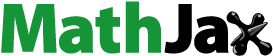
Abstract
This paper aimed to examine the impact of flavonoid osajin (OSJ) on liver damage induced by sepsis. A total of 30 male rats were divided into 5 groups (Sham, sepsis, OSJ 150, OSJ 300 and reference). During sepsis, elevated lipid peroxidation (LPO) level and catalase activity (CAT) and decreased glutathione (GSH) level and superoxide dismutase (SOD) activity were observed in hepatic tissues of sepsis group in comparison with Sham group. A strong interleukin-33 and caspase-3 expressions were detected in hepatic tissues of sepsis group. On the contrary, OSJ administration to OSJ 300 group showed a significant decrease (P < 0.001) in LPO level (176±2.926) and significant increase (P < 0.001) in GSH level (10.586±0.083) and SOD activity (29.152±0.094) in comparison with sepsis group (185.777±1.735, 8.246±0.124, 24.307±0.379 respectively). In addition, the consumption of OSJ reduced expressions of interleukin-33 and caspase-3 and improved histopathological integrity. In conclusions, OSJ has hepatoprotective effect against sepsis-induced liver injury.
KEYWORDS:
1. Introduction
Sepsis remains to be the main cause of increased mortality in the intensive care units (ICUs). In 2017, about 48.9 million people worldwide suffered from sepsis and 11 million deaths related to sepsis have been reported, representing 19.7% of all universal deaths [Citation1]. Sepsis is defined as life-threatening organ dysfunction caused by an excessive immune response against infection [Citation2]. Sepsis pathogenesis includes infection, excessive inflammatory response, activation of coagulation system that leads to local perfusion defects and tissue hypoxia [Citation3,Citation4]. During sepsis, neutrophils and macrophages produce reactive oxygen species (ROS) such as superoxide radical (O2•-) and hydroxyl radical (•OH) [Citation5] that directly attack endothelial cells, thus contribute to multiple organ failure [Citation6]. The imbalance between antioxidants and ROS production causes increased oxidants in cells called oxidative stress. There are increasing evidences about the implication of oxidative stress in sepsis pathogenesis. Moreover, oxidative stress has been considered a cornerstone on the pathogenesis of sepsis [Citation7]. If sepsis pathogenesis were limited only to microorganisms and inflammation, cases of sepsis would respond to treatment with anti-inflammatories. Anti-inflammatory drugs that are used to treat sepsis were frustrating [Citation8,Citation9]. Rowe et al. showed that ROS induced antibiotic tolerance during systemic infection by Staphylococcus aureus [Citation10]. In addition, oxygen delivery did not diminish mortality among septic patients [Citation11]. The liver is one of the most important organs affected by sepsis. It plays an important role in homeostasis by being a primary centre for the metabolism and synthesis of bile, urea and clotting factors and detoxification. Because of these vital functions of the liver, its damage during sepsis complicates sepsis pathway. The role of liver cells in metabolism is modified towards the inflammatory response during sepsis. Various cytokines and ROS, that are excessively produced by kupffer cells, devastate liver endothelial cell and hepatocytes [Citation12], thus contributing to hepatic failure and increase mortality. Accordingly, targeting oxidative stress and inflammation could be an effective strategy for treating sepsis. Due to its therapeutic properties, Maclura pomifera (Raf.) Schneid. plant has been widely used in folk medicine. Many studies have shown that extracts of fruits, leaves and roots of Maclura pomifera contain antioxidants and anti-inflammatory agents such as flavonoids osajin (OSJ) and pomiferin. In addition, OSJ from Maclura pomifera attenuated myocardial injury [Citation13], ovarian damage [Citation14] and kidney harm [Citation15] induced by ischemia-reperfusion (IR) in rats through inhibiting oxidative stress. Based on these evidences, our present study aimed to examine the protective effect of isolated OSJ from the fruits of Maclura pomifera against liver harm caused by sepsis in rats. To achieve this aim, lipid peroxidation (LPO), glutathione (GSH), superoxide dismutase (SOD) and catalase (CAT) as parameters of oxidative stress as well as, interleukin-33 (IL-33) and caspase-3 as indicators of inflammation and programmed cell death (apoptosis) were measured in hepatic tissues of rats.
2. Materials and methods
2.1 Plant material
Maclura pomifera (Raf.) Schneid fruits were collected from a park in Kilis/Turkey by Murat KOC, Public Health Institute, Ankara Yildirim Beyazit University, Ankara/Turkey. The samples were examined by the same researcher and identification and verification of the species were performed using international diagnostic methods. The samples were given the code M.Koç 1292 and stored in the Herbarium of Department of Biology, Yozgat Bozok University (Yozgat/Turkey). OSJ was separated and purified using chromatographic methods. Spectroscopic methods (UV-VIS, IR, NMR) were used to confirm the chemical structure of OSJ (Figure ).
Briefly, the dry fruits were ground and extracted with ethyl acetate 5 times under room temperature. Silica gel (Kieselgel 60, 70–230 mesh, Merck) column chromatography was used with mobile phase (chloroform-ethyl acetate, 8:2) for first separation. Pure 15 g of OSJ was obtained by using TLC silica gel (Kieselgel 60 F254, 0.2 mm, Merck, 5554) with mobile phase (ethyl acetate: chloroform, 2:8). The spots were determined at UV254 and UV366 nm in the UV lamp.
The structure was elucidated by IR, 1H-NMR, 13C-NMR, DEPT-NMR, 1H,1H-COSY, HMQC and HMBC spectroscopic methods (IR and NMR results were added in Supplementary Data, Supp Data 1).
Characterization of OSJ: IR (KBr, v, cm−1) 3437, 3229, 2980, 2932, 2128, 1651, 1568, 1434, 1205, 1074. 1H-NMR (400 MHz, CDCl3, δ, ppm)1.51 (6H, s, CH3), 1.71 (3H, s, CH3), 1.80 (3H, s, CH3), 3.40 (2H, d, J = 7.3 Hz, CH2), 5.20 (1H, d, J = 7.3 Hz, C = CH), 5.58–5.68 (1H, m, C = CH), 6.68–6.76 (1H, m, C = CH), 6.78–6.83 (2H, m, PhH), 7.26–7.35 (2H, m, PhH), 7.88 (1H, d, C = CH), 13.03 (1H, s, OH), 13.07 (1H, s, OH). 13C-NMR (100 MHz, CDCl3, δ, ppm) 18.0, 21.5, 25.0, 28.5, 77.7, 100.8, 105.7, 107.7, 115.0, 115.8, 121.9, 123.4, 123.7, 128.2, 130.4, 131.8, 152.8, 154.8,156.2, 157.1, 159.3, 181.5 (C25H24O5; MA:404 g/mol).
Details of the isolation and purification of OSJ and confirmation of the chemical structure of this flavonoid can be found in the previous paper Citation16.
2.2 Chemicals
All chemicals were obtained from Sigma Chemical Co (Munich, Germany).
2.3 Animals
A total of 30 adult male Sprague Dawley rats (weight 200–250 g) were kept in the medical experimental application and research center, Ataturk University, Erzurum/ Turkey under standard laboratory conditions (Temperature 22°C ± 2, 12/12 h light–dark cycle, ideal diet for laboratory rodents with water ad libitum).
2.4 Technique of cecal ligation and puncture (CLP)
Rats were fasted for 12 hours before the surgery. Then, general anaesthesia was administered by intraperitoneal injection of 25 mg/kg thiopental sodium. After shaving the abdominal skin, laparotomy was achieved through a 3-cm midline incision. CLP technique was carried out for inducing polymicrobial sepsis as described previously by Fujimura et al. [Citation17]. Briefly, the cecum was pulled across the peritoneum and muscles. The ascending colon was gently pressed. Then the cecum was tied under the ileocecal valve using silk thread 4.0. To allow faecal contents to spread into the peritoneum, the ventral side of the cecum was punctured twice with 18-gauge needle. After that, the cecum and the intestine section were returned and the abdomen was closed with suture using 3.0 silk thread.
2.5 Experimental design
The animals were randomly divided into five groups (6 ones in each group) and treated as follows:
Sham group: The surgical procedure was performed without applying the CLP technique.
Sepsis group: CLP technique.
OSJ 150 group: CLP technique + administration of OSJ orally at a dose of 150 mg/kg body weight.
OSJ 300 group: CLP technique + administration of OSJ orally at a dose of 300 mg/kg body weight.
Reference group (REF group): CLP technique + administration of ceftriaxone intraperitoneally at a dose of 50 mg/kg body weight.
The doses of 150 and 300 mg/kg of OSJ obtained from the fruits of Maclura pomifera were prepared as a suspension in distilled water and the solutions (1 ml for each rat) were administered orally by using a steel gavage needle (G 18, FST, California, USA). Ceftriaxone, which was used as a standard drug compared to OSJ, was prepared at the dose of 50 mg/kg and given to the rats of the REF group intraperitoneally.
OSJ and ceftriaxone were administered 15 min before applying CLP. Under general anaesthesia by thiopental sodium 50 mg/kg, all the rats were sacrificed 24 h after the operation and the livers were promptly removed. Two biopsies were taken from each liver. Part of these biopsies was kept at −80°C for biochemical analysis, while the other section of biopsies was fixed with 10% formalin solution for histopathological and immunohistochemical examination. All procedures on rats were in accordance with the European/international standards and were approved by the local ethics council of animal experiments at Ataturk University, Erzurum/Turkey (Approval No: 133, Date 1.09.2015).
2.6 Biochemical analysis
All biochemical parameters were measured in the tissue of liver. The tissue homogenizer machine “TissueLyser II (Qiagen, Hilden, Germany)’ was used for crushing the frozen liver tissues with liquid nitrogen. The mashed tissues were homogenized using tissue homogenizer machine “TissueLyser II (Qiagen, Hilden, Germany)” in appropriate buffer and centrifuged using a refrigerated centrifuge, “Universal 320R (Hettich, Tuttlinger, Germany)” at 4°C. All biochemical parameters were measured in the supernatants obtained after centrifugation by “Spectrophotometer Epoch (Bio-Tek, USA)”.
2.6.1 Determination of LPO
Malondialdehyde (MDA), which is the final product of LPO, was detected in hepatic tissues using thiobarbituric acid (TBA) test for the measurement of LPO according to the method previously described by Ohkawa et al. [Citation18]. Briefly, the mashed liver tissue (25 mg) was homogenized into 2.5 ml of KCL(10%) buffer. The homogenates were centrifuged at 4000 rpm and 4°C for 30 min. LPO level was measured in the supernatants. The solution consisting of 100 µl sodium lauryl sulphate, 750 µl acetic acid, 750 µl TBA and 150 µl distilled water was added to 250 µl supernatant. The mixture was incubated at 100°C for 60 minutes. 2.5 ml of n-butanol:pyridine mix was added to the mixture. The mixture was vortexed and centrifuged. The supernatant was measured at 532 nm. LPO level in tissue was calculated by utilizing the standard curve which obtained using 1,1,3,3-tetramethoxypropane. The result was expressed as nmol MDA/g tissue.
2.6.2 Determination of GSH
The method previously described by Sedlak and Lindsay [Citation19] and based on the reaction between 5,5′-Dithiobis (2-nitrobenzoic acid) (DTNB) and the sulfhydryl group of GSH was used for the determination of GSH level in hepatic tissue. Briefly, the mashed liver tissue (25 mg) was homogenized into 2.5 ml of 50 mmol Tris–HCl (pH 7.4) buffer. The homogenates were centrifuged at 4000 rpm and 4°C for 30 minutes. GSH level was measured in obtained supernatants. The solution consisting of 25 µl DTNB, 1975 µl methanol and 375 µl Tris buffer (EDTA and Tris–HCl) was added to 125 µl supernatant. The mixture was vortexed and incubated at 37°C for 30 minutes. The supernatant was measured at 412 nm. GSH level in tissue was calculated using the standard curve of GSH. The result was expressed as nmol/mg tissue.
2.6.3 Determination of SOD
SOD activity was measured according to the method formerly described by Sun et al. [Citation20]. SOD activity was determined by monitoring the capability of SOD to block the reaction of formazan dye formation from nitro blue tetrazolium (NBT) in the presence of O2•– which generated from xanthine by xanthine oxidase. Briefly, the mashed liver tissue (25 mg) was homogenized into 2.5 ml of 50 mmol KH2PO4,10 mmol EDTA (pH 7.8) buffer. The homogenates were centrifuged at 6000 rpm and 4°C for 60 minutes. SOD activity was measured in obtained supernatants. 980 µl of measuring mix (Xanthine solution, EDTA solution, NBT solution, Na2CO3 solution, Bovine Serum Albumin solution) and 20 µl of xanthine oxidase solution were added to 200 µl of supernatant. The mixture was vortexed and incubated at 25°C for 20 minutes. The absorbance of formazan dye was measured at 560 nm and the formula specified in the cited reference was used to calculate SOD activity. Data were expressed as mmol/min/mg tissue.
2.6.4 Determination of CAT
CAT activity was measured using the method already described by Aebi [Citation21] through detecting the rate of hydrogen peroxide (H2O2) conversion to H2O at 240 nm. Briefly, the mashed liver tissue (25 mg) was homogenized into 2.5 ml of 50 mm KH2 PO4 phosphate buffer (pH 7). The homogenates were centrifuged at 10000 g and 4°C for 60 minutes. CAT activity was measured in obtained supernatants. 100 µl from 30 mm H2O2 solution was added to 200 µl supernatant. The absorbance was measured at 240 nm. The decrease in absorbance was monitored for 3 minutes. The formula specified in the cited reference was used to calculate CAT activity and the results were expressed as µmol/min/mg tissue.
2.7 Histopathological examination
Livers were fixed in 10% neutral formalin solution, washed by water, passed through alcohol and xylol chain and implanted into paraffin. Sections 4 µm thicknesses were prepared. Histopathological evaluation was carried out using light microscope “Leica DM 1000 (Leica Microsystems, Wetziar, Germany)” after staining sections with haematoxylin and eosin (H&E). The examined histological slides were evaluated according to the severity of the lesions, no (–), mild(+), moderate (++) and severe (+++).
2.8 Immunohistochemical study
All sections taken to adhesion (Poly-L-Lysine) lames were passed through the xylol and alcohol chain. Endogenous peroxidase inactivation was achieved by placing the sections in 3% H2O2 for 10 minutes after washing with PBS. The tissues were treated with antigen retrieval solution in the microwave oven at 500 watts for 2 × 5 minutes and then allowed to cool. Tissues were incubated at 37˚C for 30 minutes with the primary antibodies of caspase-3 and IL-33 (Catholock no: ab184787, dilution ratio: 1/100, Catholock no: ab187060, dilution ratio: 1/100). Immunohistochemistry was followed according to the kit procedure (AbcamHRP/ DAB Detection IHC kit). 3–3’Diaminobenzidine (DAB) was used as a chromogen. Background staining was performed with hematoxylin. Sections were evaluated according to their immune positivity as no (–), mild (+), moderate (++) and severe (+++).
2.9 Statistical analysis
The biochemical results were reported as mean ± standard error (SE). The data were analysed by SPSS program (version 20). Kolmogorov–Smirnov test was used for checking normality of data distribution (P > 0.05). One-way analysis of variance (ANOVA) followed by Duncan's multiple range test was used to detect the significant differences among the means. P < 0.05 was accepted to be the least limit of significance. SPSS program (version 13) was used for statistical analyses of histopathological findings. Histopathological and immunohistochemical scores were analysed using non-parametric Kruskal–Wallis test to determine the differences between the groups and Mann–Whitney U test for paired comparisons.
3. Results
3.1 Biochemical examination of liver tissue
As to the levels of LPO indicated in (Figure A), our results showed a significant increase (P < 0.001) for sepsis group (239.333 ± 3.306) in comparison with the sham group (185.777 ± 1.735). The administration of OSJ showed a significant decrease (P < 0.001) in OSJ 300 group (176 ± 2.926) in comparison with the sepsis group (185.777 ± 1.735). A significant decrease (P = 0.008) was observed in OSJ 300 group (176 ± 2.926) compared to REF group (192.222 ± 2.574). Regarding the levels of GSH shown in (Figure B), sepsis caused a significant decrease (P < 0.001) in sepsis group (8.246 ± 0.124) compared to sham group (11.891 ± 0.149), while this level significantly increased (P < 0.001) in each of OSJ 150 (10.213 ± 0.128), OSJ 300 (10.586 ± 0.083) and REF (11.703 ± 0.148) groups compared to sepsis group (8.246 ± 0.124). With regard to SOD activity shown in Figure (C), our findings demonstrated a significant decrease (P < 0.001) in the sepsis group (24.307 ± 0.379) in comparison with the sham group (31.989 ± 0.496). On the other hand, OSJ significantly (P < 0.001) increased this activity for OSJ 150 (27.335 ± 0.373) and OSJ 300 (29.152 ± 0.094) groups compared to the sepsis group (24.307 ± 0.379). This activity significantly increased (P = 0.001) in OSJ 300 group (29.152 ± 0.094) compared to OSJ 150 group (27.335 ± 0.373). On the subject of CAT activity demonstrated in (Figure D), a considerable elevation (P < 0.001) was observed in the sepsis group (1060.349 ± 15.665) in comparison with the sham group (814.156 ± 19.496). CAT activity significantly decreased (P = 0.011) in OSJ 300 group (857.021 ± 26.659) compared to OSJ 150 group (947.456 ± 23.571).
Figure 2. Oxidative stress parameters in hepatic tissue. A: (LPO level). B: (GSH level). C: (SOD activity). D: (CAT activity). Data are given as mean ± standard error (SE); n = 6. Different small letters indicate significant difference for each parameter among means (P < 0.05). Significant differences were determined by using one-way analysis of variance (ANOVA) followed by Duncan's multiple-range test. LPO: Lipid peroxidation, GSH: Glutathione, SOD: Superoxide dismutase, CAT: Catalase, OSJ: Osajin and REF: Reference (Ceftriaxone).

3.2 Histopathological examination of liver
The normal histological structure was observed in hepatic tissues of the sham group (Figure A). When the hepatic tissues of the sepsis group were examined, severe histopathological changes were observed such as inflammation, mononuclear cell infiltration in serosa and parenchyma, degeneration and coagulative necrosis in hepatocytes (Figure B). The severity degree of these changes decreased to moderate in OSJ 150 group (Table , Figure C) and to mild in OSJ 300 (Table , Figure D) and REF (Table , Figure E) groups.
Figure 3. Photomicrography of liver sections. A (Sham group): Normal histological appearance, B (Sepsis group): Severe inflammation in the serosa, mononuclear cell infiltration (star), necrosis (arrows) and degeneration (arrowheads) in hepatocytes, C (OSJ 150 group): Moderate mononuclear cell infiltration (star), degeneration (arrowheads) and necrosis (arrows) in hepatocytes, D (OSJ 300 group): Mild degeneration of hepatocytes (arrowhead), E (REF group): Mild degeneration of hepatocytes (arrowhead), H&E, Bar: 20µm.

Table 1. Histopathological and immunohistochemical assessment of liver tissue.
3.3 Immunohistochemical examination of liver
IL-33 and caspase-3 expressions were negative in the liver tissues for the sham group (Table , Figure A and Figure A). Unlike, strong expression of IL-33 was detected in sinusoids, portal spaces and mononuclear cells and severe expression of cytoplasmic caspase-3 was observed in hepatocytes in the sepsis group (Table , Figure B and Figure B). The degrees of these expressions decreased to moderate in OSJ 150 group (Table , Figure C and Figure C) and to mild in OSJ 300 (Table , Figure D and Figure D) and REF (Table , Figure E and Figure E) groups.
Figure 4. IL-33 expression of liver sections. A (Sham group): Negative IL-33 expression, B (Sepsis group): Severe IL-33 expression in mononuclear cells cytoplasm, portal and sinusoidal spaces (arrowheads), C (OSJ 150 group): Moderate IL-33 expression in portal and sinusoidal spaces (arrowheads), D (OSJ 300 group): Mild IL-33 expression (arrowheads) in the portal and sinusoidal spaces, E (REFgroup): Mild IL-33 expression (arrowheads) in the portal and sinusoidal spaces, IHC-P, Bar: 20µm

Figure 5. Caspase-3 expression of liver sections. A (Sham group): Negative expression of cytoplasmic caspase-3, B (Sepsis group): Severe expression of cytoplasmic caspase-3 in hepatocytes (arrowheads), C (OSJ 150 group): Moderate expression of cytoplasmic caspase-3 in hepatocytes (arrowheads), D (OSJ 300 group): Mild caspase-3 expression (arrowheads), E (REFgroup): Mild caspase-3 expression in hepatocytes (arrowheads), IHC-P, Bar: 20 µm.

4. Discussion
Despite the accepted role of oxidative stress in the development of organ failure in sepsis, the definitive evidences about the beneficial effects of antioxidants are insufficient in sepsis patients [Citation22]. We examined the impact of OSJ on liver damage during sepsis by assessing oxidative stress, inflammation, apoptosis and histopathologic structure in the livers excised at 24 h after CLP induction.
In normal condition, there is a balance between generating oxidants and their scavenging by antioxidants. Increased levels of LPO, decreased levels of GSH and reduced activity of SOD are indications of the development of oxidative stress in the sepsis group in this study. During sepsis, excessively produced ROS attack polyunsaturated fatty acids located in the cell membrane and give rise to LPO [Citation23]. Today, LPO is accepted as an indicator of oxidative stress, as well as cell and tissue damage. MDA, which is the final product of LPO, interacts with proteins, nucleic acids and glutathione, thus leads to tissue damage and many diseases [Citation24]. The sulfhydryl (SH) group in the structure of proteins undergoes oxidation by reacting with hydrogen peroxide (H2O2) [Citation25]. The high concentration of H2O2, which was inferred by the high activity of CAT in this study, is believed to be the reason for the sabotage of the SH group in the GSH structure. The measuring principle of GSH in liver tissue is based on the interaction between DTNB and SH group of GSH. This pathogenesis may be implicated in decreased GSH levels observed in the sepsis group. The conversion of O2•− into oxygen and H2O2 is catalysed by SOD [Citation26]. Zhong et al. showed that sepsis decreased the activity of SOD in the livers of mice [Citation27]. Diminished SOD activity in this study indicates that the livers of the sepsis group were not protected from the attack of O2•−. Enhanced activity of any enzyme may be related to improved production of its substrate. CAT enzyme diminishes ROS level by catalysing H2O2 decomposition into water and oxygen [Citation28]. Increased CAT activity in the sepsis group in this study is an indication of the high availability of H2O2 in the hepatic tissues of this group. Increased levels of O2•− and H2O2 were observed in livers of mice with sepsis in an experimental study conducted by Zhong et al. [Citation27]. In the case of fatty liver, which is a manifestation of sepsis, fatty hepatocytes generate more H2O2 than healthy hepatocytes [Citation29]. Generally, stimulation of endogenous antioxidants is an important cellular response for protecting from oxidative stress [Citation14]. We think that this cellular response was accountable for the high activity of CAT enzyme in sepsis group in this study, especially CAT enzyme is present primarily in the peroxisomes in mammals [Citation30], so it is protected from the influence of ROS. Unlike SOD enzyme, which is mainly found in mitochondria and cytoplasm [Citation30], is directly exposed to ROS attack. In general, sepsis is associated with a clear difference in the activity of these two enzymes.
Although the antioxidant capacity of OSJ was low by using the spectrophotometric test, a strong protective effect of OSJ was observed against oxidative damage of DNA by using Fenton’s reaction [Citation31]. OSJ from Maclura pomifera attenuated myocardial injury [Citation13], ovarian damage [Citation14] and kidney harm [Citation15] induced by IR in rats through inhibiting oxidative stress and changing in antioxidant system. In addition, Bozkurt et al. demonstrated that isolated pomiferin from Maclura pomifera protected against gastric ulcer in rats through reducing oxidative stress [Citation32]. On the other hand, acetylsalicylic acid, the active ingredient of aspirin, is actually not an effective anti-inflammatory agent. It is activated after entering the body and metabolized to salicylic acid. Likewise, OSJ itself was found to have no antioxidant property in in vitro trials, but in vivo results demonstrated this property. Therefore, we may think that OSJ has antioxidant potential. In our study, OSJ markedly abolished oxidative stress by attenuating LPO levels and returning GSH levels and SOD and CAT activities almost to normal values. The decrease of LPO levels and the improvement of the antioxidant system after treating by OSJ in this study can be attributed to the potential of OSJ or its metabolites as an antioxidant in scavenging free radicals and ROS that are excessively produced due to the irregular immune response.
We performed the hepatic histopathological examination to support the biochemical findings and to obtain a visualization of the structure of hepatic tissue in septic rats and in septic rats treated with different doses of OSJ. The elevation of LPO and breakdown of the antioxidant system were accompanied by histopathological changes in the hepatic tissue of the sepsis group, most notably, necrosis, infiltration of mononuclear cells, acute inflammation and degeneration. A previous study showed similar results when compared with ours [Citation33]. Fibrin deposition and intravascular coagulopathies during sepsis lead to cell necrosis due to oxygen deprivation. Then inflammation often occurs as a result of phagocytosis of the necrotic tissue [Citation34]. Based on the disorders described above, we think that necrosis observed in the livers of the sepsis group is associated with LPO-induced cell membrane damage and tissue hypoxia as necrosis begins by damaging cell membrane [Citation34]. OSJ administration caused a considerable decline in the pathological changes depending on the used dose. Cigsar et al. showed that OSJ attenuated infiltration of leukocytes and reversible and irreversible cell damage in ovarium tissues [Citation14]. Reduced degeneration in the OSJ 300 group may be an indication of the possible role of OSJ in alleviating steatosis during sepsis.
We carried out immunohistochemical examination for IL-33 and caspase-3. Endothelial cells, epithelial cells and fibroblasts, which are the first line of defence against pathogens, mainly produce IL-33 [Citation35]. IL-33 is released when these cells are damaged. Released IL-33 activates different types of immune system cells, thus it has a pivotal role in initiating and perpetuating inflammation [Citation36]. We observed that OSJ suppressed the progression of inflammation by attenuating the expression of IL-33. Scandenone, which is an isoflavone isolated from the fruits of Maclura pomifera, exhibited anti-inflammatory activity in in vivo experimental study [Citation37]. OSJ is a chemically structural isomer of scandenone [Citation37]. A strong anti-inflammatory activity for copper complexes that contain flavonoid osajin, was detected in in vitro study by reducing of nuclear factor kappa-B/activator protein 1 (NF-κB /AP-1) system and tumor necrosis factor-α (TNF-α) [Citation38].
During the host response to the pathogen, protein complexes called inflammasomes are formed by aggregation of some pattern recognition receptors of innate immune cells. These massive protein complexes are responsible for the production of caspases which are involved in apoptosis during sepsis [Citation4]. Caspase-3 is the most important caspase that executes apoptosis by inducing DNA degradation [Citation39]. In addition to the proven role of MDA in inducing apoptosis [Citation23], released IL-33 in large amount from liver cells, in response to hepatic tissue damage, may be a potent inducer of apoptosis in this study through its role in the stimulation of mast cells, macrophages and dendritic cells to secrete TNF-α [Citation36] that triggers apoptosis in the target cell by activation caspase-8 and caspase-3 [Citation34]. Therefore, blocking IL-33 and MDA may be an influential way to attenuate apoptosis in sepsis. The usage of OSJ resulted in a clear decrease in apoptosis by attenuating the expressions of IL-33 and caspase3. The greatest effect was observed in the OSJ 300 group.
The possible mechanisms of OSJ in alleviating oxidative stress, inflammation and apoptosis can be summarized in Figure . More studies are needed to clarify the exact mechanisms underlying the effect of OSJ.
Figure 6. Schematic figure of the possible mechanisms of OSJ in the alleviation of liver damage induced by sepsis. CLP: Cecal ligation and puncture, ROS: Reactive oxygen species, LPO: Lipid peroxidation, MDA: Malondialdehyde, TNF-α: Tumour necrosis factor, IL-33: interleukin-33, CAT: Catalase, GSH: Glutathione and SOD: Superoxide Dismutase.

Ceftriaxone, which is a member of third-generation cephalosporins, was used as a standard drug compared to OSJ. It is a broad-spectrum antibiotic and commonly prescribed as an empirical or documented treatment in ICUs for a wide range of infections [Citation40]. The results obtained by using the dose of 300 mg/kg OSJ were approximately close to those obtained by using the dose of 50 mg/kg ceftriaxone. On the other hand, we think that the early use of ceftriaxone in this study played a critical role in curtailment liver injury in the REF group.
The possible protective effect of OSJ against sepsis-induced liver injury was examined by checking parameters of oxidative stress (LPO, GSH, SOD, CAT), inflammation (IL-33) and apoptosis (Caspase-3) as well as, histopathologic structure in hepatic tissues of rats. Due to the role of IL-33 in releasing of inflammatory cytokines by activating various types of immune system cells during sepsis [Citation36], the level of inflammatory cytokines such as IL-1β, IL-6, TNF-α were not measured in this study. Because of the critical role of MDA in tissue damage [Citation24], ALT and AST, as markers of liver injury, also were not performed in serum. However, checked parameters gave promising results about the protective effect of OSJ against sepsis-induced liver injury.
5. Conclusion
There is no doubt that liver failure complicates sepsis and plays a primary role in multiple organ dysfunction syndrome. Because of the critical role of oxidative stress, inflammation and apoptosis in liver damage during sepsis, targeting these perturbations is one of the most important foundations of sepsis therapy. This is the first work evaluating the hepatoprotective effect of OSJ from Maclura pomifera against sepsis-associated liver injury by assessing oxidative stress parameters, initiator of inflammation and the cause of its continuation (IL-33) and executor apoptosis (Caspase-3). In conclusion, we demonstrate that OSJ is capable to reverse liver damage during sepsis by blocking oxidative stress, inflammation and apoptosis. Thus OSJ may be suggested as a promising therapy in the management of sepsis-associated liver injury.
Acknowledgments
This study was produced from the doctoral thesis (Investigation of the Effects of Osajin Substance Isolated from Maclura pomifera Plant on Cecal Ligation-Puncture Induced Sepsis Model at Rats). (Mohammad Alhilal, Graduate School of Health Sciences, Ataturk University, 2021, Erzurum, Turkey).
Ethics approval and animal rights statement
All procedures on rats were in accordance with the European/international standards and were approved by the local ethics council of animal experiments at Ataturk University, Erzurum/Turkey (Approval No: 133, Date 1.09.2015).
Author contributions
MA, HSE, DC and MBH participated in project planning, experimental design, experimental work and data analysis. MK performed plant collection and systematics studies. AC carried out experiments for isolation and characterization. MA, HSE and DC achieved experiments for CLP animal models. MA and SY performed statistical analyses. SY executed experiments for histopathological and immunohistochemical analysis. MA, HSE and MBH performed biochemical analyses. MBH supervised the study, wrote the paper with MA and MA committed to writing the correspondence of the manuscript. All authors commented on previous versions of the manuscript and approved the final article.
Disclosure statement
No potential conflict of interest was reported by the author(s).
Additional information
Funding
References
- Rudd KE, Johnson SC, Agesa KM, et al. Global, regional, and national sepsis incidence and mortality, 1990–2017: analysis for the global burden of disease study. Lancet. 2020;395:200–211. doi:10.1016/S0140-6736(19)32989-7.
- Singer M, Deutschman CS, Seymour C, et al. The third international consensus definitions for sepsis and septic shock (sepsis-3). J Am Med Assoc. 2016;315:801–810. doi:10.1001/jama.2016.0287.
- Prauchner CA. Oxidative stress in sepsis: pathophysiological implications justifying antioxidant co-therapy. Burns. 2017;43:471–485. doi:10.1016/j.burns.2016.09.023.
- Gyawali B, Ramakrishna K, Dhamoon AS. Sepsis: the evolution in definition, pathophysiology and management. SAGE Open Med. 2019;7:1–13. doi:10.1177/2050312119835043.
- Riedemann NC, Guo RF, Ward PA. Novel strategies for the treatment of sepsis. Nat Med. 2003;9:517–524. doi:10.1038/nm0503-517.
- Parihar A, Parihar MS, Milner S, et al. Oxidative stress and anti-oxidative mobilization in burn injury. Burns. 2008;34:6–17. doi:10.1016/j.burns.2007.04.009.
- Mantzarlis K, Tsolaki V, Zakynthinos E. Role of oxidative stress and mitochondrial dysfunction in sepsis and potential therapies. Oxid Med Cell Longevity. 2017;2017:1–10. doi:10.1155/2017/5985209.
- Sprung CL, Annane D, Keh D, et al. Hydrocortisone therapy for patients with septic shock. N Engl J Med. 2008;358:111–124. doi:10.1056/NEJMoa071366.
- Lefering R, Neugebauer EAM. Steroid controversy in sepsis and septic shock. A meta-analysis. Crit Care Med. 1995;23:1294–1303. doi:10.1097/00003246-199507000-00021.
- Rowe SE, Wagner NJ, Li L, et al. Reactive oxygen species induce antibiotic tolerance during systemic staphylococcus aureus infection. Nat Microbiol. 2020;5:282–290. doi:10.1038/s41564-019-0627-y.
- Gattinoni L, Brazzi L, Pelosi P, et al. A trial of goal-oriented hemodynamic therapy in critically III patients. Intensivmed Notfallmedizin. 1995;34:740–743.
- Woznica EA, Inglot M, Woznica RK, et al. Liver dysfunction in sepsis. Adv Clin Exp Med. 2018;27:547–551. doi:10.17219/acem/68363.
- Florian T, Necas J, Bartosikova L, et al. Effects of prenylated isoflavones osajin and pomiferin in premedication on heart ischemia-reperfusion. Biomed Pap-Olomouc. 2006;150:93–100. doi:10.5507/bp.2006.013.
- Cigsar G, Keles ON, Can S, et al. The protective effects of osajin on ischemia/reperfusion injury to rat ovaries: biochemical and histopathological evaluation. Kafkas Universitesi Veteriner Fakultesi Dergisi. 2015;21:753–760. doi:10.9775/kvfd.2015.13387.
- Bartošíková L, Nečas J, Suchý V, et al. Protective effects of osajin in ischemia-reperfusion of laboratory rat kidney. Pharmazie. 2006;61:552–555.
- Erol HS, Cakir A, Koc M, et al. Anti-ulcerogenic effect of osajin on indomethacin-induced gastric damage in rats. Acta Vet Brno. 2020;89:391–400. doi:10.2754/avb202089040391.
- Fujimura N, Sumita S, Aimono M, et al. Effect of free radical scavengers on diaphragmatic contractility in septic peritonitis. Am J Respir Crit Care Med. 2000;162:2159–2165. doi:10.1164/ajrccm.162.6.9912144.
- Ohkawa H, Ohishi N, Yagi K. Assay for lipid peroxides in animal tissues by thiobarbituric acid reaction. Anal Biochem. 1979;95:351–358. doi:10.1016/0003-2697(79)90738-3.
- Sedlak J, Lindsay RH. Estimation of total, protein-bound, and nonprotein sulfhydryl groups in tissue with Ellman’s reagent. Anal Biochem. 1968;25(C):192–205. doi:10.1016/0003-2697(68)90092-4.
- Sun Y, Oberley LW, Li Y. A simple method for clinical assay of superoxide dismutase. Clin Chem. 1988;34:497–500. doi:10.1093/clinchem/34.3.497.
- Aebi H. [13] Catalase in vitro. Methods Enzymol. 1984;105(C):121–126. doi:10.1016/S0076-6879(84)05016-3.
- Galley HF. Oxidative stress and mitochondrial dysfunction in sepsis. Br J Anaesth. 2011;107:57–64. doi:10.1093/bja/aer093.
- Su LJ, Zhang JH, Gomez H, et al. Reactive oxygen species-induced lipid peroxidation in apoptosis, autophagy, and ferroptosis. Oxid Med Cell Longevity. 2019;2019:1–14. doi:10.1155/2019/5080843.
- Nam TG. Lipid peroxidation and its toxicological implications. Toxicol Res. 2011;27:1–6. doi:10.5487/TR.2011.27.1.001.
- Vignais PV. The superoxide-generating NADPH oxidase: structural aspects and activation mechanism. Cell Mol Life Sci. 2002;59:1428–1459. doi:10.1007/s00018-002-8520-9.
- Wang Y, Branicky R, Noë A, et al. Superoxide dismutases: dual roles in controlling ROS damage and regulating ROS signaling. J Cell Biol. 2018;217:1915–1928. doi:10.1083/jcb.201708007.
- Zhong W, Qian K, Xiong J, et al. Curcumin alleviates lipopolysaccharide induced sepsis and liver failure by suppression of oxidative stress-related inflammation via PI3K/AKT and NF-κB related signaling. Biomed Pharmacother. 2016;83:302–313. doi:10.1016/j.biopha.2016.06.036.
- Goth L. Catalase deficiency and type 2 diabetes. Diabetes Care. 2008;31:e93. doi:10.2337/dc08-1607.
- Yang S, Koteish A, Lin H, et al. Oval cells compensate for damage and replicative senescence of mature hepatocytes in mice with fatty liver disease. Hepatology. 2004;39:403–411. doi:10.1002/hep.20082.
- Ighodaro OM, Akinloye OA. First line defence antioxidants-superoxide dismutase (SOD), catalase (CAT) and glutathione peroxidase (GPX): their fundamental role in the entire antioxidant defence grid. Alexandria Jo Med. 2018;54:287–293. doi:10.1016/j.ajme.2017.09.001.
- Diopan V, Babula P, Shestivska V, et al. Electrochemical and spectrometric study of antioxidant activity of pomiferin, isopomiferin, osajin and catalposide. J Pharm Biomed Anal. 2008;48:127–133. doi:10.1016/j.jpba.2008.05.025.
- Bozkurt İ, Dilek E, Erol HS, et al. Investigation on the effects of pomiferin from maclura pomifera on indomethacin-induced gastric ulcer: An experimental study in rats. Med Chem Res. 2017;26:2048–2056. doi:10.1007/s00044-017-1913-y.
- Muftuoglu MAT, Aktekin A, Ozdemir NC, et al. Liver injury in sepsis and abdominal compartment syndrome in rats. Surg Today. 2006;36:519–524. doi:10.1007/s00595-006-3196-7.
- Oberholzer C, Oberholzer A, Clare-Salzler M, et al. Apoptosis in sepsis: a new target for therapeutic exploration. Fed Am Soc Exp Biol. 2001;15:879–892. doi:10.1096/fsb2fj00058rev.
- Liew FY, Pitman NI, McInnes IB. Disease-associated functions of IL-33: the new kid in the IL-1 family. Nat Rev Immunol. 2010;10:103–110. doi:10.1038/nri2692.
- Xu H, Turnquist HR, Hoffman R, et al. Role of the IL-33-ST2 axis in sepsis. Mil Med Res. 2017;4:1–9. doi:10.1186/s40779-017-0115-8.
- Kupeli E, Orhan I, Toker G, et al. Anti-inflammatory and antinociceptive potential of maclura pomifera (Rafin.) Schneider fruit extracts and its major isoflavonoids, scandenone and auriculasin. J Ethnopharmacol. 2006;107:169–174. doi:10.1016/j.jep.2006.02.021.
- Vančo J, Trávníček Z, Hošek J, et al. Heteroleptic copper(II) complexes of prenylated flavonoid osajin behave as selective and effective antiproliferative and anti-inflammatory agents. J Inorg Biochem. 2022;226:111639. doi:10.1016/j.jinorgbio.2021.111639.
- Shi Y. Mechanisms of caspase activation and inhibition during apoptosis. Mol Cell. 2002;9:459–470. doi:10.1016/S1097-2765(02)00482-3.
- Garot D, Respaud R, Lanotte P, et al. Population pharmacokinetics of ceftriaxone in critically ill septic patients: A reappraisal. Br J Clin Pharmacol. 2011;72:758–767. doi:10.1111/j.1365-2125.2011.04005.x.