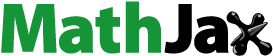
Abstract
In the present study, Microwave-assisted extraction (MAE) and Soxhlet-extraction (SE) were compared for the extraction of phytochemicals from the seed powder of Daucus carota. Results showed that only 5 min of MAE gave maximum phenolics 275 mg and flavonoids 229 mg as compared to SE, which took 5 h to give its maximum. GC-MS analysis of MAE and SE revealed 15 and 11 compounds respectively; Estragole (52.55%) and linalool (39.97%) being the major component in MAE and SE-derived extract respectively. HPLC analysis showed a variety of phenolics and flavonoids in MAE as compared to SE. MAE extracts showed significantly higher antioxidant (82%) and antifungal potential (89.3%inhibition) compared to SE (54.32% and 60.7% respectively). Results suggested that MAE can rapidly extract phytochemicals from carrot seeds without affecting their biological activities. The usage of MAE in analytical labs can safely opt for rapid extraction of required phytochemicals by optimizing the parameters.
1. Introduction
Fruits and vegetables have a large variety of bioactive compounds (phytochemicals) like phenolics and flavonoids that are known for their nutritional value and their positive effects on human health. Such substances help to avoid various ailments including cardiovascular disorders, cancer, inflammatory issues, and tumours [Citation1] because they possess strong antibacterial, antifungal, and anti-oxidative characteristics. Daucus carota L. (carrot) is one such vegetables also known as a root vegetable of the Apiaceae family that is widely cultivated all over the world for its nutritional value. Due to their high nutritional value and longer shelf life, carrot plays a significant role in the human diet [Citation2]. It has been ranked 10 out of 39 fruits and vegetables because of the variety in its nutritional value [Citation3].
Seeds of D. carota also possess several phytoconstituents like phenolics, flavonoids, carotenoids, and a series of volatile compounds. Seeds are also reported to have antioxidant, anti-diabetic, anti-carcinogenic, anti-hypertensive, antifungal, antibacterial, and anti-inflammatory characteristics but carrot seeds have not gained such commercial acceptability as they should [Citation4]. Carrot seeds have been neglected in the past due to several reasons. One of the main reasons is that carrots are mostly consumed as a vegetable, and the demand for carrot seeds has been relatively lower compared to other crops such as corn, wheat, and soybeans. As a result, seed companies have not invested much in developing improved varieties of carrot seeds or in promoting their commercial production. Moreover, carrot seeds are relatively small, and their production is labour-intensive, which makes them expensive to produce. All of these factors have contributed to the low commercial acceptability of carrot seeds in the past. Regarding the production of carrots and seeds at the same time, it is possible, but it requires careful management. Carrots require a specific growing environment, and any disturbance to their growing conditions can affect their yield and quality. Therefore, if seeds are to be produced at the same time as carrots, the production practices need to be adjusted to meet the specific requirements of both products. The average productivity per hectare of carrot seeds and carrots can vary depending on several factors such as the climate, soil type, irrigation, fertilization, and the variety of the crops. On average, carrot seed production can yield between 400 and 1000 kilograms per hectare, while carrot production can yield between 30 and 60 tons per hectare. However, these figures are highly variable and can be significantly influenced by the growing conditions and the specific variety being grown. There is a need to commercially exploit these seeds to maximize the benefits from their antioxidants with the least cost and time [Citation5,Citation6].
Extraction and separation of natural bioactive compounds using selected efficient techniques must urgently be established. There is a wide gap between plant-based antioxidant research and its commercial application. Even though these seeds have the potential to produce antioxidants commercially but they are neglected due to this gap. The most commonly used method for extraction is solvent extraction [Citation7]. Soxhlet extraction requires a large amount of solvent and prolonged extraction periods. Prolonged heating can also cause the degradation of heat-sensitive compounds, thus affecting their biological activities [Citation8].
Research on the traditional extraction methods for phytoconstituents from D. carota has shown that varying the extraction methods and their parameters have a potential effect on the amount of extracted carotenoids and their antioxidant potential [Citation9]. Because of the equipment availability, easy sample handling, and maximum extraction performance, Microwave assisted extraction (MAE) has been considered one of the most practical techniques for the industrial scale. Microwave vitality in MAE is used in increasing the efficiency of extracting solvents for extracting bioactive compounds from plants [Citation10]. For the enhanced extraction of polyphenols, optimization of the extraction process is required. The quality and quantity of these bioactive components when derived from plants are greatly influenced by microwave irradiation time and power level. Moreover, at high power levels of microwave and larger time for irradiation, degradation of the compounds can be resulted [Citation9].
If a combination of suitable extraction parameters of MAE is selected according to the nature of plant material and its phytoconstituents, then a rapid and cost-effective extraction protocol can be defined for them. Various statistical tools like Response Surface Methodology (RSM) can help researchers to do this smartly. Thus, the main aims of this research work are: (1) to determine MAE's ability to enhance the extraction of polyphenols (phenolics and flavonoids) from carrot seeds, (2) to optimize polyphenol extraction from seeds based on time of extraction, particle size and power level of MAE, (3) to analyze antimicrobial activity for estimating the stability of phytochemicals in extracts, as well as their quality compared with conventional Soxhlet extraction method.
2. Materials and method
2.1. Collection and identification of plant material
The plant material i.e. seeds of D. carota were collected from National Agriculture Research Center (NARC), Islamabad, Pakistan. The seeds were powdered, sieved to get particle sizes 40, 80, and 120 µm, and stored in air-tight jars for further analysis.
2.2. Experimental design (response surface methodology)
Design expert software (Version 11, State-Ease, USA) was used to apply RSM. A quadratic model was used. Power level (300W to 900W) “A”, time (1–5 min) “B” and particle size (40 µ to 120 µ) “C” were analyzed against two dependent parameters i.e. TPC (Total Phenolic Content) and TFC (Total Flavonoid Content). These independent microwave variables have been chosen because of the compelling effect of the MAE on these phytochemicals. A total of 19 experimental runs were conducted randomly, including 3 replicates and all the procedures were repeated three times to enhance the analysis. Diagnostics of the design were done and the Box–Cox plot for power transforms was studied.
To describe the relationship between two variables (dependent and independent) a quadratic polynomial regression model was proposed, with the expected response calculated from the relation.
The dependent variable’s predicted value is Y, which is the concentration of flavonoids and phenolics, γ1, γ2, and γ3 are linear coefficients, γ12, γ23, and γ13 are interactive coefficients, and γ11, γ22, and γ33 are squared coefficients. To validate the model’s fitness, it was statistically evaluated and an Analysis of Variance was used.
2.3. Microwave assisted extraction
Microwave Assisted Extractor (MDS-6G, Sineo, China) was used for the extraction of carrot seeds. The inner vessel was inserted into the external protective vessel and 10 mL of solvent (ethanol 99%, Sigma Aldrich, USA) was added to the inner vessel. The vessel was put in the main framework. In 10 mL solvent (ethanol), 1 g of the plant material was mixed and added to the vessel. Pressure, temperature, power, and time were set and then the microwave extractor was run according to conditions set by using the statistical design described in the above section. The obtained extracts from the plant material were filtered into the labelled vials and then dried. Dried extracts were weighed, compared for their weights, and then stored in the refrigerator at 4°C for further analysis.
2.4. Soxhlet extraction
Powdered carrot seeds were extracted by using the Soxhlet apparatus. Each particle size sample from seed powder (20 g) was taken in powdered form and placed within the thimble which is made of filter paper, then put vertically into the Soxhlet extractor main chamber for maximal solvent content. The extraction time was adjusted for maximal plant material extraction. It was extracted in 250 mL of ethanol for 2, 8, 16, and 24 h. At room temperature, the obtained extract was evaporated. The dry extract was kept in the refrigerator at 4°C.
2.5. Total phenolic content (TPC)
TPC was taken as response 1 of statistical analysis and measured by using a spectrophotometric assay [Citation11]. 1 mg/mL of plant extract was taken and then its 125 µL was mixed with 125 µL of Folin–Ciocalteu (FC) reagent, followed by the addition of 500 µL of distilled water. After that 7%, Na2CO3 (125 µL) was mixed into the solution and the final volume was increased to 3 mL by adding distilled H2O. The reaction mixture was held for 1 h and 30 min in the dark, after that, the absorbance of the reaction mixture was checked at 760 nm. TPC was recorded as the mg equivalent of gallic acid in comparison to the standard curve.
2.6. Total flavonoid content (TFC)
TFC was taken as a second marker to test the effect of various parameters of extraction methods and was measured by using a spectrophotometric assay [Citation12]. For this purpose, 250 µL of plant extract (1 mg/mL) was mixed with 1.25 mL of distilled water, and 75 µL of 5% sodium nitrite solution was added to this mixture. After 5 min 150 µL of aluminum chloride solution with 10% concentration was added. After 6 min 275 µL of distilled H2O and 500 µL of 1 Molar sodium hydroxide were mixed in the solution. The mixture was shaken well and then the absorption at 510 nm was measured. Total flavonoid content was quantified using the standard catechin curve and measured as mg of catechin equivalent per gram of dry weight (DW) of carrot seeds.
2.7. Biological activities of selected extracts
MAE and Soxhlet extract with maximum TPC and TFC optimized by RSM were selected for biological components and activities (antioxidant and reducing potential, non-polar and polar chemical profile, antifungal assay, etc.) and were also compared with each other.
2.8. High-performance liquid chromatography
Samples with the maximum amount of phenolics and flavonoids were analyzed by HPLC. HPLC (Agilent, USA) equipped with a quaternary pump and UV-Vis. detector was used. Pure standards of quercetin, catechin, ferulic acid, gallic acid, and caffeic acid (Sigma-Aldrich, USA) were used to make calibration curves. 50 mg of extracted plant material each time was vortexed for 1 h before being combined in a 2:1 ratio with ethanol and chloroform. The samples were centrifuged for the removal of solid particles. Each sample was injected directly. With the ratio of 500:500:4, of water, ethanol, and acetic acid respectively, mobile phase A was prepared. For mobile phase B, acetone, methanol, tetrahydrofuran, and acetic acid were mixed in a ratio of 500:375:125:4 correspondingly. The flow rate was 0.65 mL/min, and the injection volume was 10 µL at 10°C. Column temperature and nebulizer heater were set at 50°C and 30°C respectively. The absorption wavelength was selected at 210 nm. The chemicals were detected by using a UV-Visible Spectrophotometer detector.
2.9. Gas chromatography-mass spectrometry (GC-MS)
The qualitative and quantitative characterization of the major and minor nonpolar components of the selected extracts, as well as total monoterpenes was done by GC-MS. The series of standards and alkanes were injected first for GC-MS. Injector and detector temperatures were set at 250°C and 320°C, correspondingly. The analysis was performed on a DB-5 capillary column. The column oven temperature was regulated between 70 and 315°C, with a 5°C/min rise. The initial temperature was held for three minutes, and the final temperature was held for ten minutes. The carrier gas was helium, with a flow rate of 1 mL/min. Using split mode, 1 microliter of the sample was injected. The scan range was 35–500 amu, with the injector temperature of 250°C and the MS transfer line at 290°C. For MS analysis, 70 eV of ionization energy was utilized. Retention times and mass spectra were utilized for chemical identification and characterization of bioactive components, with NIST-20 library, 2020 confirmation. The percentage of the total volatile oils examined was used to calculate the percentage of certain monoterpenes [Citation13].
2.10. Antioxidant potential and reducing power of MAE-based extracts
For antioxidant activity 1 mL of extract was mixed with 1 mL of 1 mM DPPH (2,2-diphenyl-1-picryl-hydrazyl-hydrate) and incubated for 30 min at 37°C. Absorbance at 517 nm was checked for the reaction mixtures. The % of DPPH inhibition was calculated by using the equation
Ac is the absorption of DPPH and water, and As is the absorption of DPPH and sample. Whereas standard antioxidants used were ascorbic acid, α-Tochopherol, and BHT (butylated hydroxytoluene).
2.11. Antifungal activity
The culture medium used in the experiment consisted of malt extract. It was prepared by mixing 2 g of malt extract in 100 mL of distilled water. It was then autoclaved at 121°C for 30 min in a 250 mL flask. A 0.5% w/v concentration of ethanolic extract from carrot seeds was made by adding 0.5 mL of stock solution in 95.5 mL of the ME medium. Other concentrations of 1% and 1.5% were prepared by adding 1 and 1.5 mL of the stock solution in 99 and 98.5 mL of ME. Control treatments were without any plant extract. Each concentration was endowed with an Amoxil capsule @125 mg 100 mL−1 of medium to avoid bacterial contamination. All the concentrations were replicated three times. Mycelial discs (5 mm in diameter) were prepared using sterilized cork borer from the tip of 7 day old culture of Aspergillus niger, Rhizopus stolonifera, and Penicillium chrysogenum and were placed separately in the centre of each flask. After 7-days, fungal growth was measured by filtering the solution of each concentration through pre-weighted Whatmann no. 1 filter paper. The test fungal biomass was measured by using the formula:
2.12. Statistical analysis
Data were statistically examined using (ANOVA) multi-factorial analysis of variance. A statistical analysis of response surface plots was carried out using Design-Expert software (version 11.0, Stat-Ease, USA).
3. Results and discussion
3.1. Fitting the model and optimizing MAE parameters
To analyze the impact and correlations of MAE variables (time of extraction, particle size, and power level) on TPC and TFC, Central Composite Design (CCD) was used. Table shows the testing values obtained varied from 50 to 275 mg of TPC and 69–229 mg for TFC.
Table 1. A central composite design with dependent variables from MAE of Carrot seeds.
These values showed significant dependency on extracted phytochemicals on the conditions of extraction, which shows the need to improve the process of extraction.
Quadratic models were established, and ANOVA was used to evaluate the efficacy and fitness of the models (Table ). In the present research, extraction of phytochemicals from carrots by using Soxhlet extraction and MAE was done. Earlier Abdul Rasheed et al. [Citation14] and Fikselova et al. [Citation15] extracted phytochemicals from carrot seeds by using Soxhlet extraction. MAE of carrot seeds was done by other researchers and it was found that MAE is a much more efficient method as compared to others [Citation16].
Table 2. ANOVA for Quadratic model for TPC and TFC.
3.2. Effect of the extraction parameters on TPC and TFC
The total Phenolic Content (TPC) in carrot seed extract varied from 50 to 275 GAE/g as shown in Table . The minimum yield was obtained at a microwave power of 900W after 3 min of extraction and 120 µ of particle size, while the maximum yield was obtained at the power level of 600W after the irradiation of 5 min with a particle size of 40 µ. Table demonstrates that the power level of the microwave (X1) and time for extraction (X2) has a significantly positive impact on TPC and TFC (P < 0.05). The interaction between power level with particle size (X1X3) and with time for extraction (X1X2) was also significant. The equations for TPC and TFC were as follows:
The power level of the microwave and time for extraction had a highly significant (p < 0.001) positive linear effect, as seen in Table . The extraction yield will decrease when the MAE conditions exceed the optimal points (Figure d–f). The value of TFC eventually increased over time by increasing the extraction powers from lower power levels to higher power levels (Figure a–c). Another significant parameter that affected the extraction of TPC was the time of extraction. As in the present study Figure (a) show that with increasing extraction time, TPC also increased up to 4 min of extraction, after that by further increasing time, lesser TPC was found. In the case of MAE, the effect of the power level of the microwave and time of extraction on TPC is shown in Figure (c). As the power level and extraction time increase from 1 to 5 min, TFC also increases significantly. The minimum yield of TPC was obtained at a microwave power of 900W after 3 min of extraction and 120 µ of particle size, while the maximum yield of TPC was obtained at the power level of 600W after the irradiation of 5 min with a particle size of 40 µ. Similar findings were recorded for polyphenols from mushrooms by Maeng et al. [Citation17], from pistachio hull by Özbek et al. [Citation18], from Calop pulp by Saci et al. [Citation19], from castor oil by Ghaffar et al. [Citation20] and from cherry pomace by Kashyap et al. [Citation21]. The significant quadratic effect of power level on the extraction of TPC (Table ) may be explained by the higher degree of breakage of the sample cell membrane and the enhanced solubility of polyphenols by the eventual increase in the power level of the microwave as described by Li et al. [Citation22]. In MAE, the perfect combination of power and time may improve the separation and isolation of active compounds. Higher power levels promote abrupt cell rupture which might be helpful to obtain a particular molecule discussed by Akhtar et al. [Citation23]. The Larger surface area improves the interaction between the surface of the plant and microwaves, increasing the probability of microwave absorption through cells and generating extra plant secretions to leave the cell mentioned by Mohammad et al. [Citation24]. The experimental evaluation of MAE conditions led us to conclude that the specific parameters of 600 W power, 3 min extraction time, and 80 µ particle size yielded the best extraction efficiency. These findings provide a solid foundation for future research and applications in plant extractions involving microwave-assisted extraction techniques.
3.3. Microwave assisted extraction (MAE) and soxhlet extraction (SE)
In the present study, the MAE approach proved to be significantly faster than the SE method and demonstrated higher extraction efficiency for carrot seeds, and yielded better results. Figure shows that after 3 min of MAE at 600 W and 80 µm particle size, the maximum amount of extract was reported as 283.89 mg/g of plant material. Whereas, SE could produce only 155.55 mg per g of extract using the same plant material to solvent ratio but 300 min as compared to 3 min of extraction of MAE. Through RSM, various combinations of power levels, extraction times, and particle sizes were evaluated. Results showed that the MAE conditions of 600 W power, 3 min extraction time, and 80 µm particle size resulted in higher extraction yields and better overall performance that’s why these conditions for MAE were chosen. Earlier researchers have reported carotenes, anthocyanin, vitamins, and phenolics such as caffiec acid, protocatechenic acid, ferulic acid, quercetin, chlorogenic acid, gallic acids, and kaempferol from carrot roots. These compounds have been extracted by SE and reported on the basis of HPLC analysis [Citation16]. But there is not any report of HPLC and phenolic analysis of carrot seeds. Studies are conducted on the essential oils of carrot seeds but not the phenolics. Kumar et al. [Citation25] reported higher phenolic content from the MAE of carrot pomace. Whereas Hiranvarachat and Devahastin [Citation26], also reported enhanced production of carotenoids from MAE of carrot peels.
3.4. HPLC analysis
Results obtained by the HPLC analysis of MAE extract showed the presence of quercitin (19.5%), rutin (15%), myricetin (11%), kaempferol (10.36%), gallic acid (10.02%), chlorogenic acid (8.67%), caffeic acid (6.3%), synapic acid (5.9%) and benzoic acid (0.9%) in the carrot seeds extract as shown in Figure (a,b) and Table .
Figure 3. HPLC chromatogram of MAE-derived extract for (a) phenolics, (b) flavonoids, and HPLC chromatogram of SE-derived extract for (c) phenolics and (d) flavonoids.

Table 3. Compounds obtained by HPLC analysis of extracts obtained by MAE and SE of carrot seeds.
Results of HPLC of SE showed the presence of only 5 compounds with relatively lesser percentages as compared to MAE. These compounds included quercitin (8.6%), caffeic acid (3.59%), gallic acid (3.22%), kaempferol (2.12%), and synapic acid (1.3%) as shown in Figure (c,d). MAE extract showed the diversity of new compounds by HPLC as compared to SE. The combination of rapid and uniform heating, higher temperature and pressure, and shorter extraction time in MAE can result in a more efficient and effective extraction of the target compounds from the sample, leading to a higher number of compounds detected in the extract obtained by this method [Citation27]. Liazid et al. [Citation28] reported the recovery of 22 phenolic compounds with MAE ranging in temperature from 50 to 175°C. Casazza et al. [Citation29] also reported higher stability of phenolics in MAE extracts when ethanol is used as extracting solvent.
3.5. GC–MS analysis
The major chemical constituents of MAE and Soxhlet extract of carrot seeds are given in Table . The major nonpolar chemical constituents obtained from MAE extract were estragole (52.55%), verbenone (9.79%), carotol (24.88%), and p-cymene-8-ol (9.56%). Whereas nonpolar components of Soxhlet extract of D. carota seed powder were p-xylene (1.36%), alpha-pinene (11.6%), linalool (39.79%), verbenone (7.37%), p-cymene-8-ol (10.9%), and docosanediol (9.5%) as shown in Figure . GC-MS analysis of MAE extract identified 15 compounds that represent the composition of nonpolar bioactive constituents present in the extract. The major compounds are shown in Table . In variable amounts, the major chemical compounds were alkanes and terpenes. Few additional compounds were found in trace amounts. Soxhlet extract of carrot seeds exhibited the presence of only 11 main compounds and linalool (39.97%) was dominant of all. The major chemical compounds of MAE and Soxhlet extract of carrot seeds are given in Table . Kaur et al. [Citation30] and Angelini et al. [Citation31] reported carotol and alpha-pinene as the main constituent of carrot seed essential oil obtained by hydrodistillation respectively. There are various concentrations of nonpolar components in MAE and SE-derived extracts and particularly in GC-MS of SE, the unidentified are in the retention time range of 18–23 min. These points and the presence of trace amounts of some compounds confirm the degradation of non-polar components during SE as compared to MAE. Bampouli et al. [Citation32] used four extraction methods, ultrasound-assisted extraction, supercritical extraction, MAE, and SE; tested them for their ability to extract metabolites from Pistacia lentiscus and reported similar results with a higher extraction efficiency of MAE as compared to SE.
Table 4. Compounds obtained by GCMS analysis of extracts obtained by MAE and SE of carrot seeds.
3.6. Antioxidant activity
The degree of DPPH radical reduction is measured by a decrease in the absorption peak of mixtures containing extracts or other anti-oxidants. MAE and SE extracts from carrot seeds with maximum phenolic compounds were used to detect the antioxidant activity of the extracts. The results of the DPPH analysis of carrot seeds were shown in Figure . The highest antioxidant activity (82 percent) was generated by MAE (run #3), which is also compared to standard antioxidants (Ascorbic acid). Soxhlet extract (5hrs) from D. carota seeds generated about 54.32% of antioxidant activity which was comparatively lower than MAE. Gallic acid, caffeic acid, kaempferol, and quercitin are some of the phytochemicals known for their higher antioxidant activity. Therefore, the higher content of these compounds in the MAE extract of carrot seeds in the present study can be correlated with higher antioxidant activity. Earlier Ksouri et al. [Citation33] reported that the ethanolic extract of carrot seed produced the highest radical-scavenging activity. Fernández-Marín et al. [Citation34] also reported that Curcuma longa L exhibited higher antioxidant activity with the MAE technique at an optimum point as compared to the SE. Torres et al. [Citation35] reported the same values of antioxidant potential of MAE and SE using the leaves of Perestia aculeate and claimed higher efficiency of MAE as it required lesser time to extract the same amount of antioxidants. Higher recovery of antioxidant compounds by MAE may also be attributed to the fact that moisture in plant cells is converted into heat which causes the rapid release of phytochemicals from the cells. This leads to higher extraction of antioxidants without damaging their structures.
3.7. Antifungal activity
In the present study, the antifungal potential of carrot seed extracts (MAE and SE) against three fungal strains i.e. Aspergillus niger, Rhizopus stolonifera, and Penicillium chrysogenum is reported. Figure (a–c) shows the antifungal activity and their dry mass against three tested fungi for MAE while Figure (d–e) is showing the comparison of the antifungal potential of carrot seed extracts obtained by MAE and SE.
Figure 6. percent growth inhibition of MAE extract of carrot seeds against (a) Aspergillus niger (b) Penicillium chrysogenum (c) Rhizopus stolonifera (d) Antifungal activity of MAE extract (e) Antifungal activity of Soxhlet extract.

MAE reduced the growth of A. niger, R. stolonifera, and P. chrysogenum with % inhibition values of 68.7%, 70.5%, and 89.3% as compared to the fungal inhibition of control i.e. 0.35%, 0.33%, 0.28% respectively against the three fungal strains. Whereas SE inhibited the growth of all three strains by 59.6%, 63.6%, and 60.7% respectively (Figure ). MAE extract of carrot seeds was found to be more effective against P. chrysogenum as compared to A. niger and R. stolonifera. MAE extract exhibited 89.3% inhibition against the tested fungi (P. chrysogenum). Higher antifungal activity proves that the biological activity of extracted components in MAE is not affected. Polar and nonpolar components of a plant extract have their mode of fungicidal action. It has been reported earlier that polar extracts (like ethanol extracts) inhibit the growth of fungi by producing reactive oxygen species reported by Sherma and Rabel [Citation36]. While nonpolar components like fatty acids increase the membrane fluidity of fungal cells. It leads to the seeping out of cellular components of fungi, causing cell death described by Thibane et al. [Citation37].
4. Conclusion
The current study found that carrot seeds are a rich source of phytochemicals, and MAE was a useful approach for extracting bioactive components from carrot seeds because it reduced extraction time significantly when compared to other conventional extraction techniques. Independent variables such as time, power level, and particle size had a significant impact on the extraction yields of all responses. The efficient extraction parameters were found for the extraction of bioactive compounds. In conclusion, MAE has the capability for the efficient and economical extraction of bioactive compounds from carrot seeds.
Disclosure statement
No potential conflict of interest was reported by the author(s).
References
- Djerrou Z, Benyezzar-Kenana H, Maameri Z, et al. An ethnopharmacological survey of medicinal plants used in the traditional treatment of human infertility in eastern Algeria. Asian Pac J Reprod. 2022;11(2):77.
- Umar G, Kaur S, Gurumayum S, et al. Effect of hot water blanching time and drying temperature on the thin layer drying kinetics of and anthocyanin degradation in black carrot (Daucus carota L.) shreds. Food Tech Biotech. 2015;53:324–330.
- Acharya UR, Mishra M, Patro J, et al. Effect of Vitamins C and E on spermatogenesis in mice exposed to cadmium. Reprod Toxicol. 2008;25:84–88.
- Leja M, Kamińska I, Kramer M, et al. The content of phenolic compounds and radical scavenging activity varies with carrot origin and root color. Plant Foods Hum Nutr. 2013;68:163–170.
- Carrot W. Carrot: history and iconography. Chronica. 2011;51(2):13.
- Dean BB, Noland T, Maguire JD. Correlation of low seed quality with growing environment of carrot. HortScience. 1989;24(2):247–249.
- Zhang QW, Lin LG, Ye WC. Techniques for extraction and isolation of natural products: a comprehensive review. Chin Med. 2018;13:13–20.
- Luque MD, Priego-Capote F. Soxhlet extraction: past and present panacea. J Chromatogr A. 2010;1217(16):2383–2389.
- Chuyen HV, Minh H, Nguyen PD, et al. Microwave-assisted extraction and ultrasound-assisted extraction for recovering carotenoids from Gac peel and their effects on antioxidant capacity of the extracts. Food Sci Nutr. 2017;6(1):189–196.
- Mandal V, Mohan Y, Hemalath S. Microwave assisted extraction-an innovative and promising extraction tool for medicinal plant research. Pharmacogn Rev. 2007;1(1):7–18.
- Dewanto V, Wu X, Liu R H. Processed sweet corn has higher antioxidant activity. J Agric Food Chem. 2002;50(17):4959–4964.
- Csepregi K, Kocsis M, Hideg É. On the spectrophotometric determination of total phenolic and flavonoid contents. Acta Biol Hung. 2013;64(4):500–509.
- Radulović N, Blagojević P. Volatile profiles of Artemisia alba from contrasting serpentine and calcareous habitats. Nat Prod Commun. 2010;5(7):1117–1122.
- Abdulrasheed A, Aroke UO, Sani IM. Parametric studies of carrot seed oil extract for the production of medicated soap. Int J Recent Dev Eng Tech. 2015;4(1):1–5.
- Fikselova M, Silhar S, Marecek J, et al. Extraction of carrots (Daucus carota L.) carotenes under different conditions. Czech J Food Sci. 2008;26:268–274.
- Elik A, Yanık DK, Göğüş F. Microwave-assisted extraction of carotenoids from carrot juice processing waste using flaxseed oil as a solvent. LWT. 2020;123:109100.
- Maeng JH, Shahbaz HM, Ameer K, et al. Optimization of microwave-assisted extraction of bioactive compounds from Coriolus versicolor mushroom using response surface methodology. J Food Process Eng. 2017;40(2):201–215.
- Özbek HN, Halahlih F, Göğüş F, et al. Pistachio (Pistacia vera L.) hull as a potential source of phenolic compounds: Evaluation of ethanol–water binary solvent extraction on antioxidant activity and phenolic content of pistachio hull extracts. Waste Biomass Valorization. 2018;3(2):232–241.
- Saci F, Louaileche H, Bey M, et al. Optimization of phenolic compound recovery and antioxidant activity from carob pulp using response surface methodology. Int Food Res J. 2017;24:1094–1101.
- Ghaffar N, Javad S, Farrukh MA, et al. Optimization of parameters for microwave-assisted extraction of phenolics and flavonoids from Euphorbia hirta by response surface methodology. Phyton (Horn, Austria). 2020;60:1–9.
- Kashyap P, Riar CS, Jindal N. Effect of extraction methods and simulated in vitro gastrointestinal digestion on phenolic compound profile, bio-accessibility, and antioxidant activity of Meghalayan cherry (Prunus nepalensis) pomace extracts. LWT- Food Sci Tech. 2022;153:112570.
- Li Y, Zhang Z, Schellhorn HE. New developments and novel therapeutic perspectives for vitamin C. J Nutri. 2012;137:2171–2184.
- Akhtar I, Javad S, Yousaf Z, et al. Microwave assisted extraction of phytochemicals an efficient and modern approach for botanicals and pharmaceuticals. Pak J Pharm Sci. 2019;32(1):223–230.
- Mohammad NA, Zaidel DNA, Muhamad II, et al. Optimization of the antioxidant-rich xanthone extract from mangosteen (Garcinia mangostana L.) pericarp via microwave-assisted extraction. Heliyon. 2019;5(10):e02571.
- Kumar M, Dahuja A, Sachdev A, et al. Valorisation of black carrot pomace: microwave assisted extraction of bioactive phytoceuticals and antioxidant activity using Box–Behnken design. J Food Sci Technol. 2019;56(2):995–1007.
- Hiranvarachat B, Devahastin S. Enhancement of microwave-assisted extraction via intermittent radiation: extraction of carotenoids from carrot peels. J Food Eng. 2014;126:17–26.
- Kaderides K, Papaoikonomou L, Serafim M, et al. Microwave-assisted extraction of phenolics from pomegranate peels: optimization, kinetics, and comparison with ultrasounds extraction. Chem Eng Process – Process Intensification. 2019;137:1–11.
- Liazid A, Palma M, Brigui J, et al. Investigation on phenolic compounds stability during microwave-assisted extraction. J Chromatogr A. 2007;1140:29–34.
- Casazza AA, Aliakbarian B, Sannita E, et al. High-pressure high-temperature extraction of phenolic compounds from grape skins: grape skin polyphenols extraction. Int J Food Sci Technol. 2012;47:399–405.
- Kaur A, Chahal KK, Kataria D, et al. Assessment of carrot seed essential oil and its chemical constituents against meloidogyne incognita. Int J Pharmacogn Phytochem. 2018;7(1):896–903.
- Angelini LG, Tavarini S, Ascrizzi R, et al. Composition and antifungal activity of the essential oils hydrodistilled from three accessions of pastinocello carrot (Daucus carota L. ssp. major). Ind Crops Prod. 2022;181:114797.
- Bampouli A, Kyriakopoulou K, Papaefstathiou G, et al. Comparison of different extraction methods of Pistacia lentiscus var. chia leaves: yield, antioxidant activity and essential oil chemical composition. J Appl Res Med Aromat Plants. 2014;1(3):81–91.
- Ksouri A, Dob T, Belkebir A, et al. Chemical composition and antioxidant activity of the essential oil and the ethanol extract of Algerian wild carrot Daucus carota L. ssp. carota. (L.) Thell. J Mater Environ Sci. 2015;6(3):784–791.
- Fernández-Marín R, Fernandes SC, Andrés MA, et al. Microwave-assisted extraction of Curcuma longa l. Oil: optimization, chemical structure and composition, antioxidant activity and comparison with conventional soxhlet extraction. Molecules. 2021;26(6):1516.
- Torres TMS, Mazzutti S, Castiani MA, et al. Phenolic compounds recovered from ora-pro-nobis leaves by microwave assisted extraction. Biocatal Agric Biotechnol. 2022;39:102238.
- Sherma J, Rabel F. Review of thin layer chromatography in pesticide analysis: 2016-2018. J Liq Chromatogr Relat Technol. 2018;41(19-20):1052–1065.
- Thibane VS, Ells R, Hugo A, et al. Polyunsaturated fatty acids cause apoptosis in C. albicans and C. dubliniensis biofilms. Biochim Biophys Acta Gen Subj. 2012;1820(10):1463–1468.