role of native species in plant communities. The five tested forages showed different sensitivities to the L. sagitta extracts (P. pratensis was the most sensitive and M. sativa was the least sensitive to it), which may be due to the evolutionary differences in the resistance of the test forages to the allelochemicals.Citation15 The strongest inhibitory effect on seed germination and seedling growth of forage was exerted by EAE, indicating that the allelochemicals of L. sagitta are mainly present in EAE. AE and BE had an inhibitory effect on seedling growth only, indicating that allelochemicals related to seedling growth were also present in AE and BE. It has been shown that leaves of plants with allelopathy have greater allelopathic activity than roots, but different results were shown in this study, for example, the inhibitory effect of EAE in roots of L. sagitta on seed germination of P. pratensis, F. ovina, E. nutans was greater than that of EAE in stems-leaves. This also confirms the significant differences in plant allelopathic effects among the extract sources, concentrations and plant species tested. Compared with previous reports,Citation20,Citation37,Citation40 EAE of L. sagitta significantly inhibited seed germination and seedling growth of four Gramineae forage species at very low concentrations.
The present study also revealed that the inhibition by L. sagitta extracts of root length in forage seedlings was much greater than that of shoot length, which may be due to different sensitivity of different plant tissues to allelochemicals.Citation41 Research has shown that rainwater leaching is one of the pathways by which plants release allelochemicals into the environment. Rainwater infiltrates the soil, and the root system becomes directly exposed to allelochemicals; therefore, the root system of most plant seedlings is usually more sensitive to external allelopathic than to other indicators.Citation42,Citation43 The above-ground parts rely on the roots to absorb nutrients to satisfy plant growth needs, therefore, only when the roots are stressed to a certain extent, water and nutrients can not be supplied normally, the other parts of the plant show symptoms of damage.Citation44 We conclude that L. sagitta affects the growth and development of forage mainly by inhibiting the growth of roots of the forage seedlings.
The occurrence of allelopathy can be attributed to a particular compound or class of compounds.Citation34 Allelochemicals are found in large quantities in the secondary metabolites of plants and are mainly categorized into phenols, terpenoids, coumarins, flavonoids, alkaloids, and others.Citation45 The spotted knapweed, an invasive species in the western U.S. prairies, inhibits the emergence and growth of native ground species through root secretions (catechins).Citation46 Stellera chamaejasme L. and Artemisia frigida Willd. are common noxious weeds in the degraded grasslands of northern China. The former inhibits the growth of symbiotic plants mainly through the secretion of flavonoids by the root system,Citation47 while the latter inhibits the germination of seeds and seedling growth of other plants through the release of volatile compounds (mainly terpenoids).Citation48
In this study, 9 compounds were found to be the common up-regulated differential metabolites between R.EAE vs. R.BE, R.EAE vs. R.AE, SL.EAE vs SL.BE, and SL.EAE vs.SL.AE, including 2 aniline derivatives, 2 phenolic acids, 2 fatty acids, 1 aldehydes, 1 flavonoids and 1 lignansand, and were enriched in EAE. The contents of these compounds varied considerably between EAE and BE, EAE and AE. For example, in R.EAE, the N,N dimethylaniline level was 79.24 times higher than that of R.BE and 70.09 times that of R.AE; the caffeic acid level was 3.42 times higher than that of R.BE and 8.87 times that of R.AE; the 4- hydroxybenzaldehyde level was 3.42 times higher than that of R.BE and 8.87 times that of R.AE. Anilines significantly inhibit rice seed germination and root growth and also reduce DNA content in rice seedlings.Citation49 At the same concentration (200 μg/ml), caffeic acid inhibited seed germination and seedling growth of Arabidopsis thaliana stronger than those of the commercial herbicides acetochlor and glyphosate.Citation50 Caffeic acid and 4-hydroxybenzoic acid are considered to be allelochemicals of Bidens pilosa L., which contribute to the invasion of Bidens pilosa L.Citation51 N,N-dimethylaniline,Citation52 caffeic acid,Citation51,Citation53,Citation54 4-hydroxybenzoic acid,Citation51,Citation53,Citation54 4-hydroxybenzaldehyde,Citation54 and cis-9-octadecenoicCitation55 have been demonstrated to be allelochemicals, but eupatilin, 2-phenylethylamine, schisandragenol A, and 1-monolinolein have not yet been reported on their allelopathic activities. Therefore, N,N-dimethylaniline, caffeic acid, 4-hydroxybenzoic acid, 4-hydroxybenzaldehyde and cis-9-octadecenoic acid were potential allelochemicals in L. sagitta.
The present study confirmed the presence of allelopathy in L. sagitta on the tested native species P. pratensis, F. ovina, E. nutans, A. cristatum, and significant inhibition of the growth and development of all these species. This suggests that the ability of L. sagitta to form a dominant species in degraded grassland is aided by its allelopathic mechanism, in addition to its own superb resistance.Citation56 This result can explain the gradual replacement of dominance by Gramineae such as P. pratensis by noxious weed communities such as L. sagitta under continuous overgrazing pressure, and the subsequent loss of the former dominant species from the grassland. Such process forms a pattern dominated by noxious weeds, with a sparse mosaic distribution of Gramineae forages and short growth.Citation57,Citation58
L. sagitta currently exists in alpine grasslands in western Chinese grasslands as a invasive poisonous weeds, impacting on local biodiversity the development of grassland husbandry.Citation59 Continuous mowing and replanting of grasses are effective restoration methods on grassland restoration.Citation4 The continued presence of L. sagitta can impede the ability of native grassland vegetation to recover, and continuous mowing and biomass removal reduces competitive and undesirable species,Citation60 therefore, in the restoration of grasslands degraded by L. sagitta, removing as much as possible of L. sagitta plants. Grassland restoration be accelerated by reseeding native species, and grassland ecosystem functioning and soil quality can be restored.Citation61 However, replanting should be supplemented with forages that have strong resistance to it, such as M. sativa, while selection of P. pratensis and other Gramineae forages that are sensitive to it should be avoided. Local natural conditions and soil texture can also affect the establishment of native grasses. It is necessary to analyze the soil to avoid the residual effect of L. sagitta allelochemicals on the normal growth of plants.Citation62 Further studies should look more closely at the allelochemicals of L. sagitta, their natural concentrations and the mechanism of inhibition.
5. Conclusions
This study adds to a large body of research showing that allelopathy is one of the mechanisms by which invasive plants dominance and that invasive plants can alter plant communities by inhibiting the growth and development of native species. The results of the study showed that allelochemicals were mainly present in the ethyl acetate extracts of stems-leaves and roots of L. sagitta. Based on this study, follow-up work will be carried out to isolate and purify the ethyl acetate extracts to find out the allelochemicals in L. sagitta and further investigate the specific mechanism of allelopathy.
However, this study had some limitations. For example, in the laboratory, climate, temperature, soil fauna, and soil microorganisms were excluded from interacting with and possibly neutralizing allelopathy. Therefore, allelopathy of L. sagitta should be further explored in future studies in natural conditions.
Author contributions
Conceptualization, Kan Jiang and Fang Nian; methodology, Kan Jiang and Fang Nian; data collection, Shengxiao Wang, Chenyue Wang and Jun Zhang; formal analysis, Shengxiao Wang; writing and original draft preparation, Shengxiao Wang; writing and editing, Kan Jiang; supervision, Kan Jiang and Fang Nian. All authors have read and agreed to the published version of this manuscript.
Supplemental Material
Download PDF (775.2 KB)Acknowledgments
This research was funded by the Doctoral Research Foundation Project of Gansu Agricultural University (GAU-KYQD-2019-01).
Disclosure statement
No potential conflict of interest was reported by the author(s).
Supplementary material
Supplemental data for this article can be accessed online at https://doi.org/10.1080/15592324.2024.2335025
Additional information
Funding
References
- Seebens H, Blackburn TM, Dyer EE, Essl F. Global rise in emerging alien species results from increased accessibility of new source pools. Proc Natl Acad Sci U S A. 2018;115(10):E2264–13. doi:10.1073/pnas.1719429115.
- Haeuser E, Dawson W, Van Kleunen M, Catford J. The effects of climate warming and disturbance on the colonization potential of ornamental alien plant species. J Ecol. 2017;105(6):1698–1708. doi:10.1111/1365-2745.12798.
- Hayes SJ, Holzmueller EJ. Relationship between invasive plant species and forest Fauna in Eastern North America. Forests. 2012;3(3):840–852. doi:10.3390/f3030840.
- Świerszcz S, Czarniecka-Wiera M, Szymura TH, Szymura M. From invasive species stand to species-rich grassland: long-term changes in plant species composition during Solidago invaded site restoration. J Environ Manage. 2024;353:120216. doi:10.1016/j.jenvman.2024.120216.
- Kaushik P, Pati PK, Khan ML, Khare PK. Plant functional traits best explain invasive species’ performance within a dynamic ecosystem - a review. Trees For People. 2022;8:100260. doi:10.1016/j.tfp.2022.100260.
- Thorpe A, Thelen G, Diaconu A, Callaway RM. Root exudate is allelopathic in invaded community but not in native community: field evidence for the novel weapons hypothesis. J Ecol. 2009;97(4):641–645. doi:10.1111/j.1365-2745.2009.01520.x.
- Sobuj N, Byun C. A synthesis of plant invasion control: important factors to consider when choosing a control method. Écoscience. 2023;0(0):1–13. doi:10.1080/11956860.2023.2298754.
- Bardgett RD, Bullock JM, Lavorel S, Manning P, Schaffner U, Ostle N, Chomel M, Durigan GL, Fry E, Johnson D. et al. Combatting global grassland degradation. Nat Rev Earth Environ. 2021;2(10):720–735. doi:10.1038/s43017-021-00207-2.
- Hao LF, Han YX, Wu QM, Wang R, Lin KJ. Status of invasive alien species in grassland of China and suggestions for prevention and control. Plant Prot. 2022;48:10–20. doi:10.16688/j.zwbh.2022258.
- Chen BY, Sun YF, Han ZH, Huang HK, Zhang HB, Li YK, Zhang GL, Liu WX. Current situation, problems and countermeasures of prevention and control of alien invasive organisms in China. J Biosaf. 2020;29:157–163. doi:10.3969/j.issn.2095-1787.2020.03.001.
- Lu H, Wang SS, Zhou QW, Zhao YN, Zhao BY. Damage and control of major poisonous plants in the western grasslands of China – a review. Rangeland J. 2012;34(4):329–339. doi:10.1071/RJ12057.
- Kalisz S, Kivlin SN, Bialic-Murphy L. Allelopathy is pervasive in invasive plants. Biol Invasions. 2021;23(2):367–371. doi:10.1007/s10530-020-02383-6.
- Inderjit I, Nilsen ET. Bioassays and field studies for allelopathy in terrestrial plants: progress and problems. Crit Rev Plant Sci. 2003;22(3–4):221–238. doi:10.1080/713610857.
- Mahé I, Chauvel B, Colbach N, Cordeau S, Gfeller A, Reiss A, Moreau D. Deciphering field based evidences for crop allelopathy in weed regulation a review. Agron Sustainable Dev. 2022;42(3):50. doi:10.1007/s13593-021-00749-1.
- Uddin MN, Asaeda T, Shampa SH, Robinson RW. Allelopathy and its coevolutionary implications between native and non-native neighbors of invasive Cynara cardunculus L. Ecol Evol. 2020;10(14):7463–7475. doi:10.1002/ece3.6472.
- He W, Detheridge A, Liu YM, Wang L, Wei HC, Griffith GW, Scullion J, Wei YH. Variation in soil fungal composition associated with the invasion of Stellera chamaejasme L. in Qinghai–Tibet Plateau Grassland. Microorganisms. 2019;7(12):587. doi:10.3390/microorganisms7120587.
- Jin H, Guo HR, Yang XY, Xin AY, Qin B. Effect of allelochemicals, soil enzyme activity and environmental factors from Stellera chamaejasme L. on rhizosphere bacterial communities in the northern Tibetan plateau. Arch Agron Soil Sci. 2022;68(4):547–560. doi:10.1080/03650340.2020.1852549.
- Sun G, Luo P, Wu N, Qiu PF, Gao YH, Chen H, Shi FS. Stellera chamaejasme L. increases soil N availability, turnover rates and microbial biomass in an alpine meadow ecosystem on the eastern Tibetan plateau of China. Soil Biol Biochem. 2009;41(1):86–91. doi:10.1016/j.soilbio.2008.09.022.
- Ma L, Wu HL, Bai R, Zhou L, Hou DB. Phytotoxic effects of stellera chamaejasme L. root extract. Afr J Agric Res. 2011;6:1170–1176. doi:10.5897/AJAR10.732.
- Wang WY, Jia TH, Qi TY, Li SS, Degen AA, Han J, Bai YF, Zhang T, Qi S, Huang M. et al. Root exudates enhanced rhizobacteria complexity and microbial carbon metabolism of toxic plants. IScience. 2022;25(10):105243. doi:10.1016/j.isci.2022.105243.
- Callaway RM, Ridenour WM. Novel weapons: invasive success and the evolution of increased competitive ability. Front Ecol Environ. 2004;2(8):436–443. doi:10.1890/1540-9295(2004)002[0436:NWISAT]2.0.CO;2.
- Editorial Committee of Flora of China, Chinese Academy of Sciences. Flora of China. Beijing: Science Press; 1989; Vol. 77: p. 97.
- Jiangsu College of New Medicine. A dictionary of traditional Chinese medicines. Shanghai: Shanghai Science and Technology Press; 1977. p. 2349.
- Zhang DZ, Yu GD, Zhang M, Wang ZT. Medicinal research of Ligularia. Wild Plant Resour China. 2003;22:4–6.
- Guo YZ, Zhang RH, Sun T, Zhao SJ, You YF, Lu H, Wu CC, Zhao BY. Harm, control and comprehensive utilization of poisonous weeds in natural grassland of Gansu Province. Acta Agrestia Sin. 2017;25:243–256. doi:10.11733/j.issn.1007-0435.2017.02.004.
- Zhao BY, Liu ZY, Wan XP, Huo XH, Guo X, Wang JJ, Sun LS, Shi ZC. Damage and control of Poisonous-Weeds in Chinese Western Grassland. Sci Agric Sin. 2008;41:3094–3103. doi:10.3864/j.issn.0578-1752.2008.10.024.
- Ma RJ, Du GZ, Liu ZJ, Chen JK. Regenerative strategies of three species of Ligularia in eastern Qinghai-Tibet of China I from flowering to germination. Acta Pratac Sin. 2002;11:29–36.
- Shan BQ, Du GZ, Liu ZH. Clonal growth of Ligularia virgaurea: morphological responses to nutritional variation. Acta Phytoecol Sin. 2000;24:46–51.
- Wang L, Gao S, Zhou S, Tang CJ, Hou TP. Investigation of poisonous plants in natural grassland of Qinghai-Tibet Plateau. Acta Bot Boreali-Occident Sin. 2006;26:1428–1435.
- Chen J, Li MM, Xing HJ, Zheng GW, Pu SB. Phytotoxic eremophilane sesquiterpene isolated from Ligularia cymbulifera. Nat Prod Res Dev. 2022;34:570–578. doi:10.16333/j.1001-6880.2022.4.004.
- Ma RJ, Wang ML, Zhu XT, Lu XW, Sun K. Allelopathy and chemical constituents of ligularia virgaurea volatile. Chin J Appl Ecol. 2005;16:1826–1829. doi:10.13287/j.1001-9332.2005.0247.
- Jelassi A, Ayeb-Zakhama AE, Nejma AB, Chaari A, Harzallah-Skhiri F, Jannet HB. Phytochemical composition and allelopathic potential of three Tunisian acacia species. Ind Crops Prod. 2016;83:339–345. doi:10.1016/j.indcrop.2016.01.020.
- Zhu YP, Li YM, Wang ZB, Zhao LP, Zhao FR. Allelopathic effects of root extracts from Medicago sativa on germination of three forage species. Pratac Sci. 2023;40:761–768. doi:10.11829/j.issn.1001-0629.2022-0304.
- Xu Y, Chen X, Ding L, Kong CH. Allelopathy and allelochemicals in grasslands and forests. Forests. 2023;14(3):562. doi:10.3390/f14030562.
- Vilà M, Espinar JL, Hejda M, Hulme PE, Pyek P, Maron JL, Pergl J, Schaffner U, Sun Y, Pyšek P. Ecological impacts of invasive alien plants: a meta-analysis of their effects on species, communities and ecosystems. Ecol Lett. 2011;14(7):702–708. doi:10.1111/j.1461-0248.2011.01628.x.
- Yang H, Song JC, Yu XJ. Artemisia baimaensis allelopathy has a negative effect on the establishment of Elymus nutans artificial grassland in natural grassland. Plant Signaling Behav. 2023;18(1):e2163349. doi:10.1080/15592324.2022.2163349.
- Zhang YJ, Tang SM, Liu KS, Li XF, Huang D, Wang K. The allelopathic effect of Potentilla acaulis on the changes of plant community in grassland, northern China. Ecol Res. 2015;30(1):41–47. doi:10.1007/s11284-014-1203-9.
- Woods MJ, Bauer JT, Schaeffer D, Mcewan RW. Pyrus calleryana extracts reduce germination of native grassland species, suggesting the potential for allelopathic effects during ecological invasion. PeerJ. 2023;11:e15189. doi:10.7717/peerj.15189.
- Choudhary CS, Behera B, Raza MB, Mrunalini K, Bhoi TK, Lal MK, Nongmaithem D, Pradhan S, Song BQ, Das TK. Mechanisms of allelopathic interactions for sustainable weed management. Rhizosphere. 2023;25:100667. doi:10.1016/j.rhisph.2023.100667.
- Fu JT, Zhao X, Wang LY, Zhang YF, Luo YP. Antioxidant activities and allelopathic potential of chonemorpha splendens Chun et tsiang stem methanol extract. Chem Biodiversity. 2022;19(4):e202100973. doi:10.1002/cbdv.202100973.
- Inderjit NA, Duke SO. Ecophysiological aspects of allelopathy. Planta. 2003;217(4):529–539. doi:10.1007/s00425-003-1054-z.
- Mahanta BP, Kemprai P, Bora PK, Lal M, Haldar S. Phytotoxic essential oil from black turmeric (curcuma caesia Roxb.) rhizome: screening, efficacy, chemical basis, uptake and mode of transport. Ind Crops Prod. 2022;180:114788. doi:10.1016/j.indcrop.2022.114788.
- Zhang ZJ, Liu YJ, Yuan L, Weber E, Kleunen MV, Gurevitch J. Effect of allelopathy on plant performance: a meta-analysis. Soil Ecol Lett. 2021;24(2):348–362. doi:10.1111/ele.13627.
- Choopayak C, Aranyakanon K, Prompakdee N, Nangngam P, Kongbangkerd A, Ratanasut K. Effects of Piper betle L. Extract and Allelochemical Eugenol on Rice and associated weeds germination and seedling growth. Plants (Basel). 2022;11(23):3384. doi:10.3390/plants.11233384.
- Cheng F, Cheng ZH. Research progress on the use of plant allelopathy in agriculture and the physiological and ecological mechanisms of allelopathy. Front Plant Sci. 2015;17:1020. doi:10.3389/fpls.2015.01020.
- Bais HP, Vepachedu R, Gilroy S, Callaway RM, Vivanco JM. Allelopathy and exotic plant invasion: from molecules and genes to species interactions. Science. 2003;301(5638):1377–1380. doi:10.1126/science.1083245.
- Yan ZQ, Guo HR, Yang JY, Liu Q, Jin H, Xu R, Cui HY, Qin B. Phytotoxic flavonoids from roots of stellera chamaejasme L. (Thymelaeaceae). Phytochemistry. 2014;106:61–68. doi:10.1016/j.phytochem.2014.07.013.
- Li XF, Wang J, Huang D, Wang LX, Wang K. Allelopathic potential of Artemisia frigida and successional changes of plant communities in the northern China steppe. Plant Soil. 2011;341(1–2):383–398. doi:10.1007/s11104-010-0652-3.
- Tao N, Zhang W, Si L, Zhang RQ, Wang D, Guo CH. Effects of aniline on growth, oxidative and DNA damage of rice (Oryza sativa L.) seedlings. Environ Technol Innovation. 2022;28:102583. doi:10.1016/j.eti.2022.102583.
- Ma J, Lu C, Bai L, Zhang JY, Shen YM. Phytotoxic phenols from the needles of Cedrus deodara. Phytochemistry. 2024;219:113977. doi:10.1016/j.phytochem.2024.113977.
- Kato-Noguchi H, Kurniadie D. The invasive mechanisms of the noxious alien plant species bidens pilosa. Plants (Basel, Switzerland). 2024;13(3):356. doi:10.3390/plants13030356.
- Zhou XX, Yuan SL, Yang L, Xia D, Zhang Y, Fan W. Identification of continuous cropping tobacco root exudates and screening of potential allelopathic substances. J Agric Sci Technol. 2023;XX:1–11. doi:10.13304/j.nykjdb.2023.0034.
- Motalebnejad M, Karimmojeni H, Baldwin TC, Majidi MM. The allelopathic activity of Festuca arundinacea shreb. Rhizospheric soil is exacerbated under drought stress. J Soil Sci Plant Nutr. 2023;23(4):5495–5512. doi:10.1007/s42729-023-01417-x.
- Perveen S, Yousaf M, Zahoor AF, Rasool N, Jabber A. Extraction, isolation, and identification of various environment friendly components from cock’s comb (celosia argentea) leaves for allelopathic potential. Toxicol Environ Chem. 2014;96(10):1523–1534. doi:10.1080/02772248.2015.1031457.
- Nakai S, Yamada S, Hosomi M. Anti-cyanobacterial fatty acids released from myriophyllum spicatum. Hydrobiologia. 2014;543(1):71–78. doi:10.1007/s10750-004-6822-7.
- Ma RJ, Li G, Zhu H, Zhang H, Wang NL. Allelopathic effects of aqueous extracts from Ligularia sagitta on seed of nine pasture plants. Acta Pratac Sin. 2007;16:88–93.
- Li XP, Xu SY, Li MQ, Wang JX, Guo ZJ, Qi YH, Jing ZQ, Li JJ, Xie ZJ. Evolution regularity of degraded alpine vegetation and soil characteristics at different degrees in Gannan Tibetan Autonomous Prefecture. 生态学报. 2022;42(18):7541–7552. doi:10.5846/stxb202204040859.
- Zhou MC. Allelopathic effect of Aconitum Flavum Hand.-mazz on several gram inaceous grass species from Alpine Meadow. China Herbivore Sci. 2013;33:31–36. doi:10.3969/j.issn.2095-3887.2013.05.009.
- Dong R, HUA LM, HUA R, Ye GH, Bao D, Cai XC, Cai B, Zhao XC, Chu B, Tang ZS. Prediction of the potentially suitable areas of Ligularia virgaurea and Ligularia sagitta on the Qinghai–Tibet Plateau based on future climate change using the MaxEnt model. Front Plant Sci. 2023;14. doi:10.3389/fpls.2023.1193690.
- Leps J. Scale- and time-dependent effects of fertilization, mowing and dominant removal on a grassland community during a 15-year experiment. J Appl Ecol. 2014;51(4):978–987. doi:10.1111/1365-2664.12255.
- Wang B, Liu J, Li ZG, Morreale SJ, Schneider RL, Xu DM, Lin XK. The contributions of root morphological characteristics and soil property to soil infiltration in a reseeded desert steppe. Catena. 2023;225:107020. doi:10.1016/j.catena.2023.107020.
- Humphries T, Florentine SK, Dowling K, Turville C, Sinclair S. Weed management for landscape scale restoration of global temperate grasslands: a review. Land Degrad Dev. 2021;32(3):1090–1102. doi:10.1002/ldr.3802.