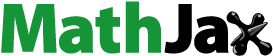
Abstract
Novel push–pull dicyanomethylenedihydrofuran(DCDHF)-based chromophores bearing 6-hydroxyl-2,5,7,8-tetramethylchroman-2-carboxylic acid (HTCX) group were synthesized to function as an antioxidant moiety. The preparation of phenylvinyl-bridged DCDHF chromophores with a hydroxyl functional group to be attached HTCX. Due to intramolecular charge transfer (ICT), HTCX-containing DCDHF chromophores showed a distinct solvatochromism in a range of organic solvents. The molecular structures of the prepared HTCX-bearing DCDHF fluorophores were verified by 1H/13C NMR and FTIR spectroscopic analyses. The emission spectra demonstrated dramatic enhancement in the photostability of the prepared fluorophores. Though, the synthesized HTCX/LSHA(Light Stabilizer Hindered Amine)-DCDHF-based hybrids were studied to evaluated antimicrobial efficiency. HTCX-based hybrid DCDHF-8 revealed sensible activity towards (+ve) Gram and (-ve) Gram bacterial strains, and LSHA-based hybrid DCDHF-15 demonstrated good antibacterial effectiveness against B. cereus (+ve gram) and K. pneumoniae (-ve gram) bactria, comparative to Ampicillin reference. Moreover, the theoretical molecular docking stimulation was realistic to sight see their binding connections towards (PDB-1LNZ).
1. Introduction
Antimicrobial medicines are vital to kill pathogenic microorganisms or stop their growth. However, pathogenic microorganisms can produce resistance to those antimicrobial active agents. Thus, extensive interdisciplinary reports have been presented on the development of innovative potent and competent antimicrobial medicines [Citation1]. The unique operating flexibility of laser dyes has made them important sources of consistent adjustable irradiation [Citation2–4]. Several chromophores have shown a good ability to lase in either gas, liquid, or solid form. Due to the great optical quality that can be attained with active laser media; nonetheless, liquid dyestuffs mixed in appropriate organic solvents are the most widely used laser media [Citation5, Citation6]. The media are self-repairing, and the cooling process can be achieved by a flow system. However, there are drawbacks to use liquid laser dyes, such as the need for huge quantities of organic solvents, which can be both harmful and expensive [Citation7]. Furthermore, multiple dyes are required for different wavelength ranges because of the dye limited tunability range (5-20 nm). Thus, efforts have been made to construct dye lasers to overcome the drawbacks of dye solutions by altering the molecular structures of dyes in the solid state [Citation8–10]. The dangers of toxicity, high cost, and flammability are avoided with solid state laser dyes. As a result, they can be used for a variety of purposes and are simple to operate and maintain. Because of their poor lasing effectiveness and quick photodegradation, widely scattered laser dyes provide undesirable results and should be avoided. Progress was made towards the construction of practically tunable solid state laser dyes due to the discovery of extremely efficient and photostable dyes [Citation11, Citation12].
The use of organic dyestuffs in electro-optics requires optimizing their structures so that they display properties important to the intended usage. In general, the photo- and thermal stability of dyestuffs is an ongoing need for many applications. Thus, a widespread aim of our work is the development of innovative dyestuffs that provide the required level of stability for a certain application [Citation13]. In comparison to many other molecular systems, the dicyanomethylenedihydrofuran(DCDHF)-containing dyestuffs developed for those applications have shown to be very stable. As a result, they have been considered seriously for a variety of uses, including laser dyes and photovoltaics [Citation14, Citation15]. For potential applications in solar cells, and laser dyes, we investigate the synthesis, characterization, antibacterial activity and photophysical characteristics of new donor–acceptor DCDHF-containing fluorophores with a HTCX or hindered amine substituents. It is well-known that both HTCX and hindered amines can improve the photostability of a given chemical system. 1H/13C NMR and FT-IR were used to verify the molecular structures of the produced HTCX or LSHA (Light Stabilizer Hindered Amine)-bearing fluorophores. In the meanwhile, DCDHF and its variants are best recognized as fluorescent probes. Certain compounds, on the other hand, have been shown to have considerable antibacterial activity [Citation16]. This activity’s mechanism might be related to the breakdown of bacterial cell membranes or the suppression of critical bacterial enzymes. HTCX, a water-soluble homologue Its antibacterial effect is due to its capacity to disrupt bacterial oxidative processes or directly damage bacterial cellular structures [Citation17]. This study is aimed to synthesis of new dicyanomethylenedihydrofuran(DCDHF)-based chromophores and confirm their correct structures by 1H/13C NMR and FTIR spectroscopic analyses, evaluate their solvatochromism in different organic solvents, assign their antimicrobial activities against (+ve gram), (-ve gram) bacterial strains in addition to their antifungal activity. Moreover, their molecular docking stimulation is assigned to evaluated their binding interaction with the crystal structure of the GTP-binding protein was obtained from using the Protein Data Bank (PDBID: 1LNZ).
2. Experimental
2.1. Materials and equipment
All chemicals were purchased from commercial suppliers (Merck, Egypt). The intermediate dicyanomethylenedihydrofuran (DCDHF) was synthesized with a yield of 57% utilizing a previously published procedure [Citation18]. Using a TA-2920 differential scanning calorimeter, we determined the melting point of the synthesized dyes. A Bruker Vectra 33 equipped with an ATR Probe was used to report FT-IR spectroscopic data. A HP8453 spectrophotometer was used to record the UV-Visible absorbance spectra. Using a VARIANCARYECLIPSE spectrophotofluorimeter, we were able to report emission spectra. A Joel spectrometer (500 MHz) was used to record NMR spectra of the synthesized fluorophores. The photostability of dyes was determined by the standard ISO(1988)105-B02 methodology using a FADE-OMETER 18-F equipped with an ultraviolet lamp [Citation19]. Compounds 1, 2, 9, 10, 11, 12, 13 and 14 were synthesized according to previously reported procedures [Citation13–16].
2.2. Synthetic approaches
Preparation of HTCX-based fluorophore DCDHF-6
2-(3-cyano-4-(4-(4-(6-hydroxy-2,5,7,8-tetramethylchromane-2-carbonyl)piperazin-1-yl)phenyl)-5,5-dimethylfuran-2(5H)-ylidene)malononitrile
Compound DCDHF-5 (800 mg, 2.22 mmol), HTCX (550 mg, 2.22 mmol), DMAP (12 mg, 0.1 mmol) and DCC (460 mg, 2.22 mmol) were stirred in tetrahydrofuran (anhydrous; 20 mL) for 10 h. The combination was filtered from CH2Cl2 and crystallized from CH3OH to give an orange powder (220 g; 33%). mp 258°C. 1H NMR (CDCl3): 7.73 (d, 2H, Ar-H), 6.94 (d, 2H, Ar-H), 5.88 (s, 1H, O-H), 3.57 (t, 4H, -CH2-N-(CO)CH2-), 3.36-3.30 (m, 4H, -CH2-N-CH2-), 2.75-2.70 (m, 2H, CH2, pyran-H4), 2.40 (s, 3H, Ar-CH3), 2.34-2.31 (m, 1H, CH, pyran-H3), 2.24 (s, 6H, 2Ar-CH3), 2.18-2.12 (m, 1H, CH, pyran-H3), 1.61 (s, 3H, pyran-CH3), 1.23 (s, 6H, furan-C(CH3)2). 13C NMR (CDCl3): 11.93 (Ar-CH3), 13.08 (2Ar-CH3), 22.21 (pyran-CH3), 25.45 (furan-C(CH3)2), 29.64 (pyran-C3), 31.88 (pyran-C4), 46.71 (2C, piperazine), 51.03 (2C, piperazine), 53.41 (furan = C-(CN)2), 87.23 (furan-C5), 89.56 (pyran-C2), 94.78 (furan-C3), 111.16 (2Ar-C), 112.39 (2C≡N), 115.50 (C≡N), 118.65 (Ar-C), 120.57 (Ar-C), 122.19 (Ar-C), 126.58 (Ar-C), 128.33 (Ar-C), 130.72 (2Ar-C), 135.78 (Ar-C), 145.37 (Ar-C), 146.42 (Ar-C), 159.57 (furan-C4), 170.12 (C = O), 183.69 (furan-C2). FTIR (cm−1): 3405 (O-H), 2234 (C≡N), 1732 (C≡O). MS m/z (%): 577 (M+, 37.05). Anal. Calcd. for C34H35N5O4 (577.27): C, 70.69; H, 6.11; N, 12.12%. Found: C, 70.91; H, 6.02; N, 12.25%.
Preparation of HTCX-based fluorophore DCDHF-7
(E)-(1-(4-(2-(4-cyano-5-(dicyanomethylene)-2,2-dimethyl-2,5-dihydrofuran-3-yl)vinyl)phenyl)piperidin-4-yl)methyl 6-hydroxy-2,5,7,8-tetramethylchromane-2-carboxylate
Compound DCDHF-3 (860 mg, 2.22 mmol), HTCX (550 mg, 2.22 mmol), DMAP (12 mg, 0.1 mmol) and DCC (460 mg, 2.22 mmol) were stirred in tetrahydrofuran (anhydrous; 20 mL) for 10 h. The combination was filtered from CH2Cl2 and crystallized from CH3OH to give a purple powder (0.4 g; 25%). mp 248°C. 1H NMR (CDCl3): 7.67 (d, 2H, Ar-H), 6.93 (d, 2H, Ar-H), 6.78 (d, 1H, olefinic CH = C), 6.64 (d, 1H, olefinic CH = C), 6.04 (s, 1H, O-H), 4.41 (d, 2H, CH2-O), 3.18-3.09 (m, 4H, -CH2-N-CH2-), 2.85-2.79 (m, 2H, CH2, pyran-H4), 2.34 (s, 3H, Ar-CH3), 2.29 (m, 1H, CH, pyran-H3), 2.25 (s, 6H, 2Ar-CH3), 2.19-2.16 (m, 1H, CH, pyran-H3), 2.11-2.06 (m, 1H, -CH-, piperidine), 1.61 (s, 3H, pyran-CH3), 1.50-1.43 (m, 4H, -CH2-C-CH2-, piperidine), 1.23 (s, 6H, furan-C(CH3)2). 13C NMR (CDCl3): 11.95 (Ar-CH3), 13.14 (2Ar-CH3), 21.98 (pyran-CH3), 25.38 (furan-C(CH3)2), 27.31 (2C, piperidine-C3), 29.61 (pyran-C3), 31.05 (piperidine-C4), 31.86 (pyran-C4), 52.30 (furan = C-(CN)2), 53.84 (2C, piperidine-C2), 66.17 (-CH2-O), 89.47 (furan-C5), 91.02 (pyran-C2), 97.19 (furan-C3), 111.71 (2Ar-C), 112.54 (2C≡N), 115.48 (C≡N), 118.28 (Ar-C), 120.77 (Ar-C), 121.98 (Ar-C), 124.63 (olefinic-C = C), 126.38 (Ar-C), 128.41 (Ar-C), 130.50 (2Ar-C), 135.60 (Ar-C), 144.57 (olefinic-C = C), 145.39 (Ar-C), 146.52 (Ar-C), 160.15 (furan-C4), 170.08 (C = O), 184.01 (furan-C2). FTIR (neat, cm−1) 3432 (O-H), 2220 (C≡N), 1726 (C≡O). MS m/z (%): 632 (M+, 31.48). Anal. Calcd. for C38H40N4O5 (632.30): C, 72.13; H, 6.37; N, 8.85%. Found: C, 72.31; H, 6.46; N, 9.75%.
Preparation of HTCX-based fluorophore DCDHF-8
(E)-1-(4-(2-(4-cyano-5-(dicyanomethylene)-2,2-dimethyl-2,5-dihydrofuran-3-yl)vinyl)phenyl)piperidin-3-yl 6-hydroxy-2,5,7,8-tetramethylchromane-2-carboxylate
Compound DCDHF-4 (880 mg, 2.22 mmol), HTCX (550 mg, 2.22 mmol), DMAP (12 mg, 0.1 mmol) and DCC (460 mg, 2.22 mmol) were stirred in tetrahydrofuran (anhydrous; 30 mL) for 10 h. The combination was filtered from CH2Cl2 and crystallized from CH3OH to give a purple powder (0.66 g; 47%). mp 210°C. 1H NMR (CDCl3): 7.71 (d, 2H, Ar-H), 6.95 (d, 2H, Ar-H), 6.74 (d, 1H, olefinic CH = C), 6.60 (d, 1H, olefinic CH = C), 6.23 (s, 1H, O-H), 4.70-4.67 (m, 1H, -CH-, piperidine), 3.40-3.33 (m, 4H, -CH2-N-CH2-, piperidine), 2.85-2.80 (m, 2H, CH2, pyran-H4), 2.49-2.47 (m, 1H, CH, pyran-H3), 2.35 (s, 3H, Ar-CH3), 2.25 (s, 6H, 2Ar-CH3), 2.18-2.16 (m, 1H, CH, pyran-H3), 1.63 (s, 3H, pyran-CH3), 1.51-1.43 (m, 4H, -CH2-CH2-, piperidine), 1.23 (s, 6H, furan-C(CH3)2). 13C NMR (CDCl3): 11.88 (Ar-CH3), 13.26 (2Ar-CH3), 19.33 (piperidine-C5), 21.84 (pyran-CH3), 25.35 (furan-C(CH3)2), 27.86 (piperidine-C4), 29.57 (pyran-C3), 32.02 (pyran-C4), 51.09 (piperidine-C6), 53.66 (furan = C-(CN)2), 63.25 (piperidine-C2), 68.47 (piperidine-C3), 89.78 (furan-C5), 90.63 (pyran-C2), 97.21 (furan-C3), 111.97 (2Ar-C), 113.04 (2C≡N), 115.51 (C≡N), 118.13(Ar-C), 120.73 (Ar-C), 122.06 (Ar-C), 124.57 (olefinic-C = C), 126.45 (Ar-C), 128.34 (Ar-C), 130.56 (2Ar-C), 135.72 (Ar-C), 143.88 (olefinic-C = C), 145.40 (Ar-C), 146.60 (Ar-C), 160.30 (furan-C4), 170.15 (C = O), 184.18 (furan-C2). IR (neat, cm−1): 3448 (O-H), 2245 (C≡N), 1713 (C≡O). MS m/z (%): 618 (M+, 24.91). Anal. Calcd. for C37H38N4O5 (618.28): C, 71.83; H, 6.19; N, 9.06%. Found: C, 71.65; H, 6.12; N, 9.20%.
Preparation of LSHA-based fluorophore DCDHF-15
(E)-2-(3-cyano-4-(4-(ethyl(2,2,6,6-tetramethylpiperidin-4-yl)amino)styryl)-5,5-dimethylfuran-2(5H)-ylidene)malononitrile
A combination of compound 14 (1.32 g, 4.8 mmol), acetic acid (290 mg), and DCDHF (0.95 g, 4.8 mmol) in dry pyridine (10 mL) was stirred at room temperature for 10 h. The combination was poured into ice water (50 mL), and filtered under vacuum. The precipitate was crystallized from methanol/chloroform to provide a purple powder (610 mg; 30%). mp 159°C. 1H NMR (CDCl3): 7.61 (d, 2H, Ar-H), 7.17 (d, 1H, olefinic CH = C), 6.93 (d, 1H, olefinic CH = C), 6.80 (d, 2H, Ar-H), 3.35-3.30 (m, 1H, CH, piperidine), 3.18 (q, 2H, -CH2-CH3), 2.84 (s, 1H, N-H), 1.70-1.63 (m, 4H, 2 -CH2-, piperidine), 1.37 (s, 6H, furan-C(CH3)2), 1.23 (s, 12H, 2 -C(CH3)2), 1.12 (t, 3H, -CH2-CH3). 13C NMR (CDCl3): 11.42 (-CH2-CH3), 24.63 (furan-C(CH3)2), 29.39 (4CH3), 43.08 (-CH2-CH3), 44.55 (2C, piperidine-C3), 47.10 (piperidine-C4), 51.58 (furan = C-(CN)2), 52.44 (2C, piperidine-C2), 96.48 (furan-C5), 98.25 (furan-C3), 112.86 (2Ar-C), 113.97 (2C≡N), 115.71 (C≡N), 124.88 (Ar-C), 128.35 (olefinic-C = C), 130.29 (2Ar-C), 132.63 (olefinic-C = C), 146.04 (Ar-C), 163.11 (furan-C4), 183.93 (furan-C2). FTIR (cm−1) 3362 (N-H), 2242 (C≡N), 1583, 1527, 1519 (C = C). MS m/z (%): 469 (M+, 47.15). Anal. Calcd. for C29H35N5O (469.28): C, 74.17; H, 7.51; N, 14.91%. Found: C, 74.32; H, 7.58; N, 15.02%.
Preparation of LSHA-based fluorophore DCDHF-16
2-(3-cyano-4-(4-(ethyl(2,2,6,6-tetramethylpiperidin-4-yl)amino)phenyl)-5,5-dimethylfuran-2(5H)-ylidene)malononitrile
Compound 12 (340 mg, 2 mmol), potassium carbonate (400 mg, 3 mmol) and DCDHF (560 mg, 2 mmol) were charged into DMSO (anhydrous; 40 mL), and stirred at 100°C for 12 h under nitrogen. The combination was cooled, poured into ice water (40 mL), filtered under vacuum, and crystallized from methanol/chloroform to provide an orange powder (650 mg; 75%). mp 277°C. 1H NMR (CDCl3): 7.22 (d, 2H, Ar-H), 6.72 (d, 2H, Ar-H), 3.35-3.31 (m, 1H, CH, piperidine), 3.18 (q, 2H, -CH2-CH3), 2.82 (s, 1H, N-H), 1.70-1.61 (m, 4H, 2 -CH2-, piperidine), 1.39 (s, 6H, furan-C(CH3)2), 1.23 (s, 12H, 2 -C(CH3)2), 1.11 (t, 3H, -CH2-CH3). 13C NMR (CDCl3): 11.37 (-CH2-CH3), 25.26 (furan-C(CH3)2), 29.44 (4CH3), 43.15 (-CH2-CH3), 44.60 (2C, piperidine-C3), 47.31 (piperidine-C4), 51.37 (furan = C-(CN)2), 52.59 (2C, piperidine-C2), 86.51(furan-C5), 94.64 (furan-C3), 112.80 (2Ar-C), 114.05 (2C≡N), 115.74 (C≡N), 126.40 (Ar-C), 130.48 (2Ar-C), 146.16 (Ar-C), 161.22 (furan-C4), 184.05 (furan-C2). FTIR (cm−1) 3333, 2229. MS m/z (%): 443 (M+, 40.76). Anal. Calcd. for C27H33N5O (443.27): C, 73.11; H, 7.50; N, 15.79%. Found: C, 73.14; H, 7.46; N, 15.71%.
2.3. In vitro antimicrobial evaluation
Using the Minimal Inhibitory Concentration (MIC) technique, the antimicrobial effectiveness of HTCX/LSHA-based hybrids was investigated toward diverse bacterial strains: two Gram-positive (+ve) bacteria, Staphylococcus aureus ATCC-6538 (S. aureus) and Streptococcus pneumoniae ATCC-49619 (S. pneumoniae), and two gram-negative (-ve) bacteria, Escherichia coli ATCC-15224 (E. coli) and Klebsiella pneumoniae ATCC-43816 (K. pneumoniae) in comparesion to Ampicillin (Amp.) as standard drug [Citation20, Citation21]. Also, the antifungal activity for prepared HTCX/LSHA-DCDHF-based hybrids was examined against C. albicans and A. fumigatus in contrast to Miconazole as a reference.
2.4. Dihydrofolate reductase inhibition assay
As previously mentioned in the literature [Citation21], a dihydrofolate reductase inhibition activity was carried out to assess the efficacy of the inhibition against bovine DHFR for the studied HTCX/LSHA-based hybrids. The DHFR inhibition (%) was computed using the formula.
In the meantime, the supplementary file contained a statement of the experimental protocol.
2.5. Molecular docking
The M.O.E “2015.10” software was used to examine molecular docking stimulation. The crystal structure of the GTP-binding protein was obtained from using the Protein Data Bank (PDBID: 1LNZ). [Citation22, Citation23] The co-crystallized ligand was re-docked into the chosen GTP binding protein, As the GTPases, are vital ribosome bosses and are essential for the transferal of DNA to descendant cells after cell division [Citation15], is highly preserved As a result, it has been a focus for the development of broad-spectrum antibacterial agents [Citation24]. Also, the molecular docking study was used to download the crystal structures of S. aureus target proteins for docking studies: Dihydropteroate synthetize complexed with OH-CH2-pterin-pyrophosphate (PDB entry code 1AD4) [Citation25], dihydrofolate reductase complexed with unique HTCX/LSHA-based hybrids. The protein structures were imported, and the protein was synthesized using the protein production wizard. After removing all heteroatoms except water, docking ligand atoms in the correct places via 10 poses, minimization, and docking the ligands, this was done to calculate the root-mean-square values.
3. Results and discussion
3.1. Syntheses and chemistry
Several conjugated materials for electro-optics included the strongly electron-withdrawing DCDHF group. Using a previously published method, cyanoacetonitrile and 3-hydroxy-3-methylbutan-2-one were condensed to produce DCDHF with a yield of around 57% [Citation13, Citation14, Citation17]. The HTCX or LSHA-bearing fluorophores were designed to improve their photostability. The hydroxyl-substituted phenylvinyl-bridged dyestuffs were synthesized in waterless pyridine using Knoevenagel condensation reaction. The DCDHF heterocycle’s active methyl substituent was subjected to Knoevenagel condensation reaction with aryl-aldehyde’s para-position secondary heterocyclic amine. In contrast to aryl-aldehyde with tertiary acyclic amine, the reaction rate and yield were found to be higher for those having tertiary heterocyclic amine at the para-position of the aryl-aldehyde, indicating superior electrophilicity. As shown in Scheme 1, Compound (1) was synthesized by combining DMSO and purified water. High yields (81-87%, respectively) were achieved during the synthesis of phenyl-bridged fluorophores (DCDHF-3 and DCDHF-4).
For the synthesis of phenyl-containing fluorophore (DCDHF-5), DCDHF is directly linked to a phenyl ring. The intermediate chemical (A) might be used as a key substrate in the production of numerous ethenyl-free DCDHF-based dyestuffs. As shown in Scheme 2, the matching chromophore (DCDHF-5) can be obtained from compound (A) by performing a straightforward aromatic nucleophilic substitution in anhydrous pydridine (B). The yield dye (DCDHF-5) containing phenyl was 63%. The strong cyano groups in the DCDHF moiety are responsible for the enhanced reactive characteristics of (A). An efficient condensation procedure between malononitrile with adduct (A) and the aromatic hydroxyketone at room temperature has been previously reported, and the intermediate (A) has been isolated and characterized.
Since HTCX is already purified for commercial uses, it was used in its unrefined form. As indicated in Scheme 3, reacting HTCX with 2° amine or hydroxyl substituted fluorophores in the presence of DMAP and DCC in anhydrous THF at room temperature yielded the corresponding amide or ester containing fluorophores, respectively. Due to the HTCX moiety’s hindered carboxylic group, only low yields of the HTCX-substituted fluorophores (DCDHF-6), (DCDHF-7), and (DCDHF-8) could be achieved. Reference model fluorophores (DCDHF-9), (DCDHF-10), and (DCDHF-11) were synthesized from compounds (DCDHF-5), (DCDHF-3), and (DCDHF-4) by a reaction with trimethylacetyl chloride in pyridine at room temperature to afford ester or amide based fluorophores, demonstrating the photostability of the produced HTCX fluorophores (DCDHF-6), (DCDHF-7), and (DCDHF-8).
LSHA, N-methyl(or butyl)-2,2,6,6-tetramethylpiperidin-4-amine (12) and (13), was prepared from the reaction of cyclic ketone with alkylamine in methanol followed by a reduction process in the presence of NaBH3CN. After bringing the mash up to a pH of 12 with saturated NH4Cl, extracting it with Et2O, and then purifying it using Kugelrohr distillation, compounds (12) and (13) were obtained as colourless oil. The matching phenyaldehyde-bearing LSHA was produced by reacting the 2° amine-based LSHA with 4-fluorobenzaldehyde and K2CO3 in anhydrous dimethylsulfoxide at 100 °C. Due to the steric hindrance of the secondary amine, it has been obtained from that only phenylaldehyde bearing LSHA (R = CH3) is produced. Chromophores (DCDHF-15) and (DCDHF-16) were formed, as shown in Scheme 4, according to the characterization procedures.
3.2. Photophysical properties
New electroactive molecular systems have been synthesized. Donor and acceptor topologies in nonlinear optical materials have been the primary focus of research into strong acceptors like DCDHF heterocycle. By creating pushpull intramolecular interactions, this strong electron acceptor can be connected to electron donating fragments throughout an aromatic π-bridge, hence increasing the emission wavelength and extension coefficient of the resulting molecule. New DCDHF fluorophores, both with and without a vinyl group, were synthesized, and their photophysical properties are investigated. An aryl-aldehyde was used as the electron donor, while DCDHF was used as the electron acceptor, in a process known as Knoevenagel condensation. The nonlinear optical properties of such DCDHF-based dyestuffs were very varied. Because of their strong dipole moment, high absorbance coefficient, polarizability, and easy tenability, they are quite important. In addition, they are distinguished by the fact that they can produce varying amounts of energy through a variety of π-bridges and donating groups.
Figure and Table display the emission spectra and extension coefficient of DCDHF fluorophores. The photostability and other qualities useful for single-molecule imaging have been greatly improved by the inclusion of DCDHF in dyes. The fluorescence spectra in the UV–visible range showed that increasing the electron donor concentration in a DCDHF reaction increased the ICT, resulting in longer wavelengths of fluorescence. In general, the fluorescence spectra of DCDHF-containing dyes were affected in various ways according to their structural diversity. Sharp fluorescence peaks at longer wavelengths and a greater attenuation coefficient were seen in the phenyl-bridged DCDHF-containing dyes comprising ester and amide groups. Dimeric aggregation in dyes (DCDHF-3), (DCDHF-4), and (DCDHF-5) resulted in an ultraviolet and visible fluorescence shoulder at lower wavelengths. Because of the broadened fluorescence band, this shoulder was not seen in fluorophores (DCDHF-3) and (DCDHF-4) with a hydroxyl group on the donor 2° amine. In an effort to provide improved photostability for various applications, it has been found useful to covalently connect the antibacterials HTCX or LSHA to DCDHF fluorophores. The materials with a single pivalate substitution have been made as standards. The degree to which donor nitrogen is alkylated has been a significant impact on the thermal stability of azo dyes with nitro acceptors [Citation13–16]. We further expect that the large donor groups will serve to stabilize these DCDHF dyes not only photochemically but also against heat degradation. Inhibition of dimerization is a potential side effect of the large donor group provided by tetramethylpiperidine. The synthesized fluorophores, including DCDHF-3, DCDHF-4, DCDHF-5, DCDHF-6, DCDHF-7, DCDHF-8, DCDHF-15 and DCDHF-16, displayed different values of light fastness, including 4-5, 4, 4, 6, 6-7, 6-7, 6-7 and 6, respectively. When compared to the reference dyes, the photostability of the synthesized LSHA/HTCX -bearing dyes were much greater. This demonstrated that the inclusion of LSHA or HTCX into the molecular structure of fluorophores was productive. However, the LSHA-containing dyes were seen to have greater photostability than the HTCX -containing dyes.
Table 1. Photophysical parameters of DCDHF fluorophores.
3.3. Antibacterial assay
The antibacterial activity of five distinct HTCX /LSHA-based hybrids was tested counter to four bacterial strains: two (+ve) Gram-bacteria (S. aureus and B. cereus) and two (-ve) Gram-bacteria (K. pneumoniae and E. coli). Ampicillin (Amp) was used as a standard control to assess the hybrids’ antibacterial efficacy (Table ). For a complete understanding, the findings and their consequences are reviewed together in this section. According to (+ve) Gram-bacteria, HTCX -based hybrid 6 had low antibacterial efficacy against both S. aureus and S. pneumoniae, with MIC values of 8.47± 0.52 µg/mL and 7.09±0.24 µg/mL, respectively. Meanwhile, HTCX-based hybrid DCDHF-7’s MIC value against S. aureus was 9.21±0.16 µg/mL, which is less effective than ampicillin (5.31±0.36 µg/mL). The higher the MIC value, the lesser the antibacterial activity. However, HTCX-based hybrid DCDHF-7 had modest efficacy against S. pneumonia, with a MIC value of 5.40±0.10 µg/mL. Temporarily, HTCX-based DCDHF-8 had shown significant action against S. aureus and S. pneumonia towards with MIC values of 5.87±0.41 µg/mL and 4.65±0.21 µg/mL, respectively. Alternatively, LSHA-based hybrid DCDHF-15 exhibited excellent antibacterial activity against S. aureus, with a MIC value of 6.14±0.28 µg/mL. When it came to S. pneumoniae, LSHA-based hybrid DCDHF-15 exhibited a much higher MIC value (8.78±0.43 µg/mL) than ampicillin (2.44±0.19 µg/mL), indicating its comparatively weaker antibacterial activity against this reference. In contrast, LSHA-based hybrid DCDHF-16 had a MIC of 8.05±0.27µg/mL against S. aureus, which is lower value than the control ampicillin, and a somewhat superior MIC of 5.11±0.36 µg/mL against S. pneumoniae.
Table 2. MIC results for the synthesized HTCX /LSHA-based hybrids.
Table 3. Binding energy (ΔG) and the percentage DHFR inhibition of the synthesized compounds against DHFR.
On the another hand, (-ve) Gram-bacteria seemed to be less vulnerable to the tested hybrids than ampicillin among the Gram-negative bacteria examined. MIC = 3.72±0.08 µg/mL for HTCX-based hybrid DCDHF-6: The MIC value of this hybrid compound against E. coli is even lower than that of the control, ampicillin (MIC = 2.43±0.33 µg/mL). The low standard deviation indicates extremely consistent performance. These data suggest that HTCX-based hybrid DCDHF-6 is a promising choice for future investigation into E. coli as (-ve) Gram-bacterial infections. Consequently, HTCX-based hybrid DCDHF-6 displayed poor effectiveness against K. pneumoniae with MIC = 6.18±0.61 µg/mL. Meanwhile, HTCX-based hybrid DCDHF-7 (MIC = 9.05±0.46 µg/mL): This hybrid has a comparatively high MIC value, suggesting reduced potency against E. coli when compared to other hybrids, particularly ampicillin. Given its very poor effectiveness, it may not be a suitable choice for treating E. coli infections in spite of it revealed acceptable effectiveness against K. pneumoniae with MIC = 4.68±0.12 µg/mL. Nonetheless, HTCX-based hybrid DCDHF-8 (MIC = 4.16±0.19 µg/mL and 3.28±0.54 µg/mL) against both of E.coli and K. pneumoniae, respectively: HTCX-based hybrid DCDHF-8 has significant antibacterial action as well; making it a contender for future research. Despite this, Hybrid DCDHF-15 (MIC = 8.01±0.22 µg/mL): LSHA-based hybrid DCDHF-15 seems to be less suited for treating E. coli since its MIC value = 5.44±0.30 µg/mL against K. pneumoniae it seems reasonable effectiveness. Furthermore, LSHA-based hybrid DCDHF-16 with (MIC = 6.28±0.56 µg/mL): This chemical has modest antibacterial action against E. coli but is displayed excellent antibacterial activity with MIC = 3.05±0.38 µg/mL against K. pneumoniae, suggesting superb antibacterial performance against this strain. Meanwhile, the antifungal potency of the hybrids was compared using probably inhibition zones (in µg/mL). Hybrid DCDHF-6 exhibited notable antifungal activity against both C. albicans (36.28±0.30) and A. fumigatus (27.13±0.26). Its activity against C. albicans is stronger than that of the standard miconazole (26.19±0.34). Hybrid DCDHF-7 Presented significant activity against C. albicans (39.71±0.34) and seems to surpass the activity of miconazole. No data is available for its activity against A. fumigatus, which might suggest that its efficacy against this fungus is negligible or hasn’t been tested. Hybrid DCDHF-8 demonstrated the highest antifungal activity among all hybrids against C. albicans (45.05±0.20). Furthermore, it also displays potent activity against A. fumigatus (41.61±0.11), which is noticeably higher than that of miconazole (23.27±0.29). This indicates the potential of Hybrid 8 as a broad-spectrum antifungal agent. Hybrid DCDHF-15 displayed moderate activity against C. albicans (30.93±0.17). Again, no data is present for its activity against A. fumigatus, pointing to possible non-efficacy or lack of testing. Hybrid DCDHF-16 offered the least activity against C. albicans (17.49±0.52) among all hybrids but demonstrates reasonable efficacy against A. fumigatus (29.67±0.04), even surpassing the standard miconazole. This suggests that its antifungal spectrum might lean more towards A. fumigatus.
3.4. Structure Activity Relationship (SAR)
On the other hand, by examining the relationship between the structural features of these hybrids and their corresponding antibacterial activity, we can discern the essential features that confer this activity. The antibacterial activity of the compounds against different bacterial strains is numerically presented. For this discussion, lower numerical values represent stronger antibacterial activity since they would most likely represent minimum inhibitory concentrations (MICs) or a similar measure. Hybrid with hydroxy and diazepane Ring DCDHF-6 exhibits strong activity against (+ve gram) bacteria such as S. aureus and S. pneumoniae as compared to (-ve Gram) bacteria K. pneumoniae and E. coli [Citation26, Citation27]. The diazepane ring might be influencing specificity against (+ve gram) strains. Meanwhile, hybrid with 4-substituted piperidine, hydroxy, carboxylate DCDHF-7, and 3-substituted piperidine, hydroxy, carboxylate DCDHF-8. Hybrid DCDHF-7shows potent activity against both (+ve gram) and (-ve gram) strains, with particularly high activity against E. coli [Citation28]. While Compound DCDHF-8 shows moderate activity across all strains, suggesting that the position of substitution on the piperidine ring has an influence on antibacterial activity, the hydroxyl and carboxylate functional groups seem to be contributing positively, especially when positioned at the 4th position of the piperidine ring.[Citation15, Citation29] Moreover, hybrid with vinyl group DCDHF-15 Displays strong activity against S. pneumoniae (+ve gram) and notable activity against E. coli (-ve Gram). The vinyl group could enhance the hybrid’s permeability or target binding across the bacterial cell walls. Furthermore, hybrid DCDHF-16 (without vinyl group) exhibits moderate activity across all strains. Its activity profile suggests that the unspecified structural component(s) in this hybrid might be universally recognized by bacterial targets or affect permeability. In conclusion, the presence of a diazepane ring confers specificity towards Gram-positive strains. While the piperidine ring, when substituted at different positions, influences antibacterial activity, with the 4th position substitution being more favourable
3.5. Dihydrofolate reductase inhibition assay
All of the HTCX/LSHA-based hybrids were established for inhibition activity against bovine DHFR, the percentage inhibition values were calculated in triplicate and the average inhibitory value of each compound is presented in table . Trimethoprim was used as a standard DHFR inhibitor. The results (mean ± standard error of the mean (SEM)) demonstrate DCDHF-15 exhibited a 74.2 ± 0.03% inhibition, whereas the standard drug (trimethoprim as the reference DHFR inhibitor) showed a 74.6 ± 0.09% inhibition.
3.6. Molecular Docking Analysis
The Molecular Operating Environment (M.OE. 2015.10) programme was used to analyse the molecular interactions of a variety of HTCX-based hybrids in this investigation. The target protein’s active site was docked with a panel of five HTCX/LSHA-based hybrids, numbered DCDHF-6, DCDHF-7, DCDHF-8, DCDHF-15, DCDHF-16, and the reference compound Amp., to assess their binding affinities, kinds of interactions, and root mean square deviations (RMSD). Meanwhile, the energy scores of the HTCX -based hybrids ranged from S=−6.0980 Kcal/mol to S=−7.4828 Kcal/mol, and RMSD values ranged from 0.9326 to 1.3932, i.e., < 1.5, presenting a good acceptable amount of binding interactions. HTCX-based hybrid DCDHF-8 has the greatest binding affinity (S=−7.4828 Kcal/mol), followed by HTCX -based hybrid DCDHF-7 (S=−7.4378 Kcal/mol). The binding affinity of the reference compound, Amp, was S=−6.0980 Kcal/mol, indicating that some of the studied HTCX-based hybrids may have better binding than the reference molecule. The docked HTCX -based hybrids had RMSD values ranging from 0.9326 to1.3932 refining units. Lower RMSD values indicate greater docking dependability, and LSHA-based hybrid DCDHF-15 exhibited the lowest RMSD (0.9326), indicating a more stable contact with the target protein (Table ).
Table 4. In silico docking results of the prepared HTCX / LSHA-based fluorophores.
In light of its binding affinity, consider the HTCX-based hybrid DCDHF-6: The low energy value of S=−6.8490 Kcal/mol indicates a decreased binding affinity. However, the RMSD at 1.3760 demonstrated a significant range with observed π-cation interaction with Lys 283. Integrated Perspective: The low binding affinity and increased RMSD show that, although HTCX -based hybrid DCDHF-6 can create stable contacts. The interaction with Lys 283 may provide opportunities to improve the compound’s binding affinity (Figure ).
Temporarily, HTCX-based hybrid DCDHF-7 had a high energy score of S=−7.4378 Kcal/mol, suggesting greater binding due to a comparatively large RMSD of 1.3932, although the complicated interaction profile might explain this due to a multi-point interaction including Lys 283, Ser 168, and Asp 265. The interactions are H-donor, π-H, and H-acceptor, in that order. Despite its greater RMSD, the HTCX-based hybrid DCDHF-7 is a tempting candidate for future exploration due to its high energy score and complicated interaction profile. The compound’s wide collection of interactions may make it more resistant to mutations in the target 1LNZ protein (Figure ).
HTCX -based hybrid DCDHF-8 had the greatest energy score of S=−7.4828 Kcal/mol with a low RMSD (1.2588), indicating a stable conformation through an H-acceptor bond with Lys 283 at a distance of 3.17 Å. While HTCX -based hybrid DCDHF-8 has the greatest binding affinity, its single-point interaction raises questions regarding selectivity and resilience to target protein mutations (Figure ).
In the meantime, the LSHA-based hybrid DCDHF-15 displayed a lower energy score of S=−6.3608 Kcal/mol, as well as the lowest RMSD of 0.9326, indicating the most stable interaction. It forms a hybrid-like π-H interaction with Asp 253. Despite the reduced binding affinity, the very low RMSD indicates a persistent, although weaker, binding to the target. It might act as a framework for further chemical changes targeted at improving binding affinity (Figure ).
Additionally, via dual H-acceptor contacts with Leu 249 and Lys 283, with distances of 3.17 and 3.44, respectively, LSHA-based hybrid DCDHF-16 demonstrated moderate binding affinity score value S= −6.3886 Kcal/mol over good RMSD at 1.3898. Because of its lower energy score and greater RMSD, LSHA-based hybrid DCDHF-16 is less appealing than others such as HTCX -based hybrid DCDHF-7 or HTCX -based hybrid DCDHF-8. However, the dual H-acceptor interactions show that there is opportunity for improvement in terms of binding affinity (Figure ).
Furthermore, the reference Amp displayed an energy score of S=−6.0980 Kcal/mol and interacts via H-acceptor bonds with Val 169 and Arg 252. Given that some of the investigated hybrids show higher energy scores, Amp assists as a baseline for estimating the relative effectiveness of the other compounds. The H-acceptor bindings observed also provide a reference for the types of interactions that might be valuable for binding (Figure ).
In spired the data of the molecular docking investigation against (PDB: 1AD4) as a representative for crystal structures of the target proteins of S. Aureus, the analyze compounds have binding energy, root mean square deviation (RMSD), interaction with the protein, types of bindings, and intramolecular distances. The hybrids (DCDHF-6, DCDHF-7, DCDHF-8, DCDHF-15, DCDHF-16) and Trimethoprim as a reference (Table and Figures ).
Table 5. In silico docking results of the prepared HTCX / LSHA-based against PDB: 1AD4.
DCDHF-6 exhibited a binding energy of −7.4279 kcal/mol and an RMSD of 1.3113. This suggests a moderately strong and relatively stable interaction with the target.
While, DCDHF-7 showed a binding energy of S=−7.7671 kcal/mol and an RMSD of 1.8342, indicating a stronger binding affinity than DCDHF-6 but with less conformational stability. Meanwhile, DCDHF-8 had the highest binding energy of S=−8.2840 Kcal/mol and an RMSD of 1.7609, suggesting the strongest binding among the tested hybrids. However, DCDHF-15 demonstrated a binding energy of S=−6.5121 kcal/mol and an RMSD of 1.8228, indicating weaker binding compared to other DCDHF compounds. Whereas, DCDHF-16 revealed a binding energy of S=−6.8618 kcal/mol and the lowest RMSD of 1.1503, suggesting a relatively stable but weaker binding.
Nevertheless, Trimethoprim (reference) showed a binding energy of S=−6.2360 kcal/mol and an RMSD of 1.2012, providing a baseline for comparison with DCDHF compounds. Furthermore, all DCDHF hybrids primarily interacted through hydrogen acceptor and π-H interactions, which are crucial for binding affinity. Notably, DCDHF-6 and DCDHF-7 interacted with Arg 204 and Arg 219, suggesting a common binding site. The interaction distancesof all DCDHF hybrids ranged from 2.97 Å to 4.04 Å. In accordance to closer interaction distances, as seen in DCDHF-16 (2.99, 3.65, 3.21 Å), suggest stronger interactions at the molecular level. The molecular docking results reveal distinct binding profiles for each compound. DCDHF-8, with the highest binding energy and moderate RMSD, may offer the most promising therapeutic profile, balancing binding affinity with conformational stability.
However, the higher RMSD values in compounds like DCDHF-7 and DCDHF-15 could indicate more flexible interactions, which might be advantageous or disadvantageous depending on the dynamic nature of the target site. The consistent interaction with Arg 204 across several hybrids suggests this residue plays a serious role in binding.
The presence of hydrogen acceptor interactions in all DCDHF compounds indicates a common binding mechanism, potentially offering a structural motif for further optimization. In comparison to Trimethoprim, the DCDHF compounds generally exhibit stronger binding energies, suggesting they might be more effective inhibitors, assuming the target protein’s function is inhibited by these interactions. However, the different interaction types (H-door in Trimethoprim versus H-acceptor and π-H in DCDHF compounds) highlight the necessity of understanding the functional implications of these interactions.
Overall, these results suggest that the DCDHF series, particularly DCDHF-8, have significant potential as inhibitors, with strong binding affinities and specific interactions that could be optimized for enhanced therapeutic efficacy. Future studies should investigate the dynamic nature of these interactions and the functional implications of binding at these specific sites.
Finally, molecular docking was introduced through a snapshot of potential bindings, and the validation of the docking process by measurement of RMSD with experimental data like enzyme inhibition assays and binding kinetics was carried out through some previous studies [Citation30]. Likewise, the docking protocol’s parameters and the prime of the scoring purpose can also affect the reliability of the consequences.
4. Conclusions
We have synthesized, characterized, and structurally optimized new pushpull fluorophores with DCDHF and LSHA/HTCX agents. Multiple analytical methods (FTIR, 1H/13C NMR spectra) validated the molecular structures of the synthesized dyes. The arylaldehyde-DCDHF heterocycle knovenagel reaction was used to create the phenylvinyl-bridged dyes. The corresponding vinyl-bridged DCDHF fluorophores are produced when the intermediate containing the DCDHF moiety connected to the 4-fluorophenyl bridge reacts through aromatic nucleophilic substitution with unrestricted 2° amines. However, due to steric hindrance, LSHA-bearing 2° amine with substituents other than methyl was not able to be produced. High extinction coefficients were found for phenylvinyl bridged fluorophores, which accounted for the vast bulk of the produced DCDHF dyestuffs. Dyes containing HTCX/LSHA were reported to have excellent photostability. However, the antibacterial effectiveness of the synthesised HTCX/LSHA-DCDHF-based hybrids was investigated. In comparison to Ampicillin, HTCX-based hybrid DCDHF-8 displayed decent action against (+ve) Gram and (-ve) Gram bacterial strains, while LSHA-based hybrid DCDHF-15 demonstrated excellent antibacterial efficiency against B. cereus (+ve gram) and Klebsiella pneumoniae (-ve gram) bacteria. Despite significant differences in their interaction patterns and RMSD values, molecular docking stimulation revealed that HTCX-based hybrids DCDHF-7 and DCDHF-8 are especially promising owing to their high energy scores. The intricate multi-point interactions of HTCX-based hybrid DCDHF-7 make it an appealing option for future investigation, since this might suggest increased specificity. HTCX-based hybrid DCDHF-8, on the other hand, with the greatest energy score but a single-point interaction, presents a contrasting binding profile that is also worth investigating.
Consent to publish
The authors agree to publish the article under the Creative Commons Attribution License.
Availability of data and materials
All relevant data are within the manuscript and available from the corresponding author upon request.
Supplemental Material
Download MS Word (2.2 MB)Disclosure statement
No potential conflict of interest was reported by the author(s).
References
- Pervaiz M, Sadiq S, Sadiq A, et al. Azo-Schiff base derivatives of transition metal complexes as antimicrobial agents. Coord Chem Rev. 2021;447:214128, doi:10.1016/j.ccr.2021.214128
- Su L, Shu L, Shi B, et al. Construction of enhanced photostability anthraquinone-type nanovesicles based on a novel two-step supramolecular assembly strategy and their application on multiband laser-responsive composites. ACS Appl Mater Interfaces. 2021;13:43458–43472. doi:10.1021/acsami.1c14352
- Uriel C, Gómez AM, García Martínez de la Hidalga E, et al. Access to 2,6-dipropargylated BODIPYs as “clickable” congeners of pyrromethene-567dye: photostability and synthetic versatility. Org Lett. 2021;23(17):6801–6806. doi:10.1021/acs.orglett.1c02380
- Avellanal-Zaballa E, Gartzia-Rivero L, Arbeloa T, et al. Fundamental photophysical concepts and key structural factors for the design of BODIPY-based tunable lasers. Int Rev Phys Chem. 2022;41(2):177–203. doi:10.1080/0144235X.2022.2096772
- Jiang G, Ren TB, D’Este E, et al. A synergistic strategy to develop photostable and bright dyes with long Stokes shift for nanoscopy. Nat Commu. 2022;13(1):2264, doi:10.1038/s41467-022-29547-3
- Ševčík J, Urbánek P, Hanulíková B, et al. The photostability of novel boron hydride blue emitters in solution and polystyrene matrix. Mater. 2021;14(3):589, doi:10.3390/ma14030589
- Adamow A, Sznitko L, Chrzumnicka E, et al. The ultra-photostable and electrically modulated stimulated emission in perylene-based dye doped liquid crystal. Sci Rep. 2019;9(1):2143, doi:10.1038/s41598-019-38484-z
- Ayare NN, Ramugade SH, Sekar N. Photostable coumarin containing azo dyes with multifunctional property. Dyes pigm. 2019;163:692–699. doi:10.1016/j.dyepig.2018.12.050
- Lewis-Thompson I, Zhang S, Noimark S, et al. PDMS composites with photostable NIR dyes for multi-modal ultrasound imaging. MRS Adv. 2022;7:499–503. doi:10.1557/s43580-022-00208-3
- Sayresmith N A, Saminathan A, Sailer J K, et al. Photostable voltage-sensitive dyes based on simple, solvatofluorochromic, asymmetric thiazolothiazoles. J Amer Chem Soc. 2019;141(47):18780–18790. doi:10.1021/jacs.9b08959
- Bera A, Bagchi D, Pal SK. Improvement of photostability and NIR activity of cyanine dye through nanohybrid formation: key information from ultrafast dynamical studies. J Phys Chem. 2019;123(35):7550–7557. doi:10.1021/acs.jpca.9b04100
- Prieto-Castañeda A, Avellanal-Zaballa E, Gartzia-Rivero L, et al. Tailoring the molecular skeleton of Aza-BODIPYs to design photostable red-light-emitting laser dyes. Chem Photo Chem. 2019;3(2):75–85. doi:10.1002/cptc.201800225
- Aldalbahi A, Periyasami G, Alrehaili A. Synthesis of high molar extinction coefficient push–pull tricyanofuran-based disperse dyes: biological activity and dyeing performance. New J Chem. 2021;45(4):2208–2216. doi:10.1039/D0NJ05510G
- Abualnaja KM, Abu-Saied M, Ghareeb RY, et al. Synthesis, antimicrobial and photostability of novel push–pull tricyanofuran dyes bearing HTCX and hindered amine. J Mater Res Technol. 2021;14:452–463. doi:10.1016/j.jmrt.2021.06.082
- Albalawi MA. Molecular docking of novel donor–π–acceptor dicyanomethylenedihydrofuran-based fluorescent chromophores. Lumines. 2023;38(5):546–553. doi:10.1002/bio.4478
- Alsaeedi H. Synthesis, characterization, antibacterial activity, and molecular docking of novel dicyanodihydrofuran-based push–pull fluorophores. Lumines. 2023;38(5):527–535. doi:10.1002/bio.4477
- Kozłowska M, Ścibisz I, Przybył JL, et al. Antioxidant and antibacterial activity of extracts from selected plant material. Appl Sci. 2022;12(19):9871, doi:10.3390/app12199871
- Al-Azmi A, John E. Synthesis and characterization of novel tricyanofuran hydrazone probe: solvatochromism, density-functional theory calculation and selective fluorescence, and colorimetric determination of iron (III). Luminesc. 2021;36:1220–1230. doi:10.1002/bio.4047
- Mostafa AM, Mwafy EA. Synthesis of ZnO and Au@ ZnO core/shell nano-catalysts by pulsed laser ablation in different liquid media. J Mater Res Technol. 2020;9(3):3241–3248. doi:10.1016/j.jmrt.2020.01.071
- Fu Y, Zhang F, Zhang W, et al. Differential expression of blaSHV related to susceptibility to ampicillin in Klebsiella pneumoniae. Int J Antimicrob Agents. 2007;29(3):344–347. doi:10.1016/j.ijantimicag.2006.10.015
- Hammouda MM, Gaffer HE, Elattar KM. Insights into the medicinal chemistry of heterocycles integrated with a pyrazolo[1,5-a]pyrimidine scaffold. RSC Med Chem. 2022;13:1150–1196. doi:10.1039/D2MD00192F
- Buglino J, Shen V, Hakimian P, et al. Structural and biochemical analysis of the Obg GTP binding protein. Struct. 2002;10(11):1581–1592. doi:10.1016/S0969-2126(02)00882-1
- Pinzi L, Rastelli G. Molecular docking: shifting paradigms in drug discovery. Int J Mol Sci. 2019;20(18):4331, doi:10.3390/ijms20184331
- Casiraghi A, Suigo L, Valoti E, et al. Targeting bacterial cell division: a binding site-centered approach to the most promising inhibitors of the essential protein FtsZ. Antibiotics. 2020;9:69, doi:10.3390/antibiotics9020069
- Jays J, Mohan S, Saravanan J. Molecular docking studies of some novel furan derivatives as potent inhibitors of staphylococcus aureus. Inter J Pharmal Res. 2019;11(1):200, doi:10.31838/ijpr/2019.11.01.025
- Uddin MN, Ahmed SS, Alam SR. Biomedical applications of Schiff base metal complexes. J Coord Chem. 2020;73(23):3109–3149. doi:10.1080/00958972.2020.1854745
- Deep A, Kaur Bhatia R, Kaur R, et al. Imidazo[1,2-a] pyridine scaffold as prospective therapeutic agents. Curr Top Med Chem. 2017;17(2):238–250.
- Zala AR, Rajani DP, Kumari P. Design, synthesis, molecular docking and in silico ADMET investigations of novel piperidine-bearing cinnamic acid hybrids as potent antimicrobial agents. J Iran Chem Soci. 2023;20:1843, doi:10.1007/s13738-023-02801-1
- Saxena M, Saxena J, Nema R, et al. Phytochemistry of medicinal plants. J pharmacogn phytochem. 2013;1(6):168–182.
- Al-Sha’er MA, Mansi I, Hakooz N, et al. Docking and pharmacophore mapping of halogenated pyridinium derivatives on heat shock protein 90. J Chem Pharma Res. 2015;7:103–112.