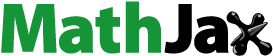
Abstract
A series of 4-amino-5-substituted-thiophene derivatives 3, 5 and 7, along with their corresponding thieno[3,2-d]pyrimidine compounds 4, 6 and 8, were synthesized and characterized. DFT calculations were utilized to determine frontier orbitals energies. The data showed that the compounds have low HOMO and LUMO energies, where 4-methyl thienopyrimidine 4 and 5 have the lowest values. According to results of antibacterial activity against two distinct strains of Gram positive and negative bacteria through two different concentrations (0.5 and 1.0 mg/mL). Compounds 6 and 8 show (IC50 = 43.53±3.26, 45.64±3.42 and 39.72±2.98, 42.57±3.19 mg/mL) good action toward S. aureus compared to chloramphenicol. Meanwhile, cytotoxic activity for inspected derivatives was evaluated toward three cancer cell lines. The investigated thiophene derivatives 4, 6 and 7 were displayed potent cytotoxic effectiveness (14.53±0.54, 11.17±0.42, 16.76±0.63 μM) against MCF-7 versus 5-fluorouracil as standard drug. In addition, the theoretical study such as molecular docking was stimulated.
1. Introduction
Population growth has been negatively impacted by infection control that can be fatal and brought on by microbes decades ago [Citation1]. Besides, contributing to the rise in infection rates and death, microbial fighting too increased the cost of health care associated with treating illnesses that are reluctant to antibiotics [Citation2]. The second-leading cause of death in human beings and one of the major worldwide health issues is cancer [Citation3,Citation4]. The dispersal and death rate of cancer continue to be high regardless of significant breakthroughs in cancer therapy. Recently, several researchers focus on finding cancer treatments that are more efficient and less harmful [Citation5–14]. Meanwhile, the discovery of new drugs for apoptosis, thymidylate synthase and NEK7 protein inhibitors, is heavily relying on various heterocyclic molecules, such as benzimidazole, 1,3,4-oxadiazole, benzo[f]chromene-2-carbonitrile, 1,2,3-Triazole, pyridine and pyrimidine [Citation15–27]. Due to its notable variation in biological effectiveness, thiophene and its products have emerged as prominent scaffolding that has attracted the attention of scientists in the domain of pharmacological applications (). The advantages of the thiophene ring have been demonstrated by the successful development of its analogues, which include antioxidant [Citation28–30], anti-inflammatory [Citation31], antibacterial [Citation32,Citation33] and anticancer effectiveness [Citation34–37].
However, thiophenes’ biological activity was greatly influenced by the type and location of the substitutions [Citation38]. Structure and activity correlation for the thiophene analogues demonstrated an outstanding comprehension of the thiophene ring in the cancer treatment of various cancer cells [Citation39]. The thiophene analogues play a significant role in the development of agents that efficiently capture the carcinogenic lead compounds inside living cells [Citation40,Citation41]. Thiophene’s role in inhibiting “topoisomerase, tyrosine kinase, tubulin interaction, and inducing apoptosis” by activating reactive oxygen species is among the key anticancer processes discovered [Citation42]. Furthermore, numerous fused pyrimidine skeletons have been created and prepared to serve as drug-like nominees for various pharmacological purposes [Citation43]. Many studies in medicinal chemistry have exploited pyrimidines and purine-like structures as curative agents meanwhile it was discovered that they are present as a main component in some nucleic acids [Citation22]. Purines, pyrimidines and other fused cyclic molecules like pteridines are displayed interesting biological effectiveness owing to their chemical structures. Amongst the pyrimidine hybrids, thieno[2,3-d]pyrimidine derivatives reveal distinguished and multilevel biological effects such as antimicrobial, antioxidant, anti-inflammatory, antitumour, antiplatelet and antidepressant () [Citation44]. We describe here the synthesis of a novel 4-amino-thiophene and thieno[3,2-d]pyrimidine derivatives using straightforward methods. The aminothiophene derivatives are prepared by the cyclizing of N-(4-chlorophenyl)-2-cyano-3-mercapto-3-(phenylamino)-acrylamide with α-halogenated reagents and then converted into their corresponding thieno[3,2-d]pyrimidine compounds by the treatment with phenyl isothiocyanate. The synthesized derivatives were investigated for antibacterial and anticancer activities, in addition, their theoretical studies, such as molecular modeling and docking, were also deliberated.
2. Experimental
2.1. Synthesis of 4-aminothiophenes and their congruous thienopyrimidines
2.1.1. General remarks
Melting points were measured using a Gallenkamp electric apparatus. The IR spectra (KBr discs) were recorded on a Thermo Scientific Nicolet iS10 FTIR spectrometer. The JEOL’s spectrometer was utilized to record the NMR spectra in DMSO-d6 at frequencies of 125 MHz (13C NMR) and 500 MHz (1H NMR). On a Quadrupole GC/MS Thermo Scientific Focus/DSQII, the mass analyses were carried out with an energy setting of 70 eV. The elemental analyses were carried out using a Perkin-Elmer 2400 analyzer (C, H, and N).
2.1.2. Synthesis of 4-amino-N-(4-chlorophenyl)-2-(phenylamino)thiophene-3-carboxamide derivatives 3, 5 and 7
In a 100 mL round-bottom flask (RBF), N-(4-chlorophenyl)-2-cyano-3-mercapto-3-(phenylamino)acrylamide (2) (1.65 g, 5 mmol) was dissolved in 35 mL dioxane. Then, 5 mmol of each of the appropriate α-halogenated reagents (namely, chloroacetone, ethyl bromoacetate and/or chloroacetonitrile) and 0.5 mL triethylamine were added. The mixture was refluxed for 6 hours and then allowed to cool. The solid that formed in each case was collected to furnish the targeting 4-amino-5-substituted-thiophene compounds 3, 5 and 7, respectively.
5-Acetyl-4-amino-N-(4-chlorophenyl)-2-(phenylamino)thiophene-3-carboxamide (3): Yield = 83%, m.p. = 211–212°C. IR (ν/cm−1): 3318, 3268 (NH2 and N-H), 1642 (C=O, amide), 1597 (C=O, acetyl). 1H NMR (δ/ppm): 2.09 (s, 3H, COCH3), 7.09–7.66 (m, 9H, Ar-H), 7.47 (s, 2H, NH2), 9.76 (s, 1H, N-H), 9.96 (s, 1H, N-H). 13C NMR (δ/ppm): 28.04, 105.70, 120.31 (2C), 121.89 (2C), 124.01, 126.97, 128.29 (2C), 129.39 (3C), 137.90, 140.85, 154.36, 157.51, 162.46, 186.67. MS m/z (%): 387 (M+ + 2, 21.44), 385 (M+, 60.87). Anal. Calcd. for C19H16ClN3O2S (385.07): C, 59.14; H, 4.18; N, 10.89%. Found: C, 59.23; H, 4.14; N, 10.81%.
4-Amino-N-(4-chlorophenyl)-5-ethoxycarbonyl-2-(phenylamino)thiophene-3-carboxamide (5): Yield = 78%, m.p. = 227–228°C. IR (ν/cm−1): 3362, 3324, 3262 (NH2 and N-H), 1663 (C=O, amide), 1636 (C=O, ester). 1H NMR (δ/ppm): 1.20 (s, 3H, OCH2CH3), 4.12 (s, 2H, OCH2CH3), 6.67 (s, 2H, NH2), 7.08–7.65 (m, 9H, Ar-H), 9.68 (s, 1H, NH), 9.90 (s, 1H, NH). 13C NMR (δ/ppm): 14.57, 59.04, 106.09, 119.91 (2C), 121.85 (2C), 123.68, 126.94, 128.30 (2C), 129.38 (3C), 137.88, 141.01, 154.03, 157.14, 162.58, 163.49. MS m/z (%): 415 (M+, 15.63). Anal. Calcd. for C20H18ClN3O3S (415.08): C, 57.76; H, 4.36; N, 10.10%. Found: C, 57.90; H, 4.42; N, 10.19%.
4-Amino-N-(4-chlorophenyl)-5-cyano-2-(phenylamino)thiophene-3-carboxamide (7): Yield = 70%, m.p. = 236–237°C. IR (ν/cm−1): 3309, 3256 (NH2 and N-H), 2207 (C≡N), 1657 (C=O, amide). 1H NMR (δ/ppm): 6.06 (s, 2H, NH2), 6.76–7.54 (m, 9H, Ar-H), 9.71 (s, 1H, N-H), 10.04 (s, 1H, N-H). 13C NMR (δ/ppm): 87.29, 106.35, 114.33, 120.21 (2C), 121.86 (2C), 124.11, 128.37 (2C), 129.54 (2C), 130.13, 133.64, 137.80, 140.93, 158.07, 162.67. MS m/z (%): 370 (M+ + 2, 13.94), 368 (M+, 40.78). Anal. Calcd. for C18H13ClN4OS (368.05): C, 58.62; H, 3.55; N, 15.19%. Found: C, 58.50; H, 3.61; N, 15.28%.
2.1.3. Synthesis of N-(4-chlorophenyl)-3-phenyl-6-phenylamino-thieno [3,2-d]pyrimidine-7-carboxamide analogues 4, 6 and 8
In a 100 mL RBF, a mixture of each 4-amino-1-(4-chlorophenyl)-thiophene derivative 3, 5 or 7 (2 mmol) and phenyl isothiocyanate (0.24 mL, 2 mmol) was refluxed for 4 hours in 25 mL pyridine (in compounds 3 and 5) or 25 mL DMF (in compound 7). The mixture was cooled and then poured into ice water (25 g). The solid produced in each case was collected and recrystallized from the EtOH-DMF mixture (4:1) to provide the targeting thieno[3,2-d]pyrimidine analogues 4, 6 and 8, respectively.
N-(4-Chlorophenyl)-4-methyl-3-phenyl-6-(phenylamino)-2-thioxo-2,3-dihydrothieno[3,2-d]pyrimidine-7-carboxamide (4): Yield = 58%, m.p. > 300°C. IR (ν/cm−1): 3233, 3171 (N-H), 1648 (C=O, amide). 1H NMR (δ/ppm): 2.35 (s, 3H, CH3), 7.03–7.74 (m, 14H, Ar-H), 9.91 (s, 1H, N-H), 10.13 (s, 1H, N-H). MS m/z (%): 504 (M+ + 2, 12.06), 502 (M+, 34.94). Anal. Calcd. for C26H19ClN4OS2 (502.07): C, 62.08; H, 3.81; N, 11.14%. Found: C, 61.91; H, 3.88; N, 11.27%.
N-(4-Chlorophenyl)-4-oxo-3-phenyl-6-(phenylamino)-2-thioxo-1,2,3,4-tetrahydrothieno[3,2-d]pyrimidine-7-carboxamide (6): Yield = 63%, m.p. > 300°C. IR (ν/cm−1): 3241, 3180 (N-H), 1671 (C=O, ring), 1656 (C=O, amide). 1H NMR (δ/ppm): 7.05–7.78 (m, 14H, Ar-H), 9.80 (s, 1H, N-H), 10.07 (s, 1H, N-H), 12.91 (s, 1H, N-H). MS m/z (%): 504 (M+, 21.37). Anal. Calcd. for C25H17ClN4O2S2 (504.05): C, 59.46; H, 3.39; N, 11.09%. Found: C, 59.21; H, 3.30; N, 11.00%.
N-(4-Chlorophenyl)-4-imino-3-phenyl-6-(phenylamino)-2-thioxo-1,2,3,4-tetrahydrothieno[3,2-d]pyrimidine-7-carboxamide (8): Yield = 55%, m.p. = 282–283°C. IR (ν/cm−1): 3351, 3243, 3188 (N-H), 1657 (C=O, amide), 1639 (C=N). 1H NMR (δ/ppm): 7.08–7.65 (m, 14H, Ar-H), 9.88 (s, 1H, N-H), 10.31 (s, 1H, N-H), 11.22 (s, 1H, N-H), 12.98 (s, 1H, N-H). MS m/z (%): 503 (M+, 15.48). Anal. Calcd. for C25H18ClN5OS2 (503.06): C, 59.58; H, 3.60; N, 13.90%. Found: C, 59.81; H, 3.68; N, 13.77%.
2.2. Computational studies
The synthesized compounds were studied using the DFT/B3LYP/6-311++G [Citation45–47] incorporated in the Gaussian 09W program [Citation48]. The optimized geometries of all derivatives exhibited positive frequencies that cleared their stability. The Fukui indices were determined by the Materials studio package DMol3 module [Citation49] utilizing the GGA and B3LYP functional with DNP (version 3.5) [Citation50].
2.3. Antimicrobial assay
At two diverse doses of 0.5 and 1.0 mg/mL, the inspected compounds were solvated in DMSO. To increase the effectiveness of the action, large concentrations of each studied derivative are utilized. It was calculated from the inhibitory zone diameter. For both categories of bacterial strains (+ve Gram) as Staphylococcus aureus “ATCC 25923” and Bacillus subtilis “ATCC 6635” and (−ve Gram) as Salmonella typhimurium “ATCC 14028” and Escherichia coli “ATCC 25922”, both chloramphenicol and cephalothin were used as benchmark antibiotics [Citation51,Citation52]. The experimental results were carried out in triplicate and the results are presented as mean standard deviation (SD).
2.4. Cytotoxic activity
Six 4-amino-thiophene and thienopyrimidine derivatives were investigated via the MTT procedure. On three individual cancer cell lines, including HepG2 (liver carcinoma), HCT-116 (colon carcinoma) and MCF-7 (breast carcinoma) besides WI38 (normal cell) in comparison to 5-Fu (5-flourouracil) as a positive control, the synthesized 4-amino-thiophene and their congruous thienopyrimidine derivatives were examined for in vitro cytotoxicity over the established MTT technique [Citation53]. Human cell lines were obtained from National Centre for Cell Sciences, Pune, India.
2.5. Molecular docking (MD)
All stable mol file conformers of synthesized molecules were prepared before using MOE 2015.10 software, and the 3D structure of topoisomerase II β was downloaded from the PDB site (PDB entry 3qx3; www.rcsb.org). First, the ligands were prepared in mol file and their energy was minimized to adjust their partial charge, render their atom’s charges and assign their potential energy. Then the choice protein was prepared by ignoring the heteroatoms and water, proton was add automatically, fixing the potential energy, selecting the targeted amino-acid sequence, applying the site finder and docking the site of ligand atoms through ten poses. Following preparation and optimization, the ligands were docked. To locate receptor-binding pockets, we employed the MOE® software’s London dG and GBVI/WSA dG tools. The 10 docked poses were scored and then re-scored as directed. The scores “S” (binding efficiency) and values of the root mean square RMS values were used to evaluate and choose the target molecule and receptor configurations.
3. Results and discussion
3.1. Chemistry
The synthetic strategy of 4-aminothiophene derivatives is based on the utilization of the versatile precursor, N-(4-chlorophenyl)-2-cyano-3-mercapto-3-(phenylamino) acrylamide (2) [Citation54], by its cyclizing with various halogenated reagents. Thus, the production of 5-acetyl-4-amino-N-(4-chlorophenyl)-2-(phenylamino)thiophene-3-carboxamide (3) has been achieved by heating the thiocarbamoyl precursor 2 with chloroacetone in dioxane and triethylamine (Scheme 1). The reaction of 5-acetyl-4-aminothiophene derivative 3 with phenyl isothiocyanate has proceeded in boiling pyridine to produce the corresponding 4-methyl-thieno[3,2-d]pyrimidine compound 4. The compatible spectral and elemental analyses support the structures of the 5-acetyl-4-aminothiophene derivative 3 and its congruous thieno[3,2-d]pyrimidine analogue 4.
Scheme 1. Synthesis of 5-acetyl-4-aminothiophene analogue 3 and its congruous theino[3,2-d]pyrimidine derivative 4.
![Scheme 1. Synthesis of 5-acetyl-4-aminothiophene analogue 3 and its congruous theino[3,2-d]pyrimidine derivative 4.](/cms/asset/1ac384dd-13a1-487e-aeb2-d885cc1e5c6e/tusc_a_2164993_f0013_ob.jpg)
In addition, the treatment of N-(4-chlorophenyl)-2-cyano-3-mercapto-3-(phenylamino)-acrylamide (2) with ethyl bromoacetate was carried out in refluxing dioxane and triethylamine to afford the corresponding 5-ethoxycarbonyl-4-amino-thiophene analogue 5. The reaction mechanism is initiated by the ability of acidic thiol function (–SH) to replace the bromine from ethyl bromoacetate. The produced intermediate (A) undergoes intramolecular cyclization through the nucleophilic addition of a methylene group on the nitrile function to produce the corresponding 4-amino-5-ethoxycarbonyl-thiophene derivative 5 (Scheme 2). The cyclization of this 4-aminothiophene derivative 5 with phenyl isothiocyanate in boiling pyridine yielded the bicyclic ring system, 4-oxo-thieno[3,2-d]pyrimidine compound 6. The structures of 4-aminothiophene analogue 5 and its congruous thieno[3,2-d]pyrimidine compound 6 were secured by compatible spectral analyses.
Scheme 2. Synthesis of 4-amino-5-ethoxycarbonyl-thiophene analogue 5 and its congruous theino[3,2-d]pyrimidine derivative 6.
![Scheme 2. Synthesis of 4-amino-5-ethoxycarbonyl-thiophene analogue 5 and its congruous theino[3,2-d]pyrimidine derivative 6.](/cms/asset/d8769cdd-a5c9-4143-9418-4ba1a23eecf1/tusc_a_2164993_f0014_ob.jpg)
Furthermore, the reaction of N-(4-chlorophenyl)-2-cyano-3-mercapto-3-(phenylamino)-acrylamide (2) with chloroacetonitrile in boiling dioxane and triethylamine furnished the corresponding 4-amino-5-cyano-thiophene derivative 7. The nucleophilic substitution of the chlorine from chloroacetonitrile by acidic thiol function (–SH) of thiocarbamoyl precursor 2 furnished the intermediate (B). This undergoes intramolecular cyclization through the nucleophilic addition of a methylene group on the nitrile function to produce the corresponding 4-amino-5-cyano-thiophene compound 7 (Scheme 3). The production of 4-imino-thieno[3,2-d]pyrimidine analogue 8 has been achieved upon heating 4-amino-5-cyanothiophene derivative 7 with phenyl isothiocyanate in boiling dimethylformamide (DMF) for four hours. The spectral analyses are utilized to secure the structures of 4-amino-5-cyanothiophene derivative 7 and its congruous 4-imino-thieno[3,2-d]pyrimidine analogue 8.
3.2. DFT structural and electronic features
The dihedral data of 4-amino-N-(4-chlorophenyl)-2-(phenylamino)thiophene-3-carboxamide derivatives 3, 5 and 7 have a non-planar configuration in which the phenylamino nitrogen and phenyl carboxamide carbon and oxygen atoms were situated out of the thiophene ring plane, e.g. the average values of C4(thio)-C3(thio)-C2(thio)-NH(Ph), CO(carb)-C3(thio)-C2(thio)-S1(thio) and C2(thio)-C3(thio)-CO(carb)-OC(carb) were 171.5, 175.6 and 19.3°, respectively. Furthermore, the carboxamide phenyl ring was tilted more than the other phenyl ring as the C3(thio)-CO(carb)-NH(carb)-C1(Phcarb) = 39.7° while S1(thio)-C2(thio)-NH(Ph)-C1(Ph) = −7.1°. The 4-amino substituent in all derivatives was slightly shifted above the thiophene plane, e.g. the NH2(thio)-C4(thio)-C5(thio)-S1(thio) = 174.5°, and so the 5-substituents, acetyl, carboxylate and cyano () (Table S1).
Alternatively, the N-(4-chlorophenyl)-3-phenyl-6-(phenylamino)-2-thioxo-2,3-dihydrothieno [3,2-d]pyrimidine-7-carboxamide derivatives, 4, 6 and 8, have also a non-planar configuration. The dihedral angle data showed that the thienopyrimidine-fused ring slightly deviated from planarity, 0.1–3.5°. Also, the methyl and oxo substituents in 4 and 6 were marginally out of the fused ring plane, i.e. S5(thpy)-C4a(thpy)-C4(thpy)-Me(thpy) = -1.3° and S5(thpy)-C4a(thpy)-C4(thpy)-O(Oxo) = 1.1°, respectively, while both imino groups were almost coplanar, S5(thpy)-C4a(thpy)-C4(thpy)-NH(imn) = 0.3°. The thioxo substituent showed perfect planarity with the thienopyrimidine in 4 as C4(thpy)-N3(thpy)-C2(thpy)-S(thoxo) = 180.0°, whereas the corresponding angle in 6 and 8 was 175.4°, which is clearly shifting out. However, the data presented that the phenyl substituent was almost perpendicular on the fused ring plane, e.g. C2(thpy)-N3(thpy)-C1(Ph)-C2(Ph) = 89.4–95.0°. Despite the phenylamino nitrogen atom exhibited a little non-planarity with respect to the thienopyrimidine moiety where NH(Phamn)-C6(thpy)-S5(thpy)-C4a(thpy) = 175.6–177.7°, the corresponding phenyl ring was strongly tilted on the fused ring plane, e.g. the C6(thpy)-NH(Phamn)-C1(Phamn)-C2(Phamn) ranged from 160.3°, in 4, to 171.3°, in 8. Likewise, the carboxamide carbon atom was placed out of the thienopyrimidine ring plane in all derivatives by 8.5–9.9°, while its oxygen and nitrogen atoms were strongly shifted out of the plane, ∼17.5° and 40.0°, respectively, in 6 and 8 () (Table S1).
As well, the DFT calculated bond lengths and angles almost coincided with those obtained from single crystal X-rays of similar compounds [Citation55–57] where lengths were longer by 1.72 Å maximum with 0.052–0.062 Å RMSD, while the angles were deviated by 10.5° maximum with 4.00–5.06° RMSD, which may be attributed to that the quantum chemical calculations carried out for isolated molecule in the gaseous state, no intermolecular columbic interactions, whereas the practical obtained from molecules interacting in solid crystal lattice [Citation58] (Tables S2 and S3).
3.2.1. Frontier molecular orbitals
The HOMO-LUMO structures and energetic values were the main factors affecting the donation or receiving of electrons [Citation59] where reducing the HOMO-LUMO gap will result in the ease of intramolecular charge transfer [Citation60,Citation61] which may affect the molecule’s bioactivity [Citation62]. The FMO graphs of the examined compounds are presented in Figures and . The diagram indicated that compounds 3, 5 and 7 have HOMO spread over the whole molecule and consisted of the π-orbital of the 4-amino-2-(phenylamino)thiophene-3-carboxamide moiety along with the heteroatoms lone pair of electrons, while their LUMO was built from the π*-orbitals of the whole molecule. Thus, the HOMO-LUMO charge transfer may be mainly described as π → π* and n → π* transitions ().
On the contrary, the HOMO of 4, 6 and 8 derivatives was built mainly of the lone pair of electrons belonging to the thioxo sulphur and nitrogen atoms of the thienopyrimidine-fused ring with a minor contribution of the π-orbital of the phenyl substituent along with moderate involvement of the oxo and imino substituents in 6 and 8. However, the LUMO of compound 4 was constructed from the π*-orbital of the fused thienopyrimidine ring in addition to the phenylamino group, whereas the corresponding 6 and 8 derivatives were made up of thephenylamino and carboxamide substituents mainly beside partial contribution of the thienopyrimidine π*-orbital. Therefore, the HOMO-LUMO charge transfer may be mainly described as n → π* transition ().
The HOMO-LUMO energy, EH and EL were affected by the abovementioned facts. Although the data displayed that all the derivatives have close values of the EH and EL, in the range of −5.43 to −6.01 and −3.23 to −3.86 eV, respectively, the thiophene derivatives 3, 5 and 7, generally, exhibited lower EH than the 4, 6 and 8. Contrarily, the EL data revealed that the thienopyrimidine derivatives have lower values than those of thiophenes. However, the ΔEH-L gap of the thiophene derivatives was lesser than that of thienopyrimidines except for compound 4 which has the lowest value where the investigated compounds may be ordered due to the energy gap as 4 < 7 < 5 < 3 < 8 < 6 ().
Table 1. The HOMO-LUMO energies and chemical reactivity descriptors (eV) of investigated compounds.
Correspondingly, chemical reactivity descriptors, electronegativity (χ), global hardness (η), softness (δ) and electrophilicity (ω) were evaluated using the EH and EL values by the following formula [Citation60].
The data revealed that according to electronegativity (χ), derivatives 5 and 6 exhibited the lowest and highest Lewis’s acid character, 4.39 and 4.75 eV, respectively. Whereas, the global softness (δ) and hardness (η) values designated that compound 4 possessed the maximum electrons receiving and charge transfer ability, 1.27 and 0.78 eV, respectively. Hence, consistent with hardness, the studied derivatives may be ordered as 4 < 7 < 5 < 3 < 8 > 6, in agreement with that of the ΔEH-L gap ().
3.2.2. Atomic Mulliken’s charges and Fukui’s indices
The charge transfer within a molecule can be correlated to Mulliken’s atomic charges [Citation63]. In the thiophene derivatives, the thienyl sulphur atom, S1(thio), has a constant negative charge in all derivatives, −0.614 to −0.691, while the thienyl carbon, C5(thio) attached to the acetyl (3), carboxylate (5) and cyano (7) groups bears decreasing negative charge, −0.783, −0.275 and −0.145, which may be attributed to the increase of the electron-withdrawing property of the substituents, respectively ().
Table 2. Selected Mulliken’s atomic charges of investigated compounds.
In thienopyrimidine derivatives, the thienopyrimidine nitrogen atom N1(thyp) and carbon C4(thyp) have a positive charge, in the methyl derivative, 4, 0.612 and 0.406, respectively, which reduced to be 0.113 and 0.100; 0.275 and 0.249, in both oxo and imino derivatives, 6 and 8, respectively, which may be attributed to the participation of the lone pair of electrons on the oxo and imino groups in the resonating structure of the fused ring of the latter derivatives. Moreover, the beforementioned behaviour was applied in the case of the thienopyrimidine nitrogen atom N3(thyp) which also has a decreasing positive charge, 0.754 to 0.568, whilst the thienopyrimidine carbon atom C4a(thyp) has an almost constant positive charge, 0.242–0.255, which may be resulted from the electron release effect of the adjacent sulphur atom S5(thyp) that has a negative charge. Finally, the data showed that the other heteroatoms had almost the same negative charge in all derivatives ().
The atomic Fukui’s reactivity indices, ,
and
, towards nucleophilic, electrophilic and radical attacks, respectively, were assessed [Citation64–67]. The nucleophilic attack Fukui’s indices
of the thiophene derivatives 3, 5 and 7 disclosed that the thienyl sulphur S1(thio) was the most labile atom followed by the carboxamide carbon and oxygen atoms, CO(carb) and OC(carb), respectively. However, the thienopyrimidine data showed that the S5(thpy), S(thox) and C6(thpy) were the top three active sites in the derivatives 6 and 8 while in the case of compound 4, they were also the most susceptible atoms but in a different order, S(thox), S5(thpy) and C4(thpy), respectively ().
Table 3. Selected top five atomic Fukui’s indices, the local relative electrophilicity and nucleophilicity descriptors’ data of the investigated compounds.
However, the electrophilic attack Fukui’s indices of the thienyl derivatives 3, 5 and 7 offered different patterns of the highly susceptible atoms wherein the thienyl sulphur atom S1(thio) occupied the first place in 3 and 5, while the cyano nitrogen NC(cyan) was the most active one in 7. The atoms in the second and third place were varied, whereas the amino nitrogen atoms NH2(thio) and NH(Ph) occupied the fourth and fifth positions, respectively. On the other hand, the thienopyrimidine compounds presented comparable patterns where the thioxo sulphur atom, S(thox), existed at the top position followed by the S5(thpy) atom except in 6 where the O(oxo) atom occupied the second place before the thienopyrimidine sulphur.
Eventually, the radical attack indices of the synthesized thienyl and thienopyrimidine derivatives exhibited comparable orderliness to those obtained for the electrophilic attack Fukui’s indices
where the sulphur of thienyl S1(thio) and thioxo S(thox) was the most susceptible site, respectively. Moreover, the oxygen of acetyl and carboxamide groups occupied the second place in compounds 3 and 5, respectively, while the cyano nitrogen was in derivative 7. However, the sulphur of the thienopyrimidine ring, S5(thpy), appeared in the second place in 4, 6 and 8 ().
To overcome the inaccurate prediction of the active sites for the nucleophilic and electrophilic attack via Fukui’s indices, the relative electrophilicity and nucleophilicity descriptors, and
, respectively, were estimated [Citation68–70], where
and δ is the global softness. The relative nucleophilicity descriptors data,
, of derivative 3 showed that carboxamide CO(carb) and phenylamine C3(Ph) were the first and second active atoms, respectively, as well as in 5 and 7. Moreover, the data indicated that the phenylcarboxamide carbon atoms, CO(carb) and C2(Phcarb), were the first two susceptible in compounds 6 and 8, whereas in 4, the thienopyrimidine carbon, C4(thpy), was the first and followed by the thienopyrimidine nitrogen atom, N1(thpy).
Alternatively, the relative electrophilicity descriptors, , data displayed almost coincided patterns for the thiophene and thienopyrimidine derivatives, wherein in 3 and 5, the thienyl carbons C5(thio) and C4(thio) were on the top positions, respectively, whilst in 7, they were in a reversed order. Likewise, the thienopyrimidine carbon C2(thpy) was the most susceptible site in the 4 and 6, whilst, in 8, the N1(thpy) was on the top and followed by the C2(thpy) ().
3.3. Biological evaluation
3.3.1. Antimicrobial activity
Using the disc-agar diffusion technique, the newly prepared thiophene and thienopyrimidine derivatives were investigated for antibacterial effectiveness across Gram +ve and Gram −ve spectra. Figures and demonstrated the antimicrobial results for the 4-amino-thiophene and thienopyrimidine derivatives towards the antibacterial action because they could block the creation of folic acid on both types of bacterial strains [Citation71]. The checked derivatives, in general, are more susceptible to Gram-positive and (−ve) Gram-negative bacterial strains. Where, compounds 3, 4, 6 and 8 designated higher activity towards Gram-positive bacterial strains rather than Gram-negative strains relative to the results obtained by Chloramphenicol and Cephalothin. Meanwhile, compounds 4, 6 and 8 (characterized by the presence of thienopyrimidine moiety) exhibited the highest activity towards S. aureus and B. subtilis as Gram-positive bacterial strains across two concentrations of 0.5 and 1.0 mg/mL, respectively.
Viewing the results, it became clear that compound 8 displayed (39.72 ± 2.98 and 42.57 ± 3.19) against S. aureus and (33.47 ± 2.51 and 35.91 ± 2.69) against B. subtilis, hybrid 6 revealed (43.53 ± 3.26 and 45.64 ± 3.42) against S. aureus and (38.22 ± 2.87 and 39.83 ± 2.99) against B. subtilis and hybrid 4 revealed (34.34 ± 2.58 and 35.16 ± 2.64) against S. aureus and (21.15 ± 1.59 and 23.41 ± 1.76) against B. subtilis. Moreover, derivative 3 having the 5-acetyl-4-amino-thiophene moiety offered better activity (37.22± 2.79 and 38.56 ± 2.89) against S. aureus and (31.82 ±2.39 and 32.40 ± 2.43) against B. subtilis. Furthermore, the presence of electron-attracting groups such as (acetyl, cyano and ester) in compounds 3, 5 and 7 containing amino-thiophene moiety represented acceptable improvement in their antibacterial activities against S. typhimurium and E. coli as Gram-negative bacterial strains in contrast to the standard Cephalothin (Table S4).
3.3.2. Anti-tumour activity
displays the cytotoxic results as IC50 values and compared them to the widely-used anti-cancer standard (5-Fu). Except for HCT-116, several synthesized thienopyrimidines showed good efficacy in preventing the growth of (MCF-7) and (HepG2). [Citation72]. The results of the studied amino-thiophene and thienopyrimidine compounds exhibit inhibitions toward MCF-7, HepG2, and HCT-116, respectively in consistence to structural activity relationship. Primarily, the 5-acetyl-4-amino-thiophene-3-carboxamide thiophene analogue 3 has a reputable weak activity with IC50 = 29.51 ± 0.47, 47.16 ± 0.12 and 37.63 ± 0.32 μM. While analogue 4 with 4-methyl-2-thioxo-2,3-dihydrothieno[3,2-d]pyrimidine moiety offered good activities with IC50 =14.53 ± 0.16, 12.27 ± 0.12 and 15.75 ± 0.42μM. Meanwhile, 2-carboethoxy-3-amino-thiophene analogue 5 displayed poor effectiveness with IC50 =38.44 ± 0.19, 36.35 ± 0.27 and 49.82 ± 0.38 μM. However, 4-oxo-2-thioxo-1,2,3,4-tetrahydrothieno[3,2-d]pyrimidine analogue 6 showed excellent activities with IC50 = 11.17 ± 0.06, 9.33 ± 0.27 and 10.63 ± 0.40 μM against MCF-7, HepG2 and HCT-116, respectively. Moreover, 4-amino-5-cyano-2-thiophene-3-carboxamide analogue 7 showed reasonable cytotoxic activities with IC50 = 16.76 ± 0.36, 15.80 ± 0.23 and 18.42 ± 0.46 μM. Furthermore, 4-imino-2-thioxo-1,2,3,4-tetrahydrothieno[3,2-d]pyrimidine analogue 8 with revealed moderate anti-proliferative activities with IC50 = 19.33 ± 0.18, 18.02 ± 0.39 and 23.81 ± 0.11 μM.
3.3.3. Structural activity relationship (SAR)
As a purine base contained in DNA and RNA-bioisosteres, adenine, can be structurally compared to the thieno-pyrimidine scaffold, which is a fused heterocyclic ring structure. We are investigating the relationship between its structure and activity because of its wide spectrum of therapeutic uses, such as anticancer preventatives (SAR). Inspired by the previous anticancer results and recent survey, the thienopyrimidine compounds, especially 6 and 8, showed potential activities against MCF-7 and HepG2 [Citation73]. Derivative 6 contains a pyrimidinone ring that recorded a superior activity than derivative 4 with the methyl-pyrimidine moiety rather and analogue 7 has amino and cyano groups on the thiophene ring which showed reasonable antiproliferative activity between thienopyrimidine derivatives which agree with the earlier literature [Citation74]. Meanwhile, derivative 8 with the imino group displayed a lower activity rather than derivatives 6,4, and 7 which may be attributed to the carbonyl group being more attractive electrons than that of the imino group. Temporarily, in analogue 3 substituted with the 5-acetyl and 2-amino group on the thiophene presented poor selective anti-tumour activity [Citation75]. Finally, the order of reactivity can be recorded as 6 > 4 > 7 > 8 > 3,5.
3.4. Molecular docking
To investigate the interaction mechanism and binding mode of the synthesized 4-amino-thiophene and thienopyrimidine derivatives and the FDA drug 5-fluorouracil at the active site of the (ID: 3qx3)protein, molecular docking studies are carried out using MOE (MOE Version 2015.10). By turning on the MOE software’s reduction algorithm, protein energy is reduced to the absolute minimum. By employing the force field topoisomerase II β and a technique known as the conjugate gradient algorithm, the MOE software determines the final and beginning energy of the proteins in kcal/mol. The peptidomimetic ligand was released from Human topoisomerase II beta in a complex with DNA and etoposide after all water molecules were removed [Citation76]. The essential residues involved in the substrate’s interaction, such as (Phe 738, Arg 743, Gly 1023, Glu 855 and Lys 843), have an adequate level of alignment (RMSD < 1.5). It is thought that they enable the substrate’s grid to open to the active state. Inspired by the docking stimulation it was found that the synthesized 4-amino-thiophene and thienopyrimidine derivatives revealed nearly acceptable binding scores ranging from −6.6321 to −7.6448 Kcal/mol (). The 5-acetyl-4-amino-thiophene-3-carboxamide derivative 3 displayed two interactions, Cl 20 on the phenyl ring with Phe 738 and Arg 743 amino acids over H-donor through (3.34 Å) and H-acceptor through (3.51 Å) (Figure S1). Similarly, 4-methyl-2-thioxo-2,3-dihydrothieno[3,2-d]pyrimidine 4 exhibited H-acceptor interaction between S 7 of the thioxo group with Arg 581, two π- H attractions between the phenyl ring of aniline moiety with Lys 1107 and a pyrimidine ring with Asn 840 over intermolecular distances 4.20, 4.27 and 4.02 Å ().
Table 4. In silico docking results of the prepared thiophene and thienopyrimidine analogues.
Likewise, 2-ethoxycarbonyl-3-amino-thiophene analogue 5 demonstrated the identical interactions between Cl 21 with Phe 738 and Arg 743 amino acids with the same H-bonds (donor and acceptors) and intermolecular distances 3.32 and 3.69 (Figure S2). Temporarily, 4-oxo-2-thioxo-1,2,3,4-tetrahydrothieno[3,2-d]pyrimidine analogue 6 displayed two H-donors between Glu855 amino acid through S11 of thiophene ring and N 28 of aniline moiety in addition to one H-acceptor between S7 thioxo moiety with Arg 689 with prefect Rmsd = 0.9029 ().
Meanwhile, 4-amino-5-cyano-2-thiophene-3-carboxamide analogue 7 presented five intermolecular H-bonds (three H-donors and two H-acceptors), N 3 of amino-thiophene moiety with Glu 855, N 10 of N-phenyl moiety with Gly 1023, Cl 17 atom on the phenyl ring with Phe 738 and N 25 of cyano group with Arg 692 and Lys 843. All these interactions were formed over intermolecular distances 3.01, 3.27, 3.22, 3.16 and 3.30 , respectively (Figure S3). Moreover, 4-imino-2-thioxo-1,2,3,4-tetrahydrothieno[3,2-d]pyrimidine analogue 8 exposed the highest binding score S = −7.6448 Kcal/mol, H-donor among N 28 of aniline moiety with His 1021, H-acceptor between Cl 21 of chlorine atom with Arg 743 and π-H between the pyrimidine ring and Gly 1023 over Rmsd = 1.1378 ().
Furthermore, 5-fluorouracil (reference) offered an H-acceptor between O3 of one of two carbonyl groups of the pyrimidine ring with Arg 688 and π-cation interaction between the pyrimidine ring with Arg 689 over 2.97 and 3.87 (Figure S4).
Lastly, a docking experiment was applied to confirm how much the prepared derivatives interactions alter as they cooperate with many 3QX3 amino acids. Each of the six synthesized amino-thiophene and thienopyrimidine derivative was reinforced by a network of H bonds as the chemical aromatic structures of amino-thiophenes and thienopyrimidines interact directly with the amino acids of 3QX3. Most of the prepared derivatives had the 3QX3 pocket, which may be seen as a fork encompassed by polar and nonpolar residues of amino acids (Phe 738, Arg 743, Gly 1023, Glu 855 and Lys 843). Additionally, the fact that the majority of derivatives belong to the same amino acids is seen as significant support for the efficacy of the docking technique.
4. Conclusion
Three functionalized 4-aminothiophenes are synthesized based on cyclizing the N-(4-chlorophenyl)-2-cyano-3-mercapto-3-(phenylamino)-acrylamide with chloroacetone, ethyl bromoacetate and/or chloroacetonitrile. Treatment of these 4-aminothiophenes with phenyl isothiocyanate was the synthetic strategy to obtain their congruous thieno[3,2-d]pyrimidine compounds. The investigated compounds’ DFT calculated structure designated that all deviated from planar conformation. The HOMO-LUMO energy gaps of thiophene derivatives have higher values than the thienopyrimidines, as shown in the following order 4 < 8 < 6 < 7< 5 < 3. The synthesized derivatives were assessed for their antimicrobial activity towards S. aureus, B. subtilis (+ve Gram) and S. typhimurium, E. coli (−ve Gram). From antimicrobial screening results, it was observed that derivatives 3, 4, 6 and 8 designated acceptable activity towards (+ve and −ve) Gram strains compared to standard drugs, Chloramphenicol and Cephalothin. Meanwhile, the examined derivatives’ cytotoxic effects on the cancer cell lines “HCT-116, MCF-7, and HepG2” were assessed. In contrast to “5-fluorouracil” the usual reference, the examined derivatives 4, 6, and 7 showed strong effectiveness towards MCF-7 and HepG2 cell lines. Additionally, theoretical molecular docking results displayed acceptable binding scores and Rmsd values for the investigated derivatives alongside the amino acids of 3QX3.
Supplemental Material
Download MS Word (5.2 MB)Disclosure statement
No potential conflict of interest was reported by the author(s).
Additional information
Funding
References
- Li B, Webster TJ. Bacteria antibiotic resistance: new challenges and opportunities for implant-associated orthopedic infections. J Orthop Res. 2018;36:22–32.
- Majumder MAA, Rahman S, Cohall D, et al. Antimicrobial stewardship: fighting antimicrobial resistance and protecting global public health. Infect Drug Resist. 2020;13:4713.
- Mattiuzzi C, Lippi G. Current cancer epidemiology. J Epidemiol Glob Health. 2019;9:217.
- Cortes J, Perez-García JM, Llombart-Cussac A, et al. Enhancing global access to cancer medicines, CA. Cancer J Clin. 2020;70:105–124.
- McGorry PD, Hickie IB, Yung AR, et al. Clinical staging of psychiatric disorders: A heuristic framework for choosing earlier, safer and more effective interventions. Aust N Z J Psychiatry. 2006;40:616–622.
- Kennedy LC, Bickford LR, Lewinski NA, et al. A new era for cancer treatment: gold-nanoparticle-mediated thermal therapies. Small. 2011;7:169–183.
- Van Straten D, Mashayekhi V, De Bruijn HS, et al. Oncologic photodynamic therapy: basic principles, current clinical status and future directions. Cancers. 2017;9:19.
- Elgiushy HR, Mohamed SH, Taha H, et al. Identification of a promising hit from a new series of pyrazolo[1,5-a]pyrimidine based compounds as a potential anticancer agent with potent CDK1 inhibitory and pro-apoptotic properties through a multistep in vitro assessment. Bioorg Chem. 2022;120:105646.
- Morsy NM, Hassan AS, Hafez TS, et al. Synthesis, antitumor activity, enzyme assay, DNA binding and molecular docking of bis-Schiff bases of pyrazoles. J Iran Chem Soc. 2021;18:47–59.
- Al Wasidi AS, Hassan AS, Naglah AM. In vitro cytotoxicity and druglikeness of pyrazolines and pyridines bearing benzofuran moiety. J Appli Pharm Sci. 2020;10:142–148.
- Ibrahim SA, Ragab A, El-Ghamry HA. Coordination compounds of pyrazolone-based ligand: design, characterization, biological evaluation, antitumor efficiency, and DNA binding evaluation supported by in silico studies. Appl Organomet Chem. 2022;36:e6508.
- Eldeeb M, Sanad EF, Ragab A, et al. Anticancer effects with molecular docking confirmation of newly synthesized Isatin sulfonamide molecular hybrid derivatives against hepatic cancer cell lines. Biomed. 2022;10:722.
- Azher OA, Hossan A, Pashameah RA, et al. Synthesis, anticancer evaluation, and molecular modeling study of new 2-(phenylamino) pyrazolo [1, 5-a] pyrimidine analogues. Arab J Chem. 2022: 104437.
- Shaaban S, Adam MSS, El-Metwaly NM. Novel organoselenium-based N-mealanilic acid and its zinc (II) chelate: catalytic, anticancer, antimicrobial, antioxidant, and computational assessments. J Mol Liq. 2022;363:119907.
- Guillemard L, Kaplaneris N, Ackermann L, et al. Late-stage C–H functionalization offers new opportunities in drug discovery. Nat Rev Chem. 2021;5:522–545.
- Nadal Rodríguez P, Ghashghaei O, Bagán A, et al. Heterocycle-based multicomponent reactions in drug discovery: from hit finding to rational design. Biomed. 2022;10:1488.
- Jiang X, Hao X, Jing L, et al. Recent applications of click chemistry in drug discovery. Expert Opin Drug Discov. 2019;14:779–789.
- Hassan AS. Antimicrobial evaluation, in silico ADMET prediction, molecular docking, and molecular electrostatic potential of pyrazole-isatin and pyrazole-indole hybrid molecules. J Iran Chem Soc. 2022: 1–13.
- Naglah AM, Askar AA, Hassan AS, et al. Biological evaluation and molecular docking with in silico physicochemical, pharmacokinetic and toxicity prediction of pyrazolo[1,5-a]pyrimidines. Molecules. 2020;25:1431.
- Ali Mohamed H, Ammar YA, Elhagali GAM, et al. In vitro antimicrobial evaluation, single-point resistance study, and radiosterilization of novel pyrazole incorporating thiazol-4-one/thiophene derivatives as dual DNA gyrase and DHFR inhibitors against MDR pathogens. ACS Omega. 2022;7:4970–4990.
- Fayed EA, Mohsen M, Abd El-Gilil SM, et al. Novel cyclohepta [b] thiophene derivative incorporating pyrimidine, pyridine, and chromene moiety as potential antimicrobial agents targeting DNA gyrase. J Mol Struct. 2022;1262 :133028.
- Chaurasiya A, Wahan SK, Sahu C, et al. An insight into the rational design of recent purine-based scaffolds in targeting various cancer pathways. J Mol Struct. 2022: 134308.
- Nazreen S, Almalki AS, Elbehairi SEI, et al. Cell cycle arrest and apoptosis-inducing ability of benzimidazole derivatives: design, synthesis, docking, and biological evaluation. Molecules. 2022;27:6899.
- Almalki AS, Nazreen S, Elbehairi SEI, et al. Design, synthesis, anticancer activity and molecular docking studies of new benzimidazole derivatives bearing 1,3,4-oxadiazole moieties as potential thymidylate synthase inhibitors. New J Chem. 2022;46:14967–14978.
- Okasha RM, Fouda AM, Bajaber MA, et al. The crystal structure of 3-amino-1-(4-chlorophenyl)-9-methoxy-1H-benzo[f]chromene-2-carbonitrile: antimicrobial activity and docking studies. Crystals. 2022;12:982.
- Ahmed Saleh Alzahrani S, Nazreen S, Elhenawy AA, et al. Synthesis, biological evaluation, and molecular docking of new benzimidazole-1,2,3-triazole hybrids as antibacterial and antitumor agents. Polycycl Aromat Compd. 2022. https://doi.org/10.1080/10406638.2022.2069133
- Ouassaf M, Belaidi S, Al Mogren MM, et al. Combined docking methods and molecular dynamics to identify effective antiviral 2,5-diaminobenzophenonederivatives against SARS-CoV-2. J King Saud Univ Sci. 2021;33 :101352.
- Mishra R, Kumar N, Sachan N. Thiophene and its analogs as prospective antioxidant agents: a retrospective study. Mini Rev Med Chem. 2022;22:1420–1437.
- Althagafi I. Molecular modeling and antioxidant evaluation of new di-2-thienyl ketones festooned with thiazole or pyridine moiety. J Mol Struct. 2022;1247 :131287.
- Abumelha HM, Bayazeed A, Alsoliemy A, et al. Molecular modeling of new thiazolyl-thiophene based compounds as antioxidant agents. J Mol Struct. 2022;1262:133112.
- da Cruz RMD, Mendonça-Junior FJB, de Mélo NB, et al. Thiophene-based compounds with potential anti-inflammatory activity. Pharm. 2021;14:692.
- Yu S-J, Yu J-H, He F, et al. New antibacterial thiophenes from Eclipta prostrata. Fitoterapia. 2020;142:104471.
- Mabkhot YN, Kaal NA, Alterary S, et al. Antimicrobial activity of thiophene derivatives derived from ethyl (E)-5-(3-(dimethylamino) acryloyl)-4-methyl-2-(phenylamino) thiophene-3-carboxylate. Chem Cent J. 2017;11:1–11.
- Pathania S, Chawla PA. Thiophene-based derivatives as anticancer agents: an overview on decade’s work. Bioorg Chem. 2020;101:104026.
- Al Shareef HF. Synthesis of some novel 2-(3-cyano-6-(thiophen-2-yl)-4, 4′-bipyridin-2-yloxy) acetohydrazide derivatives: assessment of their cytotoxic activity. BMC Chem. 2020;14 :1–13.
- Ghorab MM, Bashandy MS, AL-SAID MS. Novel thiophene derivatives with sulfonamide, isoxazole, benzothiazole, quinoline and anthracene moieties as potential anticancer agents. Acta Pharm. 2014;64:419–431.
- Ghorab MM, Al-Said MS. Antitumor activity of novel pyridine, thiophene and thiazole derivatives. Arch Pharm Res. 2012;35:965–973.
- Zarei O, Azimian F, Hamzeh-Mivehroud M, et al. Design, synthesis, and biological evaluation of novel benzo[b]thiophene-diaryl urea derivatives as potential anticancer agents. Med Chem Res. 2020;29:1438–1448.
- Keri RS, Chand K, Budagumpi S, et al. An overview of benzo [b] thiophene-based medicinal chemistry. Eur J Med Chem. 2017;138:1002–1033.
- Mishra R, Kumar N, Mishra I, et al. A review on anticancer activities of thiophene and its analogs. Mini Rev Med Chem. 2020;20:1944–1965.
- Roehrig S, Straub A, Pohlmann J, et al. Discovery of the novel antithrombotic agent 5-chloro-N-({(5 S)-2-oxo-3-[4-(3-oxomorpholin-4-yl)phenyl]-1,3-oxazolidin-5-yl}methyl)thiophene-2-carboxamide (BAY 59-7939): an oral, direct factor Xa inhibitor. J Med Chem. 2005;48:5900–5908.
- Soltan OM, Shoman ME, Abdel-Aziz SA, et al. Molecular hybrids: a five-year survey on structures of multiple targeted hybrids of protein kinase inhibitors for cancer therapy. Eur J Med Chem. 2021;225:113768.
- Schenone S, Radi M, Musumeci F, et al. Biologically driven synthesis of pyrazolo [3,4-d] pyrimidines as protein kinase inhibitors: an old scaffold as a new tool for medicinal chemistry and chemical biology studies. Chem Rev. 2014;114 :7189–7238.
- Prabhakar V, Babu KS, Ravindranath L, et al. Design, synthesis, characterization and biological activity of novel thieno[2,3-d]pyrimidine derivatives. Indian J Adv Chem Sci. 2017;5:30–42.
- Becke AD. Density-functional thermochemistry. III. The role of exact exchange. J Chem Phys. 1993;98:5648–5652.
- Lee C, Yang W, Parr RG. Development of the Colle-Salvetti correlation-energy formula into a functional of the electron density. Phys Rev B. 1988;37:785–789.
- Perdew JP, Wang Y. Pair-distribution function and its coupling-constant average for the spin-polarized electron gas. Phys Rev B. 1992;46:12947–12954.
- Frisch M, Trucks G, Schlegel H, et al. Gaussian 09, revision A. 1. Wallingford (CT): Gaussian; 2009.
- Materials Studio Dsbiovia. Dassault systèmes. San Diego; 2017.
- Delley B. Ground-state enthalpies: evaluation of electronic structure approaches with emphasis on the density functional method. J Phys Chem A. 2006;110:13632–13639.
- Azoro C. Antibacterial activity of crude extract of azudirachita indica on salmonella typhi. World J. Biotechnol. 2002;3:347–351.
- Al-Anazi KM, Mahmoud AH, AbulFarah M, et al. 2-Amino-5-arylazothiazole-based derivatives: in vitro cytotoxicity, antioxidant properties, and bleomycin-dependent DNA damage. ChemistrySelect. 2019;4:5570–5576.
- Rahmouni A, Souiei S, Belkacem MA, et al. Synthesis and biological evaluation of novel pyrazolopyrimidines derivatives as anticancer and anti-5-lipoxygenase agents. Bioorg Chem. 2016;66:160–168.
- George RF. Stereoselective synthesis and QSAR study of cytotoxic 2-(4-oxo-thiazolidin-2-ylidene)-2-cyano-N-arylacetamides. Eur J Med Chem. 2012;47:377–386.
- Zhang Q, Yu M, Yuan J, et al. Formal [4 + 1] annulation of α-arylhydrazonoketones and dimethylsulfoxonium methylide: one-pot synthesis of substituted pyrazoles and dihydropyrazoles. J Org Chem. 2016;81:6036–6041.
- Şener N, Bayrakdar A, Kart HH, et al. A combined experimental and DFT investigation of disazo dye having pyrazole skeleton. J Mol Struct. 2017;1129 :222–230.
- Wu M-H, Hu J-H, Shen D-S, et al. Regiospecific synthesis of 6-aryl-3-cyano-5-alkylamino/arylamino-1-p-tolyl-1H-pyrazolo[4,3-d]pyrimidin-7(6H)-ones via iminophosphorane-mediated annulation. Tetrahedron. 2010;66:5112–5120.
- Sajan D, Joseph L, Vijayan N, et al. Natural bond orbital analysis, electronic structure, non-linear properties and vibrational spectral analysis of L-histidinium bromide monohydrate: a density functional theory. Spectrochim Acta Part A Mol Biomole Spectrosc. 2011;81:85–98.
- Bulat FA, Chamorro E, Fuentealba P, et al. Condensation of frontier molecular orbital Fukui functions. J. Phys. Chem. A. 2004;108:342–349.
- Xavier S, Periandy S, Ramalingam S. NBO, conformational, NLO, HOMO–LUMO, NMR and electronic spectral study on 1-phenyl-1-propanol by quantum computational methods, Spectrochim. Acta Part A Mol Biomole Spectrosc. 2015;137:306–320.
- Makhlouf MM, Radwan AS, Ghazal B. Experimental and DFT insights into molecular structure and optical properties of new chalcones as promising photosensitizers towards solar cell applications. Appl Surf Sci. 2018;452:337–351.
- Bouchoucha A, Zaater S, Bouacida S, et al. Synthesis and characterization of new complexes of nickel (II), palladium (II) and platinum(II) with derived sulfonamide ligand: structure, DFT study, antibacterial and cytotoxicity activities. J Mol Struct. 2018;1161:345–355.
- Bhagyasree JB, Varghese HT, Panicker CY, et al. Vibrational spectroscopic (FT-IR, FT-Raman, (1)H NMR and UV) investigations and computational study of 5-nitro-2-(4-nitrobenzyl) benzoxazole, spectrochim. Acta Part A Mol Biomol Spectrosc. 2013;102:99–113.
- Olasunkanmi LO, Obot IB, Ebenso EE. Adsorption and corrosion inhibition properties of N-{n-[1-R-5-(quinoxalin-6-yl)-4, 5-dihydropyrazol-3-yl] phenyl} methanesulfonamides on mild steel in 1 M HCl: experimental and theoretical studies. RSC Adv. 2016;6 :86782–86797.
- El Adnani Z, Mcharfi M, Sfaira M, et al. DFT theoretical study of 7-R-3methylquinoxalin-2 (1H)-thiones (RH; CH3; Cl) as corrosion inhibitors in hydrochloric acid. Corros Sci. 2013;68:223–230.
- Mi H, Xiao G, Chen X. Theoretical evaluation of corrosion inhibition performance of three antipyrine compounds. Comput Theor Chem. 2015;1072 :7–14.
- Messali M, Larouj M, Lgaz H, et al. A new Schiff base derivative as an effective corrosion inhibitor for mild steel in acidic media: experimental and computer simulations studies. J Mol Struct. 2018;1168 :39–48.
- Roy R, Krishnamurti S, Geerlings P, et al. Local softness and hardness based reactivity descriptors for predicting intra-and intermolecular reactivity sequences: carbonyl compounds. J Phys Chem A. 1998;102:3746–3755.
- Roy R, de Proft Fd, Geerlings P. Site of protonation in aniline and substituted anilines in the gas phase: a study via the local hard and soft acids and bases concept. J Phys Chem A. 1998;102:7035–7040.
- Roy RK, Pal S, Hirao K. On non-negativity of Fukui function indices. J Chem Phys. 1999;110:8236–8245.
- Wilding B, Klempier N. Newest developments in the preparation of thieno[2,3-d] pyrimidines. Org Prep Proced Int. 2017;49:183–215.
- Fayed EA, Eissa SI, Bayoumi AH, et al. Design, synthesis, cytotoxicity and molecular modeling studies of some novel fluorinated pyrazole-based heterocycles as anticancer and apoptosis-inducing agents. Mol Divers. 2019;23:165–181.
- Safwat GM, Hassanin K, Mohammed ET, et al. Synthesis, anticancer assessment, and molecular docking of novel chalcone-thienopyrimidine derivatives in HepG2 and MCF-7 cell lines. Oxid Med Cell Longev. 2021.
- Shaaban MA, Ghorab MM, Heiba HI, et al. Novel thiophenes, thienopyrimidines, and triazolothienopyrimidines for the evaluation of anticancer and augmentation effects of γ-radiation. Arch Pharm. 2010;343:404–410.
- Omar A. Review article; anticancer activities of some fused heterocyclic moieties containing nitrogen and/or sulfur heteroatoms. Al-Azhar J Pharm Sci. 2020;62:39–54.
- El-Metwally SA, Khalil AK, El-Sayed WM. Design, molecular modeling and anticancer evaluation of thieno[2,3-d]pyrimidine derivatives as inhibitors of topoisomerase II. Bioorg Chem. 2020;94:103492.