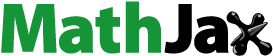
Abstract
A direct-current large-volume atmospheric pressure air glow discharge plasma (APAGDP) was developed to enhance the sewing process of denim fabric. The generated plasma was characterized optically, electrically, and spectroscopically. The generated plasma presents a positive electric resistance behaviour. The plasma emission spectra are dominated by the nitrogen second positive system. Its intensity increases with the applied voltage. The generated plasma was used as a pre-treatment to enhance the sewing of the denim fabric at different exposure times, from 5 to 600 s. The scanning electron microscopy images showed no damage to the surface morphology of the denim fabric after plasma treatment. The sound pressure level (SPL) was used to verify the plasma effect on the denim fabric as compared to the untreated fabric. The SPL decreases with plasma exposure time. This result indicates that the plasma improves the sewing performance of the denim fabric.
1. Introduction
Industrial sewing machine manufacturers are pursuing ways to increase the sewing speed and hence sewing to increase the productivity, which might affect the temperature and the penetration force of the needle [Citation1]. Also, increasing sewing speed become harder with denim fabric. Conventional industrial fabric preparation before sewing is a high-water-consumption process. Therefore, researchers proposed several techniques to control fabric moisture aiming to reduce the environmental impact of the fabric preparation process [Citation2]. Many of them tried effluent-free yarn dyeing. Others tried to reduce the amount of water used in the dye preparation and dyeing process itself. The dyeing process needs a lot of water, and the plasma treatment partially solves these problems [Citation3]. Therefore, several researchers spend a lot of efforts to enhance and develop the textile industry; they seek to introduce novel solutions to the inherited problems in the field, such as; Removing impurities and yellowness [Citation4], treating the pilling problem; [Citation5] solving ink-jet printing difficulty on cotton fabric [Citation6], improving the typological performance of denim fabric [Citation7], and improving surface energy and dynamic contact angle [Citation8,Citation9]. One of these novel solutions can be the use of cold atmospheric pressure plasmas for the pre-treatment and the finishing process of textiles. These cold plasmas opened new fields of applications to enhance the quality of certain materials, especially in the textile industries. The use of cold atmospheric pressure plasmas provides greater advantages over conventional chemical processes [Citation10]. For examples, this type of plasma is considered cheap and environmentally friendly; its system is simple and can be handled by normal trained operators; and its treatment offers high potential for achieving fine textile finishes [Citation9]. In this work we proposed a cold atmospheric pressure plasma as a novel and unique solution to enhance the sewability of denim fabric, military and defense wear fabric which have high weight per square metre.
There are many types of atmospheric pressure air glow discharge plasma (APAGDP) that can be used for various applications. Literature and prior investigations have shed light on the kinetics behind various forms of atmospheric pressure non-equilibrium plasma chemistry; the existing documentations have provided insights into the beneficial control of non-equilibrium plasma chemistry for processing applications [Citation11]. Some literatures have documented the studies of atmospheric barrier discharges, gliding discharge and surface discharges [Citation11–13]. Other studies describe atmospheric pressure plasma sources, powered by radio frequency, which produce a volumetric and homogeneous discharge [Citation14,Citation15]. Certain studies report DC glow discharge in airflow at atmospheric pressure, using anode plate and multi-pin cathode; each pin ballasted by 1MΩ resistor, with a 1-cm maximum discharge gap distance [Citation16]. Additional studies focus on a second grounded electrode was used to demonstrate the lateral extension of the atmospheric pressure plasma jet [Citation17].
Previous work shows that a great effort has been done to develop an efficient atmospheric pressure plasma system suitable for textile treatments. Atmospheric pressure air plasma system is one of the most nominated systems for this task due to the reduction in running cost. Atmospheric pressure air plasma devices can provide a crucial advantage over all low-pressure plasma systems due to the avoidance of vacuum systems; and the use of air as operating gas, which reduces the operating cost of the generated plasma. Accordingly, the research in this field is motivated by many applications, such as air plasma rampart [Citation18–20], sterilization environmental pollution control, lasers, thin-film deposition, materials processing, decontamination, ozone formation, teeth bleaching, seeds germination, wound healing, cancer treatment [Citation19–25] and plasma for textile treatments [Citation26–28].
Atmospheric pressure air plasma is enriched with generated reactive species, such as O, OH, and NO. It is also augmented with the generation of high energy photon and charged particles (electrons and ions). These reactive species interact with the textile material, causing the desired modifications [Citation29–31]. The production density of the generated species is controlled by the plasma operating parameters, such as applied voltage, discharge current, pulse duration, reputation rated, and operating gas types [Citation32]. Air plasma is active when interacted with textile. It can cause the following effects: cleaning and sterilizing the textile surface; surfacing topography modification; presenting a new chemical group of the surface; coating the textile; and changing liquid adhesion properties through the hydrophilic and heroines surface property modifications [Citation33–35].
Pervious work claims that one of the problems in the textile industry is the needle penetration forces in textile fabric, especially in technical textiles that include tent fabrics or denim fabrics [Citation36]. Therefore, with these fabrics, this type of plasma can be used in the fabric finishing and dyeing processes as the plasma is able to reduce the amount of water required in the dyeing process [Citation37]. Nevertheless, limited research exists, which tackles the utilization of atmospheric pressure cold plasma for enhancing the sewing process, which investigates the frequent needle breakage in the sewing process to improve the productivity of denim fabric. Therefore, the current research seeks to study the APAGDP operation in pulsed mode. The research seeks to investigate and characterize this plasma as a pre-treatment technique for denim fabric in the sewing process. This paper contains two phases. The first phase focuses on APAGDP diagnostics; the second phase focuses on the potential use of the characterized APAGDP to enhance the sewing process of the denim fabric.
2. Experimental setup
2.1. Plasma system and its characteristics
A large-volume atmospheric pressure air plasma was generated by applying a 100 ns high-voltage pulse between two parallel electrodes separated by a gap of up to 4.8 cm, as illustrated in Figure . The discharge frequency can be varied from 1 to 2000Hz. The power supply used in this system is a high-voltage pulse generator. It is based on magnetic pulse compression (MPC) called “HYGEIA”. The commercial name of the high-voltage nanosecond pulse generator is «Proteus-II», Electrodynamic Systems and Technologies LLC, Greifswald, Germany. The upper electrode is connected to the high-voltage power supply. It has uniformly distributed stainless-steel pins. The lower electrode is grounded and flat disc-shaped. The pins have an identical length of 2.8 cm, and each of them has a diameter of about 0.01 cm. They are separated by a distance of 1 cm from each other to form a brush shape. The pins cover a circular area of 16 cm in diameter. An automated movable stage, which is swinging by 1.5 cm, was placed on the ground electrode. The stage has a metallic flat cover that is grounded. The desired treated sample is placed on the stage cover. The swinging of the stage increases the homogeneity of treatment by the large-volume air plasma. The electric characteristics of the generated plasma were studied using a DPO7354 C −3.5 GHz – Tektronix oscilloscope with a calibrated high-voltage probe and a 1:1 Person current probe, model 6585. The applied voltage was measured across the discharge gap. The currents were measured through the grounded electrode, as illustrated in Figure . The plasma formation was monitored using a Nikon D3200 with an AF-S Micro NIKKOR 105 mm lens.
Figure 1. Schematic diagram of the experimental setup of the large-volume atmospheric pressure air plasma.

A UV-VIS-500 mm focal length spectrograph (Acton SP-2356), which is coupled by a single-leg-fibre optic bundle (LG-455-020-3), was used to investigate the plasma emission spectra. The spectrograph is triple-grating. The grating used in this work was 1800 blazed at 500. A highly sensitive photomultiplier (Princeton instrument model ARC-P2) was used as a detector with the spectrograph, which has a sensitivity range from 200 to 900 nm. The rotational temperature of large-volume air plasma was estimated from the emission spectra of the second positive system of nitrogen molecule spectra, [C3Πu→B3Πg] of the (0-0) transition. The electron-neutral collision frequency of atmospheric pressure plasmas is very high, typically in the order of THz [Citation38]. Therefore, there is a fast thermal equilibrium between the rotational and translational energies (∼6-10s). Therefore, the rotational temperature can be considered the gas temperature for atmospheric pressure plasmas [Citation39].
2.2. Fabric features
The fabric used was of medium weight denim fabric (about 302.7 grams per square metre). The yarn count in English system was 40/1 and 36/1 for both warp and weft direction respectively. The number of warp ends per centimetre was 28 and the number of weft ends per centimetre was 21. The denim fabric structure was Twill 2/1. The raw material used was 98% Cotton, 2% Spandex.
2.3. Sewing machine sound measurements
A sound pressure level (SPL) was used to measure the effect of APAGDP on the sewing process of denim fabric due to the correlation between SPL measurement and level of softness of the textile fabric [Citation40]. Low SPL measurements indicate that the textile fabric is soft and smooth. Accordingly, in this research, denim fabric with 2% Spandex was used. The APAGDP with a certain (5,300,600 s) exposure time was used to investigate the sound quality of a -single stitch Brother sewing machine (model: JV 1400). The sound quality was measured using a microphone (model: 378B02) 1/2″ prepolarized free-field condenser microphone, 50 mV/Pa ±1.5 dB, 3.15 Hz - 20 kHz (±2 dB) with 1/2″ ICP® preamplifier (426E01) and TEDS. The microphone was placed at the nearest possible position to the sewing machine needle without affecting the sewing process. The data were acquired using a high dynamic range C-series module NI- 9234 card, which is installed inside c-DAQ – 9171. It is connected to a PC, using a USB cable. A LabVIEW program was developed to acquire, store, display, and analyze the collected data with a 25.6 kS/s acquisition rate. The amount of sound pressure, in Pa unit, was measured using the following formula:
(1)
(1) where the sensitivity of the used microphone is 50 mV/Pa (±1.5 dB), 3.15 Hz - 20 kHz (±2dB).
In this research, volume plasma was generated at different operating conditions. The operating parameters were controlled through the operating program. When the plasma was set to the desired operating parameters. Then, the treated sample was placed on the movable stage above the lower plasma electrode. The plasma was turned on for the desired operating exposure time. The sample was transferred to the sewing machine directly after treatments to examine plasma treatment effect on the sewing process by recording SPL using the sensitive microphone.
2. Results and discussion
3.1. APAGDP characteristics
The APAGDP was monitored as a function of the applied voltage. A glow discharge started to ignite on the pin tip, forming a small glow. Each pin has two glow discharges due to the formation of two tips at its end that forms points of a high electric field. The glow ignition was initialized at the outer pins and formed a luminousness circle with the increase in the applied voltage (as shown in Figure ). The glow discharge plasma expanded gradually in the lateral directions over the internal pins and axial towards the ground. This fills the gap between the two electrodes as the applied voltage increases (as shown in Figure ). The pulsed discharge mechanism cannot be explained by the streamer theory since the electron avalanche time is shorter than the photon lifetime. Therefore, in sub- and nanosecond discharges the runaway electron and x-ray play an essential role in the discharge ignition. Several models and hypotheses had been proposed such as electron avalanche, electron multiplication model, fast electron model, and slow electron model [Citation41]. An individual plasma discharge column was formed between each pin and the grounded electrode. The discharge columns merged by a further increase in the applied voltage. Then, an almost homogeneous glow discharge was formed between the two electrodes. The discharge intensity and homogeneity increased with rising applied voltage and frequency. Figure shows images of APAGDP, rising the applied voltage from 4 kV to 16 kV at a frequency of 2000Hz. The merging among individual APAGDP columns were similar to the merging among atmospheric air direct-current glow discharge plasma columns [Citation42,Citation43].
Figure 2. The large-volume APAGDP formation at different applied voltage of 4, 8, 12, and 16 kV at frequency of 2000 Hz.

Figure A illustrates the typical current–voltage waveforms of the APAGDP that was operated at 1 cm gap, 500 Hz and 7.1 kV. The current pulse duration, was estimated for the positive part of the pulse, is about 100 ns. The current and voltage values were measured for the positive peak of the pulse. The current–voltage characteristics of the APAGDP (for different gap distance from 1 to 3.3 cm and fixed operating frequency of 500 Hz) is presented in Figure B. The discharge breakdown voltage increases with increasing the gap distance. The breakdown occurred at 4.14, 4.57, 4.83, and 5.47 kV at 1, 1.5, 2.3, and 3.3 cm gap distances. Then, the discharge current increases with increasing the applied voltage to reach the glow-to-arc transition point that increases with increasing the gap distances respectively. Therefore, the maximum glow discharge plasma operating voltage range increases with increasing the discharge gap as illustrated in Figure B. The current–voltage characteristics show a positive dV/dI, which means the generated discharge has a positive resistance that enables the parallel operation of the multi-glow discharge plasma column. The positive resistance value increases with increasing the discharge gap. The glow-to-arc transition point moved towards low voltage value as the gap distance between electrodes decreased. However, the pulsed discharged current values, at a constant voltage, increase with decreasing the gap distance (as shown in Figure B). The current–voltage characteristics indicated that the plasma flew in the abnormal glow mode; it was similar for negative point-to-plane corona discharges [Citation44].
Figure 3. APAGDP electric characteristics (A) typical current–voltage waveforms at 500 Hz and 1 cm discharge gap. (B) The current–voltage curves at different gaps of 1, 1.5, 2.3, and 3.3 cm at a frequency of 500 Hz.

Figure illustrates the optical emission spectra measurements at different applied voltages of 4.14, 7.1, 8.28, and 9.28 kV and at a fixed discharge gap distance and applied frequency of 1 cm and 500 Hz, respectively. The generated plasma imparted a promising material processing effect, such as surface modifications. The emission spectra from the second positive system of the nitrogen molecules were the dominant spectra; the emission spectra from NO, OH, and O radicals disappeared. This disappearance of these radicals’ spectra doesn’t mean the non-existence of these radicals. As well as the time and lateral resolutions of the emission spectra measurements could be required in future work for more precise detection of all plasma-generated species. The emission spectra of the nitrogen second positive system intensity increased with rising discharge applied voltage, which was observed in the plasma imaging from the enhancement in the plasma brightness.
Figure 4. Emission spectra of APAGDP at different applied voltages at 500 Hz and 1 cm discharge gap.

Figure 5. Gas temperature measurement for APAGDP operating at 500 Hz at the middle of a 1 cm discharge gap.

The gas temperature of the APAGDP is measured by evaluating the rotational (0,0) band of the second positive N2 system [Citation45]. The recorded spectrum was measured side-on at the middle of the 1 cm discharge gap using optical fibre bundle. The measured second positive system of nitrogen spectrum (C3Πu→B3Πg) was compared to a simulated one. The temperature was obtained by matching the two spectra. The results presented in Figure show that the estimated gas temperature was 300 K for the APAGDP, operating at 8.5 kV and 500 Hz. These results confirmed that the generated plasma is a non-thermal equilibrium plasma. Also, it can be used to treat temperature sensitive textile fabrics.
3.2. APAGDP enhancing sewing process.
The APAGDP was used to enhance the sewing process, exposing denim fabric to the plasma for different exposure times at a constant applied voltage of 8.5 kV when the discharge gap was 1 cm. The visual inspection, using Nikon digital camera D3200, shows that there are no effects on the colour of the denim fabric after plasma exposure. Moreover, the SEM (with 500 and 2000 magnifications) showed that the plasma exposure at 5, 300, and 600 s had no damage to the main structure of the fabric. It contains mild surface cleaning or etching as illustrated in Figure .
Figure 6. SEM images of denim fabric with different APAGDP exposure times of 5, 300, and 600 s at 8.5 kV and 500 Hz. A to D with a magnification of 500 while E to H with a magnification of 2000.

Figure presents the sewing SPL of the sewn denim fabric by the brother machine. Accordingly, the denim fabric was pre-treated by APAGDP, which was operated at 8.5 kV, 500 Hz, and 1 cm discharge gap for 300 s. A clear reduction in the SPL was observed when the machine needle starts to sew the plasma-treated area.
Figure 7. The SPL from the sewing machine when denim fabric was sewn. The denim fabric was pre-treated by APAGDP operated at 8.5 kV, 500 Hz, and 1 cm discharge gap for 300 s exposure time.

Figure shows the variation in the average SPL as a function of APAGDP exposure time. The results indicate that the SPL decreases with exposure time. The SPL decreased from 88.02 dB at zero plasma exposure to 86.9 dB at 600 s of plasma exposure. These signals act as an indication that the pre-treatment of denim fabric by APAGDP changes the fabric's physical characteristics. However, it is worth mentioning that there is a sudden unexplained drop of 86.69 dB in the SPL at 10 s of plasma exposure, but for sure a significant gradually decrease in the SPL of sewing machine noise level due to increasing plasma exposure time.
It has been reported previously that the low-pressure glow discharge plasma was used to modify the surface of fabric containing Spandex surface [Citation46]. This study found that plasma modified the fabric surface in both crystalline and etching. It provides an increase in the surface roughness and crystallinity of the Spandex. Studies claim that surface morphology changes in the Spandex fibre [Citation47], which are observed in the SEM images in this paper. However, the SEM images in this study showed no changes in the surface morphology as a result of APAGDP application to denim fabric (with different exposure times of up to 600 s). The short pulse duration of 100 ns and the low gas temperature of 300 K could be the reason behind this conclusion.
The sound pressure variation, as a function of plasma exposure, could be interpreted by the reorientation of the cellulose particles in denim fabric. It could also be interpreted by the plasma exposure that causes etching on the surface of the fabric [Citation9]. This reorientation of the cellulose particles reduces the penetration force of the sewing needle, which lowers the sound pressure level. Correspondingly, plasma treatment could remove organic surface contaminations from the surface of denim fabric to improve the swing process; to reduce the fraction of the lateral sides [Citation48–50].
Moreover, the atmospheric pressure plasma jet system has been proposed to change the topographical properties of the textile fabric, besides the improvement of the fabric wettability and dyeability properties of textile fabric [Citation36]. These changes in the topographical properties could be the reason behind the improvement in the sewing process.
The decrease in the SPL with increasing exposure time could be due to the reorientation of the cellulose particles in denim fabric [Citation51]. As well as the improvement in the sewing process could be interpreted by plasma etching on the surface of the fabric that changes the topographical properties of the textile fabric [Citation7]. Also, could be interpreted as an improvement due to the removal of impurities from the fabric surface due to the plasma exposure [Citation4].
4. Conclusion
In this work, a 100 ns pulsed APAGDP was generated. This plasma was characterized using electrical, optical, and spectroscopic techniques. It aimed to enhance the sewing process of denim fabric. The used plasma system consisted of a brush-like electrode, high-voltage, with multi-pins uniformly distributed on its surface and a flat grounded electrode.
The glow discharge ignited at the outer pins of the upper electrode and spread to the internal pins with rising applied voltage. The glow discharge columns were formed between the pins and the ground electrodes. The columns merge and its glow intensity increased with rising applied voltage and applied frequency. Also, the plasma emission spectra, which were dominated by the second positive system of nitrogen, increased with rising applied voltage and frequency. The current–voltage characteristics showed that the current increased with rising applied voltage; and decreased with rising discharge gap at constant operating parameters. The generated plasma gas temperature was 300 K at 8.5 kV, 500 Hz, and 1 cm gap. These results indicated that the generated plasma was a non-thermal plasma.
In this study, the generated plasma was used at different exposure times to enhance the sewing process of the denim fabric. The SPL of the sewing machine was used as an indicator to prove the improvement in the sewing process. The results showed that SPL from a plasma-treated area was lower than that of an untreated area. The SPL is reduced by rising plasma exposure time. Based on the results that emerged in this study, the conclusions could lead to a great benefit for machine manufacturers. The authors may assure that the plasma had a great effect on needle penetration force which is transformed as a decrease in the sewing machine sound. The authors are aiming to develop a portable plasma module associated with the sewing machine. These could be a new trend for the next generation of sewing machines which might speed up the sewing process of heavy-weight fabric and reduce the needle breakage rate. APAGDP offers a multipurpose, flexible, clean, and eco-friendly sewing process approach for improving the sewability of heavy-weight fabric to meet the specific requirements of the industry. Nevertheless, the limitation can be found due to the additional cost of the plasma and the thermalization.
Acknowledgements
We are very thankful to Prof. X. Lu for using his temperature simulation software.
Disclosure statement
No potential conflict of interest was reported by the author(s).
Additional information
Funding
References
- Haghighat E, Etrati SM, Najar SS. Modeling of needle penetration force in denim fabric. Int J Cloth Sci Technol. 2013;25:361–379. doi:10.1108/IJCST-01-2012-0031
- Haji A, Naebe M. Cleaner dyeing of textiles using plasma treatment and natural dyes: A review. J Clean Prod. 2020;265:121866. doi:10.1016/j.jclepro.2020.121866.
- Hossain MY, Liang Y, Pervez MN, et al. Effluent-free deep dyeing of cotton fabric with cacao husk extracts using the Taguchi optimization method. Cellulose. 2021;28:517. doi:10.1007/s10570-020-03525-8.
- Kan C, Lam C. Atmospheric Pressure Plasma Treatment for Grey Cotton Knitted Fabric. Polymers (Basel). 2018;10:53. doi:10.3390/polym10010053.
- Kan CW, Yuen CWM, Hung ON. Improving the pilling property of knitted wool fabric with atmospheric pressure plasma treatment. Surf Coat Technol. 2013;228:S588. doi:10.1016/j.surfcoat.2011.10.062.
- Thakker AM, Sun D, Bucknall D. Inkjet printing of plasma surface–modified wool and cotton fabrics with plant-based inks. Environ Sci Pollut Res. 2022;29:68357. doi:10.1007/s11356-022-20659-3.
- Kan CW, Yuen CWM. Effect of atmospheric pressure plasma treatment on the desizing and subsequent colour fading process of cotton denim fabric. Color Technol. 2012;128:356. doi:10.1111/j.1478-4408.2012.00388.x.
- Zhang Y, Matthews S, Tran ATT, and Hyland, etal. Effects of interfacial heat transfer, surface tension and contact angle on the formation of plasma-sprayed droplets through simulation study. Surf Coat Technol. 2016;307:807–816. doi:10.1016/j.surfcoat.2016.09.066
- Jelil RA. A review of low-temperature plasma treatment of textile materials. J Mater Sci. 2015;50(18):5913–5943. doi:10.1007/s10853-015-9152-4
- Shishoo R. Plasma technologies for textiles. Cambridge: Woodhead Publishing Limited; 2007.
- Massines F, Rabehi A, Decomps P, et al. Experimental and theoretical study of a glow discharge at atmospheric pressure controlled by dielectric barrier. J Appl Phys. 1998;6:2950–2957. doi:10.1063/1.367051
- Konelschatz U, Eliasson B, Egli W. Dielectric-Barrier Discharges. Principle and Applications. J Phys. 1997;7:47. doi:10.1051/JP4:1997405 Corpus ID: 85452836.
- Tschiersch R, Nemschokmichal S, Bogaczyk M, et al. Surface charge measurements on different dielectrics in diffuse and filamentary barrier discharges. J Phys D Appl Phys. 2017;50(10):105207. doi:10.1088/1361-6463/aa5605
- Baránková H, Bárdoš L. Fused hollow cathode cold atmospheric plasma. Appl Phys Lett. 2000;76:285. doi:10.1063/1.125723.
- Schütze A, Jeong JY, Babayan SE, et al. The atmospheric-pressure plasma jet: a review and comparison to other plasma sources. IEEE Trans Plasma Sci. 1998;26:1685. doi:10.1109/27.747887.
- Akishev YS, Deryugin AA, Kochetov IV, et al. DC glow discharge in air flow at atmospheric pressure in connection with waste gases treatment. J Phys D Appl Phys. 1993;26:1630. doi:10.1088/0022-3727/26/10/014.
- Mohamed A-AH, Aljuhani MM, Almarashi JQM, et al. The effect of a second grounded electrode on the atmospheric pressure argon plasma jet. Plasma Res Express; 2:015011. doi:10.1088/2516-1067/ab7b36.
- Song Y, Liu D, Wang w, et al. The Brush-Shape Device Used to Generate Atmospheric and Homogeneous Plasmas for Biomedical Applications. Plasma Process Polym. 2013;10:88. doi:10.1002/ppap.201200042.
- Stark RH, Schoenbach KH. Direct current glow discharges in atmospheric air. Appl Phys Lett. 1999;74:3770. doi:10.1063/1.124174.
- Laroussi M, Anderson1 WT. Attenuation of Electromagnetic Waves by a Plasma Layer at Atmospheric Pressure. Int J Infrared and Millimeter Waves. 1998;19:453. doi:10.1023/A:1022559710578.
- Bauer G. Plasma Medicine. Plasma Med. 2019;9:57. doi:10.1615/PlasmaMed.2019029462.
- Weltmann KD, Kolb JF, Holub M, et al. The future for plasma science and technology. Plasma Process Polym. 2019;16(1):e1800118. doi:10.1002/ppap.201800118
- Sakudo A, Yagyu Y, Onodera T. Disinfection and sterilization using plasma technology: fundamentals and future perspectives for biological applications. Int J Mol Sci. 2019;20:5216. doi:10.3390/ijms20205216
- Bekeschus S, Favia P, Robert E, et al. White paper on plasma for medicine and hygiene: Future in plasma health sciences. Plasma Process Polym. 2019;16:e1800033. doi:10.1002/ppap.201800033.
- Fadhlalmawla SA, Mohamed A-AH, Almarashi JQM, etal. The impact of cold atmospheric pressure plasma jet on seed germination and seedlings growth of fenugreek. Plasma Sci Technol2019;21:105503. doi:10.1088/2058-6272/ab2a3e.
- Deng X, Nikiforov AY, Coenye TC, et al. Antimicrobial nano-silver non-woven polyethylene terephthalate fabric via an atmospheric pressure plasma deposition process. Sci Rep. 2015;5:10138. doi:10.1038/srep10138.
- Akter S. Medical textiles: significance and future prospect in Bangladesh”. Eur Sci J. 2014;10:488–502.
- Sasmazel HT, Manolache S, Gümüsderelioglu M. Functionalization of nonwoven Pet fabrics by water/O2 plasma for biomolecule mediated cell cultivation. Plasma Process Polym. 2010;7:588. doi:10.1002/ppap.200900096
- Kan CW, Lam CF, Chan CK, et al. Using atmospheric pressure plasma treatment for treating grey cotton fabric. Carbohydr Polym. 2014;102:167. doi:10.1016/j.carbpol.2013.11.015.
- Ullah MH, Akther H, Rahman MM, et al. Surface modification and improvements of wicking properties and dyeability of grey jute-cotton blended fabrics using low-pressure glow discharge air plasma. Heliyon. 2021;7:e07893. doi:10.1016/j.heliyon.2021.e07893.
- Li D, Xu K, Zhang Y. A Review on Research Progress in Plasma-Controlled Superwetting Surface Structure and Properties. Polymers (Basel). 2022;14:3759. doi:10.3390/polym14183759.
- Cai ZS, Qiu YP. The mechanism of air/oxygen/helium atmospheric plasma action on PVA. J Appl Polym Sci. 2006;99:2233–2237. doi:10.1002/app.22307
- Šimončicová J, Kryštofová S, Medvecká V, et al. Technical applications of plasma treatments: current state and perspectives. Appl Microbiol Biotechnol. 2019;103:5117. doi:10.1007/s00253-019-09877-x.
- Manjula S, Shanmugasundaram OL, Ponappa K. Optimization of plasma process parameters for surface modification of bamboo spunlace nonwoven fabric using glow discharge oxygen plasma. J Indus Text. 2021;51(2):225–245. doi:10.1177/1528083719871265.
- Frías E, Iglesias Y, Alvarez-Ordóñez A, et al. Evaluation of Cold Atmospheric Pressure Plasma (CAPP) and plasma-activated water (PAW) as alternative non-thermal decontamination technologies for tofu: Impact on microbiological, sensorial and functional quality attributes. Food Res Int. 2020;129:108859. doi:10.1016/j.foodres.2019.108859.
- Rogina-Car B, Kovačević S. The study of needle type influence on woven fabric surface area during the sewing process. Text Res J. 2022;92:3016–3033. doi:10.1177/00405175211019133
- Höcker H. Plasma treatment of textile fibers. Pure Appl Chemist. 2002;74:423. doi:10.1351/pac200274030423.
- Bruggeman PJ, Felipe F, Brandenburg R. Foundations of atmospheric pressure non-equilibrium plasmas. Plasma Sources Sci Technol. 2017;26:123002. doi:10.1088/1361-6595/aa97af.
- Raud J, Laan M, Jõgi I. Rotational temperatures of N2(C,0) and OH(A,0) as gas temperature estimates in the middle pressure Ar/O2 discharge. J Phys D Appl Phys. 2011;44:345201. doi:10.1088/0022-3727/44/34/345201
- Cho J, Cho G. Determining the Psychoacoustic Parameters That Affect Subjective Sensation of Fabric Sounds at Given Sound Pressures. Text Res J. 2007;77:29. doi:10.1177/0040517507074023.
- Bokhan PA, Gugin PP, Lavrukhin MA, et al. Nanosecond pulse breakdown in noble gases. Phys Plasmas. 2023;30:043504. doi:10.1063/5.0138062
- Mohamed A-AH, Al-Mashraqi AA, Shariff SM, et al. Generation of large volume atmospheric pressure air plasma. IEEE Trans Plasma Sci. 2014;42(10):2488–2489. doi:10.1109/TPS.2014.2324999
- Mohamed A-AH, Block R, Schoenbach KH. Direct current glow discharges in atmospheric air. IEEE Trans Plasma Sci. 2002;30(1):182–183. doi:10.1109/TPS.2002.1003984
- Zhang H, Zhu J. A general empirical formula of current–voltage characteristics for point-to-plane geometry corona discharges. J Phys D Appl Phys. 2008;41:065209. doi:10.1088/0022-3727/41/6/065209
- Seo YS, Lee HW, Mohamed A-AH, et al. Correlation of striated discharge patterns with operating conditions in helium and argon atmospheric-pressure plasma jets. IEEE Trans Plasma Sci. 2011;39:2324–2325. doi:10.1109/TPS.2011.2159400
- Bhat N, Netravali A, Gore A, et al. Surface modification of cotton fabrics using plasma technology. Text Res J. 2011;81:1014. doi:10.1177/0040517510397574.
- Vijayalakshmi KA, Karthikeyan N, Vignesh K. Surface Modification of Spandex Fiber Using Low Temperature Plasma International Journal of Science and Research (IJSR), ISSN (Online): 2319-7064, Impact Factor (2012): 3.358. www.ijsr.net 2014.
- Ngo H-T, Vu Thi Hong K, Nguyen T-B. Surface Modification by the DBD Plasma to Improve the Flame-Retardant Treatment for Dyed Polyester Fabric. Polymers (Basel). 2021;13:3011. doi:10.3390/polym13173011.
- Väänänen R, Heikkilä P, Tuominen M, et al. Fast and efficient surface treatment for nonwoven materials by atmospheric pressure plasma-. AUTEX Res J. 2010;10 8. http://www.autexrj.com/cms/zalaczone_pliki/2_328.pdf.
- Bhat N, Netravali A, Gore A, et al. Surface modification of cotton fabrics using plasma technology. Textile Res J. 2011;81:1014–1026. doi:10.1177/0040517510397574
- Yun YI, Kim KS, Uhm SJ, et al. Aging behavior of oxygen plasma-treated polypropylene with different crystallinities. J Adhes Sci Technol. 2004;18:1279. doi:10.1163/1568561041588200.