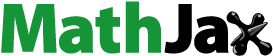
Abstract
Allantoin eutectics with choline chloride (ACM) and zinc chloride (AZM) are reported. The solid–liquid phase diagram showed the eutectic point in allantoin-rich region (Xallantoin = 0.9) for ACM. In AZM, the eutectic formation occurred in the equimolar mixture. The H-bonded template in pure allantoin was disrupted upon addition of choline chloride or zinc chloride, which led to freezing point depression. Classical and non-classical hydrogen bonding, electrostatic and donor–acceptor interactions existed among the components. Infrared vibrational frequencies indicated the involvement of ureido NH2, and ring carbonyl of allantoin, OH group of choline chloride, and Zn2+ in interactions. Thermogravimetric analysis showed that among equimolar mixtures ACM5 was more stable than AZM5. An increase in zinc chloride content markedly altered the thermal stability in AZM. Both categories of eutectics were universally soluble in dimethyl sulfoxide. Skin wounds in rabbit models were healed more effectively by concoctions containing relatively smaller percentages of eutectics.
1. Introduction
Wound healing involves complex cellar and molecular pathways that occur in a highly regulated fashion. Similar processes occur anomalously in cancer [Citation1]. Hence control as well as potency is essential in any wound healing intervention [Citation2]. Allantoin boosts the healing of wound through a multifaceted process [Citation3]. It helps in maintaining optimum hydration, stimulates epithelization and facilitates keratolytic action. In addition, it produces analgesic effects, removes necrotic tissues and enhances cell mitosis [Citation4]. Allantoin has a log P value of ∼ −2.89 (ChemSketch/ACD), which reflects its limited permeation capacity across biological barriers. The pH (wound site) > 7.0, which affects allantoin’s stability [Citation5,Citation6]. The dermal level of zinc could be related to wound healing [Citation7]. Zinc is involved in the activation of enzymes that catalyse cross-linking and act on collagen and elastin during wound mending. Zinc also acts as a cofactor in enzymes involved in wound healing. Topical zinc has been found superior to orally administrated zinc in producing healing effects [Citation8]. Allantoin in combination of metals namely zinc, silver and aluminium has appeared in scientific literature/patents starting 70s. However, none of these studies explored their structural features or prepared eutectics [Citation9]. Only recently, Ag(I) complexes of hydantoins were prepared that also used allantoin as a ligand [Citation10]. A “team chemistry” approach between zinc chloride and antibiotic proflavine has been presented recently [Citation11]. It involves solid-state interactions between the constituents. Choline chloride is one of the widely harnessed molecules in deep eutectic solvents [Citation12]. However, emphasis on investigation of their therapeutic potential has been laid not long ago [Citation13].
Eutectics formation occurs owing to the lower free energy of the liquid mixture than the average of its constituents [Citation14]. Deep eutectic mixtures are next-generation ionic liquids (ILs) that are nearly free of shortcomings associated with the preparation or applications of ILs [Citation15,Citation16]. Deep eutectics can deliver drugs or act as therapeutics in the regulation of release or bioactivity [Citation17]. They can be prepared by using simple procedures and their properties can be adjusted merely by changing the proportion of ingredients [Citation18–21]. The release from eutectic mixtures can be regulated using the competing interactions or the local environment at the wound site. This can help minimizing the side effects without affecting efficacy [Citation22]. The significance of deep eutectic mixtures as therapeutics may be reflected in these words from a recently appeared review [Citation23]
What if a new technology based on therapeutic deep eutectic systems would disrupt the current treatment of major economic and socially burden diseases?
The restricted mobility of constituents in eutectics and competing interactions are expected to regulate release in these systems [Citation22,Citation25–27], similar to other advanced materials [Citation28–30]. These systems may also be devoid of undesirable effects originating from the presence of large quantities of ions or excipients. In addition, no additional controlling process such as trapping in polymeric aggregates will be required in such self-regulated materials [Citation23,Citation31]. The development of similar minimum or no-waste systems will not only improve the effectiveness of treatment, but also economize the healthcare.
2. Material and methods
2.1. Material
Allantoin and choline chloride in purity ≥ 98% were purchased from Sigma-Aldrich. The purity of anhydrous zinc chloride (Alfa Aesar) was also greater than 98%. High-purity solvents meant for chromatography were acquired either from Sigma-Aldrich or Alfa-Aesar. All aqueous preparations used ultrapure water from Milli-Q Advantage A10 System of Millipore (France).
2.2. Preparation of allantoin–choline chloride/zinc chloride binary mixtures (ACM/AZM)
Nine binary mixtures between allantoin and choline chloride were prepared (mole fraction 0.1–0.9). For each preparation, first a fixed quantity of allantoin was mixed with a particular quantity of choline chloride in a solid state in a precleaned and thoroughly dried glass vial and heated on a hot plate till a homogeneous liquid is formed. The mixture was maintained at that temperature and stirred for 30–120 min. The mixtures between allantoin and zinc chloride were prepared in a similar fashion.
2.3. Molar masses, melting points and solubility of mixtures
Gallenkamp digital melting point apparatus was used to determine the melting points of pure components and each corresponding mixture. Thermal analysis is one of the widely harnessed methods of preparing phase diagram. However, microscopic and X-Ray analyses have also been used to gather information on phases and miscibility, and to relate freezing point change with mixture composition [Citation32]. A detailed description of the phase diagram is provided in supplementary Figure S1. The solubility of each mixture was obtained in solvents of different dielectric constants viz. water (80.1), methanol (32.7), ethanol (24.5), acetone (20.7), dimethyl sulfoxide (46.7), and acetic acid (6.15). In each case, a fixed quantity of ACM/AZM was added per millilitre (ml) of solvent gradually with stirring. The solubility was determined at ambient temperature as well as on heating. The molar mass of each mixture was computed from molar masses of pure components (i.e. allantoin = 158.121 g/mol; choline chloride = 139.62 g/mol; zinc chloride = 136.286 g/mol) using Equation (3). These calculations were based on mole fractions of hydrogen bond donor and acceptor obtained using Equations (1) and (2). Allantoin was selected as a hydrogen bond donor, whereas choline chloride and zinc chloride were chosen as hydrogen bond acceptors
(1)
(1)
(2)
(2) where n is the number of moles
(3)
(3)
2.4. Characterization of binary mixtures
FT-IR spectra of selected ACM/AZM were recorded with Varian/Digilab FTS7000 spectrometer using a KBr disc. The thermogravimetric analysis was carried out in a TA Instruments Q600 V20.9 using a nitrogen atmosphere. The heating rate was 20°C/min.
2.5. Wound healing investigations
All wound healing procedures were performed in accordance with international/national guidelines. Ethical approval was obtained from Institutional Review Committee in this regard. Twelve Rabbits each weighing between 1.5 and 2.0 kg were divided into three groups. 1st group (n = 2) was treated with placebo (F). The 2nd group (n = 5) was treated with ACM and 3rd group (n = 5) with AZM Each rabbit was injected with 40 mg ketamine hydrochloride combined with 4 mg of xylazine (IM) per kg of body weight. To create skin wounds, the dorsa of rabbits were shaven, and a 1.0 cm cutaneous wound was created using scalpel. After incision, povidone-iodine (USP) was used to prevent excessive bleeding or infection. The ointment (A–E) containing 5–25% ACM or AZM in polawax was applied to the wound area once in 24 h for 8 days [Citation33–36]. The images were processed by using CellProfiler [Citation37].
3. Results and discussion
3.1. Solid–liquid binary phase diagram
Figure shows the solid–liquid phase diagram for allantoin–choline chloride system. In allantoin–choline chloride mixtures (ACM), the ΔT value observed with X1 = 0.1 is 128°C. Further depression occurs with an increase in the proportion of allantoin up to X1 = X2 = 0.5, after which a 7°C increase in melting point was observed. This was followed by a decline of melting point and the lowest melting point was exhibited by allantoin rich mixture (X1 = 0.9). The temperature difference between the two minima is 4°C. This anomalous behaviour is probably due to microheterogeneity as observed earlier with choline chloride [Citation26]. There are at least three hydrogen bond acceptors in allantoin originating from carbonyl groups and five hydrogen bond donors –NH or –NH2 moieties. The extensive intermolecular hydrogen bonding leads to a complex array where ureidyl groups form a channel [Citation9]. The presence of choline chloride causes the disruption of this template and leads to freezing point depression [Citation38].
Figure 1. Solid–liquid binary phase diagram for allantoin–choline chloride mixtures (left) and allantoin–zinc chloride mixtures (right). The variation in melting point is depicted as a function of mole fraction of allantoin (X1).

Figure 2. Interactions in ACM8 (left) and ACM5 (right) as identified by predictive software. Classical H-bonding, non-classical H-bonding, metal acceptor, electrostatic and metal–metal repulsive interactions exist among components. Allantoin molecules are represented by stick models while choline chloride and zinc chloride are shown as ball and stick model.

In the case of allantoin-zinc chloride mixtures (AZM) shown in Figure , the eutectic point is observed at X1 = 0.5. The eutectic temperature is 168°C. In AZM, the ΔT for X1 = 0.1 is 111°C. It is also interesting to note that the anomalous behaviour observed in the case of ACM is not seen in the solid–liquid phase diagram of AZM, which endorses that the microheterogeneity in the former is due to choline chloride. The metal-containing associates of allantoin have appeared in patents starting 70s, referring to them as complexes but none of them covered their structural features [Citation9]. Only recently, a study on silver complexes of hydantoins was presented that also discussed allantoin. Unlike other hydantoins, the –NH of ureidyl group was engaged in bonding with silver ion [Citation10]. Zn2+ ions may engage the carbonyl of allantoin into donor–acceptor interactions and consequently disrupt the H-bonding network in allantoin responsible for long-range order [Citation9,Citation39]. Similar effect may also be produced by allantoin in zinc chloride [Citation40].
The interactions in ACM8 and AZM5 were further investigated using predictive software Avogadro [Citation41] and BIOVIA Discovery Studio [Citation42]. The results are shown in Figure . This analysis unravelled non-classical H-bonding involving alkyl groups in choline and carbonyl of allantoin in addition to classical H-bonds and electrostatic interactions. The classical H-bonding occurs between –NH2 of ureidyl group of allantoin and oxygen of –OH in choline chloride. For AZM5, additional modes of interactions viz. favourable metal acceptor interactions with carbonyl of allantoin and unfavourable metal–metal repulsion were found. The donor–acceptor interaction between zinc ion and carbonyl of hydantoin group may be considered the main reason for the disruption of H-bonded network and channel formation in allantoin [Citation39].
3.2. Thermal stability
The intermolecular interactions, channel formation by ureidyl moieties in allantoin, and TGA plots of selected mixtures are shown in Figure .
Figure 3. Intermolecular hydrogen bonding (a,b) and ureidyl channel formation (c) in allantoin (left). Reproduced from Ref [Citation9]; and TGA plots of selected binary mixtures ACM5, AZM5 and AZM2 (right).
![Figure 3. Intermolecular hydrogen bonding (a,b) and ureidyl channel formation (c) in allantoin (left). Reproduced from Ref [Citation9]; and TGA plots of selected binary mixtures ACM5, AZM5 and AZM2 (right).](/cms/asset/20f28e73-91a1-4522-a10b-929b56638612/tusc_a_2208727_f0003_oc.jpg)
At 100°C about 4% degradation occurs in ACM5 and merely 2% in AZM5. AZM2 shows high stability and degrades negligibly (∼ 0.2%) up to 357°C. A gradual weight loss is observed in ACM5 and AZM5 up to 231°C after which the former losses weight steeply, while the latter continues to degrade steadily. At 231°C, 1/10th of the total weight is lost in both. At 290°C, around 54% of ACM5 is disintegrated but further slowing down produces the total degradation of 64% at 600°C. AZM5 on the other hand, continually gets defragmented till complete degradation at 599°C. In comparison, a weight of 56% remains in the case of ACM2 at 600°C. This is probably due to the formation of inorganic–organic network [Citation38] that is more stable than zinc chloride [Citation43] and allantoin [Citation44]. In ACM5, the thermal stability is due to the presence of choline chloride which is reduced on eutectic formation [Citation45]. In all the cases, interactions in pure allantoin are severed.
3.3. FT-IR analysis
An in-depth analysis based on IR absorption by mixture was carried out. The FT-IR spectra of selected ACM and AZM are collected in Figure . The corresponding labelled molecule to specify atoms is shown in Figure . The vibrational frequencies are gathered in supplementary Table T1. A comparison between mixtures and pure constituents has been made considering the vibrational mode and interactions involved in at least three distinct wavenumber regions.
Figure 5. Labelling of allantoin for specification of bonds corresponding to different vibrational modes observed in FT-IR spectra.

3.3.1. Region 1: 3550–2700 cm−1
A broad peak at 3537 cm−1 is due to O–H stretching of water. This peak only appears in AZM5 that is allantoin–zinc chloride equimolar mixture. This peak is due to moisture in the sample added from the environment. The peak at 3425 cm−1 is due to anti-symmetric stretching of the solitary NH2 group of allantoin. The νas(OH) in choline chloride appears at 3418 cm−1. The overlapping of the frequency of two vibrational modes broadens the signal, making it arduous to identify them. They appear as shoulders in ACM5 and ACM8 but remain obscure in ACM2. In AZM, choline chloride is replaced by zinc chloride. In AZM2, a shoulder is seen at 3437 cm−1, whereas the corresponding stretching frequencies in AZM5 and AZM8 are 3450 and 3437 cm−1, respectively. These values are blue-shifted and indicate H-hydrogen bonding associations involving ureido –NH2. With the exception of AZM2, νs(NH2) is red-shifted compared to allantoin (i.e. 3340 cm−1) in mixtures. These features point towards the existence of weak hydrogen bonds [Citation46]. The broadening and shifting of NH2 stretching vibrations to lower wave number may also be ascribed to an increase in a number of hydrogen bonds in the system [Citation47].
The O–H stretching in choline chloride appears at 3259 cm−1. ν(N3–H3) has frequency 3125 cm−1 in allantoin [Citation48]. In ACM, bands are observed at 3220, 3194 and 3199 cm−1 for mixtures having X2 = 0.2, 0.5 and 0.8, respectively. The shifting of these values reflects an increase in hydrogen bonding interactions between allantoin and choline chloride [Citation45]. They are also shifted to 3195, 3186 and 3188 cm−1 in the case of AZM2, AZM5 and AZM8. The frequencies in the range of 2964–2722 cm−1 are either due to δas(OH) or C–H stretching of alkyl group in choline chloride or hydantoin moiety i.e. ν(C5–H5) [Citation47,Citation48].
3.3.2. Region 2: 1800–1250 cm−1
The significant peaks in this region include νas[(C1 = O1) + (C2 = O2)], ν (C3 = O3), βscis(NH2), CH3 rocking and C–N stretching (allantoin) that appear at 1740, 1680, 1603, 1478 and 1400 cm−1, respectively in allantoin or choline chloride. Amongst them, the frequencies of C = O in the hydantoin ring are shifted to a lower wave number region, while those of ureido C = O experience blue shift. This indicates that the interactions at ring carbonyl in ACM and AZM are different from those occurring with ureido C = O [Citation45–48]. The magnitudes of these shifts were greater for AZM than ACM. The scissoring frequency of NH2 does not alter much in AZM, but an upfield shift of greater than 15 cm−1 is observed for ACM. This reveals enhanced coupling between C = O and NH2 [Citation49]. ρ(CH3) is only observed in ACM, the wave number representing choline chloride. These values are only slightly changed to 1481, 1474 and 1471 cm−1 in ACM2, ACM5 and ACM8, showing dependence on choline chloride proportion. The C–N stretching frequency of allantoin does not suffer any shift in AZM, but an average shifting of band ∼ 13 cm−1 towards a higher wave number region occurs with choline chloride. The combined in-plane bending vibrations β[H3–N3–C + H5–C5–N3] and stretching ν[ N3–C + N1–C5] is manifested at 1530 cm−1 in pure allantoin [Citation48,Citation50]. These frequencies were blue-shifted (Δν = 1–29 cm−1) in AZM depending upon the mole fraction of zinc chloride. Among ACM, this peak was red-shifted ∼ 3 cm−1 in ACM8. β [H2–N2–C2 + H5–C5–C2] combined with stretching frequency of five-membered ring is centred at 1326 cm−1[Citation48]. This value remains the same in mixtures with X1 = 0.8 and is observed at 1341 and 1349 cm−1 for the rest. β[H4–N4–C3] + β[H5–C5–N3 + H14–N–C] + νas(N–C3) + β[O3 = C3–N] are exhibited at 1284 cm−1 [Citation48]. The vibrational frequency of this band was not changed in ACM5, ACM8 and AZM8. It was however red shifted with magnitudes 28 and 32 cm−1 in AZM5 and AZM2. Being complex absorption, it is not possible to infer based on these shifts.
3.3.3. Region 3: 1200–450 cm−1
Asymmetric deformation of choline and its C–O stretch are manifested at 1137 cm−1 [Citation51]. The allantoin absorptions also occur with wave number 1184 cm−1 due to summation of ν(N3–C5), stretching frequency of hydantoin ring and bending vibrations β(H5–C5–C2). Additionally, ν(N3–C5) appears at 1136 cm−1 [Citation48]. For ACM, a sharp peak is seen at 1181 cm−1 in ACM8 only that has X1 = 0.8. However, AZM2, AZM5 and AZM8 show peaks at 1169, 1153 and 1182 cm−1 due to allantoin. All these peaks are red-shifted and indicate the existence of intramolecular or intermolecular interactions [Citation46,Citation47]. The CH2 rocking of choline moiety (1083 cm−1) is shifted to a lower wave number in ACM and magnitude of Δν increases with an increase in the proportion of choline chloride in the mixture [Citation52]. In the case of allantoin, ν(C–NH2) vibrations produce a low-intensity band in the region 1120–910 cm−1. N–H out-of-plane bending appears between 860 and 510 cm−1 and additionally at 450 cm−1. The C = O vibration is also observed in the range of 780–760 cm−1 [Citation50]. Choline chloride also absorbs in this region with ν(C–O), νas(CCO) and νs(N-CH3) having wave number 1004, 950 and 869 cm−1, respectively [Citation50]. Except for 950 cm−1 absorption, all these peaks are red-shifted in ACM. The ν(C = O) of allantoin is observed in the range of 787–760 cm−1 in the mixtures. The Zn–Cl stretching vibrations originally located at 511 cm−1 in ZnCl2 [Citation43] are shifted to 512, 531 and 532 cm−1 in AZM8, AZM5 and AZM2, respectively, showing the modulation of hydrogen bonding interactions [Citation39].
3.4. Solubility in media with a different dielectric constant
Deep eutectics are capable of solubilizing diverse molecules including drugs [Citation16,Citation53]. The integrity of eutectics when exposed to media of different dielectric is essential for their applications. For instance, in ionothermal reactions deep eutectics undergo self-immolation to deliver the organic component of the template in a controlled way [Citation38]. This breakdown is dependent on competing interactions [Citation22].
Table shows the solubility of ACM and AZM in the solvents of different dielectric constants ranging from 6.13 (acetic acid) to 80.1 (water). Allantoin has very low aqueous solubility, however, it is soluble in ethanol. Choline chloride and zinc chloride have high aqueous solubility. Both ACM and AZM exhibited universal solubility in dimethyl sulfoxide. ACM were partially soluble in water, whereas most AZM were insoluble. Both categories of mixtures showed very little or no solubility in methanol and ethanol. They were partially or sparingly soluble in acetic acid, where protonation of allantoin in acidic medium is expected to have played a role. Recently, a combination of ionic liquids with dimethyl sulfoxide has been investigated for cellulose dissolution, where the role of hydrogen bonding and hydrophobic forces has been discussed [Citation54,Citation55]. The synergy between dimethyl sulfoxide and eutectics is of significance in dermal applications considering the ability of DMSO to enhance permeation through the skin barrier [Citation56].
Table 1. Solubility of ACM and AZM in media of different dielectric constant (S – soluble, IS – insoluble, PS – partially soluble, SS – sparingly soluble).
3.5. Wound healing progression
The progressive wound healing was monitored from day 1 to day 7 after the application of an ointment containing different percentages of ACM5/AZM5. The images taken on alternate days are shown in Figure . The healing occurred in all the cases. However, maximum wound contraction was observed with C, containing 15% of ACM5. Slightly less healing was observed with B. The control-F showed delayed healing with the least contraction occurring over time. On the final day of observation, the maximum contraction was just above 70% in control. It was also found out that increasing the percentage of ACM5 beyond 15% had a detrimental effect on healing. With wound treated with 25% ACM5 (E) less than 80% contraction was seen on day 7. The effect can be explained based on the oxygen requirement at wound site for effective healing [Citation57]. Choline chloride-urea eutectics have been reported to absorb carbon dioxide [Citation58] and similar behaviour may be expected of ACM5. Hence, benefits of using ACM5 are limited to the extent they affect oxygen levels at the wound site [Citation59]. The competing interactions at the wound site that cause the release of allantoin/choline from ACM may effectively operate in a concentration-dependent manner [Citation60].
Figure 6. Progressive wound healing caused by allantoin–choline chloride mixture ACM5 (left) and allantoin–zinc chloride mixture AZM5 (right). The percentage of mixture in ointment was varied from 5% to 25% in A–E. The wound treated with placebo is designated by F.

The progressive wound healing caused by allantoin–zinc chloride mixture AZM5 unravelled accelerated healing with A (5% AZM5), where 88% wound contraction occurred on day 3. Ointment B (10% AZM5) also showed excellent efficacy in the early days. The healing in control (F) and ointments containing higher percentages of AZM5 showed lesser healing. Among them, the best results were obtained for E having 25% AZM5, where above 90% contraction was seen on day 8. Zinc chloride (ZnCl2) is not cytotoxic as predicted by Protox-II. The theoretical studies carried out during the research design stage indicated with high certainty that it had no hepatotoxicity. Also, it had been inactive at other toxicity endpoints such as immunotoxicity and mutagenicity. The LD50 value of ZnCl2 that is 329 mg/kg places it among toxicity class 4 materials. These outcomes endorse the earlier time-dependent investigations of the effect of zinc ions (used in the form of zinc chloride) on human keratinocytes (HaCaT) cells [Citation61]. The results of this study that encompassed cell proliferation, cytotoxicity and gene expression revealed that zinc chloride could be safely employed for wound healing and regeneration up to 10 μg/ml for 72 h of exposure. In fact, zinc ions promoted glycoprotein synthesis, gene expression and wound healing, confirming their significance in biomedicine [Citation61,Citation62]. However, in higher percentages of AZM5, the toxicity may slightly overweight healing benefits as reported previously in zinc-containing hydrogels where cell viability was affected due to toxicity [Citation63].
4. Conclusions
Eutectic phenomena in binary allantoin eutectics with choline chloride (ACM) and zinc chloride (AZM) have been reported for the first time. Two minima were observed in ACM with an overall eutectic point existing in the allantoin-rich region having X1 = 0.9. The use of predictive software revealed the association of four allantoin molecules to a choline moiety through classical and non-classical hydrogen bonding. Both categories of eutectics exhibited universal solubility in dimethyl sulfoxide. In depth, FT-IR spectroscopic analysis revealed the formation of weak H-bonds and the existence of donor–acceptor interactions between carbonyl and cationic centre. ACM5 was more thermally stable than AZM5, however, an increase in the proportion of zinc chloride significantly enhanced degradation resistance in AZM. Wound healing of incisional wounds in oryctolagus cuniculus domesticus models showed that concoctions with small amounts of eutectics were more effective than those possessing relatively greater percentages. This may be related to a reduction in oxygen supply at wound site or toxicity of zinc chloride.
Supplemental Material
Download MS Word (121.5 KB)Acknowledgements
This research received no external funding.
Disclosure statement
No potential conflict of interest was reported by the authors.
References
- MacCarthy-Morrogh L, Martin P. The hallmarks of cancer are also the hallmarks of wound healing. Sci Signal. 2020;13:1–13. doi:10.1126/SCISIGNAL.AAY8690.
- Lu SH, Samandari M, Li C, et al. Multimodal sensing and therapeutic systems for wound healing and management: a review. Sens Actuators Rep. 2022;4:100075. doi:10.1016/j.snr.2022.100075.
- Paller A, Nardi R, Do H, et al. An investigation into multifaceted mechanisms of action of allantoin in wound healing. J Am Acad Dermatol. 2017;76:AB40. doi:10.1016/j.jaad.2017.04.176.
- Araújo LU, Grabe-Guimarães A, Mosqueira VCF, et al. Profile of wound healing process induced by allantoin. Acta Cir Bras. 2010;25:460–466. doi:10.1590/S0102-86502010000500014.
- Maliyar K, Persaud-Jaimangal R, Sibbald RG. Associations among skin surface pH, temperature, and bacterial burden in wounds. Adv Ski Wound Care. 2020;33:180–185. doi:10.1097/01.ASW.0000655488.33274.d0.
- Zhang J. Topical compositions containing solubilized allantoins and related methods. US 0081052 A1. Apr. 3, 2008.
- Olaifa AK, Fadason ST. Studies on zinc and copper ion in relation to wound healing in male and female West African dwarf goats. Niger J Physiol Sci. 2016;31:171–176.
- Lansdown ABG, Mirastschijski U, Stubbs N, et al. Zinc in wound healing: theoretical, experimental, and clinical aspects. Wound Repair Regen. 2007;15:2–16. doi:10.1111/j.1524-475X.2006.00179.x.
- Dall’Olio L. Survey of methodologies of pharmaceutical interest for quantification of crystal form via X-ray powder diffraction, Doctoral dissertation, University of Bolonga, 2021.
- Puszyńska-Tuszkanow M, Grabowski T, Daszkiewicz M, et al. Silver(I) complexes with hydantoins and allantoin: synthesis, crystal and molecular structure, cytotoxicity and pharmacokinetics. J Inorg Biochem. 2011;105:17–22. doi:10.1016/j.jinorgbio.2010.09.013.
- Fiore C, Shemchuk O, Grepioni F, et al. Proflavine and zinc chloride “team chemistry”: combining antibacterial agents via solid-state interaction. Cryst Eng Commun. 2021;23:4494–4499. doi:10.1039/D1CE00612F.
- van der Bruinhorst A, Gomes MC. Is there depth to eutectic solvents? Curr Opin Green Sustain Chem. 2022;37:100659. doi:10.1016/j.cogsc.2022.100659.
- Saha M, Rahman MS, Hossain MN, et al. Molecular and spectroscopic insights of a choline chloride based therapeutic deep eutectic solvent. J Phys Chem A. 2020;124:4690–4699.
- Nordlie BE. Eutectic melting. In: Petrology. Boston, MA: Springer; 1989. p. 155–157 (Encycl. Earth Sci.). 10.1007/0-387-30845-8_65.
- Wang G, Chen S, Qiu N, et al. Virus-mimetic DNA-ejecting polyplexes for efficient intracellular cancer gene delivery. Nano Today. 2021;39:101215. doi:10.1016/j.nantod.2021.101215.
- Yu D, Xue Z, Mu T. Eutectics: formation, properties, and applications. Chem Soc Rev. 2021;50:8596–8638. doi:10.1039/d1cs00404b.
- Huang C, Chen X, Wei C, et al. Deep eutectic solvents as active pharmaceutical ingredient delivery systems in the treatment of metabolic related diseases. Front Pharmacol. 2021;12:794939. doi:10.3389/fphar.2021.794939.
- Santos F, Duarte ARC. Therapeutic deep eutectic systems for the enhancement of drug bioavailability. In: Lichtfouse E, Fourmentin S, Costa Gomes M, editors. Deep Eutectic Solvents for Medicine, Gas Solubilization and Extraction of Natural Substances. Cham: Springer; 2021. p. 103–129 (Environ. Chem. a Sustain. World). doi:10.1007/978-3-030-53069-3_3.
- Qader IB, Prasad K. Recent developments on ionic liquids and deep eutectic solvents for drug delivery applications. Pharm Res. 2022;39:2367–2377. doi:10.1007/s11095-022-03315-w.
- Pedro SN, Freire MG, Freire CSR, et al. Deep eutectic solvents comprising active pharmaceutical ingredients in the development of drug delivery systems. Expert Opin Drug Deliv. 2019;16:497–506. doi:10.1080/17425247.2019.1604680.
- Marques Da Silva JM, Velhinho Pereira C, Mano MF, et al. Eutectic menthol-fatty acid combinations for wound healing. WO2020174340A1, Sep. 3, 2020.
- Shah SWH, Bibi I, Yousaf S. Cetrimonium surfactants with biologically active counter ions as self-immolative drug delivery systems. Sci Lett. 2022;16:31–45. doi:10.24191/sl.v16i1.14547.
- Santos F, Duarte ARC. Therapeutic deep eutectic systems towards the treatment of tuberculosis and colorectal cancer: opportunities. Molecules. 2021;26:7022. doi:10.3390/molecules26227022.
- Grada A, Mervis J, Falanga V. Research techniques made simple: animal models of wound healing. J Invest Dermatol. 2018;138:2095–2105.e1. doi:10.1016/j.jid.2018.08.005.
- Srinivasan H, Sharma VK, Sakai VG, et al. Transport mechanism of acetamide in deep eutectic solvents. J Phys Chem B. 2020;124:1509–1520. doi:10.1021/acs.jpcb.9b11137.
- Spittle S, Poe D, Doherty B, et al. Evolution of microscopic heterogeneity and dynamics in choline chloride-based deep eutectic solvents. Nat Commun. 2022;13:219. doi:10.1038/s41467-021-27842-z.
- Kim J, Gao Y, Zhao Z, et al. A deep eutectic-based, self-emulsifying subcutaneous depot system for apomorphine therapy in Parkinson ‘ s disease. Proc Natl Acad Sci U.S.A. 2022;119:e2110450119. doi:10.1073/pnas.2110450119.
- Liu G, Zhou Y, Xu Z, et al. Janus hydrogel with dual antibacterial and angiogenesis functions for enhanced diabetic wound healing. Chin Chem Lett. 2023;34:107705. doi:10.1016/j.cclet.2022.07.048.
- Xian C, Zhang Z, You X, et al. Nanosized fat emulsion injection modulating local microenvironment promotes angiogenesis in chronic wound healing. Adv Funct Mater. 2022;32; doi:10.1002/adfm.202202410.
- Zhou Y, Liu G, Huang H, et al. Advances and impact of arginine-based materials in wound healing. J Mater Chem B. 2021;9:6738–6750. doi:10.1039/d1tb00958c.
- Qu W, Qader IB, Abbott AP. Controlled release of pharmaceutical agents using eutectic modified gelatin. Drug Deliv Transl Res. 2022;12:1187–1194. doi:10.1007/s13346-021-00998-3.
- Yamashita H, Hirakura Y, Yuda M, et al. Detection of cocrystal formation based on binary phase diagrams using thermal analysis. Pharm Res. 2013;30:70–80. doi:10.1007/s11095-012-0850-1.
- Rajab A, Al-Wattar W, Taqa GA. The roles of apigenin cream on wound healing in rabbits model. J Appl Vet Sci. 2022;7:1–5. doi:10.21608/javs.2021.97151.1104.
- Ravishankar K, Kiranmayi GVN, Prasad YR, et al. Wound healing activity in rabbits and antimicrobial activity of hibiscus hirtus ethanolic extract. Braz J Pharm. Sci. 2018;54:e17075. doi:10.1590/s2175-97902018000417075.
- Ersel M, Uyanikgil Y, Akarca FK, et al. Effects of silk sericin on incision wound healing in a dorsal skin flap wound healing rat model. Med Sci Monit. 2016;22:1064–1078. doi:10.12659/MSM.897981.
- Gelsi PRF. Topical use of canine extracellular matrix components derived from the dermis for wound healing [master’s thesis]. Sao Paulo (BR): University of Sao Paulo, 2022. doi:10.11606/D.10.2022.tde-30052022-105007.
- Sanka I, Bartkova S, Pata P, et al. Investigation of different free image analysis software for high-throughput droplet detection. ACS Omega. 2021;6:22625–22634. doi:10.1021/acsomega.1c02664.
- Parnham ER, Drylie EA, Wheatley PS, et al. Ionothermal materials synthesis using unstable deep-eutectic solvents as template-delivery agents. Angew Chemie – Int Ed. 2006;45:4962–4966. doi:10.1002/anie.200600290.
- Zhang Q, Ma Y, Lu Y, et al. Modulating electrolyte structure for ultralow temperature aqueous zinc batteries. Nat Commun. 2020;11:4463. doi:10.1038/s41467-020-18284-0.
- Chen L, Liao WQ, Ai Y, et al. Precise molecular design toward organic–inorganic zinc chloride ABX3 ferroelectrics. J Am Chem Soc. 2020;142:6236–6243. doi:10.1021/jacs.0c00315.
- Hanwell MD, Curtis DE, Lonie DC, et al. Avogadro: an advanced semantic chemical editor, visualization, and analysis platform. J Cheminform. 2012;4:17. doi:10.1186/1758-2946-4-17.
- Dassault Systemes B. Discovery Studio Visualizer, v16.1.0.15350, San Diego: Dassault Systemes, 2015.
- Trivedi M, Sethi K, Panda P, et al. A comprehensive physicochemical, thermal, and spectroscopic characterization of zinc (II) chloride using X-ray diffraction, particle size distribution, differential scanning calorimetry, thermogravimetric analysis/differential thermogravimetric analysis. Int J Pharm Investig. 2017;7:33–40. doi:10.4103/jphi.JPHI_2_17.
- Svetlichny G, Külkamp-Guerreiro IC, Dalla Lana DF, et al. Assessing the performance of copaiba oil and allantoin nanoparticles on multidrug-resistant Candida parapsilosis. J Drug Deliv Sci Technol. 2017;40:59–65. doi:10.1016/j.jddst.2017.05.020.
- Delgado-Mellado N, Larriba M, Navarro P, et al. Thermal stability of choline chloride deep eutectic solvents by TGA/FTIR-ATR analysis. J Mol Liq. 2018;260:37–43. doi:10.1016/j.molliq.2018.03.076.
- Joseph J, Jemmis ED. Red-, blue-, or no-shift in hydrogen bonds: a unified explanation. J Am Chem Soc. 2007;129:4620–4632. doi:10.1021/ja067545z.
- Du C, Zhao B, Chen XB, et al. Effect of water presence on choline chloride-2urea ionic liquid and coating platings from the hydrated ionic liquid. Sci Rep. 2016;6:29225. doi:10.1038/srep29225.
- Alam MJ, Ahmad S, FT-Raman FTIR. UV-Visible spectra and quantum chemical calculations of allantoin molecule and its hydrogen bonded dimers. Spectrochim Acta – Part A Mol Biomol Spectrosc. 2015;136:961–978. doi:10.1016/j.saa.2014.09.119.
- Araujo CF, Coutinho JAP, Nolasco MM, et al. Inelastic neutron scattering study of reline: shedding light on the hydrogen bonding network of deep eutectic solvents. Phys Chem Chem Phys. 2017;19:17998–18009. doi:10.1039/c7cp01286a.
- Dinica RM, Sandu C, Veronica A, et al. Allantoin from valuable Romanian animal and plant sources with promising anti-inflammatory activity as a nutricosmetic ingredient. Sustainability. 2021;13:10170. doi:10.3390/su131810170.
- Gautam R, Kumar N, Lynam JG. Theoretical and experimental study of choline chloride-carboxylic acid deep eutectic solvents and their hydrogen bonds. J Mol Struct. 2020;1222:128849. doi:10.1016/j.molstruc.2020.128849.
- Li Y, Li Y, Li H, et al. Insights into the tribological behavior of choline chloride—urea and choline chloride—thiourea deep eutectic solvents. Friction. 2023;11:76–92. doi:10.1007/s40544-021-0575-4.
- Hoang Pham UG. Pharmaceutical applications of eutectic mixtures. J Dev Drugs. 2013;02:3–4. doi:10.4172/2329-6631.1000e130.
- El Seoud OA, Bioni TA, Dignani MT. Understanding cellulose dissolution in ionic liquid-dimethyl sulfoxide binary mixtures: quantification of the relative importance of hydrogen bonding and hydrophobic interactions. J Mol Liq. 2021;322:114848. doi:10.1016/j.molliq.2020.114848.
- Paiva TG, Zanatta M, Cabrita EJ, et al. DMSO/IL solvent systems for cellulose dissolution: binary or ternary mixtures? J Mol Liq. 2022;345:117810. doi:10.1016/j.molliq.2021.117810.
- Otterbach A, Lamprecht A. Enhanced skin permeation of estradiol by dimethyl sulfoxide containing transdermal patches. Pharmaceutics. 2021;13:320. doi:10.3390/pharmaceutics13030320..
- Sen CK. Wound healing essentials: Let there be oxygen. Wound Repair Regen. 2009;17:1–18. doi:10.1111/j.1524-475X.2008.00436.x.
- Liu F, Chen W, Mi J, et al. Thermodynamic and molecular insights into the absorption of H2S, CO2 and CH4 in choline chloride plus urea mixtures. AIChE J. 2019;65:e16574. doi:10.1002/aic.16574.
- Wang Y, Zhang Y, Su J, et al. Preparation of a multifunctional wound dressing based on a natural deep eutectic solvent. ACS Sustain Chem Eng. 2020;8:14243–14252. doi:10.1021/acssuschemeng.0c05754.
- Iorio V, Troughton LD, Hamill KJ. Laminins: roles and utility in wound repair. Adv Wound Care. 2015;4:250–263. doi:10.1089/wound.2014.0533.
- Salesa B, Serra RSI, Serrano-Aroca Á. Zinc chloride: time-dependent cytotoxicity, proliferation and promotion of glycoprotein synthesis and antioxidant gene expression in human keratinocytes. Biology (Basel). 2021;10:1072. doi:10.3390/biology10111072.
- Liu Z, Tang W, Liu J, et al. A novel sprayable thermosensitive hydrogel coupled with zinc modified metformin promotes the healing of skin wound. Bioact Mater. 2023;20:610–626. doi:10.1016/j.bioactmat.2022.06.008.
- Mohandas A, Sudheesh KPT, Raja B, et al. Exploration of alginate hydrogel / nano zinc oxide composite bandages for infected wounds. Int J Nanomedicine. 2020;10:53–66. doi:10.2147/IJN.S79981.