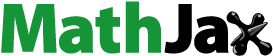
Abstract
The development of environmental remedies using ecofriendly and green technologies is attracting increasing research attention. Herein, the green synthesis of molybdenum oxide (MoO3) nanoparticles (NPs) using extract of selected Asteraceous flora species is reported for the first time along with the investigation of the degradation ability for organic pollutants and pollen morphological characteristics of wetland species of the family Asteraceae. An X-ray diffraction analysis confirmed the formation and crystalline structure of the MoO3 NPs. Fourier transform infrared spectra of Lactuca Serriola extract indicated the presence of capping agents, reducing agents, and phytochemicals. Scanning electron microscopy images revealed the almost spherical shape of the MoO3 NPs. A qualitative and quantitative palynological investigation of 21 species belonging to 12 genera of the Asteraceae from different wetlands of Azad Jammu and Kashmir is also reported. The MoO3 NPs degraded 99% of crystal violet dye within 30 minutes with a degradation rate of 0.022 min−1. The cost-efficient and green synthesis method for MoO3 NPs with high catalytic activity provides a new platform for the development of photocatalysts.
1. Introduction
Organic dyes like azo dyes are synthesized at a large scale for diverse applications [Citation1] in the textile and printing industries [Citation2], resulting in contamination of water bodies [Citation3] and an increase in the chemical oxygen demand, which affects the process of photosynthesis in aquatic plants [Citation4]. Other sources of contamination of aquatic environments are pharmaceutical and agriculture industries owing to the discharge of effluents containing organic chemicals [Citation5], which pose a serious risk to aquatic life and the food chain [Citation6].
For the removal of organic pollutants, nanomaterials have emerged as promising photocatalysts, which operate under the action of ordinary sunlight [Citation7], owing to their special structure [Citation8] and size (10−9 nm) [Citation9] as well as the variety of starting materials and synthesis methods [Citation10]. In photocatalytic processes, visible light activates the photocatalyst [Citation11], which produces reactive oxidative species (ROS) that convert organic chemicals adsorbed on the photocatalyst surface into H2O and CO2 [Citation12].
Various chemical methods have been used to synthesize nanomaterials [Citation13]; however, the chemicals used in these synthesis routes are absorbed on the photocatalyst surface, enhancing the toxicity of the nanomaterials [Citation14] to the environment, which overshadows their great success in the degradation of organic pollutants [Citation15]. To enhance the properties of nanomaterials without using chemicals, extracts of plants and plant materials [Citation16] provide a cost-effective, ecofriendly, less toxic, and safe source for the synthesis of nanomaterials [Citation17,Citation18], including phenolic compounds, tannins, and flavonoids, which play a critical role in treatment [Citation19]. These phytochemicals also play an important role as capping agents and stabilizing agents in nanomaterial synthesis processes [Citation20]. In fact, plant extract–assisted nanoparticles (NPs) were reported as efficient photocatalysts for organic dye degradation [Citation21]. In the green synthesis of NPs using plant extracts, very low temperature is required [Citation22] and the formation of toxic byproducts is minimized [Citation23], thus reducing the energy required [Citation24] and the negative impact of nanotechnology for the production of NPs [Citation25]. Plant extracts contain reducing agents and phytochemicals [Citation26], which convert Mo6+ to Mo0 and act as capping agents, respectively [Citation27]. Furthermore, the green synthesis routes to NPs can be applied at large scale [Citation28] and contribute to maintain a pollution-free environment [Citation29].
Molybdenum trioxide (MoO3) is an n-type semiconductor [Citation30] with a bandgap lying in both the visible and ultraviolet regions, i.e. from 2.69–3.6 eV [Citation31]. MoO3 exists in three phases, i.e. hexagonal (h), monoclinic (β), and orthorhombic (α) phases [Citation32], depending on the synthesis method [Citation33]. The β and h phases have been widely studied owing to their excellent physicochemical properties [Citation34]. MoO3 is an attractive photocatalyst for oxidation reactions because of the high positive potential of the valence band [Citation35]; however, its low visible-light absorbance and fast recombination of electron–hole pairs limit its efficiency [Citation31]. Moreover, MoO3 cannot reduce the oxygen molecule owing to the high potential of the conduction band [Citation36].
MoO3 has been previously synthesized using plant extracts. For instance, Mamatha et al. synthesized MoO3 from Centella Asiatica plant, achieving a degradation rate of 81% for direct green dye [Citation37]. Thekkethil et al. prepared nano-MoO3 with a removal efficiency of 93.83% under daylight illumination [Citation38]. Al-Otaibi et al. synthesized yttrium-doped molybdenum oxide NPs, which degraded 88% of methylene blue dye [Citation39]. Khan et al. prepared a ZnIn2S4/MoO3 heterojunction for the degradation of methyl orange with an efficiency of 90% within 100 min [Citation40]. Zhongxiang Yang et al. prepared Bi2Mo3O12 and Bi2Mo3O12/MoO3 composites for the degradation of crystal violet (CV) dye, reaching an efficiency of almost 83% in 60 min [Citation41].
In this research, MoO3 NPs were synthesized for the first time using an extract of Lactuca Serriola and evaluated for photocatalytic activity in environmental remediation applications. L. serriola, a member of Asteraceae commonly used to treat cough, bronchitis, asthma, and gastrointestinal symptoms [Citation42], is composed of organic (fat, gums, glycosides, and volatile oil) and inorganic compounds (vitamin B1, vitamin C, vitamin E, and vitamin K) as well as lactucarium (triterpenoid saponin) [Citation43] and participates in the reduction of metal ions [Citation44]. The synthesized material was characterized via X-ray diffraction (XRD), Fourier transform infrared (FTIR) spectroscopy, energy-dispersive spectroscopy (EDS), and scanning electron microscopy (SEM). Using the as-prepared MoO3 NPs and a locally purchased 60 W bulb, 99% photodegradation of CV dye was achieved in just 30 min.
The pollen morphological characteristics of wetland species of the Asteraceae family were also investigated in this study. The family Asteraceae is eurypalynous [Citation45] and contain zonocolporate pollen [Citation46]. The study of pollen fertility is important because it plays a major role in the production of fruits and seeds, especially in flowering plants [Citation47]. Pollen fertility depends on the weather conditions; for instance, the pollen fertility of some species of the genus Tradescantia is greater in hot weather [Citation48]. Due to its cosmopolitan distribution and predominance, Asteraceae is attracting interest among the angiosperm families. Different researchers in Pakistan studied the Asteraceae family with reference to the pollen morphology; e.g. Perveen et al. examined the pollen morphology of 24 species from the Asteraceae family via light and scanning microscopy [Citation49]. Hayat et al. studied the 22 species of the genus Artemisia using both light microscopy and SEM [Citation50]. The morphology of pollen and especially the study of the exine sculpture of 10 species belonging to the Asteraceae family of the Deosai Plateau of the northern side of Pakistan have been analyzed [Citation51]. Moreover, Sardar et al. analyzed different aspects of aquatic plants in Pakistan, especially their palynomorphology, anatomy, and medicinal characteristics [Citation42]. However, the pollen morphology of the Asteraceae family of wetland flora in Pakistan has not been studied yet. This study aims to provide useful in-depth information on the pollen morphological diversity and fertility of selected species of Asteraceae having economic impact to identify freely reproducing species for environmental conservation, as well as a qualitative and quantitative analysis of the exine structure as a basis for future studies.
2. Materials and methods
2.1. Materials
Ammonium molybdate [(NH4)2MoO4, 99.98%] and CV dye (97%) were purchased from Sigma Aldrich. Identification of L. serriola plant was confirmed by the botanist Dr. Hafiza Amina from the University of Narowal.
2.2. Preparation of extract from L. serriola plant
Plants were collected from different localities of Azad Kashmir (Section S1.1). Leaves of L. serriola plant (20 g) were collected, cut into smaller pieces, and added to ethanol (100 mL) in a round bottom flask [Citation52]. The flask was heated on a heating mantel at 25 °C for a day. The ethanolic extract of L. serriola was collected after filtering the solution.
2.3. Synthesis of MoO3 NPs
For the synthesis of MoO3 NPs, the method proposed by Tanveer et al. [Citation53] and Baig et al. [Citation54] was used. Briefly, 2 M (NH4)2MoO4 solution (25 mL) was poured in a beaker, which was put on a hot plate for continuous stirring. A dilute solution of the prepared extract in a 1:1 ratio was poured drop by drop in the beaker under stirring to obtain a homogeneous mixture. The mixture was filled in centrifuge tubes and centrifuged to separate the MoO3 NPs. After the separation, the solvent precipitation method was used to purify the MoO3 NPs by adding a sufficient amount of ethanol to the mixture [Citation55]. The product was taken to an oven for drying. In a furnace, the dried sample was annealed at 400 °C for 3–4 h. The synthesis process is depicted in Figure .
2.4. Plant collection, identification, and preservation
Plants in their flowering condition were collected from different localities of Azad Kashmir (Figure S1). Three stations of wetlands including Banjosa Lake, Mangla Lake, and the wetlands of Kotli valley were visited in August and September (Table S1). The plants were collected in fresh form to obtain their pollen from closed ripe anthers, dried using blotting paper to remove the moisture content, and pressed. The plants were identified by comparison with herbarium samples of the Herbarium of Pakistan (ISL) and with the morphology reported in Flora of Pakistan [Citation56]. The identified species were poisoned by mixing 1 L of ethanolic plant extract with 6 g of mercuric chloride for 20 s followed by drying using blotting paper [Citation57]. Standard herbarium sheets were used for mounting the poisoned plants.
2.5. Palynological studies using light microscopy
Anthers from the flower were separated using needles or forceps, placed on microscopic glass slides, crushed using a glass rod, and then acetolyzed. The pollen was observed using a light microscope Nikon with 10× resolution, and photographs were taken with different resolutions using a Leica Dialux 20 light microscope. Ten pollen grains were measured to obtain the mean value. Other measurements include polar diameter, equatorial diameter, thickness of exine, number of colpi, width and length of colpi, width and length of pores, width and length of spines, and number of spines present among the colpi. For the data analysis, the arithmetic mean and standard error were determined using SPSS 16.0 version. The size and shape of the pollen grains were determined using Equation Equation1(1)
(1) as follows [Citation58]:
(1)
(1)
where E is the equatorial diameter and P represents the polar diameter.
The fertility of pollen was calculated using Equation Equation2(2)
(2) :
(2)
(2)
where fertile pollen and sterile pollen refer to completely stained pollen and lightly stained and damaged pollen, respectively, based on ocular observation.
2.6. Pollen study via SEM
Samples for SEM observation were prepared according to the method proposed by Manfred Von Ardenne. Briefly, pollen grains were crushed in 45% acetic acid and dusted onto double-sided tape fixed to aluminum stubs using a fine pipette. A Jeol JSM-T200 microscope at 15 kV was used for observing the pollen and capturing images. Descriptive pollen terminologies according to Punt, Hoen et al. were used [Citation59].
2.7. Photocatalytic analysis
The organic pollutant used in this study was CV dye [Citation27]. Visible light was provided by a 60 W bulb [Citation54]. A 7 ppm CV dye solution (30 mL) was poured in a 200 mL beaker and 10 mg of MoO3 NPs was then added. A catalytic chamber was made to perform this experiment. The beaker was set on an orbital shaker for 1 h in the dark to reach the adsorption–desorption equilibrium as previously reported [Citation60]. The first reading of absorbance, which corresponded to the adsorption capacity of the catalyst, was recorded on a ultraviolet–visible (UV–vis) spectrophotometer after 1 h. The visible-light source was switched on to evaluate the catalytic degradation activity. The first value under visible-light irradiation was recorded after 15 min. The absorbance was measured every 15 min.
The degradation of the organic chemical was calculated using Equations Equation3(3)
(3) and Equation4
(4)
(4) [Citation61]:
(3)
(3)
(4)
(4) where C0 and C1 are the initial concentration of organic chemical before adding the catalyst and after adding the catalyst at specific intervals, respectively, and A0 and A1 represent the initial absorbance of organic dye before adding the catalyst and after adding the catalyst at specific intervals, respectively.
The mineralization degree of CV dye after and before the photocatalytic experiment was investigated by determining the total organic content (TOC) in the dye solution using a TOC-VCPH/CPG analyzer and calculated according to Equation Equation5(5)
(5) [Citation62]:
(5)
(5)
Factors affecting the photodegradation process and radical scavenging experiments were also performed and discussed [Citation63].
The Scherrer equation (Equation Equation6(6)
(6) ) was used to calculate the crystallite size of the NPs [Citation36]:
(6)
(6)
where k (0.9) is the shape factor, λ
is the wavelength (Cu Kα 1 radiation), θ is the Bragg diffraction angle, and β represents the full wavelength at half maximum.
2.8. Characterization
The structural features and formation of MoO3 NPs were investigated via XRD using a PANalytical X’Pert PRO diffractometer. The FTIR spectra of L. Serriola plant extract were recorded using a Bruker Alpha Platinum ATR spectrometer. The shape of the MoO3 NPs was determined via SEM using a LEO 1530 microscope. The elemental content was determined via EDS (JEOL JCM-6000 Plus SEM). The photocatalytic and optical analyses were performed using a UV–vis spectrophotometer (Cecil 7000 series).
3. Results and discussion
3.1. XRD analysis
The crystalline structure of the synthesized material was examined via XRD. The presence of sharp peaks in the XRD pattern shown in Figure confirmed the synthesis of the MoO3 NPs as a highly crystalline material with a crystallite size of 37.43 nm. The peaks at 16.8°, 27°, 32.4°, 49°, 52°, 57.2°, 67°, and 78° of MoO3 are in good agreement with the JCPDS card No. 05-0508 [Citation52]. Meanwhile, the peaks at 43.5°, 59.5°, and 75.4° confirmed the presence of molybdenum (JCPDS 01-1208) [Citation64,Citation65].
3.2. FTIR
To investigate the presence of reducing and capping agents in the extract, the FTIR spectrum of the sample was recorded to identify the functional groups [Citation22]. A sharp peak at 1051.1 cm−1 indicated the presence of siloxane (Si–O–Si) [Citation60]. Peaks at 1642, 1164.2, and 724 cm−1 can be attributed to carbonyl (C = O) [Citation61], ether (C–O) [Citation62], and halide (C–Cl) groups [Citation66]. Two side-by-side peaks at 2860.4 and 2932.6 cm−1 showed the presence of hydrocarbons (CH2 and CH3, respectively) [Citation67]. A wider and broad peak at 3339.3 cm−1 can be ascribed to alcohol (OH) group [Citation53]. The observation of the peaks for these functional groups and the formation of the MoO3 NPs are indicative of the presence of reducing and capping agents in L. Serriola extract (Figure ).
3.3. SEM and EDS analysis of MoO3 NPs
The shape and surface morphology of the MoO3 NPs was studied via SEM (Figure a and b), which revealed the presence of nearly spherical NPs with a particle size ranging from 80 to 110 nm. Furthermore, according to the EDS results shown in Figure c, the elemental composition was 73.81% molybdenum and 11.65% oxygen, and the presence of three peaks of molybdenum evidenced the purity of MoO3.
3.4. Optical properties
3.4.1. Bandgap analysis
The bandgap of an efficient photocatalyst should be in the visible region. Using the Tauc equation (Equation Equation7(7)
(7) ) and the UV–vis spectra of the MoO3 NPs, the bandgap energy was calculated to be 2.27 eV [Citation68], which is suitable for photocatalytic reactions under visible-light irradiation.
(7)
(7)
Plasmonic photocatalysis improves the visible-light absorption of materials [Citation69]. Also, the charge recombination can be suppressed by shifting the Fermi level under visible light [Citation70]. The higher bandgap of metal oxide NPs tends to redshift when a plasmonic material (Mo) is anchored on a metal oxide (MoO3), enhancing the visible-light absorption [Citation71]. Mo(0) shows tunable surface plasmonic resonance (SPR), which results in a longer wavelength [Citation72]. Therefore, the low bandgap of 2.27 eV irrespective of the size of the MoO3 NPs can be attributed to the presence of Mo(0) in MoO3, for which the capping agents in the L. Seriola plant extract are most likely responsible. Mo(0) was also detected in the XRD analysis.
3.4.2. Photocatalytic activity of MoO3 NPs
A photocatalytic experiment was performed using CV dye under visible-light irradiation. Control photodegradation experiments were also conducted in the dark and under visible light without photocatalyst [Citation73]. In the absence of a photocatalyst, no decrease in absorbance was observed with increasing contact time, which proved the efficiency of the MoO3 NPs (Figure ).
The MoO3 NPs degraded 99% of CV dye in just 30 min. This enhanced photocatalytic activity can be ascribed to the presence of Mo(0) in MoO3 reducing the positive potential of the conduction band (Figure 10).
To investigate the effect of the catalyst dose, dye concentration, and pH on the degradation process, a series of experiments were performed, i.e. varying the catalyst dose from 5 to 20 mg at a fixed dye concentration of 7 ppm and 30 mL volume, changing the dye concentration from 3 to 9 ppm dye with a photocatalyst dose of 10 mg and 30 mL volume, and the pH from 6 to 9 with a dye concentration of 7 ppm, a photocatalyst dose of 10 mg, and 30 mL volume. Results revealed that the optimized conditions were a dye concentration of 7 ppm, a photocatalyst dose of 10 mg, and a pH of 8 (Figure ). The photodegradation rate increased efficiently with increasing photocatalyst dose due to the increased number of active sites at a constant concentration of CV dye [Citation74]. A slight decrease in the photodegradation rate was observed upon moving from 10 to 20 mg, which may be due to photocatalyst aggregation lowering the scattering of incident light. Excessive amount of catalyst usually blocks the penetration of incident light into the solution, which decreases the photocatalytic efficiency [Citation75].
In the case of the effect of the CV dye concentration, the photodegradation rate increased first due to the availability of binding sites of the photocatalyst but then decreased upon increasing the dye concentration from 7 to 9 ppm, which can be attributed to the lack of binding sites of photocatalyst.
The effect of pH on the degradation process is important because it affects both the photocatalyst and dye molecule, particularly the surface charge of the former and the speciation (pKa) of the latter [Citation76]. Generally, electrostatic interactions between metal oxide surface, solvent molecules, substrate, and charged radicals generated during the photocatalytic reaction depend largely on the pH [Citation77]. Furthermore, protonation and deprotonation of the dye molecule and the surface of the photocatalyst depend on the solution pH [Citation78].
The degradation efficiency for CV dye was found to increase when changing from acidic to basic conditions. At low pH, the protons in the solution compete with the cationic CV dye, resulting in a low photodegradation rate of CV dye. Generally, the photocatalyst surface becomes positively charged at low pH values, which causes an electrostatic repulsion between the photocatalyst and the cationic part ( = N+ = ) of CV dye, decreasing the adsorption and, in turn, the photocatalytic efficiency [Citation79]. Meanwhile, at alkaline pH, the bonding between metal and hydroxyl ions causes a negative charge on the photocatalyst surface, toward which the cationic CV dye is electrostatically attracted, leading to an efficient CV dye degradation. At higher pH values, hydroxyl radicals, which exhibit a high oxidation potential, are the predominant species [Citation68,Citation80].
The optimal contact time for the photocatalytic reaction in the dark was determined to be 60 min by performing a series of experiments with different durations, i.e. 40, 50, 60, and 70 min (Figure ).
Figure 7. Optimization of contact time between the photocatalyst and crystal violet dye in the dark.

The decrease in particle size tends to increase the surface area and number of NPs particles attacking CV dye [Citation81]. Therefore, the small size of the MoO3 NPs (80–110 nm) contributed to the enhanced photocatalytic activity.
A TOC analysis was conducted to test the MoO3 NPs for mineralization of CV dye (Table ). The TOC value of CV dye before the photocatalytic experiment was 5.23 mg/L, which decreased after adding the photocatalyst due to mineralization of CV dye. A degree of mineralization of almost 92% was observed within 50 min (Figure ).
Figure 8. Degree of mineralization after adding the MoO3 nanoparticles in a crystal violet dye solution.

Table 1. Total organic content (TOC) before and after dye mineralization.
ROS play an important role in the degradation of organic dyes. To identify the ROS responsible for the photodegradation, scavenger tests were performed using EDTA-2Na for holes (h+), isopropanol for hydroxyl radicals (OH•), H2O2 for electrons (e−), and benzoquinone for superoxide radicals (O2−•). The most pronounced decrease in photodegradation was observed when using isopropanol. The addition of EDTA-2Na also caused a dramatic decline in degradation, whereas the organic dye was degraded to some extent in the presence of benzoquinone and H2O2 (Figure ) [Citation82]. Therefore, the predominant ROS responsible for the photodegradation of CV dye was OH•, followed by h+, O2−•, and e− in this order.
3.5. Proposed photocatalytic reaction mechanism
In a photocatalyst, electrons in the valence band are excited under the influence of visible light into the conduction band, leaving holes behind (Equation 8) [Citation83–85]. These newly generated holes exhibit a very strong oxidizing ability and interact with H2O (Equation 9), converting OH− to OH• (Equation Equation1(1)
(1) 0). This unstable OH• attacks the organic dye to generate CO2 and H2O (Equations 1 and Equation1
(1)
(1) 2) [Citation85]. Meanwhile, an electron present in the conduction band converts O2 to O2•− (Equation Equation1
(1)
(1) 3), which is in turn transformed to HOO• (Equation Equation1
(1)
(1) 4). HOO• produces OH− to oxidize the organic dye (Equations 15 and Equation1
(1)
(1) 6) [Citation86]. Here, fast recombination of the electron–hole pairs generated under visible-light irradiation [Citation86] does not occur because Mo(0) captures electrons from the conduction band of MoO3, thus enhancing the photocatalytic activity of the MoO3 NPs (Figure and 12) [Citation38].
3.6. Comparison of this work with recent efficient photocatalysts
Table summarizes a comparison of the removal efficiency of the MoO3 NPs with that of previously reported efficient photocatalysts. As can be extracted from the table, the low bandgap (2.27 eV), inhibition of recombination of electron–hole pairs, cost effectiveness, and environment-friendly synthesis of the MoO3 NPs render this material a superior photocatalyst compared with previously reported photocatalysts.
Table 2. Comparison of the photocatalyst prepared in this work with recent efficient photocatalysts.
3.7. Stability of MoO3 NPs
A stability test was performed to determine the suitability of the MoO3 NPs for industrial applications (Figure ) [Citation87]. After five cycles of photocatalytic reaction, only a 12% decrease in the photocatalytic efficiency was observed. The decrease in efficiency may be due to deactivation or loss of photocatalytic material [Citation68]. The stability of the MoO3 NPs was also confirmed via a SEM analysis, which revealed that after the 5th cycle of photodegradation, the porosity of the MoO3NPs was slightly reduced and aggregation occurred due to photodissolution and photocorrosion [Citation88]. Moreover, no considerable change in the SEM image of the synthesized MoO3 NPs was observed after the photocatalysis experiment, demonstrating the stability of the MoO3 NPs (Figure ).
3.8. Shape and size of pollens
The pollen grains evaluated in this study exhibited oblate spheroidal, spheroidal, and prolate shapes (Figures S2 and S3). Prolate spheroidal pollen was observed in Galinsoga parviflora, Erigeron canadensis, Erigeron bonariensis, Eclipta prostrata, and Bidens bipinnata. Oblate spheroidal pollen was seen in Achillea millefolium and Bidens pilosa. Spheroidal pollen was found in Anaphalis nepalensis, Aster squamatus, Cyathocline purpurea, and Myriactis nepalensis. The prolate shape is characteristic of Launaea procumbens and Ageratum conyzoides. Thus, considerable differences in the pollen shape of studied species were observed (Table S2).
The size of the pollen was determined according to the P/E ratio. The largest size of pollen in polar view was observed in Bidens pilosa 26 (28.66 ± 1.76) 32 μm, while the smallest pollen was found in Ageratum conyzoides 15 (18 ± 2.64) 20 μm and Eclipta prostrata 17.9 (19.3 ± 0.93) 21.1 μm (Figure S2). The largest pollen size in equatorial view was observed in Bidens bipinnata 27 (29 ± 1.15) 31 μm, and the smallest pollen size in equatorial view was seen in Eclipta prostrata 16.5 (18.23 ± 1.04) 20.1 μm and Ageratum conyzoides 16 (18.33 ± 2.08) 20 μm. The P/E ratio also shows the diversity of pollen grains since the highest value was observed in Anaphalis nepalensis (1.22) and Myriactis nepalensis (1.11) (Figure S2).
3.8.1. Pollen aperture and type
The aperture types varied between species, with the lacuna type being present in Achillea millefolium, Aster squamatus, Bidens pilosa, and Erigeron bonariensis and nonlacuna types in Ageratum conyzoides, Anaphalis nepalensis, Bidens bipinnata, Cyathocline purpurea, Eclipta prostrata, Erigeron canadensis, Galinsoga parviflora, Launaea procumbens, and Myriactis nepalensis. Ten species of Asteraceae showed tricolpate pollen [Citation50], as documented for the 22 taxa of the genus Artemisia. The dizonocolporate pollen of the studied species varied from previously reported results. Different shapes of pollen have been studied according to the P/E ratios [Citation89]. Garnatje and Meo [Citation45,Citation90] observed that the pollen of the genus Echinops exhibits similar characteristics, except that of the species E. strigosus, which was therefore associated with another genus of Asteraceae.
3.8.2. Micromorphology of spines
Variations in the number and length of spines were observed in this study via SEM. The length of spines ranged from 7.4 μm for Bidens pilosa to 2.7 μm for Erigeron bonariensis. The highest number of spines was observed in Bidens bipinnata (6–9 μm), while the lowest number of spines was found in Erigeron Canadensis, L. serriola, and Launaea procumbens (3–5 μm) (Figure S4 and S5). Between colpi, the number of spine rows is also a distinguishing characteristic in this family and varied from 3–4 μm to 12–13 μm. Some genera of the Asteraceae can be distinguished according to their pollen shape, size, and number of spine rows between the colpi and spine length [Citation91], i.e. A. millefolium and A. santolina of the Achellia genus. Variations were also observed in the number of spines between the colpi; A. mellifolium has 5–6 rows of spines, while A. santilina has 4–5 rows of spines among each colpi. The 4–5 rows of spines in the pollen of A. mellifolium are in accord with recently published results [Citation92]. Pollen with spines is considered to be the primitive characteristic compared with spineless pollen. Tomb, Larson et al. and Abid and Qaiser [Citation93,Citation94] also analyzed the number and length of spines among the colpi and considered them as distinguishing characteristics for the genus Inula of the Asteraceae family. The present results regarding Ageratum conyzoides are consistent with those previously reported [Citation49,Citation95], that is, equatorial diameters ranging from 16.5–20 μm and polar diameters ranging from 15 to 20.5 μm.
3.9. Fertility percentage
Table S2 summarizes the pollen fertility results, showing average pollen fertility values of 97.2 for Eclipta prostrata, 93.1 for Erigeron bonariensis, 66.2 for Myriactis nepalensis, and 70 for Bidens pilosa. The P/E ratio is taxonomically useful at the species level [Citation95], with a P/E ratio of 1.03 μm in Ageratum conyzoides evidencing a similarity in the exine thickness, shape, and type of pollen.
3.9.1. Exine ornamentation
Species can be differentiated on the basis of their exine. All types of pollen evaluated in this study exhibit echinate exine sculpturing with different types of spines. Achillea millefolium have spines with a short muronate tip and a short base, Ageratum conyzoides, Galinsoga parviflora, and Enhydra flactuans show spines with sharp ends, and Anaphalis nepalensis spines have tapering ends. The exine thickness ranges from the highest value of 6.2 μm in Bidens bipinnata to the lowest value of 1.2 μm in Ageratum conyzoides (Figure S2).
The pollen and spine morphology as well as data on pollen fertility of the studied species are summarized in Table S5. The exine thickness varies substantially and provides information at the level of species; for instance, Bidens bipinnata and Bidens pilosa have different exine thickness. Four distinct pollen types are documented on the basis of the exine sculpturing in this study, i.e. nonechinate, echinate, spinulose, and echinolophate tectum. Telleria is also observed in the exine ornamentation of pollen grains of some other taxa of the Asteraceae family [Citation96]. Pollen fertility of the genus Launaea was reported, revealing that fertility of pollen is important in cytological studies of chromosomes [Citation97]. The qualitative and quantitative characteristics of pollen have been partially studied [Citation98].
The present study will be helpful for the correct identification and species delimitations of Asteraceous flora from wetlands. To achieve more conclusive results, further studies are required using cosmopolitan taxa.
3.10. Taxonomic key of Asteraceae species based on pollen micromorphological features
1, Echinate sexine and trizonocolporate pollen having lacuna aperture. … Achillea millefolium
1', Echinate sexine and trizonocolporate pollen with nonlacuna aperture 2
2, Oblate spheroidal pollen shape with a P/E index of 1.04 … … … Ageratum conyzoides
3, Echinate sexine and a number of spines between colpi of 4–8 3
2', Spheroidal pollen shape with a P/E index of 1.22 Anaphalis nepalensis
3' Echinate sexine and a number of spines between colpi of 5–7 4
4, Spheroidal pollen shape with a P/E index of 0.9 Aster squamatus
5, Echinate sexine and a number of spines between colpi of 6–9 5
4', Prolate to spheroidal pollen shape and a P/E index of 1.07 Bidens bipinnata
5', Echinate sexine and a number of spines between colpi of 4–8 6
6, Oblate spheroidal pollen shape and a P/E index of 1.11 Bidens pilosa
7, Exine thickness of 3.93 μm and a number of spines between colpi of 4–6 7
6', Oblate spheroidal pollen shape and a P/E index of 1.03 Cyathocline purpurea
7', Exine thickness of 2.6 μm and a number of spines between colpi of 4–6 8
8, Prolate to spheroidal pollen shape and a P/E index of 1.05 Eclipta prostrate
9, Echinate, dizonocolporate pollen and a number of spines between colpi of 3– 9
8', Prolate to spheroidal pollen shape with a P/E index of 1.08 Erigeron bonariensis
9', Echinate, trizonocolporate pollen and a number of spines between colpi of 3–5 10
10, Prolate to spheroidal pollen shape with a P/E index of 1.10 Erigeron Canadensis
11, Exine thickness of 3.6 μm and a number of spines between colpi of 6–8 11
10', Prolate to spheroidal pollen shape and a P/E index of 1.04 Galinsoga parviflora
11', Exine thickness of 4.16 μm and a number of spines between colpi of 3–5 12
12, Prolate pollen shape with a P/E index of 1.04 Launaea procumbens
13, Exine thickness of 2.96 μm and a number of spines between colpi of 5–6 13
12', Spheroidal pollen shape with a P/E index of 1.1 Myriactis nepalensis.
4. Conclusion
MoO3 NPs were successfully synthesized using the extract of selected Asteraceous flora species and characterized using different techniques. The optical bandgap analysis showed that the bandgap of the green synthesized MoO3 NPs was 2.27 eV, suggesting its suitability as a photocatalyst for the degradation of organic pollutants. In fact, an excellent degradation ability of up to 99% for CV dye was achieved in just 30 min. The morphological characteristics of the pollen of wetland species of the Asteraceae family were also investigated with the help of light and scanning microscopy analyses. Variations in the pollen characteristics revealed that the pollen morphology can be used to classify and identify taxa. Furthermore, pollen fertility is an important parameter to study the adaptation in plants and can contribute to the discovery of new species when considered along with other taxonomic characteristics. The single-step green and economical synthesis, bandgap of 2.27 eV in the visible region, fast reaction kinetics of 0.022 min−1, and excellent degradation ability demonstrated that the MoO3 NP material is a promising photocatalyst for environmental water pollution remediation.
Supplemental Material
Download MS Word (27.7 MB)Disclosure statement
No potential conflict of interest was reported by the author(s).
Additional information
Funding
References
- Adeleye AS, Conway JR, Garner K, et al. Engineered nanomaterials for water treatment and remediation: costs, benefits, and applicability. Chem Eng J. 2016;286:640–662. doi:10.1016/j.cej.2015.10.105
- Abdipoor A, Taheri A, Rangin A. New magnetic graphene oxide core–shell functionalized SBA-15 dual template imprinted polymer for µ-solid phase extraction of nortriptyline and amitriptyline in mice plasma. Sep Purif Technol.
- Ateeb M, Asif HM, Ali T, et al. Photocatalytic and antibacterial activities of bio-synthesised silver nanoparticles (AgNPs) using Grewia asiatica leaves extract. Int J Environ Anal Chem. 2023: 1–19. doi:10.1080/03067319.2022.2158328
- Khalid A, Zubair M. A comparative study on the adsorption of Eriochrome Black T dye from aqueous solution on graphene and acid-modified graphene. Arab J Sci Eng. 2018;43(5):2167–2179. doi:10.1007/s13369-017-2543-x
- Asghar MZ, Katubi KM, Hassan M, et al. A new Y-Zr/g-C3N4 nanoflakes anchored mesoporous silica composite for efficient environmental remediation applications. Diamond Relat Mater. 2023;135:109850. doi:10.1016/j.diamond.2023.109850
- Jing H-P, Wang C-C, Zhang Y-W, et al. Photocatalytic degradation of methylene blue in ZIF-8. RSC Adv. 2014;4(97):54454–54462. doi:10.1039/C4RA08820D
- Sakthivel S, Neppolian B, Shankar M, et al. Solar photocatalytic degradation of azo dye: comparison of photocatalytic efficiency of ZnO and TiO2. Sol Energy Mater Sol Cells. 2003;77(1):65–82. doi:10.1016/S0927-0248(02)00255-6
- Tariq N, Fatima R, Zulfiqar S, et al. Synthesis and characterization of MoO3/CoFe2O4 nanocomposite for photocatalytic applications. Ceram Int. 2020;46(13):21596–21603. doi:10.1016/j.ceramint.2020.05.264
- Jianhua L, Rong Y, Songmei L. Preparation and characterization of the TiO2-V2O5 photocatalyst with visible-light activity. Rare Met. 2006;25(6):636–642. doi:10.1016/S1001-0521(07)60005-9
- Baig MM, Zulfiqar S, Yousuf MA, et al. Structural and photocatalytic properties of new rare earth La3+ substituted MnFe2O4 ferrite nanoparticles. Ceram Int. 2020;46(14):23208–23217. doi:10.1016/j.ceramint.2020.06.103
- Tayebee R, Pejhan A, Ramshini H, et al. Equisetum arvense As an abundant source of silica nanoparticles. SiO2/H3PW12O40 nanohybrid material as an efficient and environmental benign catalyst in the synthesis of 2-amino-4H-chromenes under solvent-free conditions. Appl Organomet Chem. 2018;32(1):e3924. doi:10.1002/aoc.3924
- Zhang L, Mohamed HH, Dillert R, et al. Kinetics and mechanisms of charge transfer processes in photocatalytic systems: a review. J Photochem Photobiol C. 2012;13(4):263–276. doi:10.1016/j.jphotochemrev.2012.07.002
- Kothaplamoottil Sivan S, Padinjareveetil AK, Padil VV, et al. Greener assembling of MoO3 nanoparticles supported on gum arabic: cytotoxic effects and catalytic efficacy towards reduction of p-nitrophenol. Clean Technol Environ Policy. 2019;21(8):1549–1561. doi:10.1007/s10098-019-01726-9
- Bhosale RR, Kulkarni A, Gilda S, et al. Innovative eco-friendly approaches for green synthesis of silver nanoparticles. Int J Pharm Sci Nanotechnol. 2014;7(1):2328–2337. doi:10.37285/ijpsn.2014.7.1.3
- Iravani S, Korbekandi H, Mirmohammadi SV, et al. Synthesis of silver nanoparticles: chemical, physical and biological methods. Res Pharm Sci. 2014;9(6):385.
- Ikram M, Abid N, Haider A, et al. Towards efficient dye degradation and bactericidal behavior of Mo-doped La2O3 nanostructures. Nanoscale Adv. 2022.
- Moeen S, Ikram M, Haider A, et al. Comparative study of sonophotocatalytic, photocatalytic, and catalytic activities of magnesium and chitosan-doped tin oxide quantum dots. ACS Omega. 2022;7(50):46428–46439. doi:10.1021/acsomega.2c05133
- Nadagouda MN, Varma RS. Green synthesis of silver and palladium nanoparticles at room temperature using coffee and tea extract. Green Chem. 2008;10(8):859–862. doi:10.1039/b804703k
- Al-Mussawi AA. Screening for antibacterial activity of twenty two Iraqi wild plants. Global Advanced Research and Journal of Microbiology. 2014;9:177–182.
- Shayegan Mehr E, Sorbiun M, Ramazani A, et al. Plant-mediated synthesis of zinc oxide and copper oxide nanoparticles by using ferulago angulata (schlecht) boiss extract and comparison of their photocatalytic degradation of Rhodamine B (RhB) under visible light irradiation. J Mater Sci: Mater Electron. 2018;29(2):1333–1340. doi:10.1007/s10854-017-8039-3
- Taghavi Fardood S, Ramazani A, Moradi S, et al. Green synthesis of zinc oxide nanoparticles using arabic gum and photocatalytic degradation of direct blue 129 dye under visible light. J Mater Sci: Mater Electron. 2017;28(18):13596–13601. doi:10.1007/s10854-017-7199-5
- Naseem T, Baig MM, Warsi MF, et al. Mesoporous silica prepared via a green route: a comparative study for the removal of crystal violet from wastewater. Mater Res Express. 2020;8(1):015005. doi:10.1088/2053-1591/abd45d
- Chaudhary K, Shaheen N, Zulfiqar S, et al. Binary WO3-ZnO nanostructures supported rGO ternary nanocomposite for visible light driven photocatalytic degradation of methylene blue. Synth Met. 2020;269:116526. doi:10.1016/j.synthmet.2020.116526
- El Shafey AM. Green synthesis of metal and metal oxide nanoparticles from plant leaf extracts and their applications: A review. Green Process Synth. 2020;9(1):304–339. doi:10.1515/gps-2020-0031
- Karpagavalli S, Vethanathan SJK, Perumal S. Effect of nickel doping on the structural, optical, electrochemical and magnetic properties of hausmannite (Mn3O4) nanoparticles. Int J Nanoparticles. 2019;11(4):305–321. doi:10.1504/IJNP.2019.104266
- Veisi H, Karmakar B, Tamoradi T, et al. Bio-inspired synthesis of palladium nanoparticles fabricated magnetic Fe3O4 nanocomposite over Fritillaria imperialis flower extract as an efficient recyclable catalyst for the reduction of nitroarenes. Sci Rep. 2021;11(1):1–15. doi:10.1038/s41598-020-79139-8
- Beheshtkhoo N, Kouhbanani MAJ, Savardashtaki A, et al. Green synthesis of iron oxide nanoparticles by aqueous leaf extract of Daphne mezereum as a novel dye removing material. Appl Phys A. 2018;124(5):1–7. doi:10.1007/s00339-018-1782-3
- Ramesh P, Rajendran A, Meenakshisundaram M. Green syntheis of zinc oxide nanoparticles using flower extract cassia auriculata. J Nanosci Nanotechnol. 2014;2(1):41–45.
- Kaur K, Sidhu AK. Green synthesis: an eco-friendly route for the synthesis of iron oxide nanoparticles. Front Nanotechnol. 2021;3:655062. doi:10.3389/fnano.2021.747329
- Jiang W, Meng L, Zhang S, et al. Enhanced resistive acetone sensing by using hollow spherical composites prepared from MoO3 and In2O3. Microchim Acta. 2019;186(6):1–9. doi:10.1007/s00604-019-3471-0
- Manivel A, Lee G-J, Chen C-Y, et al. Synthesis of MoO3 nanoparticles for azo dye degradation by catalytic ozonation. Mater Res Bull. 2015;62:184–191. doi:10.1016/j.materresbull.2014.11.016
- Song J, Ni X, Zhang D, et al. Fabrication and photoluminescence properties of hexagonal MoO3 rods. Solid State Sci. 2006;8(10):1164–1167. doi:10.1016/j.solidstatesciences.2006.05.002
- Mahajan S, Mujawar S, Shinde P, et al. Concentration dependent structural, optical and electrochromic properties of MoO3 thin films. Int J Electrochem Sci. 2008;3(8):953–960. doi:10.1016/S1452-3981(23)15494-0
- Xue S, Wu C, Pu S, et al. Direct Z-Scheme charge transfer in heterostructured MoO3/g-C3N4 photocatalysts and the generation of active radicals in photocatalytic dye degradations. Environ Pollut. 2019;250:338–345. doi:10.1016/j.envpol.2019.04.010
- Lu M, Shao C, Wang K, et al. p-MoO3 nanostructures/n-TiO2 nanofiber heterojunctions: controlled fabrication and enhanced photocatalytic properties. ACS Appl Mater Interfaces. 2014;6(12):9004–9012. doi:10.1021/am5021155
- Alam U, Kumar S, Bahnemann D, et al. Harvesting visible light with MoO3 nanorods modified by Fe(iii) nanoclusters for effective photocatalytic degradation of organic pollutants. Phys Chem Chem Phys. 2018;20(6):4538–4545. doi:10.1039/C7CP08206A
- Sardar AA, Perveen A, Khan Z. A palynological survey of wetland plants of Punjab, Pakistan. Pak J Bot. 2013;45(6):2131–2140.
- Alebiosu C, Yusuf A. Phytochemical screening, thin-layer chromatographic studies and UV analysis of extracts of Citrullus lanatus. J Pharm Chem Biol Sci. 2015;3(2):214–220.
- Zare M, Namratha K, Alghamdi S, et al. Novel green biomimetic approach for synthesis of ZnO-Ag nanocomposite; antimicrobial activity against food-borne pathogen, biocompatibility and solar photocatalysis. Sci Rep. 2019;9(1):1–15. doi:10.1038/s41598-018-37186-2
- Mamatha K, Ravikumar C, Murthy HA, et al. Facile green synthesis of molybdenum oxide nanoparticles using Centella Asiatica plant: Its photocatalytic and electrochemical lead sensor applications. Sens Int. 2022;3:100153. doi:10.1016/j.sintl.2021.100153
- Thekkethil AJ, Sreekuttan S, Madhavan AA, editors. Application of nano molybdenum trioxide in thermal storage and photocatalysis. In Journal of physics: conference series. IOP Publishing; 2021.
- Al-Otaibi AL. Yttrium doped single-crystalline orthorhombic molybdenum oxide micro-belts: synthesis, structural, optical and photocatalytic properties. J Inorg Organomet Polym Mater. 2021;31(8):3416–3429. doi:10.1007/s10904-021-01999-y
- Khan A, Danish M, Alam U, et al. Facile synthesis of a Z-scheme ZnIn2S4/MoO3 heterojunction with enhanced photocatalytic activity under visible light irradiation. ACS Omega. 2020;5(14):8188–8199. doi:10.1021/acsomega.0c00446
- Yang Z, Du X, Shang Z, et al. Solution combustion synthesis of Bi2Mo3O12 and Bi2Mo3O12/MoO3 composites with enhanced photocatalytic properties. J Electron Mater. 2020;49(9):5346–5352. doi:10.1007/s11664-020-08252-1
- Meo A, Khan M. Pollen morphology as an aid to the identification of Scorzonera (Cichorieae-Compositae) from Pakistan. Pak J Bot. 2004;36(4):701–710.
- Sachdeva R, Schmidt Z, Brill J, et al., editors. Two-phase flow through chokes. In SPE annual technical conference and exhibition. OnePetro; 1986.
- Rigamoto RR, Tyagi AP. Pollen fertility status in coastal plant species of Rotuma Island. South Pac J Nat Appl Sci. 2002;20(1):30–33. doi:10.1071/SP02007
- d'Urville JSCD. Voyage pittoresque autour du monde: résumé général des voyages de découvertes de Magellan, Tasman, Dampier. Tenré; 1835.
- Devi HS, Boda MA, Shah MA, et al. Green synthesis of iron oxide nanoparticles using Platanus orientalis leaf extract for antifungal activity. Green Proc Synth. 2019;8(1):38–45. doi:10.1515/gps-2017-0145
- Hayat MQ, Ashraf M, Khan MA, et al. Palynological study of the Genus Artemisia (Asteraceae) and its systematic implications. Pak J Bot. 2010;42(2):751–763.
- Ahmad M, Bano A, Zafar M, et al. Pollen morphology of some species of the family asteraceae from the alpine zone, Deosai Plateau, northern Pakistan. Palynology. 2013;37(2):189–195. doi:10.1080/01916122.2012.758920
- Ali T, Warsi MF, Zulfiqar S, et al. Green nickel/nickel oxide nanoparticles for prospective antibacterial and environmental remediation applications. Ceram Int. 2022;48(6):8331–8340. doi:10.1016/j.ceramint.2021.12.039
- Baig MM, Hassan M, Ali T, et al. Green 2D simonkolleite/zinc based nanostructures for superior antimicrobial and photocatalytic applications. Mater Chem Phys. 2022;126292.
- Gupta A, Koirala AR, Gupta B, et al. Improved method for separation of silver nanoparticles synthesized using the Nyctanthes arbor-tristis shrub. Acta Chemica Malaysia. 2019;3(1):35–42. doi:10.2478/acmy-2019-0005
- Harriman NA. Flora of Pakistan. Econ Bot. 2004;58(4):742. doi:10.1663/0013-0001(2004)058[0742:BREDFA]2.0.CO;2
- Fish L. Preparing herbarium specimens. National Botanical Institute; 1999.
- Karahan F, Ozyigit II, Saracoglu IA, et al. Heavy metal levels and mineral nutrient status in different parts of various medicinal plants collected from eastern Mediterranean region of Turkey. Biol Trace Elem Res. 2020;197(1):316–329. doi:10.1007/s12011-019-01974-2
- Punt W, Hoen P, Blackmore S, et al. Glossary of pollen and spore terminology. Rev Palaeobot Palynol. 2007;143(1–2):1–81. doi:10.1016/j.revpalbo.2006.06.008
- Ahmed MA, Abou-Gamra ZM, Medien HAA, et al. Effect of porphyrin on photocatalytic activity of TiO2 nanoparticles toward Rhodamine B photodegradation. J Photochem Photobiol, B. 2017;176:25–35. doi:10.1016/j.jphotobiol.2017.09.016
- Hu H, Deng C, Xu J, et al. Metastable h-MoO3 and stable α-MoO3 microstructures: controllable synthesis, growth mechanism and their enhanced photocatalytic activity. J Exp Nanosci. 2015;10(17):1336–1346. doi:10.1080/17458080.2015.1012654
- Ayi AA, Anyama CA, Khare V. On the synthesis of molybdenum nanoparticles under reducing conditions in ionic liquids. J Mater. 2015;2015:1–7.
- Kang J, Kim J, Lee M, et al. Electrochemically synthesized nanoporous molybdenum carbide as a durable electrocatalyst for hydrogen evolution reaction. Adv Sci. 2017;5:1700601. doi:10.1002/advs.201700601
- Su D, Li Y-L, An H-J, et al. Pyrolytic transformation of liquid precursors to shaped bulk ceramics. J Eur Ceram Soc. 2010;30(6):1503–1511. doi:10.1016/j.jeurceramsoc.2009.11.015
- Nasrollahzadeh M, Issaabadi Z, Sajadi SM. Green synthesis of a Cu/MgO nanocomposite by Cassytha filiformis L. extract and investigation of its catalytic activity in the reduction of methylene blue, congo red and nitro compounds in aqueous media. RSC Adv. 2018;8:3723–3735. doi:10.1039/C7RA13491F
- Ali I, Peng C, Ye T, et al. Sorption of cationic malachite green dye on phytogenic magnetic nanoparticles functionalized by 3-marcaptopropanic acid. RSC Adv. 2018;8:8878–8897. doi:10.1039/C8RA00245B
- Max J-J, Blois S, Veilleux A, et al. IR spectroscopy of aqueous alkali halides. Factor analysis. Can J Chem. 2011;79:13–21. doi:10.1139/v00-160
- Wongsa P, Phatikulrungsun P, Prathumthong S. FT-IR characteristics, phenolic profiles and inhibitory potential against digestive enzymes of 25 herbal infusions. Sci Rep. 2022;12(1):6631. doi:10.1038/s41598-022-10669-z
- Baig MM, Zulfiqar S, Yousuf MA, et al. DyxMnFe2-xO4 nanoparticles decorated over mesoporous silica for environmental remediation applications. J Hazard Mater. 2021;402:123526. doi:10.1016/j.jhazmat.2020.123526
- Mori K, Kawashima M, Che M, et al. Enhancement of the photoinduced oxidation activity of a ruthenium (II) complex anchored on silica-coated silver nanoparticles by localized surface plasmon resonance. Angew Chem. 2010;122(46):8780–8783. doi:10.1002/ange.201004942
- Saravanan R, Karthikeyan N, Gupta V, et al. Zno/Ag nanocomposite: an efficient catalyst for degradation studies of textile effluents under visible light. Mater Sci Eng: C. 2013;33(4):2235–2244. doi:10.1016/j.msec.2013.01.046
- Murray WA, Barnes WL. Plasmonic materials. Adv Mater. 2007;19(22):3771–3782. doi:10.1002/adma.200700678
- Zheng Y, Zheng L, Zhan Y, et al. Ag/ZnO heterostructure nanocrystals: synthesis, characterization, and photocatalysis. Inorg Chem. 2007;46(17):6980–6986. doi:10.1021/ic700688f
- Hamza MA, Abd El-Rahman SA, El-Shazly AN, et al. Facile one-pot ultrasonic-assisted synthesis of novel Ag@RGO/g-C3N4 ternary 0D@2D/2D nanocomposite with enhanced synergetic tandem adsorption-photocatalytic degradation of recalcitrant organic dyes: kinetic and mechanistic insights. Mater Res Bull. 2021;142:111386. doi:10.1016/j.materresbull.2021.111386
- Adeel M, Saeed M, Khan I, et al. Synthesis and characterization of Co–ZnO and evaluation of its photocatalytic activity for photodegradation of methyl orange. ACS Omega. 2021;6(2):1426–1435. doi:10.1021/acsomega.0c05092
- Isai KA, Shrivastava VS. Photocatalytic degradation of methylene blue using ZnO and 2% Fe–ZnO semiconductor nanomaterials synthesized by sol–gel method: a comparative study. SN Appl Sci. 2019;1:1–11.
- Kenchappa Somashekharappa K, Lokesh SV. Hydrothermal synthesis of K2Ti6O13 nanotubes/nanoparticles: a photodegradation study on methylene blue and rhodamine B dyes. ACS Omega. 2021;6(11):7248–7256. doi:10.1021/acsomega.0c02087
- Ahmed S, Rasul M, Martens WN, et al. Heterogeneous photocatalytic degradation of phenols in wastewater: a review on current status and developments. Desalination. 2010;261(1-2):3–18. doi:10.1016/j.desal.2010.04.062
- Bendjabeur S, Zouaghi R, Kaabeche O, et al. Parameters affecting adsorption and photocatalytic degradation behavior of gentian violet under UV irradiation with several kinds of TiO2 as a photocatalyst. Int J Chem Reactor Eng. 2017;15(4):20160206. doi:10.1515/ijcre-2016-0206
- Tanaka K, Padermpole K, Hisanaga T. Photocatalytic degradation of commercial azo dyes. Water Res. 2000;34(1):327–333. doi:10.1016/S0043-1354(99)00093-7
- Sanakousar M CCV, Jiménez-Pérez VM, Jayanna B, et al. Efficient photocatalytic degradation of crystal violet dye and electrochemical performance of modified MWCNTs/Cd-ZnO nanoparticles with quantum chemical calculations. J Hazard Mater Adv. 2021;2:100004. doi:10.1016/j.hazadv.2021.100004
- Reddy BP, Rajendar V, Shekar M, et al. Particle size effects on the photocatalytic activity of BiFeO 3 particles. Digest J Nanomater Bios. 2018;13(1).
- Zheng X, Yuan J, Shen J, et al. A carnation-like rGO/Bi 2 O 2 CO 3/BiOCl composite: efficient photocatalyst for the degradation of ciprofloxacin. J Mater Sci: Mater Electron. 2019;30:5986–5994. doi:10.1007/s10854-019-00898-w
- Munir S, Rasheed A, Zulfiqar S, et al. Synthesis, characterization and photocatalytic parameters investigation of a new CuFe2O4/Bi2O3 nanocomposite. Ceram Int. 2020;46(18):29182–29190. doi:10.1016/j.ceramint.2020.08.091
- Touqeer M, Baig MM, Aadil M, et al. New Co-MnO based nanocrsytallite for photocatalysis studies driven by visible light. J Taibah Univ Sci. 2020;14(1):1580–1589. doi:10.1080/16583655.2020.1846966
- Humayoun UB, Mehmood F, Hassan Y, et al. Harnessing bio-immobilized ZnO/CNT/chitosan ternary composite fabric for enhanced photodegradation of a commercial reactive dye. Molecules. 2023;28(18):6461. doi:10.3390/molecules28186461
- Aadil M, Rahman A, Zulfiqar S, et al. Facile synthesis of binary metal substituted copper oxide as a solar light driven photocatalyst and antibacterial substitute. Adv Powder Technol. 2021;32(3):940–950. doi:10.1016/j.apt.2021.01.040
- Nawaz HZR, Falak U, Naz T, et al. Synthesis of silver/silver oxide heterostructures via partial reduction of AgNO3 using a novel green reducing agent. Ceram Int. 2022;48(24):37194–37202. doi:10.1016/j.ceramint.2022.08.296
- Kasirajan P, Kumar S, Pandiaraj S, et al. Controllable synthesis of SnO 2 photocatalyst with superior photocatalytic activity for the degradation of methylene blue dye solution. J Exp Nanosci. 2016;11:1–18. doi:10.1080/17458080.2015.1015461
- Mbagwu F, Chime E, Unamba C. Palynological studies on five species of Asteraceae. Afr J Biotechnol. 2009;8(7).
- Garnatje T, Martin J. Pollen studies in the genus Echinops L. and Xeranthemum group (Asteraceae). Bot J Linn Soc. 2007;154(4):549–557. doi:10.1111/j.1095-8339.2007.00671.x
- Meo A, Khan M. Pollen morphology of Achillea (Compositae-Anthemoideae) species fromPakistan. Pakistan J Weed Sci Res (Pakistan). 2003.
- Dauti A, Kapidani G, Pupuleku B, et al. Palynomorphological data of some species of the genus Achillea. Acad J Interdiscipl Stud. 2017;5(3 S1):74.
- Tomb AS, Larson DA, Skvarla JJ. Pollen morphology and detailed structure of family Compositae, tribe Cichorieae. I. Subtribe Stephanomeriinae. Am J Bot. 1974;61(5):486–498. doi:10.1002/j.1537-2197.1974.tb10788.x
- Abid R, Qaiser M. A micromorphological study for the generic delimitation of Inula L.(s. str.) and its allied genera (Inuleae-Compositae) from Pakistan and Kashmir. Pak J Bot. 2004;36(4):719–724.
- Zafar M, Ahmad M, Khan M. Palynology of family Asteraceae from flora of Rawalpindi-Pakistan. Int J Agr Biol. 2007;9(1):156–161.
- Tellería MC. Spines vs. microspines: an overview of the sculpture exine in selected basal and derived Asteraceae with focus on Asteroideae. J Plant Res. 2017;130(6):1023–1033. doi:10.1007/s10265-017-0956-y
- Qureshi SJ, Awan AG, Khan MA, et al. Study of pollen fertility of the genus Launaea from Pakistan. Asian J Plant Sci. 2002.
- Dematteis M, Pire SM. Pollen morphology of some species of Vernonia sl (Vernonieae, Asteraceae) from Argentina and Paraguay. Grana. 2008;47(2):117–129. doi:10.1080/00173130802151643