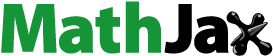
Abstract
In this study, a silver nanoparticle-chitosan (AgNPCh) composite film was developed for the determination of antioxidant capacity of polyphenolic compounds. The optimum conditions of solid nanosensor based on chitosan-based polymeric membranes containing AgNPs such as content of the composite film, pH and incubation time were idendified. The sensor measurement is based on the fact that the addition of polyphenols to the sample solution increases the absorption of Ag(0) NPs, which exhibit a characteristic plasmon absorption band at a wavelength of 423 nm and the developed method exhibits a linear variation versus the concentrations standard polyphenolic compounds. The method was applied to the apple, peach, cherry, grape and pomegranate juices and rosemary, marjoram and sage plant extracts. This sensor may be an alternative to conventional spectroscopic methods to design portable, low-cost, simple and easy-to-use field analyses without the need for special equipment for the determination of antioxidant capacity of polyphenolic compounds.
1. Introduction
In recent years, the importance of preventing reactive oxygen species (ROS) from damaging macromolecules such as protein, lipid and DNA in living organisms under oxidative stress conditions has been better recognized and the interest in compounds that prevent the damage caused by these reactive species in the organism has increased [Citation1,Citation2]. As it is known, having both hydroxyl groups [Citation3] and double bonds that increase the delocalization of π-electrons in ring structures, polyphenolic compounds that show antioxidant properties play a major role in protecting against diseases by significantly reducing the oxidative properties of free radicals. This has led to the development of many antioxidant capacity/activity assays in the literature. The determination of the antioxidant activity/capacity of a wide variety of food samples/biological samples/synthetics is based on very different methodologies such as molecular spectrometric methods [Citation4]: CUPRAC, ABTS/TEAC, FRAP, Folin–Ciocalteu, ORAC, etc.; electrochemical methods, chromatographic methods, biochemical sensors and nanotechnological methods: silver and gold nanoparticle based methods, etc. Nanotechnological techniques are generally colorimetric total antioxidant capacity (TAC) determination tests, usually based on the formation of gold and silver nanoparticles (AgNPs) by reaction between metallic salt (Au3+, Ag+) and antioxidant compounds [Citation5].
Chitosan is a linear polysaccharide consisting of N-acetyl–D–glucosamine and D–glucosamine copolymers, obtained by deacetylation of chitin in alkaline media, and is the most commonly found in nature after cellulose [Citation6]. Its molecular weight is between 3,200 and 2,000,000 g/mol. Although there are numerous studies on chitosan-based sensors and membranes in the literature, only a few studies on chitosan-based polymeric hybrid membranes containing AgNPs have been found. While Maruthupandy et al. [Citation7] prepared chitosan/AgNPs synthesized for colorimetric sensor of glucose molecules in aqueous media, Khalaf et al. [Citation8] developed chitosan polymer complex-derived nanocomposite containing AgNPs for electrochemical non-enzymatic glucose sensor. Imran et al. [Citation9] synthesized and used positively charged chitosan stabilized AgNPs as a sensitive electrochemical nanobiosensor for the detection of lipopolysaccharide. It is seen in the literature that some different nanoparticles are used in sensor studies together with chitosan. Khan et al. [Citation10] used zinc oxide nanoparticle and chitosan composite films as cholesterol biosensors. Wang et al. [Citation11] developed an amperometric tyrosinase biosensor based on iron oxide (Fe3O4) nanoparticle–chitosan nanocomposite for the detection of phenolic compounds. Cardenas et al. [Citation12] synthesized a colloidal Cu nanoparticle/chitosan composite film obtained by microwave heating for food packaging applications and the preparation and characterization of colloidal Cu nanoparticle/chitosan composite film by microwave heating and solution-casting technique was investigated.
In particular, methods based on the direct reduction of silver salts by polyphenols and the spectrophotometric measurement of localized surface plasmon resonance (LSPRs) of AgNPs [Citation13] formed as a function of polyphenol concentration are limited in the literature [Citation14,Citation15]. Vilela et al. [Citation16] showed the ability to reduce Au(III) of endogenous food polyphenols to Au(0) in the form of AuNPs in aqueous solvents, and the wavelength at which AuNPs showed maximum optical absorption was determined according to LSPR. AgNPs synthesis studies using various reducing compounds and natural extracts have also been carried out [Citation17]. Compared to commonly used Au0/Au3+ pair, the standard redox potential of the Ag0/Ag+ pair shows a higher reducing power. However, extremely rapid kinetics occur in the polyphenol-mediated formation of AgNPs that cause aggregation, NPs precipitation or unstable NPs suspensions. In another method based on core-mediated growth developed by Teerasong et al. [Citation18], Ag+ is reduced to Ag0 by polyphenols and accumulates on poly(vinyl alcohol)-AgNP surface. Özyürek et al. [Citation19] proposed a colorimetric method based on citrate-stabilized AgNPs core growth by LSPR increase at 423 nm in the presence of polyphenolic compounds. With this AgNPs-based method, polyphenolic compounds without the effect of some potential interferants studied have been successfully classified according to TAC. Similar to this methodology, the development of new methods of determination based on the direct and spontaneous bonding of AgNPs with polyphenolic compounds that allow for simple, selective, sensitive and a wide range of analysis has gained importance.
To the best of our knowledge, the chitosan-based polymeric membranes containing AgNPs were used for determination of TAC for some polyphenolic compounds with high sensitivity and selectivity in this study, for the first time. Antioxidant capacity values were determined by measuring the surface plasmon absorbances corresponding to the analyte concentration by analyte-mediated seeding growth of NPs. The developed AgNPCh method was applied to some polyphenolic compounds, synthetic mixtures and real samples (fruit juices and plant extracts) and the results were compared with the findings of the CUPRAC method. The proposed assay could pave the way for AgNP-based products/sensors for TAC detection of polyphenolic compounds, especially in food chemistry.
2. Experimental
2.1. Instrumentation
A Varian Cary Bio 100 model UV–visible field A double quartz cuvette of HELMA brand with 10 mm light path was used for spectrophotometric measurement and a FEI Model Quanta 450 FEG brand SEM used for characterization of the NPs.
2.2. Materials and chemicals
All chemicals used in this study were of analytical grade. Trisodium citrate, silver nitrate (AgNO3), glacial acetic acid, sodium acetate, ethyl alcohol (96%) were supplied from Merck (Darmstadt, Germany); chitosan (low molecular weight), (3-Glycidyloxypropyl) trimethoxysilane (GPTMS), gallic acid (GA), caffeic acid (CFA), chlorogenic acid (CHA), quercetin (QR), (–) epicatechin gallate (ECG), (–) epicatechin (EC), (+) catechin (CT), L–ascorbic acid (AA), rutin (RT), α–tocopherol, trolox (TR), and ferulic acid (FR) were purchased from Sigma–Aldrich (Steinheim, Germany). Fruit juices and green tea were obtained from local markets, and rosemary, marjoram and sage were bought from local herbalists in Istanbul, Turkiye.
2.3. Preparation of solutions
AgNO3 stock solution was prepared by weighing 0.0424 g of AgNO3 to be 5.0 mM and completed to 50 mL with distilled water. Trisodium citrate solution was prepared by weighing 0.5 g of trisodium citrate-2-hydrate as 1% (w/v) and making it up to 50 mL with distilled water.
2.4. Synthesis of AgNPs
The method applied by Özyürek et al. [Citation19] was used for the synthesis of AgNPs. A 50 mL of AgNO3 working solution (1.0 mM) was heated under reflux until it boiled (for 10 min), and 5 mL of 1% trisodium citrate solution was added dropwise to the solution while stirring with a magnetic stirrer. Afterwards, a light yellow coloured AgNPs solution was obtained by heating under reflux for another 5 min. With the applied synthesis method, Ag+ ions were reduced with trisodium citrate and nano-sized spherical NPs were formed (Equation (1)).
(1)
(1)
2.5. Synthesis of AgNPCh
To prepare the solid phase nano-sensor based on silver nanoparticle-chitosan (AgNPCh) composite film, 1.5% (w/v) chitosan solution was prepared by adding 0.75 g low molecular weight chitosan into 50 mL AgNPs solution which was acidified with 1 mL of glacial acetic acid (2% w/v), and the mixture was stirred at room temperature at 150–200 rpm in a mechanical stirrer (3 h). Subsequently, 0.375 g of GPTMS (0.35 mL) was added to the medium as a crosslinker and mixed for 1 h more at room temperature. All of the prepared homogeneous mixture was cast on a Teflon PTFE plate and the film was dried on a levelled surface in an oven by applying a heating programme (Table ). Finally, modified chitosan composite films with AgNPs were cut to 4.5 × 0.5 cm and solid phase nano-sensors were prepared for the determination of antioxidants.
Table 1. Optimal parameters for the synthesis of modified chitosan film containing AgNPs.
2.6. Preparation of real samples
Fruit juice samples were passed through a 0.45 µm-pore polyester filter (GF/PET-45/25) before the analysis and diluted with ethanol at the desired ratio and used in the related analyses.
For the preparation of plant extracts, extraction of herbal samples was done by microwave extraction method. Plant samples (rosemary, marjoram and sage) were weighed 0.1 g and 20 mL of MeOH – H2O (80:20, v/v) was added. The samples were extracted at 80°C for 5 min in a microwave extractor and after filtering through ordinary filter paper, they were passed through a 0.45 µm-pore diameter polyester filter (GF/PET-45/25) and diluted with ethanol at the desired ratio before analysis.
For the preparation of green tea infusions, 2 g of green tea were mixed with 250 mL of boiling water and soaked for 2 min under agitation. After 3 min of brewing time, the infusion was cooled at room temperature, they were passed through a 0.45 µm-pore diameter polyester filter (GF/PET-45/25) and diluted with EtOH at the desired ratio before analysis.
2.7. Determination of antioxidant capacity
In the proposed AgNPCh-based method, x mL of polyphenol standard or real sample solution and (8–x) mL of EtOH were added to a test tube (1 × 15 cm), respectively. AgNPCh composite film was treated with polyphenol standard solutions to determine the optimum conditions (incubation time and pH) for antioxidant capacity determination. The absorbance value at 423 nm of the nanosensor immersed in the presence of polyphenolic compounds is higher than the absorbance value in the polyphenol-free reference solution.
As a result of the reaction (Equation (2)), the reactive Ar–OH groups of the polyphenols are oxidized to the corresponding quinones, while the Ag+ ions are reduced to coloured AgNPs (core–shell formation) with maximum absorption at 423 nm (standard potential for physiologically important antioxidants E0 = 0.2–0.6 V, E0Ag(I), Ag = 0.8 V).
(2)
(2) The differences between the absorbance values (ΔA) obtained in the presence and absence of antioxidants could be determined. The antioxidant capacity of polyphenol standards in terms of trolox equivalents (TEAC) was calculated according to the equation given below, with the help of the molar absorption coefficient (ε) of trolox (Equation (3)).
(3)
(3) where Vf the total volume, Vs the sample volume taken for analysis, and r the dilution factor.
Single, binary and ternary synthetic mixtures of polyphenol standards in EtOH were prepared in suitable volume ratios and were subjected to the AgNPCh method. The predicted and experimentally measured antioxidant capacities of synthetic mixtures were calculated according to Equations (4) and (5) in terms of mM TR equivalent by applying AgNPCh and CUPRAC methods [Citation20].
(4)
(4) (C: concentration)
(5)
(5) The TAC of the fruit juices containing polyphenols were calculated according to the Equation (6):
(6)
(6) The TAC of the extracts prepared by the extraction method of various food samples containing polyphenols were calculated according to the Equation (7):
(7)
(7) In Equation (7), Vi; the solvent volume taken for extraction, m; the sample amount taken.
The reaction mixture containing AgNPCh solid nano-sensor and gallic acid was adjusted to pH 3.0–7.0 using acetic acid-sodium acetate buffers to evaluate the effect of pH on absorbances. Additionally, the influence of incubation time on responses was investigated and optimum pH and incubation time were determined.
2.8. Statistical analysis
In this study; samples were analysed in triplicate. All obtained results were expressed as the mean ± standard deviation (SD). Statistical analyses and standard errors of the mean were performed using Microsoft Excel software (2013). The evaluation of the statistical significance of the data was determined by Student's t-test and F-test. One-way analysis of variance (ANOVA) and F test of obtained results were performed for comparison of means and significant differences.
2.9. Scanning electron microscopy (SEM) analysis
In order to obtain SEM images of the synthesized AgNP-modified composite film, the composite film was placed on the carbon tape adhered to the aluminum layer. The samples were frozen by spraying SHUR/Freeze Cryogen and dried under vacuum (6 × 10–2 mbar). Images of AgNPs were acquired in SEM mode and at the desired magnification.
3. Results and discussions
3.1. SEM analysis and absorption spectra of AgNPs
AgNPs on the nano sensor surface were characterized by SEM. Accordingly, it is seen that AgNPs formed are spherical, monodisperse and homogeneous particles in nanometre size. The sizes of the formed AgNPs are in the range of 30–60 nm (Figure ).
Absorption spectra of AgNPs in the absence (a) and presence (b) of GA were given in Figure . Adding GA to the sample solution results in the formation of Ag(0) NPs, which exhibit a characteristic plasmon absorption band at 423 nm wavelength. This band originates from the surface plasmon resonance of AgNPs and can be attributed to the collective oscillation of electrons in particles with a periodic change in electron density at the surface.
3.2. Development of AgNPCh method
In order to determine incubation time, which is one of the optimum experimental conditions of the developed AgNPCh method to determine the antioxidant properties of polyphenols, the time-dependent change in the absorbance of AgNPs formed in the presence and absence of polyphenols (GA, CT, CFA and AA) was observed (Figure ).
Figure 3. The obtained reaction kinetics of GA, CT, CFA and AA by AgNPCh method (measured at 423 nm for 0.1 mM antioxidant solution at room temperature).

According to the experimental results in Figure , in the presence of these polyphenols, the optimum incubation time was determined as 60 min, due to an increase in the absorbance of AgNPs.
It is known that pH has an effect on AgNP formation. For this reason, to determine the optimum pH value of the developed method and examine the pH effect were aimed. As can be seen in Figure , pH 5.0 (0.10 M acetic acid-sodium acetate buffer), where the plasmon absorbance of AgNPs was the highest, was determined as the optimum working pH.
3.3. Determination of antioxidant capacity of polyphenols
The developed modified chitosan film containing AgNPs is based on the growth of silver nanoparticles within the chitosan structure in polyphenol medium and the measurement of the plasmon absorbance of these growing nanoparticles at 423 nm wavelength. The absorbance values of AgNPs increase linearly depending on the concentrations of the polyphenolic compounds [Citation21,Citation22]. Accordingly, as the concentration of polyphenol standards (CT, CFA and GA) increased, the absorbance increased in relation to the plasmon resonance of AgNPs. The plasmon absorbances of AgNPs formed in the presence of these polyphenols were linear in the concentration range of 0.5–2.5 mM (Table ). The calibration curves of other polyphenolic compounds studied within the scope of the study and the molar absorption coefficients (ε) of these antioxidant compounds were given in Table .
Table 2. Calibration equations (A = mC + n), correlation coefficients (r) and molar absorptivities (ε) of polyphenols obtained by nano-sensor method based on AgNPCh (N = 3).
TEAC coefficients of polyphenols based on AgNPCh and the reference CUPRAC assays were calculated according to the Equation (3) and the findings were given in Table .
Table 3. TEAC coefficients of polyphenols according to AgNPCh-based and CUPRAC assays (N = 3).
Among the polyphenols studied, QR shows the highest capacity, and AgNPCh assay coefficients decrease in the following order: QR > RT > GA > CFA > CHA > AA > CT > ECT.
While there is no difference between the TEAC coefficients found for GA, CFA, CHA, QR, RT and AA, TEACAgNPCh coefficients for CT and ECT were significantly lower than TEACCUPRAC (n = 3, p < 0.05), as compared by Student’s t test. The one-way ANOVA test was calculated, accompanied by the F test. There is no significant difference between TEACAgNPCh and TEACCUPRAC coefficients at significance level, p < 0.05.
3.4. TAC of synthetic antioxidant mixture solutions
Synthetic mixtures consisting of binary and triple combinations of standard antioxidant compounds were prepared and after dilution with ethyl alcohol solution, their antioxidant capacity was determined using the AgNPCh-based assay (Table ). It has been observed that the theoretical expected capacities and the experimentally found capacities (mM TR) for synthetic mixtures give consistent results and the maximum deviation is only ± 5.4%. In the light of the results of this study, it is understood that there are no chemical deviations from the Lambert–Beer law, the plasmon absorbance of AgNPs formed in the presence of antioxidant mixtures is additive, and the sum of the individual capacities of the antioxidants contained in the antioxidant mixture determines the TAC of the mixture. According to t test and F test, the experimentally found capacity results and the expected capacity results are similar at 95% confidence level by the proposed method. Thus, it can be said that the validity of the AgNPCh-based analysis has been proven.
Table 4. Comparison of expected and experimentally measured TAC values in mM trolox (TR) of synthetic antioxidant mixture solutions according to AgNPCh-based assay (N = 3).
3.5. Selectivity, repeatability and accuracy of AgNPCh
In order to determine the repatability and recovery of the AgNPCh-based assay, known amounts of RT, GA and CT standard solutions were added to green tea extracts. 0.8 mL of green tea infusions and 0.8 mL of 10 mM polyphenol solution (final conc. 1.0 mM) were taken into tubes and completed to 8 mL with EtOH. The AgNPCh-based assay was applied separately to the solutions with RT, GA and CT additives and the obtained recovery values were calculated (Table ). According to the data in Table , recovery (%) values between 90.14% and 92.38% and RSD (%) values between 2.16 and 3.56 indicate that the developed method is accurate and precise.
Table 5. Recovery of RT, GA and CT in green tea samples with respect to the AgNPCh-based assay (N = 3).
The interference effect of components that may be present in some food samples and have the potential for interference in absorbance measurement of various polyphenols on the AgNPCh sensor was examined. According to the results, in the presence of QR (1 mM), AgNPCh showed a significant absorptivity, while almost no interaction with other components (5 mM) was observed (Figure ).
3.6. Application of the developed method to real samples
Fruit juice samples were prepared as described in experimental section and TAC of fruit juice samples was determined by applying the developed method and the reference CUPRAC assay. TAC values were calculated in terms of antioxidant capacity (mM TR) using Equation (6) and the results obtained are presented in Table .
Table 6. Comparative TAC values (mM–TR equivalent) of some fruit juices measured according to AgNPCh and CUPRAC methods (N = 3).
TAC values of fruit juices obtained with the AgNPCh-based assay and the CUPRAC method chosen as the reference method were found to be quite compatible with each other. TAC values of fruit juices are listed as follows according to the AgNPCh-based assay: peach < apple < cherry < grape < pomegranate juice.
TAC of the plant extracts was determined using Equation (7) in terms of trolox equivalent antioxidant capacity (mmol TR /g-sample) by applying AgNPCh-based assay and CUPRAC methods.
The developed method was applied to rosemary, marjoram and sage extracts and the total antioxidant capacity values obtained for the plant extracts are summarized below (Table ).
Table 7. TAC of some plant extracts determined by AgNPCh-based assay and CUPRAC methods (N = 3).
TAC values obtained with the developed AgNPCh-based assay and the CUPRAC method chosen as reference method were found to be compatible with each other. TAC values of the plant extracts are listed as follows according to AgNPCh method: Sage > marjoram > rosemary.
4. Conclusions
In this study; an AgNPs based nano-sensor (AgNPCh) developed for the evaluation of total antioxidant capacity for different polyphenolic compounds. All AgNP-chitosan film parameters and conditions of measurement have been optimized so that the method can be stable, versatile and reproducible. Analysis results of synthetic antioxidant mixture solutions and real samples clearly show that the spectral changes and plasmon absorbance are in consistent with the reducing capacities of polyphenolic compounds, and the growth of AgNPs gave a linear signal depending on the concentration. According to the AgNPCh-based assay, TEAC coefficients of 8 different polyphenolic compounds were found. Comparison with reference findings showed that the highest ability of reducing Ag(I) to AgNPs corresponds to the highest TAC, consistent with the tendency of polyphenolic compounds to donate electrons. This assay was validated through linearity, precision and recovery, demonstrating that the assay is reliable and precise. Additional AgNPs formation on the preformed Ag seeds proceeds by polyphenolic compound reduction of Ag+ ions under optimized conditions, and the findings of the AgNPCh-based assay correlate well CUPRAC assay. Interference studies showed that the proposed assay is not adversely affected from common food ingredients such as citrate, oxalate, tartarate, fruit acids, simple sugars and amino acids. With the use of the AgNPCh-based assay, it will be easier for researchers in the fields of food and biochemistry to monitor the antioxidant capacities of various food extracts taken in the daily diet, even in relatively less equipped laboratories, to fight oxidative stress-induced diseases.
The proposed assay could pave the way for AgNP-based products/sensors for TAC detection of polyphenolic compounds, especially in food chemistry.
Disclosure statement
No potential conflict of interest was reported by the author(s).
Additional information
Funding
References
- Shields HJ, Traa A, Van Raamsdonk JM. Beneficial and detrimental effects of reactive oxygen species on lifespan: a comprehensive review of comparative and experimental studies. Front Cell Dev Biol. 2021;9:181. doi:10.3389/fcell.2021.628157
- Kılınçer M, Çiçek G, Özyürek M, et al. Uncertainty estimation for total antioxidant capacity measurement of apple juice using main CUPRAC method. J Chem Metrol. 2022;16:28–37. doi:10.25135/jcm.67.2201.2311
- Kongpichitchoke T, Hsu JL, Huang TC. Number of hydroxyl groups on the B-ring of flavonoids affects their antioxidant activity and interaction with phorbol ester binding site of PKCδ C1B domain: in vitro and in silico studies. J Agric Food Chem. 2015;63:4580–4586. doi:10.1021/acs.jafc.5b00312
- Apak R, Özyürek M, Güçlü K, et al. Antioxidant activity/capacity measurement. 1. Classification, physicochemical principles, mechanisms, and electron transfer (ET)-based assays. J Agric Food Chem. 2016;64:997–1027. doi:10.1021/acs.jafc.5b04739
- Bedlovičová Z, Strapáč I, Baláž M, et al. A brief overview on antioxidant activity determination of silver nanoparticles. Molecules. 2020;25:3191. doi:10.3390/molecules25143191
- Elieh-Ali-Komi D, Hamblin MR. Chitin and chitosan: production and application of versatile biomedical nanomaterials. Int J Adv Res (Indore). 2016;4:411–427.
- Khalaf N, Ahamad T, Naushad M, et al. Chitosan polymer complex derived nanocomposite (AgNPs/NSC) for electrochemical non-enzymatic glucose sensor. Int J Biol Macromol. 2020;146:763–772. doi:10.1016/j.ijbiomac.2019.11.193
- Maruthupandy M, Rajivgandhi G, Muneeswaran T, et al. Chitosan/silver nanocomposites for colorimetric detection of glucose molecules. Int J Biol Macromol. 2019;121:822–828. doi:10.1016/j.ijbiomac.2018.10.063
- Imran M, Ehrhardt CJ, Bertino MF, et al. Chitosan stabilized silver nanoparticles for the electrochemical detection of lipopolysaccharide: a facile biosensing approach for gram-negative bacteria. Micromachines (Basel). 2020;11(4):413. doi:10.3390/mi11040413
- Khan R, Kaushik A, Solanki RS, et al. Zinc oxide nanoparticles-chitosan composite film for cholesterol biosensor. Anal Chim Acta. 2008;616:207–213. doi:10.1016/j.aca.2008.04.010
- Wang Y, Qu J, Liu G. Preparation of Fe3O4–chitosan nanoparticles used for hyperthermia. Adv Powder Technol. 2010;21:461–467. doi:10.1016/j.apt.2010.01.008
- Cardenas G, Diaz VJ, Melendrez MF, et al. Colloidal Cu nanoparticles/chitosan composite film obtained by microwave heating for food package applications. Polym Bull. 2009;62:511–524. doi:10.1007/s00289-008-0031-x
- Syafiuddin A, Salmiati, Salim MR, et al. A review of silver nanoparticles: research trends, global consumption, synthesis, properties, and future challenges. J Chin Chem. 2017;64:732–756. doi:10.1002/jccs.201700067
- Prieto-Blanco MC, Pardo-Puñal M, Moliner-Martínez Y, et al. Determination of antioxidant activity by in situ synthesis of AgNPs using in-tube SPME coupled on-line to capillary liquid chromatography. Microchim Acta. 2023;190:299. doi:10.1007/s00604-023-05886-w
- Chen Z, Zhang X, Cao H, et al. Chitosan-capped silver nanoparticles as a highly selective colorimetric probe for visual detection of aromatic ortho-trihydroxy phenols. Analyst. 2013;138:2343–2349. doi:10.1039/c3an36905f
- Vilela D, Castañeda R, González MC, et al. Fast and reliable determination of antioxidant capacity based on the formation of gold nanoparticles. Microchim Acta. 2015;182:105–111. doi:10.1007/s00604-014-1306-6
- Marambio-Jones C, Hoek EMV. A review of the antibacterial effects of silver nanomaterials and potential implications for human health and the environment. J. Nanoparticle Res. 2010;12:1531–1551. doi:10.1007/s11051-010-9900-y
- Teerasong S, Jinnarak A, Chaneam S, et al. Poly(vinyl alcohol) capped silver nanoparticles for antioxidant assay based on seed–mediated nanoparticle growth. Talanta. 2017;170:193–198. doi:10.1016/j.talanta.2017.04.009
- Özyürek M, Güngör N, Baki S, et al. Development of a silver nanoparticle–based method for the antioxidant capacity measurement of polyphenols. Anal Chem. 2012;84:8052–8059. doi:10.1021/ac301925b
- Apak R, Güçlü K, Özyürek M, et al. Novel total antioxidant capacity index for dietary polyphenols and vitamins C and E, using their cupric Ion reducing capability in the presence of neocuproine: CUPRAC method. J Agric Food Chem. 2004;52:7970–7981. doi:10.1021/jf048741x
- Murphy CJ, Saub TK, Gole AM, et al. Anisotropic metal nanoparticles: synthesis, assembly, and optical applications. J Phys Chem B. 2005;109:13857–13870. doi:10.1021/jp0516846
- Rostami S, Mehdinia A, Jabbari A. Seed-mediated grown silver nanoparticles as a colorimetric sensor for detection of ascorbic acid. Spectrochim Acta -A: Mol Biomol Spectrosc. 2017;180:204–210. doi:10.1016/j.saa.2017.03.020