ABSTRACT
Alginate fibers have excellent flame-retardant properties and make up for other material defects by blending. To investigate the influence of the blending ratio of alginate fibers on the flame-retardant properties of waterproof and breathable layers for firefighting suits, this paper utilizes the needle-punching and hot-pressing nonwoven reinforcement processes to prepare waterproof and breathable layers based on alginate/aramid base cloths and conducts a series of performance tests on them. The results show that the char residue content of alginate blended base cloth is significantly improved relative to pure aramid, and the addition of alginate fibers to the base cloth of the waterproof and breathable layer improves its flame retardancy and thermal stability. The overall performance of the alginate/aramid blended base fabric waterproof and breathable layer was better than that of the aramid-based waterproof and breathable layer. Moreover, in the flame-retardant multilayer fabric system for firefighting apparel, the multilayer fabric system containing the alginate/aramid-based waterproof and breathable layer showed higher thermal protection performance. Therefore, the alginate/aramid-based waterproof and breathable layer can enhance the overall flame-retardant performance of firefighting clothing to a certain extent.
1 Introduction
Alginate fibers have excellent flame-retardant properties and can meet the demand for flame-retardant materials in a variety of fields [Citation1–3]. Calcium alginate has a limiting oxygen index (LOI) value of 48% [Citation4] and is incombustible in the natural environment. The thermal decomposition process of alginate fibers is extremely slow, with no molten droplets or smoke during vertical combustion testing. In the combustion process, alginate fiber thermal decomposition will generate a large amount of carbon dioxide, and water, and take away a lot of heat [Citation5]. At the same time, the metal ions will generate carbonate compounds and other residues, covering the fiber surface, isolating the oxygen, and hindering the combustion process. In the further heat process, it plays the role of heat-absorbing decomposition to generate calcium oxide to hinder the secondary combustion of the fabric. Under the dual flame-retardant effect, alginate fiber shows excellent flame-retardant properties. Therefore, the use of alginate fibers in the thermal properties to study its application in the field of fire extinguishing apparel has a very broad prospect.
Waterproof and breathable layer also known as waterproof and moisture permeable layer, is a fabric in a class of waterproof, breathable, moisture permeable, and other properties of multifunctional laminated fabrics. Usually, it uses coating treatment [Citation6], covering film [Citation7], and other means to make the fabric to achieve the effect of waterproof and breathable. 97 type fire fighting protective clothing commonly used waterproof and breathable layer belongs to the composite fabrics, by the aramid fiber material base cloth and polytetrafluoroethylene (Polytetrafluoroethylene, PTFE) membrane composite and become. The waterproof breathable layer of the base cloth is critical, in addition to flame-retardant properties, its other performance is also an important point of selection of the study. Aramid fiber is the most commonly used synthetic fiber in protective clothing, aramid 1313, is an intrinsic flame-retardant fiber, structurally stable below 200 ℃, can be used for a long time, has fire resistance, dimensional stability, flame-retardant properties, and spinnability are excellent [Citation8–12]. However, its disadvantage of poor comfort is also very significant, which is manifested in the hard hand feel, poor finishing effect, and a large amount of smoke produced when burning in the air [Citation13].
Most of the existing large studies focus on the performance changes of fabrics or garments under high-intensity heat flow, and there are fewer studies on the performance of firefighting garments under low- and medium-intensity heat radiation. From the data of fire situation reports released by the Fire and Rescue Bureau of the Ministry of Emergency Management in the past 10 years, most of the firefighters suffering from skin burns occurred in low-intensity heat environments, and the low-intensity heat radiation ranges from 2 kW/m2-20 kW/m2, i.e., firefighters are exposed to ambient temperatures of between 60 and 300°C. Thermal exposures below flash fire conditions are not sufficient to cause degradation of clothing, but they are usually of long duration, and firefighters working in such environments for extended periods can similarly cause second-degree burns to the skin. Therefore, this study focuses on the performance of flame-retardant materials under low to medium heat exposure.In the past, some studies on waterproof and breathable layers were mostly focused on thermal stability and hydrostatic pressure resistance. To cope with the severe and complex fire environment, it is necessary to improve the flame-retardant performance of the waterproof and breathable layer, to enhance the performance of the overall fire suit. Some new functional materials and technologies have emerged [Citation14,Citation15], such as aerogel [Citation16–19], phase change materials [Citation20], basalt fibers [Citation21], and other materials and their applications. Aerogel nonwoven fabrics were used as reinforcement materials for waterproof and breathable layers of firefighting suits to reduce the risk of burns, increase comfort, and enhance the protection of the human body [Citation22]. Activated charcoal fabric as a base fabric laminated with an ePTFE membrane can add chemical and biological protection to the waterproof and breathable layer [Citation23]. The use of PAN/silica aerogel membranes as a waterproof breathable layer allows for a lighter fabric system with improved wearer mobility and thermal comfort [Citation24]. However, no one has yet used nonwoven fabrics after blending alginate as a base fabric for waterproof and breathable layers, so this study starts from the study of new material alginate fibers, which in turn expands the application field of alginate fibers and expands the range of material applications for waterproof and breathable layers.
In this paper, alginate/aramid hybrid base cloths were prepared by simply mixing alginate fibers and aramid fibers in different proportions, and laminated composite fabrics were prepared by using PA hot-melt adhesive mesh film as the connecting agent. Scanning electron microscopy was used to observe the mixing of the base fabric fibers, and the fibers were fully mixed after carding and needling was processed by a small prototype machine. The new waterproof and breathable layer was tested for air permeability, moisture permeability, and thermal stability. The new waterproof and breathable layer was combined with the other three layers of fabrics, and its comfort performance and flame-retardant performance were tested. On this basis, the overall performance of the multilayer fabric system for firefighting apparel containing the waterproof and breathable layer of alginate/aramid hybrid base fabric was investigated.
2 Materials and methods
2.1 Materials
The materials used in this study include polyamide (PA) hot melt adhesive net (Suzhou Hanruisi New Material Technology Co., Ltd) with a melting point of 115–135℃; calcium alginate nonwoven fabric (Qingdao Yuanhai New Material Science and Technology Co., Ltd); PTFE film (Zhejiang Hangzhou Changnuo Fluorine New Material Science and Technology Co., Ltd., 20um); aramid 1313 fibers (1.76dtex x 38 mm, production process of dry-spinning and wet-spinning, Yantai Taihe New Material Co. Ltd); Calcium alginate fiber (1.53dtex × 38 mm, production process of wet spinning, Qingdao Yuanhai New Material Technology Co).
2.2 Preparation of alginate/aramid laminated composite fabrics
Calcium alginate fibers and aramid 1313 fibers were weighed and mixed according to the weight ratios 0/100, 20/80, 50/50, 80/20, and 100/0. First, the blended fibers were prepared by carding using a digital small sample clearing and combing combined machine to obtain a membrane-like fiber web with a specification of 50 cm × 80 cm. After that, the membrane fiber mesh was reinforced using a needle-punching composite small sample machine (DSNm-01–50, Tianjin Jiacheng Electromechanical Equipment Co., Ltd) to obtain nonwovens with different mixing ratios, and the alginate/aramid mixed nonwovens with mass ratios of 0/100, 20/80, 50/50, 80/20, and 100/0 were noted as Aramid, Alginate 1/Aramid 4, Alginate 5/Aramid 5, Alginate 4/Aramid 1, Alginate.
In this experiment, a high melting point PA hot melt adhesive mesh was selected, which can resist water washing below 60℃. Using PA hot melt adhesive mesh as the adhesive, the hot melt adhesive method was adopted, and the corresponding hot pressing temperature, time, and pressure were set on the plate vulcanizing machine (Polystat300s, Servitec, Germany). The nonwoven fabric, PA hot melt adhesive mesh, and PTFE film were stacked to form a sandwich structure and wrapped with aluminum foil. The materials were placed on a 34.5 cm × 34.5 cm instrument plate, and the instrument button was activated to perform the fabric hot press fusion process. When the hot pressing time was up, the pressure was removed and the samples were moved to room temperature for cooling treatment, and the laminated composite fabric was obtained after cooling. The specific physical parameter data of the prepared waterproof and breathable layer are shown in .
Table 1. Basic properties of waterproof and breathable layers.
2.3 Preparation of flame-retardant multilayer fabrics
GA10–2014 Firefighter Firefighting Protective Clothing stipulates that firefighter firefighting protective clothing consists of four layers of fabrics: the outer layer, waterproof and breathable layer, heat insulating layer, and comfort layer. Therefore, in this study, one outer fabric, four waterproof and breathable layer fabrics, one insulating layer fabric and one comfort layer fabric were selected to form five different fabric combinations to prepare flame-retardant multilayer fabrics for firefighting clothing. And the thermal protection performance and thermal comfort performance of the multi-layer fabric system were tested strictly according to the test standards.
For the outer layer, a twill fabric with a fabric composition of 93% Nomex + 5% Kevlar + 2% AS was used, recorded as A. According to the performance test of the waterproof and breathable layer, fabrics B2, B3, and B4 with better performance were selected, and another 70g80% Nomex + 20% Kevlar+PTFE membrane was used for the comparative test, recorded as B6. The thermal insulation layer was made of a heat-pressed fabric with 70g80% Nomex + 20% Kevlar. The heat insulating layer is made of 70g80%Nomex +20%Kevlar hot-pressed nonwoven fabric, marked as C, and the comfort layer is made of 50%Nomex +50%VicoseFR twill fabric, marked as D. lists the specific fabric specifications for the outer layer, insulation layer, and comfort layer. The thicknesses and surface density values of the waterproof and breathable layers B2/B3/B4 have been listed in and are replaced by slashes in to avoid repetition.
Table 2. Basic fabric specification information.
Each of the four layers of fabrics is placed in a textile static box for 24 hours until equilibrium is reached in accordance with the provisions of GB/T 6529 in a secondary standard atmosphere, i.e., at a temperature of 20°C ±2°C and a relative humidity of 65% ± 3%. Then, the layers were stacked in the order of outer layer, waterproof and breathable layer, heat insulating layer and comfort layer. And again placed in the above conditions for 24 hours until equilibrium is reached. In this way, the preparation of the flame-retardant multilayer fabric was completed, and the preparation process is shown in . The combination of multi-layer fabric systems and the main parameters are shown in .
Table 3. Multi-layer fabric combinations for firefighting clothing.
2.4 Test methods
2.4.1 Scanning electron microscopy
TESCAN (VEGA3, Czech) was utilized to observe the micro-morphology of hybrid fabrics of 4 mm × 4 mm size. The accelerating voltage was set at 10 kV, and each sample was sputter sprayed with gold under vacuum for 1 min before SEM testing.
2.4.2 Peel strength
The HD026PC multifunctional electronic fabric strength tester (Nantong Hongda Experimental Instrument Co., Ltd) was used to test the samples of 200 mm length and 25 mm width according to the test standard FZ/T 010107–1991, and the traction gripper was set to move at a speed of 100 mm/min, with a clamping distance of 30 mm, and a range of 300N. Before the test, the membrane and the base cloth were peeled back by 30 mm and pre-wetted in a standard atmosphere for 24 hours.
2.4.3 Air permeability
According to the GB/T5453–1997 standard, the YG461E-III automatic air permeability meter (Ningbo Textile Instrument Factory) was used to test the air permeability of fabrics under the pressure difference of 100 Pa.
2.4.4 Moisture permeability
YG601H-II type computerized fabric moisture permeability tester (Ningbo Textile Instrument Factory) was used to test the moisture permeability of the samples with GB/T12704.2–2009 as the test standard. The specimen was covered with a specimen cup containing 34 ml of distilled water, put into the moisture permeability tester, and quickly weighed every 1 hour of moisture permeability. Calculate the moisture permeability performance of the specimen per unit of time, and take the average value of three samples as the result.
2.4.5 Thermal stability
Concerning the GA10–2014 GB/T 8628–2001 standard, the samples of mixed-base waterproof and permeable layer with the size of 10 cm × 10 cm were tested by using DHG-9140A type electric heating constant temperature blast drying oven (Shanghai Qixin Scientific Instrument Co., Ltd). Each sample was measured 5 times in different positions in horizontal and vertical directions, and the results were averaged.
2.4.6 Limiting oxygen indexability
According to GB/T 5454–1997 standard, the combustion behavior of aramid, alginate, and aramid/alginate mixed base waterproofing breathable layer was studied by using JF-5 automatic oxygen index tester (Beijing AVIC Times Instrument Co., Ltd).
2.4.7 Thermal cracking properties
TGA5500 Thermogravimetric Analyzer (TA Instruments Waters Inc., U.S.A.) was used to study the thermal stability of the mixed base waterproof and breathable layer samples ((4 ± 0.2) mg). Nitrogen atmosphere: airflow rate 25 ml/min, heating rate 10℃/min; air atmosphere: airflow rate 25 ml/min, heating rate 10℃/min, and set the heating range from room temperature to 700℃. The experiment was repeated 3 times to take the average value.
2.4.8 Thermal protection performance testing
TPP(Thermal Protection Performance) tests were conducted on multilayer fabric combinations concerning ISO 6942–2002 (EN366) and GA 10–2014. The RFH-V thermal protection performance tester (Shaanxi Yuanfeng Textile Technology Research Co., Ltd) was used to test the specimens with a size of 15 cm × 15 cm. Each sample was sampled three times at different positions and the test results were averaged. Before testing, the samples were humidified for 24 h in a constant temperature and humidity chamber at a temperature of (20 ± 2)℃ and a relative humidity of (65 ± 4)%.
2.4.9 Cone heat test
The burning behavior, heat release rate, and smoke release rate of the samples at a heat flow density of 35 kW/m2 were measured using a cone calorimeter (Fire Testing Technology, UK) based on the ISO5660–1 standard. The tests were repeated three times for each sample.
3 Results and discussion
3.1 Properties of waterproof breathable layer
3.1.1 Microstructural morphology
The surface microstructures of calcium alginate nonwovens, aramid 1313 nonwovens and aramid/calcium alginate hybrid nonwovens with different blending ratios were observed by scanning electron microscopy, as shown in . The longitudinal surface of aramid fibers prepared by dry-spraying and wet-spinning method is relatively smooth, but there are certain grooves and grooves, and particles are attached to the surface. Calcium alginate fiber prepared by wet spinning method has a larger difference compared with aramid, with uniform longitudinal thickness, smoother surface and very little particle attachment. By observing the surface micro-morphology of the hybrid nonwoven, it can be found that the inter-fibers are interlaced and haphazardly distributed, and it is difficult to distinguish calcium alginate and aramid fibers, which suggests that the use of the small carding machine mixes the aramid fibers and calcium alginate fibers sufficiently. Moreover, the addition of high-strength aramid fibers supported the backbone structure in the blends, which greatly enhanced the spinnability and tensile strength of the blended nonwoven fabrics.
3.1.2 Air and moisture permeability
The good or bad performance of fabric breathability and moisture permeability has a direct relationship with the comfort of the garment [Citation25]. Good moisture permeability performance makes the sweat produced on the skin surface move from the inside of the garment to the outside in a timely manner, so that the skin surface remains dry, thus keeping the body itself comfortable. The results of air permeability and moisture permeability properties of the waterproof breathable layer are shown in .
Table 4. Air and moisture permeability data for fabrics.
From the test results, among the five different base fabrics of the waterproof-breathable layer, the order of preference is B2>B4>B3>B1>B5, and only B2, B3, and B4 can reach the Chinese standard: the water vapor permeability of waterproof breathable layer in 24 hours shall not be less than 5000(g/m2·24 h). It indicates that the waterproof and breathable layer of blended base fabric has improved moisture permeability, and B2 has the best moisture permeability. In the breathability test results, fabric B1 has the best breathability, and B5 has the worst breathability.
Combined with the basic data of the fabric in , and the breathability performance is compared. It is found that the change rule of air permeability has some correlation with the fabric composition, but it is relatively limited. The higher the content of calcium alginate fiber in the nonwoven fabric, the breathability of the fabric will be relatively poor. However, at the same time, fabrics made by weighing and blending calcium alginate fibers with aramid 1313 fibers at a weight ratio of 50/50 have better air permeability than fabrics with other weight ratios.
Comparison with the moisture permeability properties revealed that the blended fabrics had overall higher moisture permeability than the fabrics with a single fabric component.In order to explore the effect of blend composition on moisture permeability properties, all moisture permeability data were entered into IBM SPSS Statistics26 for normal distribution test and ANOVA.The S-W (Shapiro-Wilk) normal distribution test resulted in a value of 0.609, which is greater than 0.05 and not statistically significant, thus the moisture permeability data obeyed a normal distribution. The ANOVA data for fabric moisture permeability is shown in with a significance level of 0.063, which indicates that the blended base fabric composition has a significant effect on the moisture permeability of the fabric.
Table 5. Fabric moisture permeability ANOVA.
3.1.3 Thermal stability
The good dry heat stability of the fabric can ensure the thermal protection performance of the garment when firefighters are exposed to thermal hazards and protect them from heat, flames, and other hazards. shows the results of the dimensional test of the waterproof and breathable layer placed in a drying oven at 2605℃ for 5 minutes.
Table 6. Thermal stability of breathable waterproofing layers.
From the test results, the transverse and longitudinal dimensional change rates of all fabric specimens ranged from 0.97 to 4.44%. From , the transverse dimensional change rate, the longitudinal dimensional change rate, and the combined dimensional change rate of alginate are higher than those of aramid fabrics. This may be due to the large amount of water contained in the alginate fibers, which underwent dehydration condensation at 260℃. Therefore, after blending alginate and aramid, the dry heat stability of the composite fabrics was greatly improved as the proportion of aramid increased.
3.1.4 Limiting oxygen index
The oxygen index of flammable fabrics was greater than 22%, the oxygen index of combustible fabrics ranged from 22% to 27%, while the oxygen index of flame-retardant fabrics was greater than 27% [Citation26]. The limiting oxygen indices of alginate, aramid, and alginate/aramid hybrid-based waterproof and breathable layers are shown in . It can be seen that the limiting oxygen index of alginate fibers is higher than that of aramid, and the limiting oxygen index increases with the increase in the proportion of alginate fibers incorporated.
Table 7. Fabric limiting oxygen index.
3.1.5 Thermal cracking properties
This experiment investigates the thermal cracking behavior of waterproof and breathable layers of five different compositions in air and nitrogen atmosphere, respectively. and show the TG (Thermal Gravity Analysis) images and DTG (Microcommercial Thermogravimetric Analysis) images of the waterproof-breathable layers in nitrogen and air atmospheres, and the specific experimental data are shown in and .
Figure 3. Waterproof breathable layer (a) TG curve under nitrogen atmosphere; (b) DTG curve under nitrogen atmosphere.
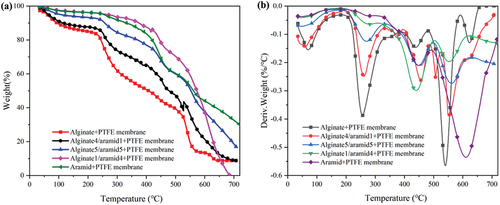
Figure 4. Waterproof and breathable layer (a) TG curve under air atmosphere; (b) DTG curve under air atmosphere.
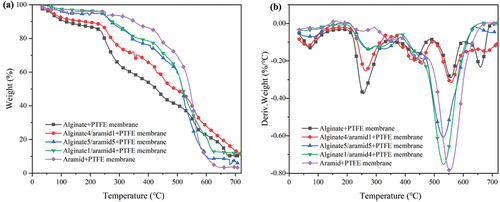
Table 8. Thermogravimetric test data under nitrogen atmosphere for waterproof and breathable layers (where Tn is in ℃; Rn is in %/℃).
Table 9. Thermogravimetric test data under air atmosphere for waterproof and breathable layers (where Tn is in ℃; Rn is in %/℃).
Calcium alginate fiber contains a large number of carboxyl and hydroxyl groups. Before 200 ℃, alginate fiber dehydration and glycosidic bonding, a waterproof breathable layer made of calcium alginate fiber mass fraction change is greater than 10%. Before 100 ℃, the aramid waterproof breathable layer mass fraction changes little until about 370 ℃, the rate of change is only 10%. 200 ~ 500 ℃ is the main thermal degradation interval of alginate fibers, glycosidic bonds are further broken intermediate substances are generated, and the intermediate substances are then decomposed, decarboxylated, and carbon-based [Citation27]. PA hot melt adhesive mesh, the chemical composition of which is a co-polyamide, the basic thermal degradation is completed above 500℃, with very little residual carbon remaining [Citation28].
Alginate fibers improved the thermo-oxidative stability of the alginate/aramid hybrid waterproof-breathable layer in the higher temperature region. From the important parameters of the TG and DTG curves of the waterproof-breathable layer in the nitrogen atmosphere (), it can be seen that the coke residues of the alginate/aramid blended waterproof breathable layer were 8.94% (alginate 4/aramid 1), 18.61% (alginate 5/aramid 5), and 32.66% (alginate 1/aramid 4), which were higher than those of aramid (0.34%) and alginate (8.82%). Aramid 1313 is a serrated linear macromolecule consisting of peptide amine groups interconnected with interstitial phenyl groups [Citation29], the melting point is not clear, but the mass decreases sharply above 400℃, and begins to decompose and carbonize rapidly. With the increase of aramid doping content, the temperature is greatly enhanced when the heat loss reaches 5% and 10%. Therefore, doping a certain amount of aramid can improve the thermal stability of fabrics in the pre-thermogravimetric period. Compared with the air environment, the chemical inertness of nitrogen makes the degradation rate of the samples slower in the nitrogen environment. In the late stage of thermal cracking, the presence of PA hot melt adhesive film and PTFE film similarly increased the thermal degradation rate of the waterproof-breathable layer.
Compared with the simple hybrid nonwoven, the thermal cracking image of the waterproof and breathable layer is more complicated. The melting temperature of the PA hot melt adhesive membrane is 110–125°C, and the weight loss rate of the PTFE membrane is related to its density in the thermal cracking test. The temperature of a low-density PTFE membrane at 2% weight loss is 406.2°C, and the temperature of a high-density PTFE membrane at 2% weight loss is 500.9°C. The temperature of the waterproof and breathable layer is more complicated than that of simple hybrid nonwoven. During the thermal cracking process, the PA hot melt adhesive mesh film and PTFE film undergo chemical reactions such as carbonization, dehydration, and cyclization, which affect the thermal degradation process of the hybrid base cloth.
When calcium alginate was burned in an air atmosphere, the pure alginate-based waterproof and breathable layer reduced the peak of the rate of mass change curve after 350℃ due to the presence of PA hot-melt adhesive film and PTFE film. The incorporation of alginate fibers reduced the Rmax of the aramid material after 400℃, which decreased with the increase of the incorporation ratio. The coke residues of the alginate/aramid blended waterproofing breathable layers in air atmosphere were 13.28% (alginate 4/aramid 1), 6.94% (alginate 5/aramid 5), and 11.63% (alginate 1/aramid 4), which were significantly higher than that of aramid (3.55%), respectively, when the temperatures reached 700℃. During the thermal degradation of alginate fibers under air atmosphere, hydroxyl and carboxyl groups and hydrogen ions undergo redox and dehydration reactions to produce gases that escape [Citation4,Citation30]. Calcium ions react with carbon and oxygen to form calcium carbonate, which adheres to the surface of the combustible material and serves as a mass preservation as well as an air barrier. Therefore, in an air atmosphere, the residual carbon content of the waterproof and breathable layer of pure calcium alginate at 700℃ is much larger than that of pure aramid.
3.1.6 Comprehensive performance
Different evaluations of the five waterproofing and permeable layers were conducted using multiple tests, and ultimately, the results of the mixed-base waterproofing and permeable layer tests need to be processed for comprehensive evaluation and selection of the best. TOPSIS is a methodology for the comprehensive evaluation of all the different portfolios, which can make effective use of the data from multiple tests and reflect the gaps between different samples [Citation31]. The results of the TOPSIS evaluations are referenced in .
Table 10. TOPSIS evaluation results.
From the TOPSIS evaluation results, pure aramid and pure alginate waterproof and breathable layers had the lowest overall scores, and hybrid fabrics showed excellent performance in the performance test. Based on the results of the TOPSIS evaluation, hybrid fabrics were selected for the overall performance test in the subsequent multilayer fabric system test.
3.2 Flame-retardant multilayer fabric system performance
3.2.1 Air and moisture permeability
Breathability is an important thermal comfort indicator for fabrics in the dry state, and the test data related to the breathability of multilayer fabric systems are shown in . According to the test results, the air permeability of combination 5 is optimal, which may be because the combination does not have a waterproof and breathable layer, which results in better air permeability within the fabric; at the same time, the thickness of combination 5 is small, which indicates that there exists a certain relationship between the thickness and the air permeability. Thus the correlation between the thickness and the air permeability was analyzed, and the result was 0.886, which shows a high correlation, and it is significant at the 0.05 level of significance. This indicates that there is a significant correlation between the air permeability and thickness of the fabric. Therefore, the relationship between thickness and performance must be carefully balanced when selecting flame-retardant fabrics for firefighting wear.
Table 11. Air and moisture permeability data for multi-layer fabric systems.
In terms of the overall comfort of the firefighting suit, the greater the water vapor permeability, the higher the comfort of the wearer. shows the values of WVT(Water-Vapour Transmission) rate of each single-layer fabric and its multi-system combination of fabrics for fire-fighting clothing. After 3 tests, the average value and standard deviation were taken. From , the water vapor permeability of the multi-layer fabric system is in descending order: 5 > 4 > 3 > 2 > 1. The water vapor permeability of combination 5 is much greater than that of the other combinations, indicating that the waterproof-breathable layer has a significant effect on the overall water vapor permeability of the fabric. In terms of the fabric system consisting of waterproof and breathable layers of blended base fabrics alone, the overall moisture permeability increases with decreasing thickness. Thus, the moisture permeability increases with decreasing number of layers and thickness.
Table 12. Water-vapour transmission rate of multilayer fabric systems.
3.2.2 Fabric surface morphology after thermal exposure
demonstrates the comparison of surface morphology changes before and after thermal protection testing of the multi-fabric system under a radiant/convective 82.7 kw/m2 heat source.
The multi-layer fabric system combination had a flat fabric surface before the combined thermal radiation/heat convection heat exposure, and after the heat exposure test, the outer fabric appeared to have different degrees of bulging. The reason may be that there is a certain gap between the outer layer and the waterproof and breathable layer, and the gas generated by the combustion of the outer layer is stored between the outer layer and the waterproof and breathable layer during the combustion process. Increasing the thickness of the air layer can slow down the burning rate of the fabric, thus delaying the time to cause second-degree burns to the skin. The transverse and longitudinal sizes of the exposed portion of the combustion are 9.6 cm and 9.7 cm, respectively, while it can be seen from that the phenomenon of bulging of the outer layer significantly increases the combustion area, causing the outer fabric to shrink.
3.2.3 Thermal protective performance test analysis
In this study,TPP(Thermal Protection Performance) of a multilayer fabric system was tested using a thermal protection tester by simulating thermal radiation and convective heat sources in an actual fire situation. Indirect contact was used in the test to simulate the situation of a firefighter wearing a fire suit, where there is a certain static air layer situation between the fabric and the skin surface. The heat flux of the apparatus was set to 82.7 KW/m2 (2 cal/m2⋅ s). When the heat flux is 2.68J/cm2, the human body has a burning sensation. When the heat flux is 5.02J/cm2, second-degree burns occur on the human skin, at which time the skin requires medical attention. The second-degree burn time is designated as the protection time. See for TPP test results for multi-layer fabric combinations.
Table 13. TPP test results for multi-layer fabric systems.
The TPP value is directly proportional to the secondary burn time and the greater the secondary time, the better the fabric’s thermal protection performance [Citation32]. According to the provisions of GA10–2014 Firefighting Protective Clothing for Firefighting Clothing for thermal protection performance, the TPP value should be less than 28cal/cm2.From , it can be seen that all combinations of TPP values are higher than the standard value. And there is no melting, brittle cracking and shrinkage during the burning process. The TPP value of combination 3 is significantly higher than the other combinations and has the best thermal protection performance.
3.2.4 Characterization and discussion of flame-retardant properties
To gain an insight into the overall combustion performance of the fabricated multilayer fabric system, tests were carried out using a cone calorimeter. shows the HRR(Heat Release Rate), THR(Total Heat Release), SPR(Smoke release Rate), and TSR(Total Smoke Release) images. Cone calorimetry test data is presented in . All multi-layer flame retardant fabrics have no melting or dripping phenomenon during the burning process.
Table 14. Cone calorimetric test data for multi-layer fabric systems.
The outer layer of the multi-layer fabric system consists of 93% Nomex + 5% Kevlar + 2% AS fibers, the aramid fibers are structurally stable and are in direct contact with the flame. When the test temperature reaches the glass transition temperature of the aramid fibers, the outer fabric is destroyed and the fabric system begins to release a large amount of heat.
The ignition time reflects the degree of combustion resistance of fabrics [Citation33], and there is a certain gap in the ignition time of multi-layer fabric combinations. This may be because the fabric fully absorbs external heat during the pre-ignition period. In particular, the moisture adsorbed by the alginate fiber in the waterproof and breathable layer is volatilized by heat, absorbing a large amount of heat and reducing the total heat energy transferred, which enhances the overall flame-retardant properties to a certain extent and prolongs the ignition time of the fabric combination.
In the cone calorimetry test, the HRR is one of the most important performance parameters to measure the burning behavior of the material to characterize the fire intensity, and the maximum degree of heat release rate is the peak heat release rate (p-HHR), and the larger the HRR and p-HHR of the test samples are, the higher the heat of combustion of the material is, and the more hazardous the material is in the fire. In the heat release rate, it can be seen from that the heat release rate curves of all combinations are in the form of sharp peaks, and all combinations release a large amount of heat within 50 seconds before the start of the experiment, among which, the peak heat release rate of combination 2 reaches 159 kW/m2, and the combination 1 is relatively the smallest, 123 kW/m2. In the process of combustion, residual carbon will be formed in the outer layer of the fabrics, which will impede the transfer of the heat flow to the inner layer. The peak heat release rate occurs at 20 seconds for all combinations and combination 2 has the highest total heat release. Combination 4 had the largest average peak heat release rate throughout the cone calorimetry test and combination 3 had the smallest. Lower heat release metrics in the cone calorimetry experiments represent a lower potential fire hazard for the test samples, i.e., smaller heat release metrics imply a lower thermal hazard. The combination of heat release rate, peak heat release rate, and ignition time can be used to determine the degree of hazard of the material, thus combination 3 has the smallest combustion hazard.
Figure 6. Heat release rate, total heat release, smoke release rate, total smoke release for tested combinations.
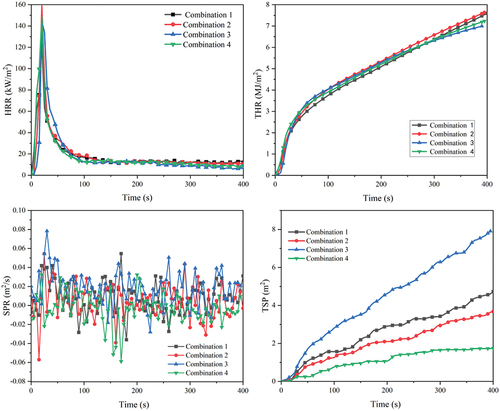
The higher the amount of carbon residue formed by combustion, the better the flame retardancy of the material. Upon completion of the combustion test, the amount of residual carbon in the test combination containing alginate fibers was greater than that in combination 4, and the amount of residual carbon in combination 3 containing 80% alginate fibers was as high as 27.23%. The calcium ions of calcium alginate act as a catalyst in combustion, helping to form a strong carbon layer that hinders heat transfer and improves overall flame retardancy.
In the statistics of factors causing casualties in fires, toxic gases are the most influential lethal factor [Citation34]. Therefore, a comprehensive evaluation of the potential smoke hazards of fabrics is needed. According to the data in , combination 3 produces a large amount of smoke during combustion, but the carbon monoxide generation is lower than the other combinations. From , it can be seen that the rate of smoke release is in the form of a wavy line, which may be because due to the surface effect of the fabric, the residual carbon generated will cover the surface of the unburned fabric, hindering combustion and at the same time impeding the production of smoke.
4 Conclusion
In this paper, a waterproof and breathable layer of alginate/aramid-based fabric was successfully prepared and tested for various aspects of comfort and flame-retardant properties. The results of scanning electron microscopy showed that the alginate and aramid fibers were adequately blended. In the thermogravimetric test, the char residue of the alginate blended base cloth was significantly improved relative to the pure aramid. Through the tests of moisture permeability, thermal stability, and ultimate oxygen index, it can be found that the performance of the waterproof and breathable layer of alginate/aramid-based fabric has been improved in various aspects such as thermal dimensional stability and flame-retardant properties relative to the single-material nonwoven fabric. The performance of the multilayer fabric system containing an alginate hybrid base fabric waterproof and breathable layer was tested and analyzed. Comfort performance tests showed that the presence of the waterproof and breathable layer hindered its overall comfort. However, the moisture permeability and air permeability of the alginate/aramid-based fabric waterproof-breathable layer complied with Chinese standards. In the thermal protection performance test, the multilayer fabric system with an alginate/aramid-based waterproof and breathable layer showed high thermal protection performance. In the cone calorimetry test, combined with the heat release and ignition time indicators, the higher proportion of alginate fibers in the base fabric has a lower potential thermal hazard. The waterproof and breathable layer of alginate/aramid base cloth improved the overall flame retardancy to some extent. Therefore, in summary, the effect of alginate/aramid-based fabric waterproof and breathable layer on the overall fabric flame retardancy is positive.The waterproof and breathable layer has an indispensable position in the whole flame retardant fire suit system. In future research, expanding the application of new materials in waterproof and breathable layers, reducing the thickness and quality of waterproof and breathable layers, developing thin and lightweight firefighting clothing systems, and at the same time, improving its breathability and moisture permeability to prolong the time of human body scalded and to protect the firefighters’ life and health, are of great significance for the future development of flame retardant material research.
Availability of data and material
Provide the experimental data according to the periodical reasonable request.
The data that support the findings of this study are available from the corresponding author, LI M, upon reasonable request.
Acknowledgments
The authors would like to thank Textile and Clothing College Experimental Teaching Center of Qingdao University for providing the experimental equipment and site.
Disclosure statement
No potential conflict of interest was reported by the author(s).
Additional information
Funding
References
- Wu NJ, Niu FK, Lang WC, et al. Highly efficient flame-retardant and low-smoke-toxicity poly(vinyl alcohol)/alginate/montmorillonite composite aerogels by two-step crosslinking strategy. Carbohydr Polym. 2019;221:221–230. doi: 10.1016/j.carbpol.2019.06.007
- Pan Y, Wang W, Liu LX, et al. Influences of metal ions crosslinked alginate based coatings on thermal stability and fire resistance of cotton fabrics. Carbohydr Polym. 2017;170:133–139. doi: 10.1016/j.carbpol.2017.04.065
- Wang YL, Li ZP, Li YY, et al. Spray-drying-assisted layer-by-layer assembly of alginate, 3-aminopropyltriethoxysilane, and magnesium hydroxide flame retardant and its catalytic graphitization in ethylene–vinyl acetate resin. ACS Appl Mater Inter. 2018;10(12):10490–10500. doi: 10.1021/acsami.8b01556
- Zhang CJ, Liu Y, Cui L, et al. Bio-based calcium alginate nonwoven fabrics: flame-retardant and thermal degradation properties. J Anal Appl Pyrolysis. 2016;122:13–23. doi: 10.1016/j.jaap.2016.10.030
- Zhang XL, Yang J, Weng L, et al. Enhanced mechanical performance of cellulose nanocrystal doped eco-friendly calcium-alginate based bio-composite fiber with superior flame retardancy. Text Res J. 2022;92(11–12):1820–1829. doi: 10.1177/00405175211073351
- Shaid A, Wang LJ, Padhye R. Textiles for firefighting protective clothing. In: Guowen S Faming W, editors. Firefighters’ clothing and equipment: performance, protection, and comfort. Boca Raton, FL: CRC; 2018. pp. 1–30.
- Song G, Lu Y. Flame resistant textiles for structural and proximity fire fighting. In: Handbook of fire resistant textiles. Cambridge, UK: Woodhead Publishing; 2013. pp. 520–548.
- Atanassov A, Koleva D, Yordanov M. Synthesis and properties of composites of ultra-high molecular weight polyethylene with aramid or aramid and carbon fibres. Oxid Commun. 2007;30:849–858.
- Ding HQ, Kong HJ, Sun H, et al. Improving aramid pulp dispersion in epoxy resin via the in situ preparation of SiO2 on an aramid pulp surface. Polym Composites. 2020;41(4):1683–1693. doi: 10.1002/pc.25489
- Jassal M, Ghosh S. Aramid fibres - An overview. Indian J Fibre Text Res. 2002;27:290–306.
- Sun GH, Wang WP, Wang LC, et al. Effects of aramid honeycomb core on the flame retardance and mechanical property for isocyanate-based polyimide foams. J Appl Polym Sci. 2017;134(28):8. doi: 10.1002/app.45041
- Zhao HF, Zhu YB, Sha LZ. Study of the relationship between characteristics of aramid fibrids and mechanical property of aramid paper using DSC. E-Polymers. 2014;14(2):139–144. doi: 10.1515/epoly-2013-0063
- Li M, Yu M, Liu JC, et al. 1313/Properties of calcium alginate fibre nonwovens. Text Technol Int. 2020;50(10):16–21.
- Jiang LY, Li Z, Liang YM, et al. High-efficient fire-safe epoxy enabled by bio-based atomic-level catalytic engineering. Chem Eng J. 2023;461:141967. doi: 10.1016/j.cej.2023.141967
- Zhang L, Li Z, B QQ, et al. Strong yet tough epoxy with superior fire suppression enabled by bio-based phosphaphenanthrene towards in-situ formed diels-alder network. Composites. 2023;251:110490. doi: 10.1016/j.compositesb.2022.110490
- Gong XB, Liu YJ, Zhao XM. Research progress of aerogel materials for thermal protection. Text Res J. 2022;43:187–196.
- Shaid A, Bhuiyan MAR, Wang LJ. Aerogel incorporated flexible nonwoven fabric for thermal protective clothing. Fire Saf J. 2021;125:8. doi: 10.1016/j.firesaf.2021.103444
- Shaid A, Wang LJ, Fergusson SM, et al. Effect of aerogel incorporation in PCM-Containing thermal liner of firefighting garment. Cloth Text Res J. 2018a;36(3):151–164. doi: 10.1177/0887302X18755464
- Zhao YA, Zeng QT, Lai XJ, et al. Multifunctional cellulose-based aerogel for intelligent fire fighting. Carbohydr Polym. 2023;316:13. doi: 10.1016/j.carbpol.2023.121060
- Zhu XR, He JZ, Wang M. Application research progress in phase change materials for thermal protective clothing. Text Res J. 2022;43:194–202.
- Zhang N. Thermal protection performance of basalt filament/aramid flame-retardant fabric. Tiangong University; 2017.
- Shaid A, Wang LJ, Padhye R, et al. Aerogel nonwoven as reinforcement and batting material for firefighter’s protective clothing: a comparative study. J Sol Gel Sci Techn. 2018b;87(1):95–104. doi: 10.1007/s10971-018-4689-8
- Meena M, Kerketta A, Tripathi M, et al. Moisture barrier layer with supplemental chemical and biological protective functionality for firefighting clothing applications. J Ind Text. 2022;51(4_suppl):6110S–6133S. doi: 10.1177/15280837211073360
- Altay P, Eryürük SH, Özcan G, et al. A new thermal protective clothing design with silica aerogel filled acrylic nanofibers. Int J Cloth Sci Technol. 2023;35(1):32–47. doi: 10.1108/IJCST-12-2020-0185
- Li YW. The test of limiting oxygen index and its influencing factors. Sci Technol Stud. 2014;12:224.
- Shen D, Xu YJ, Long JW, et al. Epoxy resin flame-retarded via a novel melamine-organophosphinic acid salt: thermal stability, flame retardance and pyrolysis behavior. J Anal Appl Pyrolysis. 2017;128:54–63. doi: 10.1016/j.jaap.2017.10.025
- Tan LW, Yao TT, Xia YZ, et al. Preparation and characterization of calcium alginate/nano-TiO, blend fiber. China Synthetic Fiber Indus. 2013;36:6–8+12.
- Wei X. Preparation, structure and properties of PA6/PA66 copolyamide and its fibers. Donghua University; 2022.
- Kong FR, Gu FC, Zhu JZ. Thermal and corrosion resistance properties of aramid 1313 fiberl. J Text Sci Eng. 2016;33(3):109–112.
- Liu Y, Zhang CJ, Zhao JC, et al. Bio-based barium alginate film: preparation, flame retardancy and thermal degradation behavior. Carbohydr Polym. 2016;139:106–114. doi: 10.1016/j.carbpol.2015.12.044
- Xu QL. Design and lmplementation of comprehensive evaluation system based on TOPSIS. J Inf Manag. 2020;5:70–81.
- Behnke WP. Thermal protective performance test for clothing. Fire Technol. 1977;13(1):6–12. doi: 10.1007/BF02338881
- Wanna JT, Tewaeson A. Ignition properties of fabrics. J Fire Sci. 2001;19(6):401–411. doi: 10.1177/073490401773732418
- Giebultowicz J, Ruzycka M, Wroczynski P, et al. Analysis of fire deaths in Poland and influence of smoke toxicity. Forensic Sci Int. 2017;277:77–87. doi: 10.1016/j.forsciint.2017.05.018