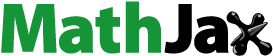
Abstract
In this research, the influence of hirsutidin on mice with alcoholic liver disease was examined. A total of 30 mice were randomly allocated to five groups (n = 6): Normal control group, Ethanol (EtOH) control group, hirsutidin-10, hirsutidin-20, and hirsutidin-20 per se for 4-week. Furthermore, 1 h after the last dosage of the treatment, 5 mL/kg (i.p.) EtOH (56%) was injected to cause hepatic injury. After 5 h of EtOH administration, blood samples were taken, and then the liver was excised for biochemical analysis. Hirsutidin treatment restored the biochemical changes induced by EtOH: serum levels of liver function tests, lipid profile, blood urea nitrogen, antioxidants levels, malondialdehyde, and cytokines. Hirsutidin supplementation also significantly decreased the histopathological abnormalities in EtOH-induced mice. In the present study, hirsutidin was found to have therapeutic potential for the treatment of alcoholic liver disease. Hirsutidin prevented ethanol-induced biochemical and histopathological alterations in the liver, suggesting its hepatoprotective effect.
1. Introduction
Globally, a common ailment is known as alcoholic liver disease (ALD), which denotes defects in the liver triggered by too much alcohol consumption [Citation1,Citation2]. The key contributing factors to ALD include inflammation, cytokine damage, lipid peroxidation damage, and excessive development of reactive oxygen species (ROS) [Citation3]. Due to a rise in the occurrence of ALD, a serious threat to human health has emerged recently. Excessive alcohol intake is linked to several disorders, including ALD [Citation1,Citation4]. ALD encompasses liver illnesses such as alcoholic steatosis (accretion of fat in hepatocytes), alcoholic hepatitis (tenderness of hepatocytes), and alcoholic cirrhosis (hepatocyte impairment and scar formation) [Citation5,Citation6]. The liver serves as the primary location of ethanol (EtOH) breakdown [Citation7]. Numerous important processes that are necessary for digestion, storage, absorption, and detoxification are carried out by the liver [Citation8]. Alcohol itself or the influence of its metabolites may cause pathophysiologic changes in cellular function as a result of excessive alcohol use [Citation8]. ALD is associated with considerable morbidity and mortality, According to World Health Organization (WHO) estimation in 2018, every year three million people die worldwide because of alcohol consumption [Citation7], accounting for nearly 500,000 fatalities per year caused by ADL [Citation9].
The liver primarily contains both oxidative and non-oxidative processes for alcohol metabolism [Citation7,Citation10]. The main process for metabolizing alcohol is the oxidative pathway, in which alcohol dehydrogenase (ADH) changes alcohol into acetaldehyde [Citation11]. Acetaldehyde is the primary metabolite of EtOH that harms the liver [Citation10]. Aldehyde dehydrogenase (ALDH) then quickly converts acetaldehyde into acetate and excretes it from the liver [Citation7]. Acetate breaks down into carbon dioxide, fatty acids, and water in marginal tissues. When drinking excessively, the cytochrome P450 2E1 enzyme (CYP2E1) expresses and functions more quickly than ADH. ROS produced by the activated CYP2E1 converts alcohol to acetaldehyde [Citation7,Citation12].
Alcohol and acetaldehyde can stimulate the cellular resistant system in the liver and encourage the creation and secretion of tumour necrosis factor-alpha (TNF-α) [Citation13]. TNF-α and nuclear factor kappa B (NF-κB) amplify the incendiary reaction, create additional oxidative damage, and ultimately culminate in alcohol-induced liver damage [Citation14,Citation15]. ROS are significantly involved in a variety of apparently irrelevant hepatopathies, according to a broad array of experimental evidence [Citation16]. While enzymatic and nonenzymatic defence mechanisms protect the antioxidant/oxidant status. Oxidative stress, a metabolic imbalance brought on by an excess of ROS production or a decreased antioxidant capacity, overwhelms these defensive mechanisms [Citation17]. The fundamental reason how ROS are detrimental for cells is that they damage lipids, proteins, and nucleic acids, which results in structural and functional defects [Citation16]. To strengthen the antioxidant defence mechanisms and resist the effects of ROS, numerous therapies have been proposed [Citation18].
ALD has a complicated aetiology, and it is yet unknown what molecular processes underlie its development. Inflammation and oxidative damage were created to be major contributors to the formation of ALD, and drugs having anti-oxidative or antiinflammatory properties were found to slow the growth of ALD in animal models [Citation19]. ALD prevention and treatment are important public health issues everywhere in the world [Citation9,Citation20,Citation21]. Rodents are the most used animal paradigms for ALD investigation. EtOH challenge in rodents can imitate some characteristics of human ALD and help identify the mechanism(s) involved in the progression and screening of the drugs for the prevention and treatment of ALD [Citation4,Citation22]. Anthocyanins are polyphenols that contribute to the colour of flowers, vegetables, and fruits [Citation23]. Anthocyanin flavonoid colourants are abundant in nature and have been shown to exhibit antioxidant effects [Citation24]. This ability is hypothesized to be a defence mechanism besides a wide range of ailments induced by ROS [Citation24]. Nutritional consumption of anthocyanin-rich foods has been reported to possess beneficial effects on eye health [Citation25], cardiovascular health [Citation26,Citation27], bacterial infection [Citation28], cancer [Citation29], obesity [Citation30,Citation31], diabetes [Citation31], and neurotoxicity [Citation32].
Hirsutidin is an O-methylated anthocyanidin (Figure ), and it is a part of the anthocyanin family. It can be discovered in Catharanthus roseus (Madagascar periwinkle), where it is the most abundant chemical in the petals, as well as in callus cultures [Citation33]. According to the assessment of the literature, there were only few studies reported on hirsutidin [Citation34–39]. The current investigation aimed to examine the hepatoprotective effect of hirsutidin against EtOH-induced liver injury in a mice model.
2. Methodology
2.1. Chemicals
Biochemical kits for serum albumin, blood urea nitrogen (BUN), creatinine, direct bilirubin alanine aminotransferase (MSW-ALT), aspartate aminotransferase (AST), alkaline phosphatase (MSW-ALP), total cholesterol (MSW-TC) and triglyceride (MSW-TG) were obtained from MSW Pharma, Maharashtra, India. The levels of A commercial enzyme-linked immunosorbent assay kit was employed to produce the analytical kits for interleukin-1β (IL-1β, KLU0012), IL-6 (KLM6306), interferon-gamma (IFN-γ, KB2011) and tumor necrosis factor-α (TNF-α, KB2145) were determined using rat enzyme-linked immunosorbent assay (ELISA) kits from Krishgen Biosystems, India. IL-12 (MSW-IL-12) was obtained from MSW Pharma, Maharashtra, India.
2.2. Experiment
Male mice were employed in the investigation. Before being used in research, all of the mice were acclimatized to laboratory conditions. The institutional animal ethical committee approved the study procedure (CPCSEA/IAEC/918/01) and followed the ARRIVE guideline.
A total of 30 mice were randomly assigned to five groups, each containing six animals (n = 6):
For four weeks, each of these treatments was administered orally. Furthermore, 1 h after the final oral dose, mice in the normal group were given 5 mL/kg (i.p.) of normal saline, and mice in the EtOH, hirsutidin-10, and hirsutidin-20 groups were given 5 mL/kg of EtOH (56%) to cause liver injury [Citation9,Citation40].
Blood samples were obtained after 5 h of EtOH treatment and were collected from the retro-orbital plexus of each rat under anesthesia. and serum was separated and used for biochemical analysis. The livers of mice from all groups were then collected for further research.
2.3. Liver function tests
The levels of serum albumin, BUN, creatinine, direct bilirubin, ALP, AST, and ALT using a biochemical test kit following the manufacturer's guidelines.
2.4. Serum lipid profile
The amounts of TG, TC, high-density lipoprotein (HDL) using a biochemical test kit following the manufacturer's guidelines.
2.5. Inflammatory cytokine
The amounts of serum interleukins (IL-1β, IL-6, IL-12), TNF-α, and IFN-γ using a biochemical test kit following the manufacturer's guidelines. Protein content was determined using the Bradford assay according to the manufacturer’s instructions.
2.6. Biochemical examination of liver tissue
2.6.1. Tissue homogenate preparation
In ice-cold conditions, liver tissue was washed with isotonic saline and homogenized in phosphate buffer (0.1 M, pH 7.4). The supernatant was biochemically analyzed [Citation41].
2.6.2. Endogenous antioxidants
The quantity of reduced glutathione (MSW-GSH, MSW Pharma, India) was determined by employing the Ellman technique [Citation42]. Superoxide dismutase (MSW-SOD, MSW Pharma, India) was dignified employing the Misra and Frodvich procedure [Citation43]. To measure catalase (MSW-CAT, MSW Pharma, India) activity, 0.1 ml of supernatant was added to 1.9 ml phosphate buffer (pH 7.0, 50 mM) in the cuvette. 1.0 ml of freshly prepared H2O2 (30 mM) was added to start the reaction [Citation44,Citation45].
2.6.3. Malondialdehyde
The amount of malondialdehyde (MSW-MDA, MSW Pharma, India) in liver samples was determined by employing the Wills technique. After the MDA's interaction with the thiobarbituric acid, the MDA concentration was assessed at 532 nm. The quantity of MDA was intended as nanomoles per milligram of protein [Citation46,Citation47].
2.6.4. Histology investigation of liver tissue
To assess the pathological modifications to the liver, liver tissues were secured in 10% buffered formalin for 24 h, immersed in paraffin, cut into 5-µm thick pieces, and coloured with hematoxylin and eosin (H and E). To examine the ultrastructural alterations of the liver tissues using a light microscope (Olympus LS, India).
2.6.5. Statistical analysis
All the statistics are presented in mean ± SEM, GraphPad Prism software (Version 8.0.2) was employed to examine the data. One-way analysis of variance (ANOVA) followed by Tukey’s test, a P value less than 0.05 was measured as statistically remarkable.
3. Results
3.1. Liver function tests
3.1.1. Albumin, BUN, creatinine, and direct bilirubin
EtOH induced abnormality in liver function and it was indicated by altered liver function parameters in alcohol-administered mice associated with the normal control. EtOH-treated animals showed reduced albumin and abruptly increased levels of BUN, creatinin and direct bilirubin when compared to the normal control mice. One-way ANOVA followed by Tukey’s test demonstrated that hirsutidin at the dosage of 10 and 20 mg/kg b.w. to EtOH ingested mice restored the albumin levels [F (4, 25) = 13.41, P < 0.0001] and attenuated the levels of BUN [F (4, 25) = 20.82, P < 0.0001], creatinine [F (4, 25) = 7.061, P = 0.0006] and direct bilirubin [F (4, 25) = 40.97, P < 0.0001]. Hirsutidin per se (20 mg/kg b.w.) did not show any significant changes. The impact of hirsutidin on serum levels of albumin, BUN, creatinine, and direct bilirubin is shown in Figure .
3.1.2. AST, ALT, and ALP
One-way ANOVA followed by Tukey’s test revealed that EtOH resulted in increased intensities of AST, ALT and ALP in the serum of mice associated to normal control Hirsutidin mitigated the amounts of AST [F (4, 25) = 10.52, P < 0.0001], ALT [F (4, 25) = 8.748, P = 0.0001] and ALP [F (4, 25) = 5.853, P = 0.0018] when associated to EtOH cluster. Hirsutidin per se (20 mg/kg b.w.) did not show any changes (Figure ).
3.2. Serum lipid profile
The serum lipid profile levels were disturbed in EtOH-treated mice, TC and TG were increased and HDL was decreased in comparison to normal control. One-way ANOVA followed by Tukey’s test revealed that hirsutidin (10 and 20 mg/kg) to EtOH-treated mice attenuated TC [F (4, 25) = 10.57, P < 0.0001] and TG [F (4, 25) = 10.61, P < 0.0001] and improved the HDL [F (4, 25) = 4.777, P = 0.0053] levels. Hirsutidin per se (20 mg/kg b.w.) did not show any significant changes (Figure ).
3.3. Proinflammatory cytokines
Alcohol treatment elevated the IL-1β, IL-6, IL-12, TNF-α, and IFN-γ in mice when associated with the normal control. One-way ANOVA followed by Tukey’s test demonstrated that hirsutidin (10 and 20 mg/kg) decreased IL-1β [F (4, 25) = 12.78, P < 0.0001], IL-6 [F (4, 25) = 8.145, P = 0.0002], IL-12 [F (4, 25) = 19.54, P < 0.0001], TNF-α [F (4, 25) = 9.569, P < 0.0001] and IFN-γ [F (4, 25) = 10.30, P < 0.0001] in EtOH-treated mice. No remarkable alterations were observed in the hirsutidin per se (20 mg/kg) cluster. The effect of hirsutidin on proinflammatory cytokines are shown in Figures and .
3.4. Endogenous antioxidants
SOD, GSH, and CAT were depleted in alcohol-treated mice vs normal. One-way ANOVA followed by Tukey’s test shows that Hirsutidin (10 and 20 mg/kg b.w.) restored the SOD [F (4, 25) = 6.211, P = 0.0013], GSH [F (4, 25) = 4.671, P = 0.0059] and CAT [F (4, 25) = 4.715, P = 0.0057] activities in EtOH-treated mice liver. Hirsutidin per se (20 mg/kg) did not show any changes (Figure ).
3.5. MDA
The MDA quantity was elevated (P < 0.001) in EtOH-given animals when associated with the normal. The increased MDA was found to be more than double in EtOH-treated mice. One-way ANOVA followed by Tukey’s test demonstrated that hirsutidin attenuated the levels of MDA [F (4, 25) = 5.357, P = 0.0030] vs EtOH group. No changes were detected in hirsutidin per se (Figure ).
3.6. Histology investigation of liver tissue
The hepatocytes and principal vein in the liver of normal control and hirsutidin per se group display normalarchitecture. The amounts of Hirsutidin augmented control mice depict hepatocytes with normal lobular architecture. Hepatocytes in the liver of mice given EtOH exhibit modifications such as inflammatory cell infiltration, micro-, and macrovesicular fatty abnormalities, erosion of the portal triad, and severe degenerative to necrotic lesions. Hirsutidin (10 and 20 mg/kg b.w.) supplementation considerably decreased the pathological abnormalities in EtOH-induced mice. The EtOH group showed a significant increase in hepatocellular necrosis which was significantly reduced by hirsutidin (10 and 20 mg/kg b.w.). The microscopic photos of liver tissues and necrotic area are shown in Figure (A–F).
Figure 9. (A–E) Effect of hirsutidin on histological changes in all the groups. Liver sections stained with hematoxylin and eosin (H&E X200, scale bar = 100 μm). A. Normal group, normal architecture indicated by black arrow B. Ethanol control group, severe degenerative to necrotic lesions indicatd by black arrow, C. hirsutidin (10 mg/kg) minor abnormalities indicated by black arrow, D. hirsutidin (20 mg/kg) restored to normal indicated by black arrow, E. hirsutidin Per se, normal architecture indicated by black arrow F. The percentage of necrotic area in mice liver samples, #P < 0.001 vs normal and **P < 0.001, ***P < 0.0001 vs EtOH.

4. Discussion
In the present study, hirsutidin was investigated primarily for its effect on alcohol- prompted ALD in mice, as well as amounts of antioxidant enzymes, cytokines, and histopathological changes.
Heavy EtOH consumption can lead to several hepatic conditions, the maximum predominant of which are cirrhosis, hepatitis, and fatty liver (steatosis) [Citation1]. Fatty liver is the first and most typical response that occurs in more than 90% of drinkers [Citation1]. Clinically, AST and ALT activity are considered to be sensitive indications of hepatotoxicity [Citation48]. In this investigation, therapy with hirsutidin reduced histological lesions and alcohol-induced increases in ALT and AST enzymatic activity.
ALP, AST, and ALT are all enzymes that are found in the liver, other organ tissues, and serum. When injuries or illnesses impair the body's tissues, transaminases are quickly released into the bloodstream [Citation9,Citation49]. According to the study's findings, which are by those of earlier studies, the serum quantities of AST, ALT, and ALP were considerably elevated in the mouse paradigm of an alcoholic liver grievance [Citation50]. The current research report is in agreement with the above findings, EtOH increased the AST, ALT, and ALP in mice. Moreover, EtOH depleted the amounts of albumin and increased BUN, creatinine, and direct bilirubin. Treatment with hirsutidin attenuated the increased AST, ALT, ALP, BUN, creatinine, and direct bilirubin and restored the levels of albumin in EtOH-treated mice. This indicates the beneficial effect of hirsutidin against EtOH-induced alteration in the liver enzyme. Cirrhosis-related severe metabolic impairment can exacerbate the serum lipoprotein pattern. This protection against oxidative stress likely contributes to the observed hirsutidin reduction in liver enzyme levels (AST, ALT, and ALP), BUN, and bilirubin levels, as these molecules are released from damaged liver cells.
Hepatic microsomes, which metabolize plasma lipids and lipoproteins, are where the majority of endogenous cholesterol is created [Citation51]. Chronic liver illness impairs cholesterol synthesis and metabolism, resulting in altered plasma lipid levels [Citation51]. In the present study, TC, and TG were significantly increased and drastically decreased HDL was seen in EtOH-treated mice. On the other hand, hirsutidin attenuated the increased TC and TG and elevated HDL in EtOH-treated mice. Furthermore, hirsutidin exhibits hypolipidemic effects, resulting in decreased levels of TC and TG. These fat-like substances, reduce fat accumulation in the liver and alleviate liver stress. These findings suggest that hirsutidin shields mice against EtOH-induced dyslipidemia.
As mentioned above ADH predominantly converts EtOH to acetaldehyde. The EtOH and acetaldehyde are direct hepatotoxins, that increase ROS generation [Citation52]. Moreover, EtOH breakdown via CYP2E1 not only creates acetaldehyde but also ROS [Citation53,Citation54]. A strong antioxidant defence system may be able to neutralize these ROS. SOD, GSH, and CAT are the three primary enzymes in a healthy human cell's anti-oxidative and anti-inflammatory defensive mechanism. This anti-oxidant defence mechanism can successfully eliminate free radicals and stop peroxide-mediated damage [Citation55]. However, oxidative damage, particularly in the liver, happens when EtOH consumption surpasses that of the body's scavenging ability [Citation3]. MDA, a precursor of membrane lipid peroxidation, can alter membrane penetrability and cause several physiological and biochemical events, some of which may result in serious liver cell necrosis[Citation3]. MDA concentration can be used to gauge the degree of cell damage. Chronic alcohol use interferes with the antioxidant defence mechanism [Citation52]. The increased ROS and decreased antioxidant defence increase oxidative stress which damages the liver [Citation52,Citation53]. The results of the study revealed a protective effect of hirsutidin, which decreased MDA levels and restored SOD, GSH, and CAT activity against EtOH-prompted oxidative pressure and liver injury in mice in the current investigation. Hirsutidin has demonstrated the potential to modulate various physiological processes through its antioxidant and anti-inflammatory properties. This hypothetical mechanism proposes that hirsutidin exerts its effects by positively increasing the levels of GSH, SOD, and CAT, which are antioxidants that play a crucial role in protecting cells from damage caused by free radicals. In addition to its effects on lipids, hirsutidin has also been observed to exert protective effects against oxidative stress-induced cell death. By reducing the level of MDA, a marker of cell death, hirsutidin supports cell viability and limits the damage caused by oxidative stress. This mechanism provides a possible explanation for the observed anti-inflammatory activity of hirsutidin.
According to earlier research, alcohol intake triggered innate immune responses, which in turn produced inflammatory markers (TNF-α, IFN-γ, IL-6, IL-12, and IL-1β) that led to liver damage [Citation3,Citation56]. As a result of increased oxidative stress, cytokine synthesis is stimulated, which can worsen the inflammatory response in alcoholic liver disease[Citation52,Citation57]. Pro-inflammatory cytokines have a significant role in different stages of liver illness, having an impact on fundamental processes such as acute-phase protein synthesis, lipid metabolism, cholestasis, and grade of fibrosis [Citation57]. The present research well supports the above findings, alcohol treatment elevated the TNF-α, IL-1β, IL-6, IL-12, and IFN-γ in mice compared to the normal. Indicating the elevated inflammatory response in the treated mice, administration of hirsutidin to alcohol-treated mice attenuated TNF-α, IL-1β, IL-6, IL-12, and IFN-γ levels. This demonstrates the hirsutidin's shielding effect against EtOH-prompted inflammatory mediators in animals. However, enhancing antioxidant defense, hirsutidin has also been observed to exert inhibitory effects on the production of pro-inflammatory cytokines. By reducing the levels of TNF-α, IL-1β, IL-6, IL-12, and IFN-γ, hirsutidin helps regulate the immune system and prevent excessive inflammation that contributes to liver damage.
Histopathological observation of liver tissue displays normal architecture of hepatocytes, the liver of mice induced with EtOH exhibits inflammatory cell infiltration, micro- and macrovesicular fatty abnormalities, and erosion of the portal triad. Hirsutidin supplementation significantly decreased the pathological abnormalities in EtOH-induced mice. The biochemical and histopathological study report of the present research indicates the shielding effect of Hirsutidin against the EtOH convinced hepatic toxicity in mice. As a result, there has been a lot of scientific interest in screening of chemicals for the therapy of ALD. There are numerous studies on the use of conventional remedies for the cure of ALD and traditional natural medicine has a long history and a variety of sources [Citation3,Citation58,Citation59]. The current study demonstrates that hirsutidin, a natural substance, has the potential to offer protection against EtOH-induced liver damage.
According to the results, hirsutidin at both doses might produce hepatoprotective effects on mice by lessen the inflammatory response brought on by oxidative stress. It is hypothesized that hirsutidin exerts its effects by increasing antioxidant defense mechanisms, reducing pro-inflammatory cytokine production, and modulating lipid metabolism. Hirsutidin has multiple functions that make it potentially therapeutic for various conditions associated with oxidative stress and inflammation. The clinical use of hirsutidin may have a strong scientific foundation according to this investigation.
The short period and few animals employed in this study are a limitation. Future research will be employed to better understand and confirm the mechanism of hirsutidin, along with other mechanistic cellular and antioxidant markers.
5. Conclusion
In conclusion, hirsutidin attenuates EtOH-induced abnormality in liver function test parameters such as AST, ALT, ALP, BUN, creatinine, direct bilirubin reduced dyslipidemia, reduced oxidative stress and reinstated the endogenous antioxidants and attenuated proinflammatory cytokines levels in mice. These findings suggest that hirsutidin may protect mice from developing alcoholic liver damage. Its antioxidant potential may be responsible for the impact that was noticed.
Authors’ contributions
Conceptualization, methodology, first draft of manuscript, and Critical Revision of manuscript: Fahad A. Al-Abbasi.
Availability of data and materials
All the data generated in this study have been included in the manuscript.
Consent for publication
The author approved and consented to the publication.
Ethics approval and consent to participate
The animal experiments were approved by the Institutional Ethical Committee of Animals (CPCSEA/IAEC/918/01) and in accordance with ARRIVE guideline.
Graphical abstract.tif
Download TIFF Image (179.9 KB)Disclosure statement
No potential conflict of interest was reported by the author.
References
- Osna NA, Donohue TM, Jr, Kharbanda KK. Alcoholic liver disease: pathogenesis and current Management. Alcohol Res Curr Rev. 2017;38:147–161.
- Subramaniyan V, Chakravarthi S, Jegasothy R, et al. Alcohol-associated liver disease: a review on its pathophysiology, diagnosis and drug therapy. Toxicol Rep. 2021;8:376–385. doi:https://doi.org/10.1016/j.toxrep.2021.02.010.
- Li X-X, Jiang Z-H, Zhou B, et al. Hepatoprotective effect of gastrodin against alcohol-induced liver injury in mice. J Physiol Biochem. 2019;75:29–37. doi:10.1007/s13105-018-0647-8
- Ghosh Dastidar S, Warner JB, Warner DR, et al. Rodent models of alcoholic liver disease: role of binge ethanol administration. Biomolecules. 2018;8:3. doi:10.3390/biom8010003
- Buzzetti E, Kalafateli M, Thorburn D, et al. Pharmacological interventions for alcoholic liver disease (alcohol-related liver disease): an attempted network meta-analysis. Cochrane Database Syst Rev. 2017;3:CD011646–CD011646. doi:10.1002/14651858.CD011646.pub2
- Theise ND. Histopathology of alcoholic liver disease. Clin Liver Dis. 2013;2:64–67. doi:10.1002/cld.172
- Hyun J, Han J, Lee C, et al. Pathophysiological aspects of alcohol metabolism in the liver. Int J Mol Sci. 2021;22:5717. doi:10.3390/ijms22115717
- Mani V, Siddique AI, Arivalagan S, et al. Zingerone ameliorates hepatic and renal damage in alcohol-induced toxicity in experimental rats. Int J Nutr Pharmacol Neurol Dis. 2016;6:125. doi:10.4103/2231-0738.184585
- Chen L, Liu L, Abdulla R, et al. Chemical components and hepatoprotective mechanism of xwak granule in mice treated with acute alcohol. Evid Based Complement Alternat Med. 2020;2020:8538474. doi:10.1155/2020/8538474
- Cederbaum AI. Alcohol metabolism. Clin Liver Dis. 2012;16:667–685. doi:10.1016/j.cld.2012.08.002
- Zakhari S. Overview: how is alcohol metabolized by the body? Alcohol Res Health. 2006;29:245–254.
- Harjumäki R, Pridgeon CS, Ingelman-Sundberg M. Cyp2e1 in alcoholic and non-alcoholic liver injury. roles of ROS, reactive intermediates and lipid overload. Int J Mol Sci. 2021;22:8221. doi:10.3390/ijms22158221
- Park JH, Lee DH, Park MS, et al. C-C chemokine receptor type 5 deficiency exacerbates alcoholic fatty liver disease through pro-inflammatory cytokines and chemokines-induced hepatic inflammation. J Gastroenterol Hepatol. 2017;32:1258–1264. doi:https://doi.org/10.1111/jgh.13657.
- Sun L, Wen S, Li Q, et al. L-theanine relieves acute alcoholic liver injury by regulating the TNF-α/NF-κB signaling pathway in C57BL/6J mice. J Funct Foods. 2021;86:104699. doi:https://doi.org/10.1016/j.jff.2021.104699.
- Li S, Tan H-Y, Wang N, et al. The role of oxidative stress and antioxidants in liver diseases. Int J Mol Sci. 2015;16:26087–26124. doi:10.3390/ijms161125942
- Fan G, Tang JJ, Bhadauria M, et al. Resveratrol ameliorates carbon tetrachloride-induced acute liver injury in mice. Environ Toxicol Pharmacol. 2009;28:350–356. doi:10.1016/j.etap.2009.05.013
- Nita M, Grzybowski A. The role of the reactive oxygen species and oxidative stress in the pathomechanism of the age-related ocular diseases and other pathologies of the anterior and posterior eye segments in adults. Oxid Med Cell Longev. 2016;2016:3164734. doi:10.1155/2016/3164734
- Snezhkina AV, Kudryavtseva AV, Kardymon OL, et al. ROS generation and antioxidant defense systems in normal and malignant cells. Oxid Med Cell Longev. 2019;2019:6175804. doi:10.1155/2019/6175804
- Yang YM, Cho YE, Hwang S. Crosstalk between oxidative stress and inflammatory liver injury in the pathogenesis of alcoholic liver disease. Int J Mol Sci. 2022;23:774. doi:10.3390/ijms23020774
- Askgaard G, Kjær MS, Tolstrup JS. Opportunities to prevent alcoholic liver cirrhosis in high-risk populations: a systematic review with meta-analysis. Am J Gastroenterol. 2019;114:221–232. doi:10.1038/s41395-018-0282-6
- Rodriguez WE, Wahlang B, Wang Y, et al. Phosphodiesterase 4 inhibition as a therapeutic target for alcoholic liver disease: from bedside to bench. Hepatology. 2019;70:1958–1971. doi:10.1002/hep.30761
- Bao Y-l, Wang L, Pan H-t, et al. Animal and organoid models of liver fibrosis. Front Physiol. 2021;12. doi:10.3389/fphys.2021.666138
- Alappat B, Alappat J. Anthocyanin pigments: beyond aesthetics. Molecules. 2020;25:5500. doi:10.3390/molecules25235500
- Tena N, Martín J, Asuero AG. State of the art of anthocyanins: antioxidant activity, sources, bioavailability, and therapeutic effect in human health. Antioxidants. 2020;9:451. doi:10.3390/antiox9050451
- Nomi Y, Iwasaki-Kurashige K, Matsumoto H. Therapeutic effects of anthocyanins for vision and eye health. Molecules. 2019;24:3311. doi:10.3390/molecules24183311
- Reis JF, Monteiro VVS, de Souza Gomes R, et al. Action mechanism and cardiovascular effect of anthocyanins: a systematic review of animal and human studies. J Transl Med. 2016;14:315–315. doi:10.1186/s12967-016-1076-5
- Wallace TC. Anthocyanins in cardiovascular disease. Adv Nutr. 2011;2:1–7. doi:10.3945/an.110.000042
- Sun X-h, Zhou T-t, Wei C-h, et al. Antibacterial effect and mechanism of anthocyanin rich Chinese wild blueberry extract on various foodborne pathogens. Food Control. 2018;94:155–161. doi:https://doi.org/10.1016/j.foodcont.2018.07.012.
- Lin B-W, Gong C-C, Song H-F, et al. Effects of anthocyanins on the prevention and treatment of cancer. Br J Pharmacol. 2017;174:1226–1243. doi:10.1111/bph.13627
- Xie L, Su H, Sun C, et al. Recent advances in understanding the anti-obesity activity of anthocyanins and their biosynthesis in microorganisms. Trends Food Sci Technol. 2018;72:13–24. doi:10.1016/j.tifs.2017.12.002
- Azzini E, Giacometti J, Russo GL. Antiobesity effects of anthocyanins in preclinical and clinical studies. Oxidative Med Cell Longevity. 2017;2017:2740364. doi:10.1155/2017/2740364
- Zhang J, Wu J, Liu F, et al. Neuroprotective effects of anthocyanins and its major component cyanidin-3-O-glucoside (C3G) in the central nervous system: an outlined review. Eur J Pharmacol. 2019;858:172500. doi:10.1016/j.ejphar.2019.172500
- Toki K, Saito N, Irie Y, et al. 7-O-Methylated anthocyanidin glycosides from catharanthus roseus. Phytochemistry. 2008;69:1215–1219. doi:10.1016/j.phytochem.2007.11.005
- Filippini R, Caniato R, Piovan A, et al. Production of anthocyanins by catharanthus roseus. Fitoterapia. 2003;74:62–67. doi:10.1016/S0367-326X(02)00296-4
- Levy LF, Robinson R. CCCLXXIX.—experiments on the synthesis of anthocyanins. Part XIII. 5-β-grlucosidyl-and 5-lactosidyl-hirsutidin chlorides. J Chem Soc. 1931:2738–2742. doi:10.1039/JR9310002738
- Bradley W, Robinson R, Schwarzenbach G. CVII.—A synthesis of pyrylium salts of anthocyanidin type. Part XIX. A synthesis of delphinidin chloride not involving a demethylation process, and syntheses of hirsutidin chloride and of delphinidin chloride 3′-methyl ether, possibly identical with petunidin chloride. J Chem Soc. 1930: 793–817. doi:10.1039/JR9300000793
- Shahid Nadeem M, Khan JA, Al-Abbasi FA, et al. Protective effect of hirsutidin against rotenone-induced parkinsonism via inhibition of caspase-3/interleukins-6 and 1β. ACS Omega. 2023;8:13016–13025. doi:10.1021/acsomega.3c00201
- Imam F, Kothiyal P, Alshehri S, et al. Hirsutidin prevents cisplatin-evoked renal toxicity by reducing oxidative stress/inflammation and restoring the endogenous enzymatic and non-enzymatic level. Biomedicines. 2023;11; doi:10.3390/biomedicines11030804
- Alharbi KS, Al-Abbasi FA, Alzarea SI, et al. Effects of the anthocyanin hirsutidin on gastric ulcers: improved healing through antioxidant mechanisms. J Nat Prod. 2022;85:2406–2412. doi:10.1021/acs.jnatprod.2c00620
- Wang S, Sui S, Liu Z, et al. Protective roles of hepatic gamma-aminobutyric acid signaling in acute ethanol exposure-induced liver injury. J Appl Toxicol. 2018;38:341–350. doi:10.1002/jat.3544
- Nagarjun S, Dhadde SB, Veerapur VP, et al. Ameliorative effect of chromium-d-phenylalanine complex on indomethacin-induced inflammatory bowel disease in rats. Biomed Pharmacother. 2017;89:1061–1066. doi:10.1016/j.biopha.2017.02.042
- Ellman GL. Tissue sulfhydryl groups. Arch Biochem Biophys. 1959;82:70–77. doi:10.1016/0003-9861(59)90090-6
- Misra HP, Fridovich I. The role of superoxide anion in the autoxidation of epinephrine and a simple assay for superoxide dismutase. J Biol Chem. 1972;247:3170–3175. doi:10.1016/S0021-9258(19)45228-9
- Aebi H, Wyss SR, Scherz B, et al. Heterogeneity of erythrocyte catalase II. Isolation and characterization of normal and variant erythrocyte catalase and their subunits. Eur J Biochem. 1974;48:137–145. doi:10.1111/j.1432-1033.1974.tb03751.x
- Veerapur VP, Thippeswamy BS, Prabhakar KR, et al. Antioxidant and renoprotective activities of Ficus racemosa Linn. stem bark: bioactivity guided fractionation study. Biomed Prev Nutr. 2011;1:273–281. doi:https://doi.org/10.1016/j.bionut.2011.06.002.
- Wills ED. Mechanisms of lipid peroxide formation in animal tissues. Biochem J. 1966;99:667–676. doi:10.1042/bj0990667
- Nagakannan P, Shivasharan BD, Thippeswamy BS, et al. Restoration of brain antioxidant status by hydroalcoholic extract of Mimusops elengi flowers in rats treated with monosodium glutamate. J Environ Pathol Toxicol Oncol. 2012;31:213–221. doi:10.1615/JEnvironPatholToxicolOncol.v31.i3.30
- Jiang Z, Chen C, Xie W, et al. Anthocyanins attenuate alcohol-induced hepatic injury by inhibiting pro-inflammation signalling. Nat Prod Res. 2016;30:469–473. doi:10.1080/14786419.2015.1020492
- Nkosi C, Opoku A, Terblanche S. Effect of pumpkin seed (Cucurbita pepo) protein isolate on the activity levels of certain plasma enzymes in CCl4-induced liver injury in low-protein fed rats. Phytother Res. 2005;19:341–345.
- Ohashi K, Pimienta M, Seki E. Alcoholic liver disease: a current molecular and clinical perspective. Liver Research. 2018;2:161–172. doi:https://doi.org/10.1016/j.livres.2018.11.002.
- Phukan JP, Sinha A, Deka JP. Serum lipid profile in alcoholic cirrhosis: a study in a teaching hospital of north-eastern India. Niger Med J. 2013;54:5–9. doi:10.4103/0300-1652.108886
- Tan HK, Yates E, Lilly K, et al. Oxidative stress in alcohol-related liver disease. World J Hepatol. 2020;12:332–349. doi:10.4254/wjh.v12.i7.332
- Ambade A, Mandrekar P. Oxidative stress and inflammation: essential partners in alcoholic liver disease. Int J Hepatol. 2012;2012:853175. doi:10.1155/2012/853175
- Galicia-Moreno M, Gutiérrez-Reyes G. The role of oxidative stress in the development of alcoholic liver disease. Rev Gastroenterol Mex Engl Ed. 2014;79:135–144. doi:https://doi.org/10.1016/j.rgmxen.2014.06.007.
- Gao B, Bataller R. Alcoholic liver disease: pathogenesis and new therapeutic targets. Gastroenterology. 2011;141:1572–1585. doi:10.1053/j.gastro.2011.09.002
- Sultana B, Yaqoob S, Zafar Z, et al. Escalation of liver malfunctioning: a step toward herbal awareness. J Ethnopharmacol. 2018;216:104–119. doi:10.1016/j.jep.2018.01.002
- Niederreiter L, Tilg H. Cytokines and fatty liver diseases. Liver Res. 2018;2:14–20. doi:10.1016/j.livres.2018.03.003
- Liu J, Zhou H, Song L, et al. Promising natural products with diverse pharmacological activities. Molecules. 2021;26:3807. doi:10.3390/molecules26133807
- Wang M, Sun J, Jiang Z, et al. Hepatoprotective effect of kaempferol against alcoholic liver injury in mice. Am J Chin Med. 2015;43:241–254. doi:10.1142/S0192415X15500160