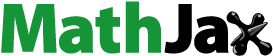
Abstract
Cancer, a global health problem due to its increasing prevalence coupled with high mortality rate. Chemotherapy is an aggressive form of drug therapy. Herbal therapy is safer and healthier to treat specific medical issues. Nigella sativa has been used as traditional medicine for centuries. The crude oil extracted from seed is effective against diseases but vegetative part of N. sativa has always been ignored for its therapeutic potential. Hence, it was hypothesized that vegetative part could be potential antineoplastic source against cancer. Therefore, reactive oxygen species determination and antineoplastic activity were performed against Hep2 and MCF7 cancer cell lines. n-butanol extract of Vegetative Part (VP) caused maximum cell death of Hep2 (58% cell viability). The chloroform VP extract showed 38% cell viability at 10 mg mL-1. The extracts with significant activity were later on validated through GCMS and LC-MS, following literature in PubChem, NIST and Spectrabase libraries.
1. Introduction
Cancer is a major health hazard worldwide. It is the second leading cause of death globally and results in millions of deaths annually [Citation1]. The cancer load is increasing in economically developing countries as a result of ageing and the growth of the population. World cancer report 2014 indicated almost 14.1 million new cases of cancer in 2012 globally [Citation2]. A large number of herbs have been in common practice for cancer therapy since eighteenth century [Citation3]. Anticancer herbs execute therapeutic effects through different mechanisms: inhibit cancer-activating enzymes, stimulate DNA repair, improve the production of protective enzymes and enhance the activity of immune cells [Citation3]. Approximately 35,000 plant species have been screened by National Cancer Institute as potential anticancer plants and about 3000 plant species among them have proved to| be reproducible anticancer [Citation4]. The plant kingdom has been the most authentic source for the extraction of 60% of drugs currently used against cancer treatment [Citation5]. The use of plants is an adventitious process over commercial products because it does not involve the long process of growing cell cultures and is more useful for large-scale synthesis. The biological synthesis is a single-step, cheap and eco-friendly method, which uses bacteria, algae, and plant extracts and has benefits over the other methods as it does not require toxic and hazardous chemicals, high temperature and pressure conditions [Citation6]. Archeological pieces of evidence narrate the cultivation and utilization of N. sativa seeds and oil in the ancient civilizations found all around the Mediterranean Sea [Citation7]. N. sativa of the family Ranunculaceaae has been a significant nutritional additive and natural therapy against many ailments for centuries in ancient systems of medicine such as Unani, Ayurveda, Chinese and Arabic Medicines [Citation8]. Various pharmacological effects of N. sativa (seed/oil) extracts have been identified to include immune stimulation, anti-inflammation, hypoglycemic, antihypertensive, anti-asthmatic, antimicrobial, antiparasitic, antioxidant and anticancer effects [Citation9]. Literature has revealed that studies have been carried out related to the anticancer activities of N. sativa and some of its active compounds, such as thymoquinone and alpha-hederin. The discovery and identification of new antitumour drugs with low side effects on the immune system has become an essential goal in many studies of immuno-pharmacology [Citation10]. Acute and chronic toxicity studies have recently confirmed the safety of N. sativa oil and its most abundant active component, thymoquinone, particularly when given orally. However, the efficacy of the vegetative part of N. sativa against cancer is yet to explore. The present study has been designed to evaluate the antineoplastic potential of vegetative parts of N. sativa in comparison to reproductive one against human cancer Hep-2 and MCF7 cell lines. Hep-2 Cells (Human epithelial type 2) are associated with the epithelial cells (Figure ).
These cells exist under the skin and line the blood vessels, urinary tract and some other organs at the superficial surface. MCF-7 is the Breast Cancer cell line, isolated in 1970. MCF-7 is the acronym of Michigan Cancer Foundation-7. These cells originated from Pleural Effusion and boost Estrogen for cell proliferation and induce primary tumour.
2. Materials and methods
The present study was conducted at the Institute of Biomedical and Genetic Engineering (IBGE) in Islamabad, Pakistan. The experimental design for the current study was a Random Complete Block Design (RCBD).
2.1. Cancer cell line collection and culturing
The human cancer cell lines Hep2 (skin cancer) and MCF-7 (Breast cancer) were provided by the Institute of Biomedical and Genetic Engineering (IBGE), Islamabad. The prerequisites for cell culturing were a suitably ventilated laboratory with a laminar flow hood, incubator, benchtop centrifuge, microscope, plasticware (flasks and plates) and a supply of media with or without serum supplements. The cell lines were cultured in RPMI-1640 media having 10% FBS (Foetal Bovine Serum, Merck, USA). The cells were maintained at 37°C in a humidified atmosphere of 5% CO2 [Citation11].
2.2. Plant extraction
Nigella sativa germplasm was collected from Agri Bioresource Conservation Institute (ABCI), National Agriculture Research Center (NARC) Islamabad. Freshly harvested N. sativa 250 g (VP and RP each) was air-dried and crushed. A voucher specimen was submitted at the herbarium, Lahore College for Women University, Lahore, Pakistan (Voucher No: LCWU-18-196). Crushed plant material was macerated in 750 ml methanol at room temperature for two weeks. The macerated extract was filtered and methanol was evaporated through a rotary evaporator. The residue was further dissolved in distilled water to prepare a stock solution of 200 mg/ml. The partitioning of distilled water extract was done to achieve n-hexane, chloroform, n-butanol and ethyl acetate extracts (100 ml each) (All Sigma Aldrich, USA). All the prepared extracts were dried at room temperature, weighed and dissolved in 10% DMSO/PBS. The stock solutions of all the extracts were further diluted to prepare concentrations such as 20, 10, 5, 2.5, 1.25, 0.6125, 0.3125, 0.1562 mg mL−1 in 10% DMSO/PBS. The prepared extracts were kept at −20°C till used.
2.3. Reactive oxygen species (ROS) determination
Reactive oxygen species determination was done using DPPH radical scavenging, total antioxidant and total phenolic contents assays to check the antiproliferative potential of Nigella sativa extracts.
2.3.1 DPPH (diphenyl picryl hydrazyl radical) assay
Radical scavenging analysis was done by following the methodology of Pang et al. [Citation12] with minor alterations. The preparation of the reaction mixture was done by dissolving 0.2 mg/mL DPPH in DMSO (Sigma Aldrich, USA) in equal concentration to the test sample and kept in dark for half an hour. The scavenging activity in the form of absorption was measured at 517 nm wavelength in a triplicate fashion. The antioxidant activity was expressed as % age of scavenging activity (SC %) through DPPH radical as
Alpha-tocopherol (α-tocopherol, Merck, USA) was used as standard/control at variable concentrations ranging 20–0.1562 mg/mL.
2.3.2 Total antioxidants assay
The total antioxidant potential of the extracts was evaluated according to Li et al. [Citation13] with minor changes. The reaction mixture was prepared by adding 0.1 ml of each extract with 1.9 ml of reagent solution (0.6M sulphuric acid, 4Mm ammonium molybdate and 28Mm sodium phosphate, Sigma Aldrich). It was incubated at 95°C for 60 min and cooled at room temperature. The antioxidant activity was measured at 695 nm wavelength. Standard/control alpha-tocopherol was run at variable concentrations ranging 20–0.1562 mg/mL.
2.3.3 Total phenolic contents (TPCs) assay
Total phenolic contents were quantified by following Dewanto et al. [Citation14]. Each extract (0.1 ml) was mixed with 2.8 ml 10% Na2CO3 and 0.1 ml 2N Folin–Ciocalteu (FC) reagent (Sigma Aldrich) Total phenolic contents of the reaction mixture were measured at 725 nm wavelength using a UV–visible spectrophotometer. TPCs were calculated as ug/g of dry weight by using the Gallic acid standard. Gallic acid equivalent standard calibration curve was prepared at variable concentrations using y = 0.005x + 0.047 equation, where R2 = 0.998). Statistical analysis of all the results was done using Costat software version 6.451.
2.4. Antineoplastic activity analysis
The antineoplastic activity was analysed through an MTT assay and the cell viability of plant extracts was noted. MTT is abbreviated for 3-4,5-Dimethylthiazol-2-yl (Sigma Aldrich, USA) a dye compound.
2.4.1 MTT assay
The assay works on the NADH dehydrogenase mechanism in the mitochondrion of living cells. The breakdown of yellow tetrazolium crystals to form purple Formazan takes place during this colorimetric method. MTT formazan is water-insoluble; thus, it converts into pro-pie needle-shaped crystals in the cells [Citation15].
2.4.2 Cell lines
MCF-7 cells are commonly used breast cancer lines that have been propagated for many years by multiple groups [Citation16]. They prove to be a suitable model cell line for breast cancer investigations worldwide, including those regarding anticancer drugs. MCF-7 cells represent a very important candidate as they are used ubiquitously in research for oestrogen receptor (ER) positive breast cancer cell experiments and many sub-clones, which have been established, represent different classes of ER-positive tumours with varying nuclear receptor expression levels [Citation17].
The Hep-2 cell line was specifically described by H. W. Toolan in 1954. Hep-2 was in fact composed of cervical adenocarcinoma cells, derived via HeLa cell line contamination [Citation18].
2.4.3 RPMI 1640 medium
Ralph et al. [Citation19] developed the RPMI 1640 medium at Rosewell Park Memorial Institute; hence the abbreviation was given as RPMI. The medium formulation was based on the RMPI-1630 series of media utilizing a bicarbonate buffering system and alternations in the amounts of amino acids and vitamins. The RPMI-1640 medium has been used for the culture of normal and neoplastic leucocytes. RPMI-1640, when properly supplemented, has demonstrated wide applicability for supporting the growth of many types of cell cultures, including fresh human lymphocytes in the 72 h phyto hema glutenin (PHA) stimulation assay.
2.4.4 Cell culturing and maintenance
Hep2 and MCF7 cells were cultured in the RPM1 1640 medium with 10% FBS. 100 units/ml penicillin and 100 mg/m1 streptomycin will be used. Sub-culturing of cell lines was maintained at 37°C at 5% CO, in an incubator. Cultures were monitored after 24 and 48 h with repetitive doses [Citation20]. Cell culturing was done in a 96-well plate at 37°C under variable concentrations of Fetal Bovine Serum (FBS, Sigma, USA) for 24 h. In negative control cells were treated with a medium only. The supernatant of each well will be removed and cells were washed twice with Phosphate Buffer Saline (PBS, Merck, USA). Then the cells were introduced to a 20-μl MTT solution (5 mg m1-I in PBS). Later on cells were incubated for 4 h to form formazan crystals. The resultant formazan crystals were dissolved in dimethyl sulphoxide (100 l).
2.4.5 Measurement of absorbance
The absorbance intensity was measured at 490 nm through a micro-plate reader (Bio-Rad 680 USA). The experiment was laid out in RCBD and percentage cell viability was taken relative to the untreated control cells [Citation21]. Statistical analysis of all the results was done using the Costat software version 6.451.
The following formula was used to measure absorbance:
where At is the absorbance value of tested plant extract; Ab is the absorbance value of blank; Ac is the absorbance value of negative control (untreated cell).
2.4.6 Cell viability assay
To evaluate the effect of plant extracts on cell viability, cells were exposed to the extract at concentrations producing 50% growth inhibition (IC50) for cancer cell lines (as determined by the MTT Assay) for 24-h and 48-h intervals [Citation22]. The percentage of dead cells was calculated from the ratio of dead cells to the total number of cells multiplied by 100.
2.5. GC-MS analysis
GC-MS analysis of all the potential extracts against certain activities was done by following the methodology of Derwich et al. [Citation23]. For all the fractions trace GC (ULTRA S/N 20062969, Thermo Fischer) equipped with HP-5MS a non-polar fused silica capillary column (30 m × 0.32 mm, film thickness 0.25 µm) was used. The operating conditions were maintained such as the oven temperature programme from 50°C (2 min) to 260°C at 4°C/min heating rate and held for 2 min, split injection ratio 1:20, carrier gas helium, flow rate 1 mL/min, the temperature of injector and detector (FID) fixed at 260°C and 280°C. The constituents were identified depending upon their Kovats Index, calculated in relation to the retention time as reference products, in comparison with those of the chemical compounds gathered by the similarity of their mass spectra with those gathered in the NIST-MS library or reported in the literature.
2.6. LC-MS analysis
LC-MS analysis was performed for the separation of compounds from the chloroform extract of Nigella sativa seeds. The obtained chromatogram was observed to have compounds a, b, c and d. For the identification of ions full Total Ion Chromatograms (TIC) scan in positive mode was obtained. All the analytes were eluded within 32 min. An overview of the TIC scan of both extracts showed that the pattern of peaks eluded was the same. The time a solute spends in the column or the time spent by a solute in non-polar stationery and the polar mobile phase is known as the retention time. Various components in the solution possess various flow velocities due to physiochemical properties [Citation24].
3. Results
3.1. Reactive oxygen species determination
3.1.1. DPPH radical scavenging activity assay
It is the most widely used method to analyse the scavenging ability of plants. Radical scavenging activity for both vegetative and reproductive plant parts was found to increase with the increasing concentrations (Figure ). N. sativa VP methanolic extract and fractions showed the results quite comparable with the standard (α-tocopherol) used. At 20 mg/mL distilled water RP extract was stronger (99 ± 0.5cd) than VP (97 ± 0.5c) but with a very minor difference (Figure ). In the case of hexane fraction RP showed (89 ± 0.5a) more efficiency towards free radical scavenging than VP (76 ± 1bc) at 20 mg/mL. Likewise, chloroform, butanol and ethyl acetate extracts of RP fractions showed more scavenging potential for free radical production as compared to VP. Among all, VP methanolic extract and its fractions showed the maximum efficiency towards free radical production, while hexane extracts were the mildest. In the case of RP, ethyl acetate extract was the strongest one, whereas the butanol extract and dilutions showed their least potential. IC50 values of the extracts were also noted. The IC50 value for distilled water parental extract was 0.3125 mg/mL, for chloroform extract again 0.3125 mg/mL and for butanol extract it was between 5 and 10 mg/mL. In the case of RP distilled water, chloroform, butanol and n-hexane all gave their IC 50 value approximately at 3.125 mg/mL.
3.1.2. Total antioxidant assay
The total antioxidants were noticed to increase with the increasing concentrations among all the extracts just as in the case of the standard @ tocopherol with the increasing concentration (Figure ) VP methanolic extract formed maximum total antioxidants at 20 mg/mL (1.44 ± 0.05e) among all the extracts and fractions, whereas distilled water VP produced least free antioxidants (0.02 ± 0.01a) at 0.156 mg/mL. Methanol extract of VP (1.44 ± 0.05e) was found more efficient than that of RP (0.98 ± 0.03bc) at 20 mg/mL. RP extracts were found more competent for antioxidant production than VP extracts (Figure ). It was noticed that n-butanol extracts (20- 0.1562 mg/mL) of both VP and RP were more antioxidants producing than the standard (@-tocopherol) used.
3.1.3. Total phenolic content assay
Total phenolic contents were determined spectrophotometrically according to the F.C. The Colorimetric method uses Gallic acid as the standard. Total Phenolic contents increased with the increasing concentration of the extracts (Figure ). Gallic acid was used to standardize the equation and for the determination of the total phenolic contents of extracts. Ethyl acetate VP extract exhibited maximum phenolic contents (616 ± 0.03c) at 20 mg/mL among all VP extracts and hexane was at the minimum with 300 ± 0.15a ug/g phenolic contents at 0.156 mg/mL concentration. In the case of RP extracts at 20 mg/ml, distilled water extract showed maximum phenolic contents (623 ± 0.03d) among all followed by ethyl acetate extract (612 ± 0.03cd) (Figure ). Hexane VP extract was found the mildest (300 ± 0.15a) for the production of phenolic contents (Figure and Table ).
Table 1. Standard α-tocopherol against DPPH radical scavenging activity and total antioxidant determination.
3.2. Antineoplastic activity analysis
The antineoplastic activity of N. sativa extracts was employed through MTT colorimetric Assay. Human Cancer cell lines Hep2 and MCF-7 were used to check the anticancer potential of the prepared extracts. Antineoplastic activities of hexane, chloroform, methanol, butanol, ethyl acetate and distilled water extracts ranged from 10 to 0.1562 mg/mL and were recorded after 24 and 48 h of intervals. Percentage cell viability was inversely proportional to the plant extract concentration. As the concentration of the extracts increased there was a significant decrease in the cell viability of cancer cells (Figures and ). The results showed that n-butanol extract of VP at 10 mg/ml caused maximum death of Hep2 cancer cells at 24 h intervals (58% cell viability) (Figure ). The trend of the anticancerous potential of other VP and RP extract after 24 h intervals was as chloroform VP extract (60%) >n-butanol RP extract (62%) > n-hexane RP extract (63%). Whereas n-butanol RP extract (10 mg/ml) showed the best results against Hep2 cancer cell lines at 48 h intervals (33% cell viability) followed by n-hexane VP extract (37%) and chloroform RP extract (38%), as presented in Figure . The IC50 value of chloroform and n-hexane VP extracts was 2.5 mg/mL, likewise, chloroform and n-butanol extracts showed 50% cell death at 2.5 mg/ml in Hep2 Cancer cells after 48 h intervals. In the present study it was observed that the chloroform extract of the VP part showed its activity against the MCF7 cancer cell line, whereas all other extracts remained inactive (Figure ). Similarly, another study conducted by [Citation25] noticed the same results in which different concentrations such as 2.5, 10 and 12.5 mg/mL showed their activity against MCF7 cancer cell lines. MCF7 VP chloroform extract was stronger (38% cell viability) at 10 mg/mL after 48 h intervals among all the VP and RP extracts. The IC50 value for Taxol was used as a positive control against Hep2 cancer cell lines, as it is a recommended medicine for cancer treatment and it showed its IC50 was recorded as 2.5 mg/mL. Likewise, Fulvestrant was used against MCF-7 cell lines and its IC50 value was also noted as 2.5 mg/mL, as mentioned in Figure . The effect of this medicine is just equal to chloroform and n-hexane VP extract (Figures ). The extracts having significant antineoplastic activity were later on analysed through GC-MS analysis to generate their phytochemical profile reported in Figures . GC-MS results indicated the presence of (α)-hederin, a pentacyclic triterpene saponin most abundantly thymoquinone in the extract of vegetative parts (VP). The proposed molecular mechanism of N. sativa action against Cancer is presented in Figure . Cancer cells are metabolically more active than normal cells thus showing their uncontrolled cell division.
Figure 5. Percentage cell viability of different extracts of N. sativa against Hep 2 cancer cell lines at 24 h intervals at p ≤ 0.05.

Figure 6. Percentage cell viability of different extracts of N. sativa against Hep 2 cancer cell lines at 48 h intervals at p ≤ 0.05.

Figure 7. Percentage cell viability of chloroform extracts of N. sativa against MCF 7 cancer cell line at p ≤ 0.05.

3.3. Putative active component identification through GC-MS analysis
The mass spectrometer analyses the compounds eluted at different times to identify the nature and structure of the compounds. The larger compounds broke into smaller ones by producing various peaks at variable retention times. Ethyl acetate and butanol extracts of vegetative parts showed the most significant results against the Hep 2 cancer cell line, thus both the extracts were checked for their chemical constituents through GC-MS analysis. Butanol fraction from methanolic extract showed significant cytotoxic activity and its GCMS analysis indicated the presence of Pentadecanoic acid, Octadecadienoic acid, 6, Pentadecen-1-ol, 9,12-Octadecadiene-1-ol and Di-n-octyl phthalate, as mentioned in Table . GCMS analysis of Ethyl acetate extract showed the presence of 1,2,3,4,-Butanetetrol, 2,Dodecenal, Pentadecanoic acid, 10, Octadecanoic acid and Stearic acid is given in Table . The n-Hexane fraction indicated Palmitic acid, Hexane decanoic acid, Octadecadienoic acid, Linolenic acid, Octa decenoic acid, Octadecanoic acid, Eicosadienoic acid, Glycerol linoleate and Phthalic, acid as illustrated in Table ).
Table 2. Phyto-constituents identified from hexane fraction of Nigella sativa L.
Table 3 Phyto-constituents identified from ethyl acetate Fraction of Nigella sativa.
Table 4. Phyto-constituents identified from n-butanolic fraction of Nigella sativa through GC-MS.
3.4. LC-MS analysis
LC-MS was performed for the separation of compounds from the chloroform extract of Nigella sativa seeds. The obtained chromatogram was observed to have compounds a, b, c and d. The mass spectra of all the compounds were observed as their mass-to-charge ratio (m/z) and retention time. Compound a was observed to have a sharp peak at 651.02 m/z. The compounds’ spectra were similar to the spectra available for Cholest-5-en-3-ol (3. beta.)-, (9Z)−9-octadecenoate in PubChem, NIST and Spectrabase. Compound a was identified at the retention time of 22.608 min. It has been reported in acetonitrile extract of Fusarium venenatum at 200°C source temperature with a retention time of 2.7 min [Citation26]. Compound b was observed to have a sharp peak at 677.1 m/z. Compound b spectra were similar to the spectra available for (7R)−4-Hydroxy-N,N,N-trimethyl-10-oxo-7-(tetradecanoyloxy)−3,5,9-trioxa-4-phosphatricosan-1-aminium 4-oxide also known as a phospholipid 1,2-Dimyristoyl-sn-glycero-3-phosphocholine documented to form a micelle. It is used to increase the solubility of a drug in the aqueous environment of the gastrointestinal tract and blood. DL-dimyristoyl phosphatidylcholine is soluble in water and it is an active ingredient in the production of Amphotericin B lipid complex (antifungal) [Citation27]; https://www.fda.gov/drugs/drug-approvals-and-databases/national-drug-code-directory). Compound c was identified as 1, 2-Dilinoleoyl-sn-glycero-3-phosphoethanolamine with 739 m/z. 1, 2-Dilinoleoyl-sn-glycero-3-phosphoethanolamine is a secondary metabolite and documented to ameliorate dementia without any negative impact on motor activities [Citation28]. Compound d is identified as Pepstain A with 685 m/z. Pepstatin A has been identified as an antitumour drug (Table , Figures and [Citation29]).
Figure 12. (a) TIC scan of chloroform extract of Nigella sativa. (b) TIC scan of butanol extract of Nigella sativa.

Table 5. Phyto-constituents identified from n-butanolic fraction of Nigella sativa through LC-MS.
Compound a was observed to have a sharp peak at 651 m/z. The compounds spectra were similar to the spectra available for Cholest-5-en-3-ol (3. beta.)-, (9Z)−9-octadecenoate in PubChem, NIST and Spectrabase. Compound a was identified at a retention time of 22.731 min. Compound b spectra were similar to the spectra available for 1,2-didodecanoyl-sn-glycero-3-phospho-(1'-sn-glycerol) in PubChem and NIST 20. The retention time was 30.25 min. Adansonia digitata L. commonly known as Sudanese baobab is among African traditional medicinal plants. Ibrahim and Musa [Citation30] performed LC-MS/MS of methanol extract from fruit pulp. A total of 52 compounds were found in fruit pulp extract. Among 52 compounds 1,2-didodecanoyl-sn-glycero-3-phospho-(1'-sn-glycerol) was also reported. Compound c was identified as 6-Hydroxy-6-(hydroxymethyl)−8-(2,3,4-trimethoxyphenyl)−7,8-dihydro-[1,3]dioxolo[4,5-g]chromene-7-carboxylic-acid;3-hydroxy-8-(2,3,4-trimethoxyphenyl)−2,5,12,14- tetraoxatetracyclo[7.7.0.03,7.011,15]hexadeca-1(16),9,11(15)-trien-6-one. The compound was observed to inhibit the growth of HOP Human Non-Small Cell Lung tumour cell line, Non-Small Cell Lung tumour and 273 Small Cell Lung cell line by the national cancer institute (NCI) (Figure , Tables and ).
Table 6. Phyto-constituents identified from n-butanolic fraction of Nigella sativa through LC-MS.
Table 7. NCI library data and reference IDs against cancer cell lines.
4. Discussion
Although the anti-cancer activity of N. sativa components was recognized thousands of years ago proper scientific research with this important traditional medicine is a history of the last 2∼3 decades. Not so much research work has been done on this important traditional medicine and very few reports exist in the scientific database [Citation31]. Most of the members of the family Ranunculaceae possess acetin which shows inhibition towards human Hep 2 cancer cell growth [Citation32]. The formulation of herbal extracts has gained huge attention to prevent evaporation, degradation of the active components and controlled release of the extract [Citation33]. The present investigation narrated that n-butanol extract showed maximum cytotoxicity effect against Hep2 cancer cells after 24- and 48-h intervals. It has been reported that alpha (α)-hederin, a pentacyclic triterpene saponin (n-butanol soluble) of N. sativa has the potential against in vivo antitumour activity [Citation34]. Another member of the Nigella genus, N. monosperma has attributed in vitro anticancer activity against colorectal and breast cancer cell lines [Citation35]. Plants rich in oleic acid and Palmitic acid cause cytotoxicity in carcinogenic cells [Citation36]. Anti-cancer activity of any compound may be either by killing the cancer cells or mutating the genetic material of the normal cells [Citation37].
The herbal extracts are composed of various phytochemicals, many of which are considered bioactive agents [Citation38]. Moreover, herbal extracts contain secondary metabolites with anti-microbial, anti-cancer, anti-inflammatory and anti-oxidant effects [Citation39]. In recent years, natural products, such as herbal extracts, have attracted the attention of many researchers as alternative anti-cancer agents [Citation33]. Similarly, N. sativa has been reported to enhance the efficacy of antioxidant enzymes such as superoxide dismutase, catalase and glutathione peroxidase to fight against various cancer types [Citation40]. It has been assumed that oral intake of N. sativa oil or TQ can also lower the toxicity of other anticancer drugs (for example, cyclophosphamide) by an up-regulation of antioxidant enzymes and minimize the toxic effects of treatment with anticancer drugs [Citation41]. It has been noticed that oral intake of N. sativa helps the body to systematically eliminate old and unhealthy cells (cancer cells) without releasing toxins into the body [Citation42].
N. sativa plant has very strong phytochemical potential due to the presence of certain compounds and thus significant healing characteristics. Muscle strength, body immunity, blood sugar regulation and rheumatism are controlled and regulated by Linoleic acid. Phthalic acid is meant for foetal health and improved functions of the reproductive system [Citation43]. Most of the dermatological issues are being resolved by Stearic acid. Palmitic acid has strong antioxidant properties thus controlling ageing and cardiovascular risk.
Thymoquinone (TQ) possess anti-neoplastic, antioxidant, anti-inflammatory, immunomodulatory, antimutagenic and anti-diabetic properties [Citation44]. It was also found that TQ possesses antiproliferative properties and enhanced apoptosis of human cancer cells. Salih et al. [Citation45] suggested that the antineoplastic activity of N. sativa may be associated with the vigorous increase of cyclin-dependent kinase inhibitor p16 and a decrease in cyclin D1 protein expression. The protein expression causes an increase in the expression of the tumour-suppressing protein p53. It has been revealed that thymoquinone-induced apoptosis results in suppressed protein expression.
Natural products-derived drugs have led to the exploration of a variety of terpenoids [Citation46]. Terpenoids inhibit cancer cell proliferation and metastasis under the ADP ribose polymerase cleavage mechanism [Citation47]. The vegetative part of N. sativa is a rich source of mono, di and triterpenes, which, are soluble in chloroform, could be a potential source to eradicate human breast cancer cells MCF 7 as in the present study (Figures and ). Similarly, MCF7 cell lines testing was done in the study of Shams and Ghafouri [Citation48]. Terpenoids have been demonstrated to suppress the growth of the liver, stomach, colon and skin cancer [Citation46]. In a previous study terpene-terminated 6-alkyl residues of TQ were tested in MCF-7/Topo breast carcinoma and found that the derivatives induced cell death by apoptosis [Citation49]. It was studied that the antiproliferative and cytotoxic activities of phenolic compounds depend on the number of OH-ring substituents. As OH-ring substituents increase, their antiproliferative and cytotoxic activities also increase [Citation50].
Dermal application of N. sativa extract could slow down skin carcinogenesis in experimental models. The intraperitoneal administration of N. sativa (100 mg/kg body weight) restricted soft tissue sarcomas to 33.3% [Citation21,Citation51]. Another supportive study conducted by Khan et al. [Citation42] concluded that intra-peritoneal administration of N. sativa (100 mg/kg body weight) 30 days after subcutaneous administration of MCA (20-methylcholanthrene) restricted soft tissue sarcomas to 33.3% compared with 100% in MCA-treated controls. The vegetative part of N. sativa extracts was reported to possess 50–69% linolenic acid. Fabian et al. [Citation52] narrated that linoleic acid plays important role in cell membrane structure, fluidity and cell signalling to prevent cancer. N. sativa (Thymoquinone) is responsible for controlling certain anti-apoptotic genes such as Caspase-3,8,9 bax, Bcl2 and control their pathways to check the overexpression oncogenes (Woo et al. [Citation1]). In LC-MS Each peak was extracted from TIC as extracted ion chromatogram (EIC) which showed that the intensities of the peak were different at the same position. MS spectra were obtained for each compound by using the individual data acquisition (IDA) method. Temperature is an important factor for all modes of chromatography as it influences the viscosity of the mobile phase. An increase in temperature causes a decrease in the viscosity of the liquid. Therefore, the temperature also influences the solute partitioning (between mobile and stationary phase) and chromatogram retention [Citation53]. Distribution of solute between both phases (mobile and stationary) depends on the solubility ability in the liquid phase and adsorption of the solid phase, respectively. The distribution ratio is designated as K and is defined as the ratio of the concentration of solute in the mobile phase to the ratio in the stationary phase. The greater the ratio (k) the higher the retention time. Therefore, the column temperature is responsible for both the overall flow rate and retention of the individual metabolite. The data presented in tables and figures are highly related to the anti-cancerous compounds. Many studies showed that N. sativa oil has antioxidant activity and increases the activities of antioxidant enzymes such as superoxide dismutase (SOD), catalase (CAT) glutathione peroxidase [Citation54]. The antioxidant enzymes are clearly related to cancer, mostly their increased activities are beneficial against different types of cancer and the compounds such as 1,2-Dimyristoyl-sn-glycero-3-phosphocholine, Cholest-5-en-3-ol (3.beta.)-, (9Z)−9-octadecenoate and Pepstatin A; identified through LC-MS analysis are human metabolites (Pubchem) and antioxidant as well. Based on the results and analysis of GCMS and LCMS data vegetative part extractions are highly recommended as anticancer pharmaceutical.
5. Conclusion
Cancer is a major lethal problem worldwide. Various synthetic drugs have been used to cure cancer but they impart hazardous side effects on adjacent normal healthy cells. Medicinal plants have largely contributed to human health as they contain various secondary metabolites showing therapeutic potential for diseases such as diabetes, cardiac diseases, hepatitis and cancer. N. sativa plant in the present study showed significant cytotoxic effects against cancer cell lines and their anticancer constituents profiling through general phytochemical analysis and GC-MS analysis confirmed the presence of anticancer compounds such as tannins, terpenoids and certain acids. Hence it is concluded that the vegetative part of N. sativa has significant anticancer potential and is much safer than the conventionally used reproductive parts such as seed and oil .
Disclosure statement
No potential conflict of interest was reported by the author(s).
References
- Woo C, Hsu A, Kumar A, et al. Thymoquinone inhibits tumor growth and induces apoptosis in a breast cancer xenograft mouse model: the role of p38 MAPK and ROS. Peer-rev Open Acces Sci J. 2013;8(10):1–14. doi:10.1371/journal.pone.0075356.
- Stewart BW, Wild CP. World Cancer Report. IARC Publications; 2014.
- McGrowder DA, Miller FG, Nwokocha CR, et al. Medicinal herbs used in traditional management of breast cancer: mechanisms of action. Medicines. 2020;7(8):47, doi:10.3390/medicines7080047.
- Kuruppu A, Paranagama P, Goonasekara C. Medicinal plants commonly used against cancer in traditional medicine formulae in Sri Lanka. Saudi Pharm J. 2019;27(4):565–573. doi:10.1016/j.jsps.2019.02.004.
- Gordaliza M. Natural products as leads to anticancer drugs. Clin Transl Oncol. 2007;9(12):767–776. doi:10.1007/s12094-007-0138-9.
- Krishnan V, Bupesh G, Manikandan E, et al. Green synthesis of silver nanoparticles using Piper nigrum concoction and its anticancer activity against MCF-7 and Hep-2 cell lines. J Antimicrob Chemother. 2016;2(3):2472–1212. DOI: 10.4172/2472-1212.1000123.
- Ahmad A, Husain A, Mujeeb M, et al. A review on therapeutic potential of Nigella sativa: a miracle herb. Asian Pac J Trop Biomed. 2013;3(5):337–352. doi:10.1016/s2221-1691(13)60075-1.
- Khan A, Chen HC, Tania M, et al. Anticancer activities of Nigella sativa (black cumin). Afr J Trad, Complement Altern Med. 2011;8(5S):671–678. doi:10.4314/ajtcam.v8i5SS.10.
- Randhawa MA, Alghamdi MS. Anticancer activity of Nigella sativa (black seed)—a review. Am J Chin Med. 2011;39(06):1075–1091. doi:10.1142/S0192415X1100941X.
- Venugopal K, Bupesh G, Manikandan E, et al. Green synthesis of silver nanoparticles using Piper nigrum concoction and its anticancer activity against MCF-7 and Hep-2 cell lines. J Antimicrob Chemother. 2016;2:3, doi:10.4172/2472-1212.1000123.
- Mirabelli P, Luigi C, Marco S. Cancer cell lines are useful model systems for medical research. Cancers (Basel). 2019;11(8):1098, doi:10.3390/cancers11081098.
- Pang Y, Ahmed S, Xu Y, et al. Bound phenolic compounds and antioxidant properties of whole grain and bran of white, red and black rice. Food Chem. 2018;240:212–221. doi:10.1016/j.foodchem.2017.07.095.
- Li A-N, Li S, Zhang Y-J, et al. Resources and biological activities of natural polyphenols. Nutrients. 2014;6:6020–6047. doi:10.3390/nu6126020.
- Dewanto V, Wu X, Adom KK, et al. Thermal processing enhances the nutritional value of tomatoes by increasing total antioxidant activity. J Agric Food Chem. 2002;50(10):3010–3014. doi:10.1021/jf0115589.
- Barkat M, Harshita Pottoo F, Beg S, et al. Evidence-based review on clinical potential of thymoquinone in breast cancer. Nanomed Bioactiv. 2020;17(1):471–486. doi:10.1007/978-981-15-1664-1_19.
- Dai X, Cheng H, Bai Z, et al. Breast cancer cell line classification and its relevance with breast tumor subtyping. J Cancer. 2017;8(16):3131–3141. doi:10.7150/jca.18457.
- Sweeney EE, Mcdaniel RE, Maximov PY, et al. Models and mechanisms of acquired antihormone resistance in breast cancer: significant clinical progress despite limitations. Horm Mol Biol Clin Investig. 2013;9:143–163. doi:10.1515/hmbci-2011-0004.
- Korch C, Capes-Davis A. The extensive and expensive impacts of HEp-2 [HeLa], intestine 407 [HeLa], and other false cell lines in journal publications. SLAS Discovery. 2021;26(10):1268–1279. doi:10.1177/24725552211051963.
- Ralph PETER, Moore MA, Nilsson K. Lysozyme synthesis by established human and murine histiocytic lymphoma cell lines. J Exp Med. 1976;143(6):1528–1533. doi:10.1084/jem.143.6.1528.
- Rubinstein LV, Shoemaker RH, Paull KD, et al. Comparison of in vitro anticancer-drug-screening data generated with a tetrazolium assay versus a protein assay against a diverse panel of human tumor cell lines. J Natl Cancer Inst. 1990;82(13):1113–1117. doi:10.1093/jnci/82.13.1113.
- Shahraki S, Khajavirad A, Shafei MN, et al. Effect of total hydroalcholic extract of Nigella sativa and its n-hexane and ethyl acetate fractions on ACHN and GP-293 cell lines. J Tradit Complement Med. 2016;6:89–96. doi:10.1016/j.jtcme.2014.11.018.
- Soltanian S, Mohamadi N, Sheikhbahaei M. Cytotoxicity evaluation of methanol extracts of some medicinal plants on P19 embryonal carcinoma cells. J Appl Pharm Sci. 2017;21(12):1801–1818. doi:10.7324/japs.2017.70722.
- Derwich E, Benziane Z, Boukir A. GC/MS Analysis and Antibacterial Activity of the Essential Oil of Mentha pulegium Grown in Morocco. Res J. Agric Biol Sci. 2010;6:191–198.
- Grimes BA, Liapis AI. Modeling and analysis of the electrokinetic mass transport and adsorption mechanisms of a charged adsorbate in capillary electrochromatography systems employing charged nonporous adsorbent particles. J Colloid Interface Sci. 2001;234(1):223–243.
- Aslany S, Tafvizi F, Naseh V. Characterization and evaluation of cytotoxic and apoptotic effects of green synthesis of silver nanoparticles using Artemisia Ciniformis on human gastric adenocarcinoma. Mater Today Commun. 2020;24:101011, doi:10.1016/j.mtcomm.2020.101011.
- Thomas AB, Shetane TD, Singha RG, et al. Employing central composite design for evaluation of biomass production by Fusarium venenatum: in vivo antioxidant and antihyperlipidemic properties. Appl Biochem Biotechnol. 2017;183(1):91–109.
- Ikeda A, Mori M, Kiguchi K, et al. Advantages and potential of lipid-membrane-incorporating fullerenes prepared by the fullerene-exchange method. Chem – Asian J. 2012;7(3):605–613.
- Yaguchi T, Nagata T, Nishizaki T. 1, 2-Dilinoleoyl-sn-glycero-3-phosphoethanolamine ameliorates age-related spatial memory deterioration by preventing neuronal cell death. Behav Brain Funct. 2010;6(1):1–9.
- Cheong H, Lu C, Lindsten T, et al. Therapeutic targets in cancer cell metabolism and autophagy. Nat Biotechnol . 2012;30:671–678.
- Ibrahim A, Musa B. Liquid Chromatography Mass Spectrometer (Lc/Ms) profile revealed flavonoids and terpenoids with antioxidant potential in aqueous fraction of Combretum micranthum leaf extract. Zanco J. Pure Appl Sci. 2021;7(4):236–247.
- Khan MA, Tania M, Zhang D-Z, et al. Antioxidant enzymes and cancer. Chin J Canc Res. 2011;22(2):87–92. doi:10.1016/j.biopha.2017.09.115.
- Einbonda LS, Soffritti M, Esposti DD, et al. Actein activates stress and statin associated responses and is bioavailable in Sprague-Dawley rats. Fund Clin Pharmacol. 2009;23:311–321. doi:10.1111/j.1472-8206.2009.00673.x.
- Haghighi T, Saharkhiz M. Phytotoxic potential of Vitex pseudo-negundo leaf and flower extracts and analysis of phenolic compounds. Biocatal Agr Biotechn. 2021;34:102018, doi:10.1016/j.bcab.2021.102018.
- Swamy SM, Huat BT. Intracellular glutathione depletion and reactive oxygen species generation are important in alpha-hederin-induced apoptosis of P388 cells. Mol Cell Biochem. 2003;245:127–139. doi:10.1023/a:1022807207948.
- Solowey E, Lichtenstein M, Sallon S, et al. Evaluating medicinal plants for anticancer activity. Sci World J. 2014;6:1–12. doi:10.1155/2014/721402.
- Cordier W, Steenkamp P, Steenkamp V. Extracts of Moringa oleifera lack cytotoxicity and attenuate oleic acid-induced steatosis in an in vitro HepG2 model. S Afr J Bot. 2020;129:123–133. doi:10.1016/j.sajb.2019.03.001.
- Majdalawieh A, Fayyad M. Recent advances on the anti-cancer properties of Nigella sativa, a widely used food additive. J Ayurveda Integr Med. 2016;7(3):173–180. doi:10.1016/j.jaim.2016.07.004.
- Van-Nguyen-Thien T, Huynh V, Vo L, et al. Two new compounds and α-glucosidase inhibitors from the leaves of Bidens pilosa L. Phytochem Lett. 2017;20:119–122. doi:10.1016/j.phytol.2017.04.015.
- Seca AML, Pinto D. Plant secondary metabolites as anticancer agents: successes in clinical trials and therapeutic application. Int J Mol Sci. 2018;19(1):368–375. doi:10.3390/ijms19010263.
- Greenwell M, Rahman PKSM. Medicinal plants: their use in anticancer treatment. Int J Pharm Sci Res. 2015;6(10):4103), doi:10.13040/IJPSR.0975-8232.6(10).4103-12.
- Alenzi FQ, El-Bolkiny YS, Salem ML. Protective effects of Nigella sativa oil and thymoquinone against toxicity induced by the anticancer drug cyclophosphamide. British J Biomed Sci. 2010;67(1):20–28. doi:10.1080/09674845.2010.11730285.
- Khan MA, Chen HA, Mousumi T, et al. Anticancer activities of Nigella sativa (black cumin) African. J Trad Complem Alternat Med. 2011;8(5 Suppl):226–232. DOI: 10.4314/ajtcam.v8i5S.10
- Bajkin Branislav, Selakovic Srecko, Mirkovic Sinisa, etal. Comparison of efficacy of local hemostatic modalities in anticoagulated patients undergoing tooth extractions. Vojnosanitetski pregled. 2014;71(12):1097–1101. https://doi.org/10.2298/VSP1412097B.
- Amin FM, Muneera W, Fayyad T. Recent advances on the anti-cancer properties of Nigella sativa, a widely used food additive. J Ayurveda Integr Med. 2016;7:173–180. doi:10.1016/j.jaim.2016.07.004.
- Salih HM, Aljabre O, Alakloby M. Dermatological effects of Nigella sativa. J Dermat Derm Surv. 2015;19(2):92–98. doi:10.1016/j.jdds.2015.04.002
- Huang M, Lu JJ, Huang MQ, et al. Terpenoids: natural products for cancer therapy. Expert Opin Invest Drugs. 2012;21(12):1801–1818. doi:10.1517/13543784.2012.727395.
- Guesmi F, Prasad S, Tyagi AK, et al. Antinflammatory and anticancer effects of terpenes from oily fractions of Teucruimalopecurus, blocker of IκBα kinase, through downregulation of NF-κB activation, potentiation of apoptosis and suppression of NF-κB-regulated gene expression. Biomed Pharmaco. 2017;9(5):1876–1885. doi:10.1016/j.biopha.2017.09.115.
- Shams R, Ghafouri-Fard S. No association between expression of RAS Guanyl releasing protein 3 (RASGRP3) in breast cancer and clinicopathological data. Int J Cancer Manage. 2018;11(9) DOI: 10.5812/ijcm.69551,
- Effenberger K, Breyer S, Schobert R. Terpene conjugates of the Nigella sativa seed-oil constituent thymoquinone with enhanced efficacy in cancer cells. Chemical Biodiversity. 2010;7:129–139.
- Tesfaye T, Ravichadran YD. A review on anticancer activity of some plant-derived compounds and their mode of action. Nat Prod Chem Res. 2018;6(4):2–9.
- Shakya AK. Medicinal plants: future source of new drugs. Int J Herb Med. 2016;4:59–64. Potential of N. sativa. Ethnobotanical Review, 13: 946–955. DOI:10.13140/RG.2.1.1395.6085.
- Fabian CJ, Bruce FK, Stephen DH. Omega-3 fatty acids for breast cancer prevention and survivorship. Breast Cancer Res. 2015;17(1):62, 39(6):1075–1091. doi:10.1186/s13058-015-0571-6.
- Djordjevic NM, Houdiere F, Fowler P. High temperature and temperature programming in capillary HPLC. Biomed Chromatogr. 2008;12(3):153–154.
- Ismail M, Al-Naqeep G, Chan KW. Nigella sativa thymoquinone-rich fraction greatly improves plasma antioxidant capacity and expression of antioxidant genes in hypercholesterolemic rats. Free Radical Biology and Medicine. 2010;48(5):664–672.