ABSTRACT
Ordinary polymers have poor adaptability in high-temperature and high-salt reservoir environments due to their properties. Organic/inorganic composite copolymer microspheres have the advantages of both of them, which are expected to break through their applicability limitations in such oil reservoirs. Therefore, its preparation and performance have always been of great concern to researchers. In this paper, AM/AMPS/Si-St ternary copolymers were synthesized by precipitation polymerization; then modified nano-silica particles were added to synthesize AM/AMPS/Si-St/g-SiO2 organic/inorganic composite quaternary copolymers. FT-IR and SEM characterized the copolymers to confirm that they were prepared successfully. Experiments were carried out to investigate the concentration and ratio of monomers, which showed that the Weissenberg effect could be avoided. The number of polymer molecules could be stabilized under AM concentration of 12 wt%, AM/AMPS/Si-St ratio of 8:1:1, nano silica of 3.3% and the modification conditions of KH570:SiO2 = 1:1. The experiments of temperature and salt resistance of two copolymers were evaluated and compared were conducted by using viscosity and particle size as parameters. The results showed that quaternary copolymers could increase the viscosity retention rate by about 10% compared with ternary copolymers under high content of Na+ and Mg2+. When the two copolymers were placed at 150°C, the appearance and morphology of the terpolymer changed obviously. Through the SEM image of the quaternary copolymers, it could be seen that although the spherical shape of the microsphere had been gradually lost, no degradation occurred, and the stable time of the modified microspheres had been effectively extended.
Introduction
Polyacrylamide-based polymer microspheres are one of the most widely used materials in the oil and gas field, with the advantages of high-swelling ratio, good elasticity, and strong designability. However, they are easy to deposit and decompose under high temperatures and high salt conditions. On the one hand, copolymers with polyacrylamide (PAM) as the main monomer hydrolysis and degradation speed at high temperature [Citation1–6]. On the other hand, the carboxyl groups in the molecules are very sensitive to salts. Under high salinity, the molecular chains will curl up severely at high mineralization, which has an irreversible impact on the viscosity. Therefore, the application of polyacrylamide polymers in high-temperature and high-salt reservoirs has certain limitations [Citation7–11]. In response to such problems, domestic and foreign researchers have proposed several ideas to improve the temperature and salt resistance [Citation12–17]: (1) Addition of salt-resistant groups. 2-acrylamido-2-methylpropane sulfonic acid (AMPS) was the main component. (2) Addition of hydrophobic groups. Hydrophobic associative polymers can increase the rigidity of the original polyacrylamide chain and inhibit the hydrolysis of the amide groups. Hydrophobic associative polyacrylamides (SHPAM) functionalized with sulfonylcalix [Citation4] aromatics by redox radical polymerization were prepared by Du et al. [Citation18], which had good long-term stability, water solubility, salt, and temperature resistance. (3) Addition of amphoteric ions. The fluid mechanic volume of zwitterion polymer itself will be greatly increased by the rich salt ions contained in the oil layer. A novel amphoteric polyacrylamide (PASD) using two anion monomers was synthesized by Jin et al. [Citation19], which also exhibited excellent heat resistance and shear resistance in concentrated salt solutions.
Although there are many means for improving the temperature and salt resistance of polyacrylamide-based polymer microspheres, there are relatively few studies on new organic/inorganic composite copolymers formed by adding inorganic materials on the basis of the original polyacrylamide copolymers [Citation20–27]. The EOR technology currently is not very optimistic for the high temperature (90°C −150°C), high salt (20,000–30,000 mg/L), and low permeability of the oil field, which the water cut is going to rise fast. Therefore, combined with the current development status and existing problems, instrument detection, chemical analysis and reservoir theory as guidance and physical simulation experiment as technical means [Citation28–33], the precipitation polymerization was used to prepare two kinds of copolymers, namely AM/AMPS/Si-St and AM/AMPS/Si-St/g-SiO2. A comprehensive evaluation of them was conducted to explore further the mechanism of inorganic materials improving the temperature and salt resistance of polyacrylamide. This study had expected to provide a structural theoretical basis for the subsequent construction of novel polyacrylamide-based polymers with superior performance.
Method
Materials
AM, AMPS, Si-St, acrylate-based crosslinking agent, water-soluble dispersants, ammonium sulfate, azo initiators, silane coupling agent (KH570) and nano silica. Deionized water was self-made in the laboratory. Water used in the experiment: deionized water and ionized water with two different levels of salinity. Quality analysis is shown in .
Table 1. Water composition analysis.
Instruments
The experimental equipment used is shown in .
Table 2. Equipments.
Preparation of copolymers
The dispersant was fully dissolved in water. AM, AMPS, Si St, ammonium sulfate, and crosslinking agent were added. pH was adjusted to 6.5 by NaOH under ice bath conditions, and an initiator was added. Low-temperature nitrogen and deoxygenation were performed on the mixture. Afterwards, precipitation, washing, and drying were carried out to obtain a powdered ternary copolymer.
Nano-silica particles were dissolved in anhydrous ethanol and fully dispersed. KH570 were placed in the H2O: anhydrous ethanol system (1:9) and stirred evenly. pH was adjusted to weakly alkaline, refluxed at 80 ℃ for 4 hours, filtered, and dried before extracting g-SiO2.
g-SiO2 particles were dissolved in deionized water and sonicated for 30 minutes to be emulsion. Repeated the preparation of ternary copolymers in this emulsion to obtain quaternary composite copolymers. The synthesis process is shown in .
Microscopic morphology and structural characterization
The copolymer powders were spread on the conductive adhesive and sprayed with gold, which were observed by Scanning Electron Microscope. The resolution of the Fourier transform infrared (FT-IR) spectrometer was 4 cm−1, and the scanning was performed 32 times. Copolymers were put and ground in a grinding dish under infrared light irradiation and then were pressed into a thin sheet for testing.
Monomer concentration and ratio experiments
The terpolymers were synthesized under the conditions of AMPS/Si-St both of 0.6 wt% and AM of 10%-18 wt%. The state and viscosity of solutions were observed; KH570:SiO2 were set to be 3:1/2:1/1:1/1:2/1:3, and modified nano-silica was 0.1/0.15/0.2/0.25/0.3 g, respectively, and the viscosity of the composite copolymers was measured.
Salt resistance tests
Salt solutions of two copolymers were prepared with two kinds of mineralized (12810/34691 mg/L) water as well as under the condition that Na+ and Mg2+ contents were 2000–10000 mg/L. Viscosity and particle size were measured after one day.
Temperature resistance tests
Solutions of the two copolymers were prepared at 5000 mg/L, which were poured into a reactor and placed at a 150°C constant temperature oven. The macroscopic changes were observed on days 0, 1, 7, and 14. When the visible changes were not obvious, particle size testing was taken further.
Viscosity and particle size testing
The apparent viscosity of the samples was determined using a Brinell viscometer. The sample was diluted with deionized water (1:100 mass ratio of sample to deionized water) and added to the cuvette. The copolymer volumetric average particle size was measured under the condition that the addition amount meets the need of making the shade 10–20%.
Results
Morphological and structural characterization
As shown in , by comparing the IR curve of SiO2, the characteristic peaks of methyl and methylene groups at around 2959 cm−1, C=O at around 1706 cm−1, and stretching vibration peaks at C=C at 1631 cm−1 were observed for SiO2 and g-SiO2 functional groups, which KH570 was grafted onto the surface of SiO2. FT-IR curves of g-SiO2/PAM compared to SiO2/PAM, the stretching vibration peaks of C=O and C=C appeared at 1729 cm−1 and 1662 cm−1, indicating the binding force between AM and the carrier g-SiO2 surface, which further confirmed the successful preparation of the quaternary composite copolymer.
As shown in , the modified silica particles were around 100 nm in size. The size distribution of the terpolymers and quaternary polymers was 2–4 μm and 3–5 μm with good dispersibility. The increase in size indicated that silica particles bridged the main chains of the copolymers, which interconnected and entangled the molecular chains until the end of the nucleation process.
Effect of monomer concentration and ratio
Similar synthetic experiments were carried out with the varied concentration of AM (10–18 wt%). As shown in , with the increase of acrylamide concentration, the emulsion became less stable and changed from liquid to gel. When the monomer addition exceeded 14 wt%, the dispersion obtained flew poorly and formed white gel after a period of time. In addition, the viscosity of the lotion gradually increased, which indicated that the increase in monomer concentration would directly lead to the rise of the relative molecular weight of the polymer, strengthening the Weissenberg effect of the system. Therefore, the reasonable monomer dosage range for preparing polymer dispersions was 10 wt%~12 wt%. To ensure the ideal copolymers, AM concentration of 12 wt% and AM/AMPS/Si-St ratio of 8:1:1 were selected.
Table 3. The effect of monomer ratio and concentration on ternary copolymers.
Based on the synthesis of terpolymers, the grafting rate and incorporation of modified silica played an important role in the properties of composite copolymers. As shown in , the viscosity of the copolymers showed a trend of increasing and then decreasing, which indicated that different grafting rates of silica had a significant effect on the structure and state of the polymer molecules in solution, thus affecting the viscosity of the copolymers. The optimal modification condition was KH570:SiO2 = 1:1 in comparison. Additionally, the viscosity of the system increased and then decreased with the increase of modified silica (as shown in . The analysis suggests that the quaternary copolymers were a composite structure material with inorganic silica nanoparticles as the core and combined with organic terpolymers by chemical bonding and physical action. As a result, the strong bonding force between them made the viscosity increase. However, when the concentration of modified silica was too high, the reactive groups on the surface of nanoparticles could play the effect of chain transfer, which affected the increase of polymer molecular weight. Thus, the viscosity decreased slightly. The optimal addition amount of it was 3.3 wt% (0.2 g) of the total monomer.
Salt resistance
The data on the effects of different inorganic salts and salt concentrations on the performance of terpolymers were presented in while (c) (d) were quaternary polymers. With the increase in inorganic salt concentration, the viscosity and particle size of the two copolymers showed a decreasing trend. When the NaCl concentration reached 10,000 mg/L, the terpolymer viscosity retention was 58.44% and the particle size was around 3 μm, while the composite copolymers were 75.66% and the particle size was about 6 μm. When the concentration of MgCl2 was 10,000 mg/L, the terpolymer viscosity retention was 42.46%, and the particle size was about 2 μm. In contrast, the viscosity retention rate of quaternary copolymers was 69.32%, and the particle size was around 5.7 μm. As a result, the divalent salt ions had a more significant effect on the copolymer properties compared to the monovalent salt ions. Moreover, compared to the terpolymer, the impact of salt ions with different valence states on their properties had been improved, which could be found that composite copolymers had stronger salt resistance. Because the addition of inorganic materials took part in the construction of ‘a dynamic cross-linked network structure’. The presence of network structure increased the volume of polymer fluid mechanics, thus enhancing its shear resistance.
Figure 4. Effects of salinity of different salt species on viscosity and particle size of tri-quaternary copolymers.
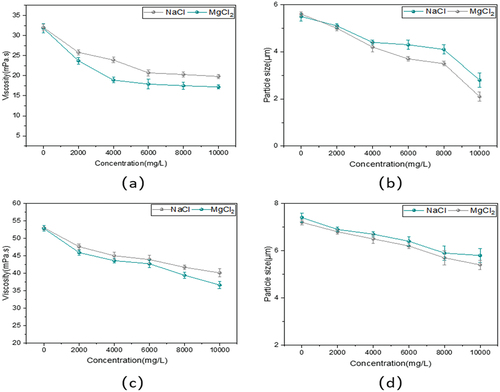
The experiments of comprehensive salt resistance of two copolymers were also carried out, as shown in (a) for terpolymers and (b) for quaternary copolymers. Due to the presence of salt ions, the microspheres undergo shrinkage and some degree of fusion under 12,810 mg/L. However, the viscosity and particle size of the two copolymers decreased slightly, indicating corresponding salt resistance. At 34,691 mg/L mineralization, the performance of the quaternary copolymers is maintained well, the particle size and viscosity within the reasonable application range. At the same time, the two reference values of the terpolymers decreased rapidly, which was due to the fact that a large increase in mineralization results in a significant increase in the interaction of the carboxylic acid groups on the polymer molecules with the metal ions in solution. Therefore, the overall salt resistance of the composite copolymers was also better than that of the terpolymers.
Temperature resistance
The macroscopic changes of the terpolymer solution on days 1, 7, and 14 in a 150°C incubator were shown in in order. It could be seen that the solution was still clarified after 7 days. But on the 14th day, white granular precipitation appeared in the lower part of the solution. Therefore, it could be assumed that its stability deteriorated after being placed at 150°C for a certain period, which specifically manifested in the molecular chain contraction and the phenomenon of agglomeration.
In were the microscopic changes of copolymers at the corresponding time. The spherical shape of it was still intact after 7 days, but the contour boundary was slightly unclear, and there was a slight fusion phenomenon between molecules. The microsphere morphology after 14 days had gradually lost its shape and intermolecular aggregation occurred, but the microsphere still existed and had no degradation.
In summary, terpolymers could stabilize at 150°C for 7 days. Composite copolymers did not fail after 14 days, the particle size of which was measurable on 14th, as shown in , so it also corroborated the above SEM conclusions. It could be concluded that the temperature resistance of quaternary copolymers was better than that of the terpolymers. On the one hand, the addition of the Si-O bond in Si-St improved its thermal stability, with a chain length approximately twice and a half that of the C-C bond. On the other hand, the addition of modified silica could be more firmly adsorbed and grafted on the polymer backbone, protecting the polymer backbone and side groups, which had good stability at high temperatures and improved the resistance of the polymer itself to high-temperature degradation.
Conclusion and discussion
From the perspective of molecular modification of polyacrylamide, two types of temperature and salt-resistant polyacrylamide copolymers were designed and prepared by precipitation polymerization, namely AM/AMPS/Si-St及 AM/AMPS/Si-St/g-SiO2. On the basis of terpolymers, nano-silica was added and modified. Four monomers were added in amounts of 12 wt%/1.5 wt%/1.5 wt%/3.3 wt%. The modification was KH570: SiO2 = 1:1. Compared with ternary copolymers, the temperature and salt resistance of quaternary composite copolymers increased by about 10% at the same salt concentration, and the stable retention time was extended to 14 days at 150°C. It indicated that the introduction of nano-silica significantly improved the temperature and salt resistance of the original polymer.
The profile control and flooding ability of two copolymers is an important indicator for its practical application in the field. The experiments on the injectability and sealing performance of them will be conducted for the next study focus.
Author contributions
Yunbao Zhang: Conceptualization, Methodology, Validation, Formal analysis, Project administration, Writing – review & editing, Supervision.
Jiamei Quan: Data curation, Investigation, Visualization, Writing – original draft, Writing – review & editing.
Chengzhou Wang: Conceptualization, Investigation, Resources, Supervision.
Wentao Li: Data curation, Writing – original draft, Formal analysis
Ruofei Du: Investigation, Validation
Hongchao Dong: Data curation, Resources
Disclosure statement
The authors declare that they have no known competing financial interests or personal relationships that could have appeared to influence the work reported in this paper.
Data availability statement
The data that support the findings of this study are available on request from the corresponding author, Jiamei Quan. The data are not publicly available due to their containing information that could compromise the privacy of research participants.
Additional information
Funding
References
- Fan M, Wang L, Li J, et al. Preparation of supramolecular viscoelastic polymers with shear, temperature, and salt resistance/sensitivity by amphiphilic functional monomer modification. Polymer Testing. 2022;116:107799. doi: 10.1016/J.POLYMERTESTING.2022.107799
- Xiaoxu T, Jianchong G, Qiuxia W. Evaluation and application effect of high performance polymer microspheres with high temperature and salt resistance. Oilfield Chem. 2022;39(3):425–430.
- Yongyu M, Meiqin L, Zhiyong W, et al. Study on plugging characteristics of novel temperature-resistant polymer microspheres. J Chem Pet Univ. 2020;33(1):48–53.
- Xiong C, Wei F, Zhang S, et al. Experimental Investigation of a mechanically stable and temperature/salinity tolerant biopolymer toward enhanced oil recovery application in harsh condition reservoirs. Energies. 2022;15(5):1601. doi: 10.3390/EN15051601
- Mingtao D, Kangwei Z, Gang L. Preparation and performance evaluation of temperature and salt resistant polymer microsphere gel system. Oilfield Chem. 2019;36(3):405–410.
- Pengao P, Ming Z, Yue G. Preparation and evaluation of high temperature and high salt resistant polyimide microspheres for oil displacement. Mater Sci Technol. 2023;31(3):41–47.
- Pengcheng L, Jie L, Jianbo C. Synthesis of temperature and salt resistant polyacrylamide nanospheres. Anhui Chem Industry. 2022;48(3):88–90+94.
- Shi J, Wu Z, Deng Q, et al. Synthesis of hydrophobically associating polymer: temperature resistance and salt tolerance properties. Polym Bull Prepublish. 2021;79(7):4581–4591. doi: 10.1007/S00289-021-03713-X
- Quan H, Zhuoke L, Huang Z. Self-assembly properties of a temperature- and salt-tolerant amphoteric hydrophobically associating polyacrylamide. RSC Adv. 2016;6(54):49281–49288.
- Akbari S, Mahmood SM, Tan IM, et al. Effect of aging, antioxidant, and mono- and divalent ions at high temperature on the Rheology of New polyacrylamide-based co-polymers. Polymers. 2017;9(10). doi: 10.3390/polym9100480
- Chen D, Liu X, Yue Y, et al. Dispersion copolymerization of acrylamide with quaternary ammonium cationic monomer in aqueous salts solution.European. Eur Polym J. 2006;42(6):1284–1297. doi: 10.1016/j.eurpolymj.2005.12.007
- Shi L-T, Li C, Zhu SS, et al. Study on properties of branched hydrophobically modified polyacrylamide for polymer flooding. J Chem. 2013;2013. doi: 10.1155/2013/675826
- Seright RS, Campbell AR, Mozley PS, et al. Stability of partially hydrolyzed polyacrylamides at elevated temperatures in the absence of divalent cations. SPE J. 2010;15(2). doi: 10.2118/121460-PA
- Weixia T, Chonggang W. Development of a new pore-throat scale inorganic-organic polymer composite microsphere profile control displacement agent. China Offshore Oil Gas. 2011;23(4):243–246.
- Lifeng B. Heat resistance salt resistant polymer microspheres particle research and evaluation [D]. Daqing: Northeast petroleum university; 2023.
- Zhong C, Luo P, Ye Z, et al. Characterization and solution properties of a novel water-soluble terpolymer for enhanced oil recovery. Polym Bull. 2009;62(1). doi: 10.1007/s00289-008-1007-6
- Sheng L, Cui J, Gao Y, et al. Preparation of polystyrene/polyacrylamide core-shell microspheres via microfluidizer-assistant emulsification template methods for oil recovery enhancement. J Phys. 2023;2463(1). doi: 10.1088/1742-6596/2463/1/012014
- Du D, Pu WF, Hu P, et al. P-Sulfocalix[4]arene functionalized hydrophobically associative polyacrylamide: flow characteristics and potential application to enhanced oil recovery. Polym Eng Sci. 2020;60:6. doi: 10.1002/pen.25380
- Jin Z, Shan G, Pan P. AM/AMPS/SSSPreparation and temperature and salt resistance of ternary copolymers. Chin J Chem Eng. 2023;74(2):916–923.
- Cao J, Song T, Zhu Y, et al. Application of amino-functionalized nanosilica in improving the thermal stability of acrylamide-based polymer for enhanced oil recovery. Energy Fuels. 2017;32(1):246–254. doi: 10.1021/acs.energyfuels.7b03053
- Ma X, Zhou Y, Yi P, et al. Design, preparation and properties of new polyacrylamide based composite nano-microspheres with like “ball in ball” structure. Colloids Surf A Physicochem Eng Asp. 2022;654. doi: 10.1016/J.COLSURFA.2022.130037
- Liu F, Zheng Z, Wang X, et al. Novel modified nano-silica/polymer composite in water-based drilling fluids to plug shale pores. Energy Sources Part A. 2022;44(4). doi: 10.1080/15567036.2021.1943071
- Menghan W. Synthesis and properties of micrometer scale phenolic resin/acrylamide temperature resistant polymer microspheres. Beijing: China University of Petroleum; 2020.
- Kim T, Kwon SH, Kim HJ, et al. Effect of the surface modification of silica nanoparticles on the viscosity and mechanical properties of silica/Epoxy nanocomposites. Compos Interfaces. 2022;29(13):1573–1590. doi: 10.1080/09276440.2022.2094572
- Junjie C, Guohong Q, Yonghui S, et al. Synthesis and performance evaluation of nano-sized silica plugging materials with core-shell structure. Drilling Prod Technol. 2018;41(5):95–97+101+13.
- Ruifeng L, Meixin C, Delan Z. Amphoterion star acrylamide copolymer modified by nano-silica. Fine Chem Intermed. 2020;50(5):49–56.
- Zhiyuan J, Guorong S, Pengju P. Preparation and temperature and salt resistance of AM/AMPS/SSS terpolymer. J Chem Eng. 2019;74(2):916–923.
- Wang Q, Chen B, Wang X, et al. Preparation and profile control evaluation of core‐shell structure and surface‐hydrophobic modified polyacrylamide microsphere. J Appl Polym Sci. 2022;139(45). doi: 10.1002/APP.53110
- Wang B, Lin M, Guo J, et al. Plugging properties and profile control effects of crosslinked polyacrylamide microspheres. J Appl Polym Sci. 2016;133(30). doi: 10.1002/app.43666
- Anle Z. Synthesis and properties of nano-silica composite microspheres by surface photografting [D]. Beijing: Beijing University of Chemical Technology; 2021.
- Kang W-L, Hu LL, Zhang XF, et al. Preparation and performance of fluorescent polyacrylamide microspheres as a profile control and tracer agent. Petroleum Sci. 2015;12(3). doi: 10.1007/s12182-015-0042-9
- Palin S. Study on mechanical properties of inorganic filler modified polypropylene. Jiangxi Const Mater. 2018;2018(11):12–14+17.
- Hua Z, Lin M, Guo J, et al. Study on plugging performance of cross-linked polymer microspheres with reservoir pores. J Pet Sci Eng. 2013;105. doi: 10.1016/j.petrol.2013.03.008