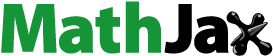
ABSTRACT
β-cyclodextrin butenate was synthesized by using N, N’-Carbonyldiimidazole (CDI) activating reagent and 4-Dimethylaminopyridine (DMAP) as catalyst. The best preparation condition of β-CD butenate was described as below: reaction temperature was 25°C, concentration of 2-butenoic acid was 450 mmol/L, concentration of DMAP was 12.5 mmol/L and reaction time was 20 minutes and at this condition the yield of β-CD butenate was 0.83 mmol/g. According to the results of FT-IR spectrum, NMR spectroscopy and HPLC-QTof-mass spectrum of β-CD butenate, there were four types β-CD butenate synthesized, which were β-CD-2-butenoic acid monoester, β-CD-2-butenoic acid diester, β-CD-2-butenoic acid triester and β-CD-2-butenoic acid tetraester, respectively.
1. Introduction
β-cyclodextrin (β-CD) is a cyclic oligosaccharide and it is produced from starch by using glucosyltransferase of Bacillus [Citation1,Citation2]. There are many active hydroxyl groups on the ringlike surface of β-cyclodextrin [Citation3,Citation4], which can react with a variety of chemical reagents and introduce new chemical groups at the hydroxyl position to form a series of chemical derivatives of β-cyclodextrin [Citation5]. β-cyclodextrin derivatives containing vinyl groups are one important precondition for the preparation of β-cyclodextrin polymers [Citation6,Citation7,Citation8]. For example, β-cyclodextrin maleate was used to prepare β-cyclodextrin-acrylamide (CDM-AM) copolymer by using K2S2O8 as initiator and irradiation method, respectively [Citation9,Citation10]. In our former research, we found out that phosphates, phosphites and polyphosphates could be used as catalyst for the esterification of binary unsaturated organic acids, such as maleic acid and itaconic acid, with β-cyclodextrin under semi-dry conditions [Citation11]. However, this reaction needs high temperature and pressure condition. So, finding another esterification method at room temperature is very important to the β-cyclodextrin derivatives with unsaturated double bonds.
N, N’-Carbonyldiimidazole (CDI) is a green activating reagent, which has a much lower toxicity in contrast to other activating reagent, such as paratoluensulfonyl chloride and thionyl chloride, and only carbon dioxide and imidazole are released as removable by-products [Citation11]. CDI is wildly used in the synthesis of esters and amide compounds. β-cyclodextrin butyric ester was synthesized by using CDI as activating reagent [Citation12]. Esterification at O-2 of β-cyclodextrin was developed by using the combination of CDI and carbonate buffer in 1,4-dioxane [Citation13]. CDI is also used in the esterification of polysaccharide, such as starch. Corn starch ferulates was synthesized by the activation of ferulic acid mediated by CDI [Citation14]. 4-dimethylaminopyridine (DMAP) is a common catalyst used in the synthesis of ester derivatives [Citation15]. In this research, CDI was used as activating reagent of 2-butenoic acid, and DMAP was used as catalyst of esterification.
β-cyclodextrin has seven glucose residues and each primary hydroxyl of glucose residues had the potential to react with organic acids. In this research, the effects of reaction time, acid dosage, catalyst dosage and reaction temperature on the synthesis of β -cyclodextrin butenate were investigated, and its structure was characterized by IR spectra and NMR spectra. At the same time, TOF mass spectrometry was used to identify the different type of β-cyclodextrin butenate. This study provided a new method for the synthesis of β-cyclodextrin derivatives containing vinyl group.
2. Materials and methods
2.1. Materials
β-cyclodextrin was purchased from Aladdin Co. Ltd. (Shanghai,China). 2-butenoic acid (99%, HPLC grade), dimethylformamide (DMF, 99%, HPLC grade), N, N’-Carbonyldiimidazole (CDI, AR grade) and 4-Dimethylaminopyridine (DMAP, AR grade) were from Sinopharm Co. Ltd (Shanghai, China). Methyl butenate was from Sigma (St. Louis, MO, U.S.A). All other reagents were of analytical grade and used as received.
2.2. Synthesis and purification of β-cyclodextrin butenate
β-cyclodextrin was dehydrated in oven at 100℃ for 24 hours before use. Calcium hydride was added to dehydrate N, N-dimethylformamide (DMF) before use to ensure that the reaction system was waterless. Four single factors including catalyst concentration, 2-butenoic acid concentration, reaction time and reaction temperature were investigated for sample preparation. The final concentration of β-cyclodextrin in the sample preparation was constant at 50 mmol/L. Cyclodextrin (5 mmol), a certain amount of 2-butenoic acid (5–45 mmol) and 4-dimethylaminopyridine(DMAP)were weighed in a triangle flask, and 50 mL DMF was added to dissolve the mixture until used. A certain amount of N, N-carbonyl diimidazole (CDI, 5–45 mmol) was weighed and dissolved by adding 50 mL of DMF. The solution of CDI was added into the aforementioned triangular flask and stirred by magnetic force at a certain reaction temperature (20–60°C) for a period of time (2–120 min). Total volume of DMF was 100 mL. After the reaction, 30 mL ultrapure water was added to stop the reaction. Each single-factor experiment was repeated 3 times. After the reaction, the mixed solution was rotated and evaporated at 60°C under the pressure of 5 mbar. Then 100 mL anhydrous ethanol was added to the rotary steaming bottle to precipitate the crude product. Crude product was added into the mixing cup and stirred thoroughly with 50 mL anhydrous ethanol; after stirring, the precipitates were collected by suction filtration, and washing operation was repeated 5 times. The above washed precipitates were transferred to empty SPE columns for vacuum filtration, and 100 mL anhydrous ethanol was added into the SPE columns. Prepared β-CD butenate sample were dried at 55℃.
2.3 Unsaturated ester bond amount of β-CD butenate
Methyl butenate was used as a reference, and its methanol solutions with concentrations of 0.1, 0.3, 0.5, 0.7, 1.0 and 3.0 mmol/L were prepared, respectively. The peak area of methyl butenate was measured by HPLC at 210 nm wavelength. The standard curve was taken the peak area as the ordinate and the molar concentration as the abscissa, and standard calibration curve was y = 3204.1× (R2 = 0.9999). 0.5 g of purified β-CD butenate was dissolved in 25 mL of 30% acetonitrile aqueous solution, and then the solution was further diluted 10 times for test on HPLC (Agilent Technologies Inc., US, 1260). Chromatographic column was used Kromasil 5 u C18 (100 A, 250 × 4.6 mm); Mobile phase A was acetonitrile (55%) and mobile phase B was 0.1% formic acid aqueous solution (45%); flow rate was 1.0 mL/min, injection volume was 10 μL and measure wavelength was 210 nm. The unsaturated ester bond amount of β-CD butenate was calculated by EquationEquation (1)(1)
(1) .
Where Au was the Unsaturated ester bond amount of β-CD butenate; As was the total peak area of β-cyclodextrin butenate, Ws was the weight of purified β-cyclodextrin butenate, Vs was solution volume of the sample, Dr was dilution ratio of sample.
2.4 Characteristic of β-cyclodextrin butenate
2.4.1 FT-IR Spectra
ATR-FTIR spectra were obtained using an infrared spectrometer Spectrum 400 (Perkin Elmer Co. United States) for testing. ATR accessories contain diamond crystal internal reflection components, infrared beam incidence angle was 45°. The spectral resolution was 4 cm−1, and the spectrum performed a total of 16 background and sample scans [Citation16,Citation17]. Each sample was approximately 10 mg and scanned immediately after addition. Make sure there are no air bubbles on the sample surface and place a small piece of aluminum foil over the sample. At the end of sampling, ATR accessory crystals were washed with 75% (v/v) alcohol, and the background scan was re-performed before the next sample profile was collected. Each sample was repeated three times, and its mean value was taken for mapping using Origin 2018 data processing software.
2.4.2 1H and 13C NMR spectra
The NMR spectra of β-CD butenate and 2-butenoic acid were determined using an NMR spectrometer (AVANCE III 500 MHz, Bruker, Switzerland). All samples were dissolved in heavy water (D2O) at an experimental temperature of 25°C.
2.4.3 HPLC-QTof- mass spectrometry
The mass spectrometric information for β-CD butenate was determined by using a HPLC-QTof mass spectrometer (Xevo G2-S, Waters Inc., U.S.A) and High-energy ion fragment information of different type of β-CD butenate was also detected. 2, 5-dihydroxybenzoic acid (DHB) was used as substrate. Mobile phase A was acetonitrile (55%) and mobile phase B was 0.1% formic acid aqueous solution (45%), and flow rate was 0.45 mL/min.
3. Results and discussion
3.1 The effect factors of the synthesis of β-CD butenate
Through the HPLC spectrum, at least two kinds of β-CD butenate were synthesized, which retention time was 1.592 min and 1.779 min as shown in , respectively, and according to the HPLC-QTof- mass spectrometry results, there were four types of β-CD butenate, which were β-CD-2-butenoic acid monoester, β-CD-2-butenoic acid diester, β-CD-2-butenoic acid triester and β-CD- 2-butenoic acid tetraester. However, at the same chromatographic conditions, the retention time of butanoic acid and methyl butenate was 2.389 min and 3.012 min, respectively. This was also a provement of the synthesis of β-CD butenate, because only butanoic acids and butenates containing vinyl group had ultraviolet absorption at 210 nm wave length. Meanwhile, 2-butenoic acid peak (2.389 min) was not found in the HPLC spectrum of β-CD butenate, which indicated that the purification method was reasonable.
Through the single factor experiment, concentration of butenic acid, concentration of DMAP and reaction time were the major factors influenced the synthesis of β-CD butenate (). The reaction temperature was seemed has no significant influence on the esterification of β-CD, although the unsaturated ester bond amount of β-CD butenate was slightly decreased when the reaction temperature was over 45°C. The esterification was finished after 20 minutes, and unsaturated ester bond amount of β-CD butenate was the highest, which were 0.83 mmol/g, when amount of catalyst (DMAP) was 12.5 mmol/L and concentration of 2-butenoic acid was 450 mmol/L.
Figure 2. The effect factors of the synthesis of β-CD butenate. a: Reaction temperature (β-CD, 15 mmol; 2-butenoic acid, 25 mmol; CDI, 25 mmol; DMAP 1.25 mmol; temperature 25, 35, 45, 55 and 65°C; reaction time 60 min); b: concentration of butenic acid (β-CD, 5 mmol; 2-butenoic acid, 5, 15, 25, 35 and 45 mmol; CDI, 5, 15, 25, 35 and 45 mmol; DMAP, 1.25 mmol; temperature, 25°C; reaction time 60 min); c: concentration of DMAP (β-CD, 5 mmol; 2-butenoic acid, 25 mmol; CDI, 25 mmol; DMAP 0.025, 0.25, 1.25, 1.875, 2.5, 6.25 and 12.5 mmol; temperature, 25°C; reaction time 60 min); d: Reaction time (β-CD, 5 mmol; 2-butenoic acid, 45 mmol; CDI, 45 mmol; DMAP, 1.25 mmol; temperature, 25°C; reaction time, 2–120 min).

3.2 Characteristic information of β-CD butenate
3.2.1 FT-IR spectrum
In the infrared spectrum of β-cyclodextrin (), the stretching vibration of hydroxyl group (−OH) in cyclodextrin was observed at 3310 cm−1, the variable angle vibration frequency of methylene group (-CH2) were appeared at 1409 cm−1, and the variable angle vibration frequency of methine group (−CH) were appeared at 1333 cm−1. The stretching vibration of C-OH group and C-O-C group in cyclodextrin was observed at 1150–1000 cm−1, and the symmetric stretching vibration of C-O-C group was observed at 944 cm−1. The above characteristic absorption was also observed in β-cyclodextrin butenate, which indicated that the intact ring structure of β-cyclodextrin was preserved in the process of esterification. In the infrared spectrum of 2-butenoic acid (), C=O stretching vibration appeared at 1683 cm−1, C=C stretching vibration appeared at 1650 cm−1, in-plane bending vibration of COH group was appeared at 1421 cm−1, and its stretching vibration was appeared at 1314 cm−1 and 1223 cm−1. In β-cyclodextrin butenate (), the characteristic frequency of 2-butenoic acid were not found, and the stretching vibration of C=O in unsaturated carbonyl ester were found at 1738 cm−1 [Citation18,Citation19]. This is because when the double bond was connected with carbonyl ester group, the conjugation effect makes the carbonyl vibration frequency move to low frequency. At the same time, the stretching vibration of unsaturated double bond C=C (1650 cm−1) was retained inβ-cyclodextrin butenate, but its intensity was significantly reduced. At the same time, the stretching vibration frequency of C-O in the ester bond was observed at 1253 cm−1 [Citation20]. The synthesis of β-cyclodextrin butenate could be proved by comparing the infrared spectra of β-cyclodextrin, 2-butenoic acid and β-cyclodextrin butenate.
3.2.2 1H and 13C NMR Spectroscopy of β-CD butenate
In 1H NMR spectrum of β-CD butenate, the chemical shift of hydrogen in vinyl group (-C=C-) was found at 4.95 and 5.10 ppm [Citation21]; however, in 1H NMR spectrum of butenic acid, the chemical shift of hydrogen in vinyl group (-C=C-) were at 4.71 and 5.75 ppm, and 1H signals for β-CD were found at 4.75 to 5.22 ppm [Citation22], as shown in . In 13C NMR spectrum of β-CD butenate, the chemical shift of 13C in vinyl group (-C=C-) was at 128.35 and 156.09 ppm [Citation23] and chemical shift of 13C in unsaturated ester group was found at 136.90 ppm; however, in 13C NMR of butenic acid, the chemical shift of 13C in vinyl group (-C=C-) were at 121.29 and 150.48 ppm. Meanwhile, the chemical shifts of 13C in β-CD group were found at 60.30, 71.56, 72.09, 73.95, 82.65 and 101.34 ppm, as shown in . Those results were also approved the synthesis of β-CD butenate.
3.3.3 Mass spectrum resolution of β-CD butenate
Four types of β-CD butenate were synthesized, which were β-CD-2-butenoic acid monoester, β-CD-2-butenoic acid diester, β-CD-2-butenoic acid triester and β-CD- 2-butenoic acid tetraester. At most four butenic acids can be esterified with the primary hydroxyl group of β-cyclodextrin under the synthesis conditions described in this paper. Mass spectrometric analysis and high energy ion fragmentation of β-CD-2-butenoic acid diester was shown in , and mass spectrometric analysis and high energy ion fragmentation of β-CD, β-CD-2-butenoic acid monoester, β-CD-2-butenoic acid triester and β-CD- 2-butenoic acid tetraester were shown in supplementary data. There were 6 specific fragments were found in the mass spectrum of β-CD, which were C12H17O11, C17H23O15, C24H34O18, C32H57O26, C34H52O22 and C38H67O31 as shown in .
Table 1. High energy ion fragmentation of β-CD-2-butenoic acid diester.
Besides 6 fragments existed in β-CD, there were 6 specific ion fragments were found in the mass spectrum of β-CD-2-butenoic acid diester, which were C47H73O37, C47H69O37, C19H33O11, C31H48O20, C44H69O35 and C34H53O22. The formation of C47H73O37 fragments could prove the synthesis of β-CD-2-butenoic acid diester, which is formed by losing one allyic group from β-CD-2-butenoic acid diester (C50H78O37), and the generation pathway of other 5 ion fragments was shown in . The detection of fragments existed in β-CD were also indicated that the ring structure of β-cyclodextrin were preserved after esterification. For β-CD-2-butenoic acid monoester, there were 3 specific ion fragments were detected, which were C44H69O35, C31H47O21 and C19H33O11, and other 7 fragments were existed in β-CD. For β-CD-2-butenoic acid triester, there were 7 specific ion fragments were found, which were C54H79O34, C51H75O34, C51H69O34, C47H69O35, C44H69O35, C31H48O20 and C19H33O11. For β-CD-2-butenoic acid tetraester, there were also 7 specific ion fragments were found, which were C57H83O36, C54H77O36, C51H69O34, C47H73O37, C34H48O20, C31H48O20 and C19H33O11, and one fragments existed in β-CD was found, which were C12H17O11. C12H17O11 fragment was found in all kinds β-CD butenate.
4. Conclusion
β-cyclodextrin (β-CD) derivatives containing vinyl were the foundation of preparing β-cyclodextrin polymer. In this research, N, N’-Carbonyldiimidazole (CDI) was used as carboxylic acid activator and 4-dimethylaminopyridine (DMAP) was used as catalyst for the synthesis of β-CD butenate. The preparation condition of β-CD butenate was described as below: reaction temperature was 25°C, concentration of 2-butenoic acid was 450 mmol/L, concentration of DMAP was 12.5 mmol/L and reaction time were 20 minutes; at this condition the yield of β-CD butenate was 0.83 mmol/g. The esterification rate of this method was higher than it using phosphates, phosphites and polyphosphates as catalyst. The FI-IR spectrum and NMR spectrum of β-CD butenate were proved the esterification of β-CD with butenic acid. According to the mass spectrum resolution of β-CD butenate, there were four types β-CD butenate synthesized, which were β-CD-2-butenoic acid monoester, β-CD-2-butenoic acid diester, β-CD-2-butenoic acid triester and β-CD- 2-butenoic acid tetraester, respectively.
Acknowledgments
This work was funded by the Science and technology fund of Guizhou (Grant No. [2018]1420 and the Science and technology fund of Guizhou (Grant No. [2020]1Z029).
Disclosure statement
There are no conflicts of interest with other people or organizations within 3 years of beginning the work submitted that could inappropriately influence the work submitted.
Additional information
Funding
References
- Ashish K, Diksha C, Amisha V. β-cyclodextrin and its derivatives: application in wastewater treatment. Environ Sci Pollut Res. 2022;29(2):1585–1604.
- Buschmann HJ, Knittel D, Schollmeyer E. New textile applications of cyclodextrins. J Inclusion Phenom Macrocyclic Chem. 2001;40(3):169–172.
- Anghel N, Melinte V, Spiridon I, et al. Antioxidant, antimicrobial, and kinetic studies of Β-cyclodextrin crosslinked with lignin for drug delivery. Pharmaceutics. 2022;14(11):2260. DOI:10.3390/pharmaceutics14112260
- Loftsson T, E ME. Pharmaceutical applications of cyclodextrins: drug solubilization and stabilization. J Pharmaceut sci. 1996;85(10):1017–1025.
- Qiang-Fang H, Hui L, Jiang L. A β-cyclodextrin-containing polymeric salicylidene Schiff base: synthesis, zinc ion coordination and fluorescence resonance energy transfer with protein. Polym Chem. 2013;4(5):1557–1564.
- Jing-Fen H, Gereltu B, Ru-Ke B, et al. Synthesis and anti-hyperlipidemic activity of a novel starch piperinic ester. Carbohydr Polym. 2008;71(3):441–447. DOI:10.1016/j.carbpol.2007.06.014
- Grégorio C, Ludovic J, Michel M, et al. Macroporous polyamines containing cyclodextrin: synthesis, characterization, and sorption properties. J Appl Polym Sci. 2015;69(7):1419–1427. DOI:10.1002/(SICI)1097-4628(19980815)69:7<1419:AID-APP17>3.0.CO;2-O
- Qi-Kun W, Lan B, Xiao-Mei Q, et al. Contrastive study on β-cyclodextrin polymers resulted from different cavity-modifying molecules as efficient bi-functional adsorbents. React Funct Polym. 2020;154(9):104686. DOI:10.1016/j.reactfunctpolym.2020.104686
- Yong-Fu L, Yi-Ming H, Qin G, et al. Synthesis of two beta-cyclodextrin derivatives containing a vinyl group. Carbohydr Res. 2015;404:55–62.
- Yong-Fu L, Hong-Tao T, Si-Jing Z. The synthesis of water-soluble CDM-AM copolymer by irradiation and its solubilization effect on hydrophobic drugs. Des Monomers Polym. 2018;21(1):105–115.
- Yong-Fu L, Jing J, Qin G, et al. Complexing natamycin and carbendazim with β-Cyclodextrin-acrylamide copolymer to improve its solubility and fungicidal activity. Carbohydr Polym. 2015;125:288–300.
- Lorna D, Stephen F, Lincoln P, et al. Reversal of Regioselectivity and Enhancement of Rates of Nitrile Oxide Cycloadditions through Transient Attachment of Dipolarophiles to Cyclodextrins. Chem A Euro J. 2006;12(33):8571–8580. DOI:10.1002/chem.200600627
- Zhi-Zhong W, Xuan-Ping M, Xiao-Gang W. Direct Regioselective Esterification at O-2 of β-Cyclodextrin and Hydrolysis by Neighboring-group Participation. E J Chem. 2012;9(3):1562–1568.
- Yu W, Fa-Yin Y, Jian-Fei Z, et al. Corn starch ferulates with antioxidant properties prepared by N, N’-carbonyldiimidazole-mediated grafting procedure. Food Chem. 2016;208:1–9.
- Bo F, Yi-Yi L, Jing W, et al. Polymerization-Induced Self-Assembly (PISA) and “Host–guest” Complexation-Directed Polymer/Gold Nanocomposites. ACS Mater Lett. 2020;2(5):492–498. DOI:10.1021/acsmaterialslett.0c00043
- Trirat N, Molin W, Chirapond C, et al. Attenuated Total Reflection-Fourier Transform Infrared Spectroscopy (ATR-FTIR) combined with chemometric modelling for the classification of clinically relevant Enterococci. J Appl Microbiol. 2021;130(3):982–993. DOI:10.1111/jam.14820
- Yuan-Yuan W, Jie-Qing L, Hong-Gao L, et al. Attenuated Total Reflection-Fourier Transform Infrared Spectroscopy (ATR-FTIR) Combined with Chemometrics Methods for the Classification of Lingzhi Species. Molecules. 2019;24(12):2210. Mole.
- Kumari L, Sinha L, Prasad O, et al. Study of molecular association in binary mixtures of poly (vinyl pyrrolidone) (PVP) with ethanol, 1-propanol and 1-butanol through thermo-acoustical, FT-IR, UV–Vis spectroscopy and DFT studies. Eur Phys J D. 2021;75(11):1–11. DOI:10.1140/epjd/s10053-021-00299-x
- Nanda AK, Ganesh K, Kishore K, et al. End-group analysis of vinyl polyperoxides by maldi-tof-ms, FT-IR technique and thermochemical calculations. Polymer. 2000;41(26):9063–9072. DOI:10.1016/S0032-3861(00)00112-9
- Ning YC. Structure Identification and Organic Spectroscopy of Organic Compounds. Beijing: Sci Pre; 2015. M
- Feng-Jiao L, Xing-Rong Z, Xue-Jun L, et al. Synthesis and characterization of polyphenylsilsesquioxane terminated with methyl and vinyl groups low-melting glass. J Adhes Sci Technol. 2017;31(22):2399–2409. DOI:10.1080/01694243.2017.1302699
- Luc C, Annabelle G, Cecile V, et al. Physicochemical Characterization of α-, β-, and γ-Cyclodextrins Bioesterified with Decanoate Chains Used as Building Blocks of Colloidal Nanoparticles. Biomacromolecules. 2011;12(8):3031–3038. DOI:10.1021/bm2006664
- Suror AM. Synthesis and Characterization of New Polymers Derivatives from Copoly (Vinyl Chloride - Vinyl Alcohol). Orient J Chem. 2017;33(5):2329–2333.