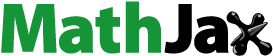
Abstract
An insight has been provided into the eutectic formation and cutaneous wound healing potential of a binary allantoin/β-D-fructose system (AF). The system was blended with folic acid for light-aided wound therapy. Solid–liquid binary phase diagram showed a differential melting depression of 88°C from the ideal line and the existence of eutectic at X1 = X2 = 0.5. Simulations of molecular mechanics showed the involvement of hydantoin C=O and ureido –NH in hydrogen bonding with hydroxyl or hydroxymethyl groups of fructose. In the eutectic-folic acid blend (AFF), H-bonds were present between =N of pterin ring A and hydantoin –NH. Additionally, pi-sigma interactions involving pterin rings and pyranose moiety were revealed. The bulk density variations showed the disruption of the allantoin template after eutectic formation. An in-depth attenuated total reflectance infrared spectroscopic analysis endorsed the findings of other investigations and vibrational frequencies of interacting functionalities were shifted to different extents. The thermal stability of the eutectic mixture was markedly compromised after the addition of folic acid. Differential scanning calorimetry indicated the presence of co-crystal in addition to eutectic. Eutectic and its blend produced nearly the same healing effects in non-infected cutaneous wounds in rabbit models under normal and photodynamic wound therapy.
1. Introduction
The potential of eutectic-based therapeutics for wound treatment has been recognized only recently [Citation1–3]. The main advantage associated with these biomaterials is the use of naturally occurring active ingredients and the possibility of the development of minimum or no-waste formulations that do not require an additional controlling agent [Citation4,Citation5]. This is expected to significantly enhance the effectiveness and economy of health care [Citation6]. An appropriate wound-healing intervention would ensure a highly regulated process to avoid complications leading to cancer [Citation7]. This is especially vital while treating non-healing and chronic wounds.
Allantoin’s facilitation of wound healing is multi-faceted [Citation8]. It ensures hydration, epithelization, mitosis and keratolytic action, and helps in the removal of necrotic tissues [Citation8,Citation9]. In the bound states such as eutectic, allantoin is less prone to degradation at pH (> 8) experienced at the infected wound site [Citation10–12]. Recently, the eutectic phenomenon and wound-healing potential of binary systems possessing allantoin have been reported [Citation12,Citation13]. Herein we present allantoin eutectics with β-D-fructose and the effect of folic acid addition on its potential to heal wounds in the presence and absence of diffused light. The direct formation of allantoin eutectic with folic acid for photodynamic wound therapy did not yield the desired results, probably due to the thermal degradation of folic acid [Citation14]. Therefore, considering that a mixture of honey and allantoin promotes wound repair [Citation15] and sugar-based deep eutectic solvents facilitate antimicrobial photodynamic therapy [Citation16], fructose was used for eutectic formation and folic acid was embedded in it [Citation17]. Sugar has effectively been used to treat antibiotic-resistant wounds [Citation18]. Also, sufficient evidence exists that the topic application of sugar-based compounds has no negative effect on wound healing [Citation19].
Folic acid and other B vitamins have applications in regenerative medicine [Citation20]. Folic acid is photostable in an anaerobic environment but degrades to 6-formylpterin by UV in the presence of oxygen. 6-formylpterin induces photooxidation of folic acid to produce hydrogen peroxide [Citation21]. These degradation pathways were observed only in the alkaline medium (pH > 7) [Citation22,Citation23]. The degradation products such as 6-carboxylpterin are highly fluorescent [Citation24] and act as photosensitizers in photodynamic wound healing [Citation25]. These factors should be considered to avoid any detrimental effects of photodynamic therapy [Citation26].
This work provides an insight into eutectic formation between allantoin and D-fructose using a combination of theoretical and experimental methods such as molecular mechanics, Fourier-transform Attenuated total reflectance infrared (ATR-IR) spectroscopy, thermal analysis, scanning electron microscopy, etc. It also emphaszes the ability of eutectic and its blend with folic acid towards healing of cutaneous wounds in rabbit models under normal conditions and in the presence of diffused light. Human and rabbit skin respond to topical medication in a similar way [Citation27].
2. Material and methods
2.1. Material
Allantoin (≥ 98%) and D-Fructose (≥ 99%) were the products of Sigma-Aldrich (Germany). Crystalline folic acid was purchased from Alfa Aesar (China). All chemicals were used as received. Ultrapure water was used in all aqueous preparations.
2.2. Preparation of allantoin-fructose mixtures (AF)
Nine binary mixtures between allantoin and D-fructose were prepared (codes AF19-AF91) through systematic variation in mole fraction of fructose from 0.1 to 0.9. In each case, first, a known quantity of D-fructose was melted in a thoroughly cleaned and completely dried glass vial. Second, a desired quantity of allantoin was added to the melt and heating was continued till liquefaction. The reaction mixture was maintained at about 1h at the same temperature to ensure completion.
2.3. Characterization of allantoin-fructose mixtures
The melting points of constituents and corresponding AF were recorded with the Gallenkamp digital melting-point apparatus (Gemini BV, Netherlands). FT-IR spectra of selected AF were obtained on a Perkin Elmer Spectrum 3 ATR-IR spectrometer. Thermal analysis was carried out using Perkin Elmer STA 8000 under a nitrogen atmosphere. The temperature varied at a rate of 10 °C/min. Scanning electron microscopy (SEM) images were taken using SEM-MIRA3 TESCAN (Czech Republic).
2.4. Simulations of molecular mechanics
Simulations of molecular mechanics and data processing were performed with Avogadro [Citation28] and BIOVIA Discovery Studio [Citation29]. The default all-atom universal force field (UFF) was utilized for this purpose. The convergence was set at 10e-7. The calculations were performed using allantoin: fructose (5:5) or AF55 system under no constraints. The system consisted of five molecules of fructose and five molecules of allantoin. The fructose comprised four pyranose and one furanose molecule. The energy minimization followed the conjugate gradient algorithm. The same procedure was repeated using eutectic and folic acid in the ratio 1:1 (AF55F). This system contained a folic acid molecule in addition to five molecules each of fructose and allantoin. The atom descriptors were assigned for explanation. The workflow is illustrated in Figure .
2.5. Wound healing in rabbit models
All wound healing procedures were performed in accordance with international/national guidelines. Ethical approval (CH444) was obtained from the Institutional Review Committee in this regard. Two male rabbits each having about 3 kg weight and 6 months of age were taken. They were anaesthetized using an injection containing xylazine (1.8 mg/kg of body weight) and ketamine (12 mg/kg of body weight). At least, six small circular skin wounds (∼1 cm wide) were created on the shaven and sanitized dorsum of each rabbit using a scalpel [Citation30,Citation31]. Potassium permanganate was used to prevent excessive bleeding. One wound in each subject remained untreated and served as control (A). The rest of the five wounds were treated with a paste possessing 10% AF55 (B), 10% folic acid (C) and folic acid-AF55 mixtures having folic acid and AF55 in the ratio 1:1 (D), 2:1 (E) and 1:2 (F), respectively. Each mixture was applied once in 24 h and therapy was continued for two weeks. One rabbit was kept in the dark, while the other was exposed to diffused sunlight for photodynamic therapy. The images were taken with a digital camera and processed using ImageJ software (NIH). At any stage, the total wound closure was calculated using Equation (1) [Citation32] and reflected in terms of the percentage of total wound area on the initial day as
(1)
(1) where W1 and Wt are wound areas measured on day 1 when the wound was created and any post-injury day t.
2.6. ADMET profiles of precursors
Adsorption-Distribution-Metabolism-Excretion-Toxicity (ADMET) profiles of β-D-fructose (in fructopyranose form), allantoin and folic acid were generated using the ADMET lab 2.0 online server [Citation33]. The molecules were drawn, and their SMILES notations were generated using ACD/ChemSketch. These SMILES were used as input for screening purposes online.
2.7. Bulk density
The bulk density was determined using a home-built volumeter using a reported procedure [Citation34]. The powdered sample was poured into a cubic cup of known volume 4.0 cm3 using a small powder funnel. The excess powder was scrapped using a spatula and the mass of the sample was determined using Laboratory High Precision Electronic Analytical Balance JJ324BC (China). The density was then calculated as mass/volume.
3. Results and discussion
3.1. Solid–Liquid phase diagram
Solid–liquid phase diagram (Figure ) shows the dependence of the melting point of allantoin-fructose mixtures (AF) as a function of the mole fraction of fructose. Two minima are observed in the system with eutectic point (85°C) existing at Xfructose = 0.5. Another minimum (87°C) exists in the fructose-rich region, where the mole fraction of allantoin is merely 0.1. Allantoin exists in an organized template where ureido moieties form a channel [Citation35]. The presence of fructose leads to the disruption of this template and causes a depression in the melting point [Citation12,Citation13]. This can be understood by keeping in view the existence of several hydrogen bond donors and acceptors in both allantoin and fructose (see ADMET profiles of precursors provided in Supplementary File 1) The eutectic point in a simple ideal mixture exists at point E in Figure . However, further depression may occur in the case of non-ideality with an ideal melting temperature existing somewhere between I and E [Citation36]. In the former, the differential melting point depression will simply be represented by ΔTIE (∼ 88°C), that is, the linear combination of the melting points of allantoin and fructose, and the experimentally determined eutectic point [Citation37]. It is worthwhile to note that both the fusion properties and complexation between HBD and HBA may be responsible for any reduction in melting point [Citation36].
3.2. Molecular mechanics
3.2.1. β-D-Fructose-allantoin system
The atom descriptors of constituent molecules are provided in Figure . Simulation of molecular mechanics performed using a predictive software system revealed the existence of conventional hydrogen bonding in a binary AF system (Figure ). The C=O in the hydantoin ring flanked by –NH acted as a hydrogen bond acceptor, whereas both the –NH groups in the ring and secondary NH in the ureidyl moiety acted as a hydrogen bond donor. In β-D-fructose, hydroxy and hydroxymethyl groups were able to accept or donate H-bonds. In addition, the oxygen in the heterocyclic ring acted as an acceptor in intermolecular hydrogen bonding with either another monosaccharide molecule or with allantoin. A detailed description of these interactions using the atom descriptors is provided in Table . These interactions show the existence of a template involving more than two molecules.
Figure 3. Ball and stick models of allantoin (left), folic acid (top), β-D-fructose (furanose and pyranose forms – bottom) with atom descriptors.

Figure 4. Conventional H-bonding (dotted lines) in allantoin and the β-D-fructose system as revealed by simulations of molecular mechanics. Fructose and allantoin are shown by thick and thin stick models, respectively.

Table 1. Conventional intra- and intermolecular hydrogen bonding interactions taking place in the allantoin-fructose and allantoin-fructose-folic acid systems.
3.2.2. Eutectic-folic acid system
The simulations of molecular mechanics in the presence of a single molecule of folic acid within a eutectic system unravelled the existence of hydrogen bonding with allantoin and pi-sigma interactions with pyranose (Figure ). The detail of these interactions using atom descriptors in Figure is provided in Table . The H-bonds were formed between =N of pterin ring A (PTA) and hydantoin -NH in allantoin. This was a rather longer bond with a length of 3.02 Å. The –OH of one of the carboxyl groups of glutamic acid was able to interact simultaneously with ureido and hydantoin C=O of allantoin. The pi-sigma interactions involved both pterin rings and CH2 in the pyranose ring and CH2 in the hydroxymethyl group substituted on the ring of monosaccharide.
Figure 5. Conventional hydrogen bonding and pi-sigma interactions in the allantoin-(β-D-)fructose (5:5) system in the presence of a single folic acid molecule as revealed by simulations of molecular mechanics. Fructose and folic acid are shown by thick and thin stick models, respectively, whereas ball and stick models represent allantoin.

Table 2. Intermolecular interactions between the folic acid and allantoin-fructose system.
3.3. Bulk density variations
The bulk density variation as a function of mole fraction of β-D-fructose is shown in Figure . It is apparent from the plot that the addition of fructose to allantoin causes a marked reduction in compactness and bulk density decreases up to Xfructose = 0.3. This proves that the organized network in allantoin is destroyed [Citation12,Citation13]. Beyond this point, the density first suffers an abrupt elevation and then starts to regain gradually. The density values exceed the density of pure allantoin when the mole fraction of fructose reaches 0.9. This is expected considering the capability of fructose to engage extensively in hydrogen bonding interactions with itself and allantoin.
3.4. Vibrational spectroscopic analysis
3.4.1. Region 3600–2500 cm−1
ATR-IR spectra of selected allantoin-fructose mixtures in the absence and presence of folic acid are displayed in Figure . The corresponding data on infrared vibrational frequencies are gathered in Supplementary Table 1 (Supplementary File 3). The vibrational frequencies in this region include symmetric and antisymmetric stretching of primary NH2 in allantoin [Citation38] and absorptions involving stretching of O–H bonds in fructose [Citation39]. The broadening of ν(OH) may be correlated to hydrogen bonding [Citation40], which may also increase the complexity of this region [Citation41]. Besides, O–H of water also appears at ∼ 3260 cm−1 [Citation42]. Earlier, the predictive software indicated the absence of the involvement of NH2 in hydrogen bonding in AF. This behaviour is unlike that of urea [Citation43]. Since νas(NH2) is changed from 3425 cm−1 in allantoin to 3434, 3435 and 3437 cm−1 in AF28, AF55 and AF82. Hence, the change in frequency may reflect that intermolecular H-bonds responsible for the network structure in pure allantoin [Citation35] are either reduced in number or weakened upon fructose addition [Citation13]. This is supported by the fact that O-H stretching frequencies are shifted to lower wavenumbers from 3395 cm−1 to 3336, 3338, and 3340 cm−1 in AF28, AF55 and AF82, which is indicative of an increase in the number of H-bonds [Citation44]. The vibrational frequencies exhibited by mixtures are also lower than that of νs(NH2), i.e. 3351 cm−1, which also endorses the inference reached above. The complexity of the system is enhanced when folic acid is added to eutectic because O–H of glutamic acid and N–H of pterin moiety have vibrational frequencies in the region 3542−3121 cm−1 [Citation45]. These values experience a redshift of 1−4 cm−1 in AF55F showing associations with eutectics involving these functionalities of folic acid.
Figure 7. ATR-IR of selected allantoin-fructose mixtures (AF28, AF55, AF82) and eutectic-folic acid blend (AF55F).

The molecular vibrations in the region 2960−2760 cm−1 are due to the stretching of C–H bonds [Citation38,Citation46]. The C–H in the hydantoin ring has a frequency of 2764 cm−1 that is shifted to 2760 cm−1 only in the case of AF82 probably due to carbon interactions [Citation47]. Also, the values are lowered compared to symmetric and antisymmetric C–H stretching frequencies exhibited by β-D-fructose that appear in the range 2960−2899 cm−1 [Citation46]. ν(CH) ∼ 2925 cm−1 in folic acid [Citation48], which is not altered in AF55F. Another peak in the IR spectrum of AF55F at frequency 2565 cm−1 is possibly due to the protonation of nitrogen in the pterin ring [Citation49]. The increase in frequency shows an increase in van der Waals interactions, which may reflect density variations in the crystal [Citation50].
3.4.2. Region 1800–1200
The symmetric and antisymmetric stretching vibrational frequencies due to hydantoin C=O are 1781 and 1740 cm−1, respectively [Citation38]. For AF, one of them is localized around 1779 cm−1 and the other below 1721 cm−1. This endorses the observation during theoretical investigations that only one of the two carbonyls in the hydantoin ring of allantoin is chiefly engaged in H-bond formation (Table ). Consequently, the latter suffered a displacement of 19−35 cm−1 towards the lower wavenumber region [Citation44]. ν(C=O) of ureidyl moiety (1680 cm−1), δ(OH) of fructose (1665 cm−1) and Amide I band of folic acid also undergo a red shift in mixtures, apparently due to associations involving these functionalities. The Amide II band of secondary NH group in folic acid is blue-shifted in AF55F to the extent of 20 cm−1 from the original position [Citation45]. Also, the stretching frequency due to the phenyl ring appearing at 1485 cm−1 in folic acid is negligibly altered in the eutectic-folic acid blend. Below this region are complex absorptions based on summative bending and stretching due to allantoin and β-D-fructose. For instance, β[H49-N11-C35] + β[H45-C33-N10 + H48-N–C] + νas(N-C35) + β[O19 = C35-N] in allantoin collectively appear at 1284 cm−1, whereas δ(OH) in-plane and δ(CCO) of fructose absorb at 1292 cm−1[Citation38,Citation46]. These frequencies were randomly altered in AF or AFF.
3.4.3. Region 1200–800
The characteristic absorptions in this region occur due to C–N and N–H stretching frequencies in allantoin/folic acid. In addition, C–O and C–C stretching including the bending vibrations δ(CCC), δ(CCO), β(COH), δ(CH) due to the pyranose ring appear in this range. Mostly, these bands are red-shifted and unravel H-bonding [Citation44]. This confirms the results obtained from molecular mechanics that ring oxygen and –OH groups in fructose form intra- or intermolecular hydrogen bonds.
3.5. Scanning electron microscopy and thermal analysis
Scanning electron microscopy images of AF55F acquired with different magnifications are shown in Figure . The structure is layered where some features of allantoin and fructose are restored [Citation51,Citation52] The edges are not sharp and cracks seem filled with eutectic, which are either due to crystallization from melt or the presence of folic acid on the surface [Citation13,Citation53]. The absence of a porous structure may produce detrimental effects during the healing process [Citation54].
Figure 8. SEM images for eutectic-folic acid blend (AF55F) with different magnifications: 2500 (left) and 5000 (right).

The thermal analysis provided further nsight into the behaviour of the system. Figure shows the change in weight per cent of the sample with an increase in temperature. It is clear from the plots that the eutectic-folic acid system (AF55F) had lower thermal stability than the corresponding binary eutectic containing allantoin and fructose only. The differential curves represented by the dotted lines in the same figure show thermodynamic events taking place because of elevation in temperature. The thermal stability of folic acid in the mixture was reduced compared to pure folic acid [Citation55]. The thermal degradation plots cross-over at a temperature nearly the same as that of the melting point of pure allantoin [Citation56]. Since allantoin decomposes at its melting point, its decomposition has a significant bearing on the stability of eutectic that degrades rapidly afterwards. The presence of folic acid or its degradation products impart stability to the ternary system and therefore, its degradation occurs less steeply after ∼ 230°C. The stability of fructose does not seem to have changed significantly after blending with allantoin [Citation57].
Figure 9. TGA plots of allantoin-fructose eutectic (AF55) and its 1:1 blend with folic acid (AF55F). The corresponding DTG plots are shown by the dashed lines.

Figure shows differential scanning calorimetry thermograms of AF55 and AF55F, respectively. These endotherms reveal the existence of a complex system instead of a simple eutectic mixture between allantoin and fructose. There are peaks which exist below the melting points of constituents of the mixture and indicate the existence of a eutectic mixture; however, if endothermic events are occurring at temperatures either below the melting point of only high melting components or above their melting points, it shows the formation of co-crystals [Citation58]. It is worthwhile to note that a eutectic system may form crystal structures with different stabilities and lead to anomalous thermal behaviour [Citation59]. If the peaks are observed in the melting point range of individual components, a system is considered to be non-interacting [Citation15].
3.6. Photodynamic wound therapy
The wounds were treated with 10% AF55 (B), 10% folic acid (C) and folic acid-AF55 mixtures having folic acid and AF55 in the ratio 1:1 (D), 2:1 (E) and 1:2 (F), whereas A remained untreated and served as control. The images taken on day 1, 7 and 9 are shown in Figure . A rapid wound healing was observed with the use of eutectic AF55 (i.e. B) and 89% wound closure occurred on day 7 (Figure ). The presence of folic acid slightly delayed healing and wound closure of about 78, 84, 86 and 85% was achieved on day 9 with C, D, E and F, respectively. This is probably due to a decline in stability of eutectic upon folic acid addition as indicated by thermal analysis earlier in the study. The control was completely healed on day 10, with a 76% reduction in wound area taking place in one-week time. All wounds were completely healed on day 12 in this case. When wounds were exposed to diffused sunlight, wounds showed signs of maximum healing after one week of treatment; however, marked wound closure was observed only after day 9, saving C (85% closure) that was treated with pure folic acid (Figure ). Around 92% of wound closures occurred on day 11 with C and E. On day 12, nearly 91, 97, and 90% were seen with eutectic B and folic acid-eutectic mixtures D and F. Here, control showed merely 60% closure on day 7. These results reflect that folic acid supports healing in the presence of light. Overall, photodynamic therapy showed a marginal difference from normal therapy because wounds were not infected and that is why antimicrobial action could not be observed.
Figure 11. Digital images of wounds for treatment in the dark and in the presence of diffused light. All wounds were created on day 1.

Figure 12. Wound contraction caused by A-F in the absence of light as observed on alternative days. Wounds were created on day 1.

Figure 13. Wound contraction caused by A-F in the presence of light as observed on alternative days. Wounds were created on day 1.

As indicated earlier, folic acid is degraded by UV light in an aerobic environment [Citation21]. 6- Carboxypterin is a highly fluorescent degradation product [Citation24], which may act as a photosensitizer and promoter of healing [Citation25]. The absence of porous structure in eutectic-folic acid blend as revealed by the scanning electron microscopy may also have limited photodynamic healing effects.
4. Conclusions
Biomaterials based on allantoin-β-D-fructose eutectic and eutectic-folic acid blends were investigated for interactions, properties and wound healing potential under normal and photodynamic therapeutic conditions. The eutectic point existed in an equimolar mixture at a temperature of 85°C. The disruption of the allantoin template occurred due to the engagement of the hydantoin ring and urediyl moiety in hydrogen bonding with hydroxyl and hydroxymethyl groups of fructose as revealed by molecular mechanics and ATR-IR spectroscopy. Carboxyl groups and pterin rings of folic acid were involved in pi-sigma and H-bond formation in the blend. Thermal analysis showed the reduction in thermal stability owing to blending with folic acid and the co-existence of eutectic and co-crystal under similar conditions. The absence of porous structure and photostability of folic acid may be considered responsible for the marginal difference in wound healing augmentation by eutectic and its blend of non-infected cutaneous wounds in rabbit models under normal and photodynamic wound therapy.
Supplemental Material
Download ()Acknowledgements
The assistance provided by CVH, Mansehra, Pakistan for wound healing investigations is gratefully acknowledged.
Disclosure statement
No potential conflict of interest was reported by the author(s).
References
- Silva E, Oliveira F, Silva JM, et al. Untangling the bioactive properties of therapeutic deep eutectic solvents based on natural terpenes. Curr Res Chem Biol. 2021;1:100003. doi:10.1016/j.crchbi.2021.100003
- Wang Y, Zhang Y, Su J, et al. Preparation of a multifunctional wound dressing based on a natural deep eutectic solvent. Chem Eng. 2020;8:14243–14252. doi:10.1021/acssuschemeng.0c05754
- Kongpol K, Chaihao P, Shuapan P, et al. Therapeutic hydrophobic deep eutectic solvents of menthol and fatty acid for enhancing anti-inflammation effects of curcuminoids and curcumin on RAW264.7 murine macrophage cells. RSC Adv. 2022;12:17443–17453. doi:10.1039/D2RA01782B
- Srinivasan H, Sharma VK, Sakai VG, et al. Transport mechanism of acetamide in deep eutectic solvents. J Phys Chem B. 2020;124:1509–1520. doi:10.1021/acs.jpcb.9b11137
- Oliveira F, Silva E, Matias A, et al. Menthol-based deep eutectic systems as antimicrobial and anti-inflammatory agents for wound healing. Eur J Pharm Sci. 2023;182:106368. doi:10.1016/j.ejps.2022.106368
- Santos F, Duarte ARC. Therapeutic deep eutectic systems towards the treatment of tuberculosis and colorectal cancer: opportunities and challenges. Molecules. 2021;26:7022. doi:10.3390/molecules26227022
- MacCarthy-Morrogh L, Martin P. The hallmarks of cancer are also the hallmarks of wound healing. Signal. 2020;13:1–13. doi:10.1126/SCISIGNAL.AAY8690
- Araújo LU, Grabe-Guimarães A, Mosqueira VCF, et al. Profile of wound healing process induced by allantoin. Acta Cir Bras. 2010;25:460–461. doi:10.1590/S0102-86502010000500014
- Paller A, Nardi R, Do H, et al. An investigation into multifaceted mechanisms of action of allantoin in wound healing. J Am Acad Dermatol. 2017;76:AAB40. doi:10.1016/j.jaad.2017.04.176
- Yamamoto S, Ohtomo M, Komatsu K, et al. Stability of allantoin and identification of its degradation compounds. Yakugaku Zasshi. 1993;113:515–524. doi:10.1248/yakushi1947.113.7_515
- Derwin R, Patton D, Strapp H, et al. Wound pH and temperature as predictors of healing: an observational study. Wound Care. 2023;32:302–310. doi:10.12968/jowc.2023.32.5.302
- Shah SWH, Imran F, Ahmad HS, et al. Allantoin eutectics with choline chloride and zinc chloride: interactions and wound healing applications. J Taibah Univ Sci. 2023;17:2208727. doi:10.1080/16583655.2023.2208727
- Shah S, Ali F, Bibi I, et al. Eutectic formation and cutaneous wound healing by binary allantoin- octadecenedioic acid system. Karbala Int J Mod Sci. 2023;9:357–364. doi:10.33640/2405-609X.3302
- Abramova AM, Kokorina AA, Sindeeva OA, et al. Molecular nature of breakdown of the folic acid under hydrothermal treatment: a combined experimental and DFT study. Sci Rep. 2020;10:19668. doi:10.1038/s41598-020-76311-y
- Koosha M, Aalipour H, Shirazi MJS, et al. Physically crosslinked chitosan/pva hydrogels containing honey and allantoin with long-term biocompatibility for skin wound repair: an in vitro and in vivo study. J Funct Biomater. 2021;12: 61. doi:10.3390/jfb12040061
- Wikene KO, Rukke HV, Bruzell E, et al. Investigation of the antimicrobial effect of natural deep eutectic solvents (NADES) as solvents in antimicrobial photodynamic therapy. J Photochem Photobiol B Biol. 2017;171:27–33. doi:10.1016/j.jphotobiol.2017.04.030
- Xiao J, Zhu Y, Huddleston S, et al. Copper metal-organic framework nanoparticles stabilized with folic acid improve wound healing in diabetes. ACS Nano. 2018;12:1023–1032. doi:10.1021/acsnano.7b01850
- Murandu M, Webber MA, Simms MH, et al. Use of granulated sugar therapy in the management of sloughy or necrotic wounds: a pilot study. Wound Care. 2011;20:206–216. doi:10.12968/jowc.2011.20.5.206
- Oryan A, Alemzadeh E, Moshiri A. Role of sugar-based compounds on cutaneous wound healing: what is the evidence? Wound Care. 2019;28:S13–S24. doi:10.12968/jowc.2019.28.Sup3b.S13
- Fernández-Villa D, Gómez-Lavín MJ, Abradelo C, et al. Tissue engineering therapies based on folic acid and other vitamin B derivatives. functional mechanisms and current applications in regenerative medicine. functional mechanisms and current applications in regenerative medicine. Int J Mol Sci. 2018;19. doi:10.3390/ijms19124068
- Dántola ML, Denofrio MP, Zurbano B, et al. Mechanism of photooxidation of folic acid sensitized by unconjugated pterins. Photochem Photobiol Sci. 2010;9:1604–1612. doi:10.1039/c0pp00210k
- Thomas AH, Suárez G, Cabrerizo FM, et al. Photochemical behavior of folic acid in alkaline aqueous solutions and evolution of its photoproducts. Chim Acta. 2002;85:2300–2315. doi:10.1002/1522-2675(200208)85:8<2300::AID-HLCA2300>3.0.CO;2-B
- Olmo F, Rodriguez A, Colina A, et al. UV/vis absorption spectroelectrochemistry of folic acid. Solid State Electrochem. 2022;26:29–37. doi:10.1007/s10008-021-05026-5
- Tsyupka DV, Mordovina EA, Sindeeva OA, et al. High-fluorescent product of folic acid photodegradation: optical properties and cell effect. J Photochem Photobiol A Chem. 2021;407:113045. doi:10.1016/j.jphotochem.2020.113045
- Tinel L, Rossignol S, Ciuraru R, et al. Photosensitized reactions initiated by 6-carboxypterin: singlet and triplet reactivity. Chem Chem Phys. 2016;18:17105–17115. doi:10.1039/C6CP03119F
- Ning X, He G, Zeng W, et al. The photosensitizer-based therapies enhance the repairing of skin wounds. Front Med. 2022;9. doi:10.3389/fmed.2022.915548
- Grada A, Mervis J, Falanga V. Research techniques made simple: animal models of wound healing. J Invest Dermatol. 2018;138:2095–2105.e1. doi:10.1016/j.jid.2018.08.005
- Hanwell MD, Curtis DE, Lonie DC, et al. Avogadro: an advanced semantic chemical editor, visualization, and analysis platform. J Cheminform. 2012;4:17. doi:10.1186/1758-2946-4-17
- B. Dassault systemes. Discovery studio visualizer, v16.1.0.15350. San Diego (CA): Dassault Systemes; 2015.
- Zanata F. Acellular dermal matrix in skin wound healing in rabbits – histological and histomorphometric analyses. Clinics. 2021;76:e2066. doi:10.6061/clinics/2021/e2066
- Rajab A, Al-Wattar W, Taqa GA. The roles of apigenin cream on wound healing in rabbits model. J Appl Vet Sci. 2021. doi:10.21608/javs.2021.97151.1104
- Chen L, Mirza R, Kwon Y, et al. The murine excisional wound model: contraction revisited. Wound Repair Regen. 2015;23:874–877. doi:10.1111/wrr.12338
- Xiong G, Wu Z, Yi J, et al. Admetlab 2.0: an integrated online platform for accurate and comprehensive predictions of ADMET properties. Nucleic Acids Res. 2021;49:W5–W14. doi:10.1093/nar/gkab255
- Pharmacopoeia E., Unit I. Bulk density and tapped density of powders, In: European pharmacopoeia 9.0. Strasbourg, France: EDQM Council of Europe; 2015: p. 359–361.
- Dall’Olio L. Survey of methodologies of pharmaceutical interest for quantification of crystal form via X-Ray powder diffraction [Doctoral dissertation]. University of Bolonga; 2021.
- Martins MAR, Pinho SP, Coutinho JAP. Insights into the nature of eutectic and deep eutectic mixtures. Solut Chem. 2019;48:962–982. doi:10.1007/s10953-018-0793-1
- Gamsjäger H, Lorimer JW, Scharlin P, et al. Glossary of terms related to solubility: (IUPAC recommendations 2008). Pure Appl Chem. 2008;80(2):233–276. doi:10.1351/pac200880020233
- Alam MJ, Ahmad S. Ftir, FT-Raman, FTIR, FT-Raman, UV–Visible spectra and quantum chemical calculations of allantoin molecule and its hydrogen bonded dimers. Biomol Spectrosc. 2015;136:961–978. doi:10.1016/j.saa.2014.09.119
- Ibrahim M, Alaam M, El-Haes H, et al. Analysis of the structure and vibrational spectra of glucose and fructose. Eclet Quim. 2006;31:15–21. doi:10.1590/S0100-46702006000300002
- Ikemoto Y, Harada Y, Tanaka M, et al. Infrared spectra and hydrogen-bond configurations of water molecules at the interface of water-insoluble polymers under humidified conditions. J Phys Chem B. 2022;126:4143–4151. doi:10.1021/acs.jpcb.2c01702
- Hansen PE, Vakili M, Kamounah FS, et al. Dictionary geotechnical engineering/wörterbuch GeoTechnik. An experimental and theoretical study. Molecules. 2014;26:1385. doi:10.1007/978-3-642-41714-6_200778
- Varrica D, Tamburo E, Vultaggio M, et al. Atr–FTIR spectral analysis and soluble components of PM10 and PM2.5 particulate matter over the urban area of Palermo (Italy) during normal days and saharan events. Int J Environ Res Public Health. 2019;16:2507. doi:10.3390/ijerph16142507
- Sagle LB, Zhang Y, Litosh VA, et al. Investigating the hydrogen-bonding model of urea denaturation. J Am Chem Soc. 2009;131:9304–9310. doi:10.1021/ja9016057
- Arunan E, Desiraju GR, Klein RA, et al. Definition of the hydrogen bond (IUPAC Recommendations 2011). Pure Appl Chem. 2011;83:1637–1641. doi:10.1351/PAC-REC-10-01-02
- Rana S, Shetake NG, Barick KC, et al. Folic acid conjugated Fe3O4 magnetic nanoparticles for targeted delivery of doxorubicin. Dalt Trans. 2016;45:17401–17408.
- Wiercigroch E, Szafraniec E, Czamara K, et al. Raman and infrared spectroscopy of carbohydrates: a review. Biomol Spectrosc. 2017;185:317–335. doi:10.1016/j.saa.2017.05.045
- Joseph J, Jemmis ED. Red-, blue-, or no-shift in hydrogen bonds: a unified explanation. J Am Chem Soc. 2007;129:4620–4632. doi:10.1021/ja067545z
- Jalilian AR, Hosseini-Salekdeh SL, Mahmoudi M, et al. Preparation and biological evaluation of radiolabeled-folate embedded superparamagnetic nanoparticles in wild-type rats. J Radioanal Nucl Chem. 2011;287:119–127. doi:10.1007/s10967-010-0661-y
- He YY, Wang XC, Jin PK, et al. Complexation of anthracene with folic acid studied by FTIR and UV spectroscopies. Biomol Spectrosc. 2009;72:876–879. doi:10.1016/j.saa.2008.12.021
- Lutz ETG, Veldhuizen YSJ, Kanters JA, et al. A variable low-temperature FT-IR study of crystalline β-d-fructopyranose and deuterated analogues. J Mol Struct. 1992;270:381–392. doi:10.1016/0022-2860(92)85041-E
- Saucedo-Acuña RA, Barrios-de la O ML, Tovar-Carrillo KL, et al. Topography and morphology of a hydrogel enriched with allantoin for biomedical purposes. Microsc Microanal. 2018;24:1420–1421. doi:10.1017/S1431927618007584
- Predoi D, Iconaru SL, Ungureanu F, et al. Characterization of sucrose thin films for biomedical applications. J Nanomater. 2011;2011. doi:10.1155/2011/291512
- Bai RG, Muthoosamy K, Tuvikene R, et al. Highly sensitive electrochemical biosensor using folic acid-modified reduced graphene oxide for the detection of cancer biomarker. Nanomaterials. 2021;11: 1272. doi:10.3390/nano11051272
- Nguyen HM, Ngoc Le TT, Nguyen AT, et al. Biomedical materials for wound dressing: recent advances and applications. RSC Adv. 2023;13:5509–5528. doi:10.1039/D2RA07673J
- Vora A, Riga A, Dollimore D, et al. Thermal stability of folic acid. Thermochim Acta. 2002;209–220:209–220. doi:10.1016/S0040-6031(02)00103-X
- Svetlichny G, Külkamp-Guerreiro IC, Dalla Lana DF, et al. Assessing the performance of copaiba oil and allantoin nanoparticles on multidrug-resistant Candida parapsilosis. J Drug Deliv Sci Technol. 2017;40:59–65. doi:10.1016/j.jddst.2017.05.020
- Verma P, Shah NG, Mahajani SM. A novel technique to characterize and quantify crystalline and amorphous matter in complex sugar mixtures. Food Anal Methods. 2020;13:2087–2101. doi:10.1007/s12161-020-01789-1
- Patel RD, Raval MK. Differential scanning calorimetry: A screening tool for the development of diacerein eutectics. Results Chem. 2022;4:100315. doi:10.1016/j.rechem.2022.100315
- Hall CL, Potticary J, Hamilton V, et al. Metastable crystalline phase formation in deep eutectic systems revealed by simultaneous synchrotron XRD and DSC. Chem Commun. 2020;56:10726–10729. doi:10.1039/d0cc04696e