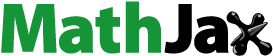
Abstract
Oncology research progresses, yet cancer remains the largest medical need. The use of a medication with an accessible lead molecule shows great potential for long-term cancer therapy. It may help target cancer therapy and drug resistance. This work uses FDA-approved, clinically proven compounds to build novel cancer therapeutic ligands to prevent treatment resistance. Based on the reported findings, an in-silico docking experiment was performed using FDA-licensed fungus medication posaconazole. The ALK TK pdb:4cmu ligand and posaconazole docked similarly in the research. Posaconazole's in silico docking findings were then tested against A549 lung cancer cells. Posaconazole, the lead, underwent an ADME investigation. Given in silico and experimental confirmation, acetyl, benzyl, and benzoyl derivatives of the lead molecule posaconazole was synthesized. IR, mass, 1H NMR, 13C NMR, and elemental analysis were performed on the synthesized compounds. Next, the three variants were evaluated against A549 lung cancer cells. They were then examined by utilizing computational approaches such as molecular docking, DFT analysis, MD simulation, and MMGBSA analysis. The ADME studies were conducted on posaconazole and its derivatives based on the in-vitro cell line investigation. Based on the results of an ADME study, two new ALK TK inhibitors that don't work as Pgp substrates are good lead candidates. Thus, the present investigation fruitfully reported two lead drug candidates against the issue of resistance in cancer therapy.
1. Introduction
In the twenty-first century, cancer has emerged as the predominant cause of mortality [Citation1]. Lung cancer is the most prevalent cancer globally, and it is responsible for a disproportionately high number of deaths and illnesses among both sexes. Patients diagnosed with lung cancer had a 15.9% five-year survival rate. In addition, there are two subtypes of lung cancer: small-cell and non-small-cell. Of these, NSCLC accounts for around 85% of all lung cancer diagnoses [Citation2]. Those who are diagnosed with lung cancer have a dismal outlook. Nevertheless, substantial advancements in therapy and detection over the past ten years have resulted in the first rise in lung cancer survival rates in this demographic [Citation3]. The advent of comprehensive molecular profiling has reshaped the pathological categorization of lung cancer by revealing fresh molecular targets for the treatment of individual patients. In addition to opening up new therapeutic options for cancer, the immunotherapy revolution is also reclassifying a number of tumour types, including lung cancer [Citation4].
In recent decades, chromosomal rearrangements in the anaplastic lymphoma kinase (ALK) receptor tyrosine kinase were discovered in a subgroup of NSCLC (non-small cell lung cancer) patients. As a result of fusion with other oncogenes (NPM, EML4, TIM, etc.) or gene amplification, mutation, or protein overexpression, anaplastic lymphoma kinase (ALK) gene activation is involved in the carcinogenesis process of several human cancers, such as anaplastic large cell lymphoma, lung cancer, inflammatory myofibroblastic tumours, and neuroblastoma. ALK is a transmembrane tyrosine kinase receptor that dimerizes and then auto phosphorylates the intracellular kinase domain in response to ligand binding to its extracellular domain. The efficacy of crizotinib, a small-molecule ATP-competitive ALK inhibitor, was promptly established as superior to chemotherapy in the treatment of patients with ALK-positive non-small cell lung cancer. Immunohistochemistry and in situ hybridization techniques are being used for the detection of ALK rearrangement. Crizotinib, ceritinib, and alectinib, which are ATP-competitive ALK inhibitors, have been authorized for use as primary therapeutic interventions in persons exhibiting such rearrangements [Citation5]. Lung cancers often include anaplastic lymphoma kinase (ALK) as an oncogenic mediator protein. Patients with ALK fusion-positive (ALK+) lung tumours benefit well from ALK tyrosine kinase inhibitors; however, the durability of this response is compromised by the development of drug resistance. Next-generation ALK inhibitors have been made possible by the discovery of on-target resistance mechanisms, although it is still difficult to overcome ALK-independent resistance mechanisms [Citation6,Citation7].
Individuals diagnosed with advanced ALK-positive lung cancer are now undergoing treatment with crizotinib, which is classified as a first-generation ALK inhibitor. As the disease advanced, medical professionals resorted to the use of ceritinib and alectinib, which are two ALK inhibitors belonging to the second generation. Even in the absence of ALK mutations that provide resistance to crizotinib, the use of second-generation inhibitors is anticipated to be beneficial due to the insufficient blocking efficacy of crizotinib against ALK in several circumstances [Citation7]. The anaplastic lymphoma kinase (ALK) family is involved in 3–7% of all lung cancer cases, making it a major percentage of the genetic defects that drive oncogenesis in this illness [Citation8]. The molecular target known as anaplastic lymphoma kinase (ALK) has been established as a validated target in several malignancies characterized by ALK rearrangements, such as non-small cell lung cancer. Nevertheless, the therapeutic effectiveness of ALK targeting with tyrosine kinase inhibitors (TKI) is consistently limited by the development of drug resistance [Citation9]. Despite the approval of many small-molecule inhibitors targeting ALK fusion proteins by the FDA, concerns over the emergence of clinical resistance to these pharmaceuticals persist. Hence, it is essential to develop innovative therapeutic approaches in order to address the issue of resistance to ALK inhibitors. Additionally, greater investigation into the genetic components of ALK rearrangements is warranted.
The increasing attraction of repurposing “old” drugs as a viable alternative for treating both common and uncommon ailments emanates from factors like high attrition rates, substantial expenditures, and a slow pace of new medication discovery and development [Citation9]. This phenomenon may be attributed to the potential cost-effectiveness of overall research expenditures and the reduced development durations associated with repurposed drugs. The use of repurposed pharmaceuticals is gaining traction as an attractive approach for cancer treatment due to its potential to expedite regulatory approval processes. [Citation10]. Statins, itraconazole, and clarithromycin represent just a handful of non-cancer drugs that have shown preclinical efficacy in combating cancer [Citation11]. Gerber and Culliges provide an investigation into the potential use of itraconazole as an anticancer therapeutic drug across many cancer types. The severity of itraconazole's early antivascular, metabolic, and anticancer effects in patients with non-small cell lung cancer exhibited variation according to the dosage administered [Citation12]. Based on research conducted by Baozhi Chen and Culliges, it has been shown that posaconazole, an antifungal medication, has a mechanism of action in inhibiting the Hh pathway that is comparable to itraconazole. However, this mechanism differs from that of cyclopamine and other SMO antagonists that compete with cyclopamine. Posaconazole has similar characteristics to itraconazole and demonstrates significant efficacy against drug-resistant SMO mutants. Additionally, it effectively inhibits the progression of Hh-dependent basal cell carcinoma in vivo [Citation13].
Hence, this study aimed to present the findings of an in silico molecular docking analysis on posaconazole, along with the outcomes of an experimental evaluation using the MTT assay on the A549 cell line. The objective was to establish posaconazole as a potential candidate for repurposing as an ALK inhibitor in the treatment of lung cancer.
In addition to this, the phenomenon referred to as multidrug resistance (MDR) is involved in the resistance of cancer cells to chemotherapy. Three proteins, namely MDR-associated protein 1 (MRP1), breast cancer resistance protein (BCRP), and P-glycoprotein (P-gp; often referred to as MDR1), have been scientifically identified as significant factors in the development of multidrug resistance (MDR) [Citation14]. P-gp is a wide substrate-specificity ATP-dependent drug efflux pump for xenobiotic substances. It controls the reduced drug accumulation in cells with multiple drug resistances and frequently controls the emergence of anticancer drug resistance. The well-known membrane transporter P-glycoprotein (P-gp) may reduce the efficacy of chemotherapy by removing medicines from cancer cells [Citation14]. P-gp expression is upregulated in cancer cells as a means of survival during chemotherapy [Citation15]. After identifying posaconazole as a promising lead, posaconazole derivatives were synthesized and evaluated in vitro against A549 lung cancer cell lines in an effort to improve posaconazole's bioactivity profile. In addition, synthesized derivatives have been evaluated by the ADME study, molecular docking, MD simulation of 200 ns, MMGBSA, and DFT calculations.
2. Materials and methods
Currently, structure-guided drug design and medication repurposing have emerged as rapid approaches for identifying new lead candidates that may target a wide range of biological entities. Moreover, the optimization of these leads facilitates the identification of new drug-like candidates. Thus, the current study used a blend of structure-based drug design and drug repurposing methodologies to discover a new lead compound capable of inhibiting ALK tyrosine kinase. The workflow used in the current study is shown in Figure .
The current study included the execution of a molecular docking analysis on posaconazole, which was afterwards followed by the MTT experiment. Based on the results obtained from the MTT assay and in silico ADME analysis, it has been observed that posaconazole exhibits activation as a P-glycoprotein (P-gp) substrate, which is a significant factor contributing to the development of multi-drug resistance. Consequently, it has been determined that the design of several derivatives of posaconazole is necessary to circumvent its tendency to function as a P-gp substrate and to identify novel drug candidates that do not induce multi-drug resistance. Subsequently, the derivatives that were created underwent further evaluation for in silico absorption, distribution, metabolism, and excretion (ADME) examination. It was shown that both benzoyl and benzoyl derivatives did not exhibit any substrate activity for P-glycoprotein (PGP). In conclusion, three compounds, namely acetyl, benzyl and benzoyl derivatives of posaconazole were synthesized and then assessed by MTT assay. To confirm the empirical findings, further computational investigations such as molecular docking, density functional theory (DFT), molecular dynamics (MD) simulation, and molecular mechanics generalized born surface area (MMGBSA) analysis were conducted, yielding encouraging results.
2.1. In-Silico hit indentification by molecular docking
2.1.1. Target preparation
The structure of the ALK TK of interest was obtained from the Protein Data Bank's structural database (https://www.rcsb.org/structure/4CMU). Subsequently, the downloaded structure was integrated into an open-source molecular editor, namely Discovery Studio Visualizer 4.0. The Chimaera, a computational algorithm used at the University of California, San Francisco, utilizes the steepest descent methodology to identify a set of one thousand steps. The best structure for each of the compounds D1, D2, D3, and D4 was determined using the conjugate gradient of energy reduction approaches [Citation16].
2.1.2. Molecular docking
The ALK TK structure (PDB ID: 4CMU) was downloaded from the Protein Data Bank's repository (https://www.rcsb.org/structure/4CMU; accessed March 7, 2022) [Citation16]. PDB: 4CMU was chosen because of its outstanding X-ray clarity and complete sequence coverage. The UCSF Chimaera Tools programme was used to optimize proteins and ligands. UCSF Chimaera used the steepest descent approach to find 1000 steps and then utilized the conjugate gradient energy minimization strategy to optimize the structure of protein 4cmu. The PDB file with the structural data of KDM5A was acquired from the Protein Data Bank at https://www.rcsb.org/structure/4CMU. Both the first and last X-ray images in the series use the pdb:4CMU file. The high resolution of protein 4cmu and the existence of a ligand at the active site, positioned near the protein structure's perimeter, are likely responsible for the strong similarity seen in the ramachandran plots before and after optimization. After optimization, the protein may be used for docking investigations. .To ensure the protein's integrity before executing precise docking simulations, ramachandran was generated before and after optimization (See Figure ). The structure of the ALK TK of interest was obtained from the Protein Data Bank's structural database (https://www.rcsb.org/structure/4CMU).
Subsequently, the downloaded structure was optimized into an open-source molecular editor, namely Discovery Studio Visualizer 4.0. The Chimaera, a computational algorithm used at the University of California, San Francisco, utilizes the steepest descent methodology to identify a set of one thousand steps. The best structure for each of the compounds D1, D2, D3, and D4 was determined using the conjugate gradient of energy reduction approach. This free software is available to the public as a plugin for the PyMOL programme (www.pymol.org). The docking simulations often use FlexAID, a computational tool, to detect surface cavities on proteins. These identified cavities are then utilized as potential binding sites for docking experiments. An additional characteristic is the use of a scoring methodology based on surface complementarity, thereby mitigating excessive dependence on limited geometric criteria. The energy parameters of the scoring method for more than 1500 complexes in the PDB binding database were established by using a substantial dataset consisting of native and near-native conformations with a root-mean-square deviation (RMSD) of less than 2. To address these challenges, a series of Monte Carlo optimization rounds were conducted on more challenging datasets consisting of lower energy decoys with root mean square deviation (RMSD) values above 2. Conformational retrieval, modelling ligand and side chain flexibility, and covalent docking are all accomplished using evolutionary algorithms. A versatile, stiff docking technique was utilized with the following settings in NRGSuite to get the best results: Flexibility of Ligands Ligand is indicated by a reference number. No limits may be set. A rigid side chain and an input method for spherical binding sites. This investigation's technique involves using spherical binding sites with a defined radius of 5 Å, along with side chain stiffness. Since ligands may take on a variety of forms, a numerical identifier was employed to denote them. The HET group's ability to enclose water molecules has no bounds. Van der Wall had a magnetic permeability of 0.1, and no solvent was used. Multiple TOP-5 complexes; 1000 chromosomes; 1000 generations; a sharing fitness model; and a population explosion reproductive strategy. The docking procedure was validated using a well-characterized AKL TK inhibitor of PDB:4CMU.
2.2. MTT assay
The A549 lung cancer cell line was obtained from the National Centre for Cell Science (NCCS) in Pune, India, at passage 68. Following thawing from cryopreservation, the cells were cultured using F-12 K medium supplemented with 10% fetal bovine serum (FBS), an antibiotic/antimycotic solution, HEPES, and the antibiotic/antimycotic solution. The production of insoluble formazan crystals in functioning mitochondria is a result of the reducing properties shown by 3(4,5-dimethyl-thiazol-2-yl)-2,5-diphenyl tetrazolium bromide (MTT). A549 cells were seeded at a density of 1 × 104 cells per well onto a 96-well plate. The plate was thereafter placed in an incubator set at a temperature of 37 degrees Celsius and a carbon dioxide concentration of five percent. The cells were allowed to incubate for a duration of 24 hours. After the medium was removed, the cells were exposed to the chemicals D1, D2, D3, and D4 at concentrations of 5 M, 10 M, 20 M, 40 M, and 80 M for a duration of 24 hours. This experiment was conducted in duplicate. For cytotoxicity assessments, we used a normal murine embryonic fibroblast cell line (BALB/3T3). Standardization was performed using posaconazole (D1). Each well had its media capacity increased to 300 µL. The cells were cultured at 37°C in a 5% CO2 environment for 4 hours after 24 hours, at which time 25 L of MTT solution (5 mg/mL) was added to each well. The absorbance at 570 nm was measured using a Biotek Instrument Epoch Microplate Spectrophotometer after the dissolution of formazan crystals in 100 L of dimethyl sulfoxide (DMSO). The IC50 values were determined by the analysis of a nonlinear plot depicting the percentage of cell inhibition against the logarithm of concentration using GraphPad Prism (version 7). The percentage of growth inhibition was calculated using this formula.
Where AT = Absorbance of Treated Cells (Drug)
AU = Absorbance of Untreated Cells
% Cell Inhibition = 100 – % Cell Viability
2.3. In-silico lead optimization by ADME analysis
In the arduous and resource-intensive phases of drug discovery and development, a multitude of molecular structures undergo comprehensive evaluation based on a wide range of parameters. This evaluation aims to guide the selection of chemicals that should be synthesized, tested, and advanced, with the ultimate objective of identifying compounds with the highest potential to serve as efficacious anticancer medicines for patients. The compounds must have a significant level of biological activity while also demonstrating little toxicity. Taking this into consideration, the current investigation used the SWISS ADME webserver to conduct an ADME analysis on the synthesized drugs, with the objective of assessing their pharmacokinetic effectiveness [Citation17]. Consequently, the first assessment of the posaconazole derivative was conducted to evaluate its absorption, distribution, metabolism, and excretion (ADME) parameters. Subsequently, the synthesis of posaconazole derivatives was carried out based on the clearance seen in the ADME parameter assessment.
2.4. Synthesis
2.4.1. General
Posaconazole was procured from Cellulose Research Labs Pvt ltd, Pune, while sodium hydride, dimethylformamide, dichloromethane, and different solvents were readily accessible via commercial sources. The melting point of the procured posaconazole was determined (Melting point:168–173) and compared with the literature [Citation18]. The principal chemical compounds were procured from reputable suppliers, namely Sigma-Aldrich and Merck laboratories. The reaction courses were observed using thin-layer chromatography (TLC) on silica gel-precoated F254 Merck plates. The plates that had been developed were subjected to examination using ultraviolet lamps with a wavelength of 254 nm. The purification of all substances was achieved by the use of column chromatography. In this study, infrared spectra were obtained using a Fourier-transform infrared spectrometer (Shimadzu model). The melting points were measured using the SRS Optimelt, a melting point device, and the values obtained have not been adjusted or altered. The 1H nuclear magnetic resonance (NMR) spectra were acquired using a 400 MHz Bruker NMR spectrometer with deuterated dimethyl sulfoxide (DMSO-d6) as a solvent. A 100 MHz (for 13C) with deuterated dimethyl sulfoxide (DMSO-d6) as a solvent were acquired using a Bruker NMR spectrometer. Mass spectra for synthesized compounds were recorded using VARIAN 4000 Mass spectrometer with ESI-probe (electrospray ionization). The chemical shifts are expressed in units of δppm. The elemental analysis was conducted using a Perkin-Elmer EAL-240 elemental analyzer.
2.4.2. Synthesis
In a 25-mL round-bottom flask, the substrate (Posaconazole, 1) (0.142 mmol) and sodium hydride (2.84 mmol) were combined in dimethylformamide (6 mL) as the solvent. The reaction mixture was stirred and cooled to ice-cold temperatures. The alkyl halide (2.84 mmol) in dimethylformamide (0.5 mL) was added to the reaction mixture in small portions under ice-cold conditions.
The resulting reaction mixture was stirred at 0°C to room temperature for 8–12 hours. The completion of the reaction was assessed using thin-layer chromatography (TLC) with a mobile phase of methanol and dichloromethane (5:95) and run twice. After completion, the reaction mixture was poured into the crushed ice. The solid product was separated out, filtered, and dried. The crude product was washed with cold methanol to produce the desired products. The scheme of the synthesis is displayed in Figure .
2.5. DFT study (Theoretical Method)
Density functional theory (DFT) is a quantum mechanical approach used to determine the electronic configuration of atoms, molecules, and solids. The system's ground state energy and characteristics are dictated by its electron density according to a theorem. Density functional theory using the 6-311++G** basis set was used to optimize the gas-phase structures of the compounds that were tested [Citation19,Citation20]. The DFT calculation was carried out using the hybrid B3LYP approach, which takes into account both the exact (HF) and DFT exchange using the B3 functional as well as the LYP correlation functional [Citation21–23]. This approach is based on the notion of Becke. Harmonic vibrational frequencies were estimated at the same theoretical level after the converged geometry was obtained to verify that there are no imaginary frequencies at the stationary point. Gaussian 09 was used to perform geometric optimizations and harmonic vibrational frequency analysis on the investigated substances. Quantum chemical descriptors were also computed for the given molecule using the conceptual DFT.
2.6. Molecular dynamic and simulation analysis
Dock complexes for apoprotein as a negative control, the 4CMU-D1 complex as a positive control, and the rest of the complexes of 4CMU with D2, D3, and D3 were simulated for 200 ns using MD on a Desmond 2020.1 from Schrödinger, LLC. The OPLS-2005 force field [Citation24] and an explicit solvent model with SPC water molecules are used in this system [Citation24,Citation25]. To neutralize the overall positive charge, Na + ions were added. Biological conditions were simulated by adding 0.15 M NaCl solutions to the apparatus. The system was first equilibrated for 150 ps with an NVT ensemble before retraining over the ALK-TK protein-four chemical complexes. Following that, a 150-ps equilibration and minimization run using an NPT ensemble was performed [Citation26]. All simulations started with identical starting conditions: 27 degrees Celsius, 1 picosecond (ps) of relaxation time, and 1 atmosphere (atm) of pressure using the Nose-Hoover chain coupling method. The whole simulation process was captured at a frame rate of 2 fs. Barostat pressure control with a relaxation duration of 2 ps was achieved using the Martyna-Tuckerman-Klein chain coupling system [Citation27]. Long-range electrostatic interactions were calculated using the particle mesh Ewald method [Citation28], which relied on the Lennard Jones potential for unbonded vdW and the Coulomb interaction at a cutoff radius of 9. The RESPA integrator was used to calculate the bonded forces at a time step of 2 fs for each trajectory. The last batch lasted for 200 ns during the simulation run [Citation29]. Hydrogen bond counts, root mean square deviations, radii of gyrations, and radial and normal fluctuations were all measured to ensure MD simulations were stable [Citation30].
2.7. Molecular mechanics generalized borne surface area
Using MD simulations of ALK TK complexes of posaconazole and the synthesized compounds D2, D3, and D4 (Schrodinger Suite, LLC., New York, NY 2017-4), we calculated the binding free energy (Gbind) of docked complexes. Utilizing the OPLS 2005 force field, the VSGB solvent model, and rotamer search methods, the binding free energy was calculated. Following the MD simulation, images were extracted from the trajectory at 10-ns intervals. The upper limit on free energy was determined by solving Equation Equation1(1)
(1) .
(1)
(1) Where, ΔGbind = binding free energy, Gcomplex = free energy of the complex, Gprotein = free energy of the target protein, and Gligand = free energy of the ligand.
Post-dynamic changes to the trajectories produced by the MMGBSA were also investigated.
3. Results and discussion
3.1. Chemistry
Following the process of in silico docking, ADME analysis, and MTT test assessment of posaconazole, three derivatives were synthesized. In order to identify a prospective lead compound for combating cancer, an endeavour was undertaken to synthesize three distinct derivatives of posaconazole and evaluate their ability to inhibit the A549 cell lines. Our technique in the first trial was using acetyl chloride to substitute the hydroxyl group in posaconazole. The reaction could not be kicked off with a concentration of acetyl chloride that was two to ten times as high. The reaction time increased, but the desired result was achieved with fifteen equivalents of acetyl chloride. The required product was separated with a satisfactory yield (85%) when twenty equivalents of acetyl chloride were utilized. A range of bases at varying concentrations were utilized, including sodium hydride, sodium hydroxide, potassium hydroxide, and potassium carbonate. Importantly, the reaction couldn't occur normally without the use of a base. The appropriate quantity of base for a displacement reaction was determined to be twenty times as much sodium hydride. Dimethylformamide was discovered to be an acceptable solvent after being tested against other solvents such as methanol, acetonitrile, dichloroethane, and diphenyl ether. Having established these optimum reaction conditions, further posaconazole derivatives using benzoyl chloride and benzyl chloride were investigated. The necessary products were separated at high yields (80% and 79%, respectively) under ideal conditions. The IR spectroscopy, NMR, and mass measurements all corroborated product formation. The absorption peak at 3300 cm−1 in the IR spectra of the parent substrate was attributed to hydroxyl stretching. The 3022 cm−1 peak is associated with aromatic C–H stretching, while the other peaks at 1602, 1527, 770, and 672 cm−1 are indicative of aromatic C = C stretching and bending frequencies. The peak at 1655 cm−1 resembled to carbonyl stretching frequency. The 3300 cm−1 absorption band of hydroxyl stretching frequency was found eliminated in the final product. However, the other absorption peaks appeared at 1705cm−1 and 1696 cm−1 frequency indicated that acetyl and benzoyl group was successfully introduced at product D2 and D3. The formation of the product D2, D3 and D4 was further confirmed by 1H NMR. The acetyl group in product D2 was observed at 1.90 ppm in the 1H NMR spectra. Similarities between the further five peaks at 7.31–7.32 ppm and the singlet at 4.63 ppm and the aromatic proton and methylene proton of the benzyl group proven that product D3 was completely formed. The D4 compound had similarly elevated levels of aromatic protons, between 7.50 and 8.04 ppm. In 13C NMR, additional peaks at 170 ppm and 165 ppm shows C = O group of the product D2 and D3 while peak 75 ppm showed the benzylic carbon in product D4. The others peak from 128.6 to 133.0 ppm demonstrated the aromatic carbon which confirmed the formation of product D3 and D4.
Compound D2
color: white solid, Molecular formula:C38H44F2N8O4, Molecular weight:714.82, M.P. 278–282 °C, IR (KBr) ν cm−1: 3022, 1685, 1602, 1527, 1496, 770, 672; 1H NMR (500 MHz, MeOD): δ 8.29 (s, 1H), 7.98 (s, 1H), 7.68 (s, 1H), 7.35–7.26 (m, 3H), 7.03 (dd, J1 = 9.0 Hz, J2 = 2.0 Hz, 2H), 6.89 (d, J = 7.4 Hz, 3H), 6.81–6.75 (m, 1H), 6.73 (d, J = 9.0 Hz, 2H); δ 5.23–5.16 (m, 1H), 5.06 (dd, J1 = 13.4 Hz, J2 = 7.3 Hz, 1H), 4.62–4.52 (m, 2H), 4.48 (s, 1H), 4.06–4.00 (m, 2H), 3.79–3.58 (m, 4H), 3.27 (t, J = 6.9 Hz, 5H), 3.14–3.07 (m, 5H), 2.83 (d, J = 63.0 Hz, 1H), 2.56–2.40 (m, 3H), 2.09 (dd, J1 = 19.2 Hz, J2 = 15.9 Hz, 2H), 1.83–1.67 (m, 3H). 13C NMR (121 MHz, MeOD): 170.2, 162.2, 158.3, 151.5, 150.2, 148.9, 146.9, 145.6, 145.2, 143.8, 131.3, 122.5, 122.5, 122.3 122.2, 122.0, 118.6, 118.6, 118.5, 114.9, 114.9, 114.6, 113.0, 113.0, 112.4, 110.9, 104.5, 78.9, 71.5, 70.8, 69.1, 65.3, 49.4, 49.4, 37.6, 37.6, 21.0, 16.2, 14.1; M+/Z: 700–710 (100%), 670–680(40%), 690(10%), 720–725 (40%), 740–750 (45–50%). Elemental Analysis: C, 63.51; H, 6.07; F, 5.41; N, 15.89; O, 9.19.
Compound D3
Color: creamy white solid, Molecular formula:C44H46F2N8O5, Molecular weight:804.90, M.P. 269–272°C, IR (KBr) ν cm−1: 3022, 1685, 1680, 1527, 1496, 770, 672; 1H NMR (500 MHz, MeOD): δ 8.29 (s, 1H), 7.98 (s, 1H), 7.68 (s, 1H), 7.69 (3H, J1 = 8.3 Hz, J2 = 1.6 Hz), 7.35–7.26 (m, 5H), 7.03 (dd, J1 = 9.0 Hz, J2 = 2.0 Hz, 2H), 6.89 (d, J = 7.4 Hz, 3H), 6.81–6.75 (m, 1H), 6.73 (d, J = 9.0 Hz, 2H); δ 5.23–5.16 (m, 1H), 5.06 (dd, J1 = 13.4 Hz, J2 = 7.3 Hz, 1H), 4.62–4.52 (m, 2H), 4.48 (s, 1H), 4.06–4.00 (m, 2H), 3.79–3.58 (m, 4H), 3.27 (t, J = 6.9 Hz, 5H), 3.14–3.07 (m, 5H), 2.83 (d, J = 63.0 Hz, 1H), 2.56–2.40 (m, 3H), 2.09 (dd, J1 = 19.2 Hz, J2 = 15.9 Hz, 2H), 13C NMR (121 MHz, MeOD): 170.2, 162.2, 158.3, 151.5, 150.2, 148.9, 146.9, 145.6, 145.2, 143.8, 133.0, 131.3, 129.9, 129.9, 128.6, 128.6, 122.5, 122.5, 122.3 122.2, 122.0, 118.6, 118.6, 118.5, 114.9, 114.9, 114.6, 113.0, 113.0, 112.4, 110.9, 104.5, 78.9, 71.5, 70.8, 69.1, 65.3, 49.4, 49.4, 37.6, 37.6, 21.0, 16.2, 14.1; M+/Z : 800–810(100%), 810–820 (35%), 820–830 (45%). Elemental Analysis: C, 66.72; H, 6.17; F, 4.82; N, 14.14; O, 8.07.
Compound D4
Color: Yellowish white solid, Molecular formula: C44H48F2N8O4, Molecular weight:790.92, M.P. 265–267°C, IR (KBr) ν cm−1: 3022, 1685, 1527, 1496, 779, 642; 1H NMR (500 MHz, MeOD): δ 8.29 (s, 1H), 7.98 (s, 1H), 7.68 (s, 1H), 7.69 (3H, J1 = 8.3 Hz, J2 = 1.6 Hz), 7.35–7.26 (m, 10H), 7.03 (dd, J1 = 9.0 Hz, J2 = 2.0 Hz, 2H), 6.89 (d, J = 7.4 Hz, 3H), 6.81–6.75 (m, 1H), 6.73 (d, J = 9.0 Hz, 2H); δ 5.23–5.16 (m, 1H), 5.06 (dd, J1 = 13.4 Hz, J2 = 7.3 Hz, 1H), 4.62–4.52 (m, 4H), 4.48 (s, 1H), 4.06–4.00 (m, 2H), 3.79–3.58 (m, 4H), 3.27 (t, J = 6.9 Hz, 5H), 3.14–3.07 (m, 5H), 2.83 (d, J = 63.0 Hz, 1H), 2.56–2.40 (m, 3H), 2.09 (dd, J1 = 19.2 Hz, J2 = 15.9 Hz, 2H), 13C NMR (121 MHz, MeOD): 170.2, 162.2, 158.3, 151.5, 150.2, 148.9, 146.9, 145.6, 145.2, 143.8, 133.0, 131.3, 129.9, 129.9, 128.6, 128.6, 127.4, 127.4, 127.4, 126.8, 126.8, 122.5, 122.5, 122.3 122.2, 122.0, 118.6, 118.6, 118.5, 114.9, 114.9, 114.6, 113.0, 113.0, 112.4, 110.9, 104.5, 78.9, 71.5, 70.8, 69.1, 65.3, 49.4, 49.4, 37.6, 37.6, 21.0, 16.2, 14.1; M+/Z : 780–800 (100%) 800–810 (40%) 810–820(10%). Elemental Analysis: C, 65.46; H, 5.45; F, 4.77; N, 13.92; O, 9.89. (see supplementary file S1, S2 and S3 for spectra data)
3.2. Design of novel derivatives of posaconazole
Based on the existing published result and the in-silico molecular docking observation, an identified lead drug (posaconazole) has been screened for MTT assay in an effort to develop a new anticancer agent targeting ALK TK. Additionally, the compound was subjected to ADME studies in order to assess its potential for inducing resistance. Based on the ADME results, posaconazole acts as a P-gp substrate, and resistance to it is possible. Consequently, a decision has been made to synthesize a limited number of posaconazole derivatives, commencing with substituents of varying bulkiness such as acetyl, benzyl, and benzoyl. Subsequently, an investigation involving in-silico molecular docking and ADME analysis will be conducted to elucidate the issue pertaining to posaconazole's interaction with P-glycoprotein as a substrate. Three derivatives were synthesized and afterward subjected to an MTT assay to evaluate their potential anticancer efficacy. Furthermore, comprehensive ADME research was conducted to address the concern of P-gp substrate interaction, including the screening of all derivatives. P-gp substrate activity is not seen in two posaconazole derivatives containing substituents, benzyl, and benzoyl. In addition, DFT analysis was used to identify probable pharmacophore sites in the synthesized derivatives, MD simulation was used to verify the in-silico docking investigation, and MMGBSA analysis was used to calculate the binding free energy of the dock complex (See Figure for the workflow).
3.3. Biology (MTT assay)
The A549 cell line is a widely used human non-small cell lung cancer cell line in the fields of basic research and drug development. A549 cells are human alveolar basal epithelial cells derived from adenocarcinoma. The cells were first created in 1972 by extracting and cultivating malignant lung tissue from the removed tumour of a 58-year-old Caucasian man [Citation31]. The cytotoxicity of compounds D1-D4 was evaluated in vitro using the A-549 lung cancer cell line. An MTT assay was conducted to assess the potential therapeutic effects of these compounds on the illness. The morphological alterations in the cells throughout the drug treatment phase are shown in Figure . At a dose of 80 µM, the morphology of each sample underwent full alteration. Figure illustrates the bar plot representing the cell viability observed in the MTT experiment. The IC50 values for compounds D1-D4 are shown in Figure , with a range of 6.633 to 18.58 µM. The IC50 values for compounds D1-D4 on normal murine embryonic fibroblast cell line (BALB/3T3) were on higher side than the anticancer effects achieved on A-549 cells, indicating more selectivity towards cancer cells than the normal once (10.8, 19.7, 19.4, 81.3 µM, respectively). The selectivity indexes are given in the Table 2. The selectivity index (SI) was calculated to evaluate the toxicity of the compounds studied against normal cells and to predict their therapeutic potential (see Table 2). High SI values result from large differences between the cytotoxicity against cancer and normal cells and this means that cancer cells will be killed at a higher rate than normal ones. For studied compounds, we retained higher SI for D4 analogue with SI: 4.37.
Figure 4. Depiction of morphological changes in A549 cell lines in MTT assay for the synthesized posaconazole derivatives: D2, D3 and D4.
Figure 5. Determination of % cell viability by MTT assay. Cell viability was determined using the MTT assay for A549 cells treated for 24 hrs. with compounds; D1, D2, D3 and D4 at concentrations of 0 µM, 5 µM, 20 µM, 80µM (a) cell viability of Compound D1 (b) cell viability of Compound D2 (c) cell viability of Compound D3 (d) cell viability of Compound D4.

Figure 6. Depiction of figure for the determination of IC50 values for the Posaconazole (D1) and its synthesized compounds; D2 (Acetyl derivative), D3 (Benzyl derivative), and D4 (Benzoyl derivative).

Compound D1 was used as a reference compound, exhibiting the lowest IC50 value in comparison to its derivatives. Consequently, compound D1 is considered to possess the highest potency, whereas compounds D2 through D4 are considered to have equivalent efficacy against lung cancer cell lines. Please refer to the supplemental material for the findings of the MTT experiment (see supplementary file S4 for MTT data).
3.4. DFT analysis
It is more appropriate to investigate the electron density distribution inside the highest occupied molecular orbital (HOMO) of a nucleophile rather than putting a focus on the electron density as a whole in a nucleophile. One possible explanation for this occurrence is that electrons that originate from the highest occupied molecular orbital (HOMO) have a greater tendency to participate in the course of nucleophilic assaults. On the other hand, the distribution of electron density at a site's lowest unoccupied molecular orbital (LUMO) may be used to determine whether or not the site is suitable for electrophilic reactivity. This is seen in Table .
Table 1. The EHOMO, ELUMO, and ΔEg in eV of the studied compounds.
A visual representation of the geometric configurations of the compounds under investigation, which were successfully optimized via the use of the B3LYP methodology, may be seen in Figure .
Figure 7. The B3LYP-optimized geometries of the Posaconazole (A) and its synthesized acetyl(B), benzoyl (C) and benzyl (D)derivatives.

The current investigation conducted a more detailed analysis of the frontier molecular orbitals of the compounds referenced in the article. The nucleophilic sites for all the compounds being studied are situated at the 4-(piperazine-1-yl)-phenolic moiety, as seen in Figure . In addition, it is worth noting that the electrophilic sites of the compounds indicated above are mostly located on the fluorophenyl fragment, except for the posobenzoyl derivative under investigation. However, it is important to mention that the benzoyl fragment functions as the electrophilic site for the investigated posobenzoyl derivative.
Figure 8. Presentation of HOMO and LUMO of the studied Posaconazole (A) and its Acetyl (B), Benzoyl (C), and Benzyl (D) derivatives compounds (the isovalue = 0.02 a.u.).

The determination of nucleophilic and electrophilic regions inside a molecule may be achieved by taking into account both its border orbital and its molecular electrostatic potentials (MEPs). The assessment of the intensity of localized interactions between charges, nuclei, and electrons relies heavily on the molecular electrostatic potential (MEP). This study offers significant observations on the spatial arrangement of charges and charge-related characteristics inside molecules. In order to augment the comprehensibility of electrostatic potential data, a visual depiction using a spectrum of colours is used. Typically, the colour red is representative of the molecule's lowest electrostatic potential value, which often aligns with the nucleophilic site. On the other hand, the hue blue is suggestive of holding the highest amount of electrostatic potential and might possibly function as the electrophilic site inside a molecule. The molecular electrostatic potential (MEP) is determined by calculating the whole density matrix of the substance being examined, which is then shown graphically on the surface of the molecule.
As seen in Figure , the compounds under investigation do not demonstrate a localized concentration of either the nucleophilic or electrophilic site on any particular moiety. The discrepancy seen between the results of the border orbitals and the molecular electrostatic potentials (MEPs) may be ascribed to the recognition that the MEP of a molecule is affected not just by the electrons but also by the atomic nuclei present inside the molecule. Also, it was seen that fluorine atoms had a bit less nucleophilicity than other elements in the compounds being studied. However, fluorine atoms had the highest electronegativity of all the atoms in the compounds mentioned in the title.
3.5. Molecular docking analysis
The examination of ALK's amino acid sequences has substantiated its classification as a newly identified receptor tyrosine kinase (RTK). This RTK has an external ligand-binding domain consisting of 1030 amino acids, a transmembrane region spanning 28 amino acids, and an intracellular tyrosine kinase domain encompassing 561 amino acids [Citation32–34]. Evident parallels exist between the tyrosine kinase domain of the human ALK protein and the intracellular area of the insulin receptor. This receptor tyrosine kinase (RTK) family member's extracellular domain is characterized by its unique composition, consisting of two MAM domains (meprin, A5 protein, and receptor protein tyrosine phosphatase mu), an LDLa domain (low-density lipoprotein receptor class A), and a glycine-rich region. Additionally, ALK's ATP binding site consists of 27 residues [Citation33–35].
Particular divergent residues of ALK were focused on to enhance its specificity towards other kinases. Most kinases at this location contain a phenylalanine (Phe) or tyrosine (Tyr) residue, but the ALK Leu1198 residue is present in 26% of all kinases. By extending into this particular pocket and interacting with the bigger Phe and Tyr residues, the smaller Leu residue may demonstrate selectivity against around 60% of kinases [Citation16].
The Protein Data Bank (PDB) supplied the pdb file for the ALK tyrosine kinase, which has a resolution of 1.80 Å. The selection of PDB 4CMU for this study was based on its superior X-ray resolution and comprehensive sequencing. The protein 4cmu was prepared by using the UCF Chimaera software. The preservation of water molecules was maintained throughout the protein production procedure. The attainment of a strong binding affinity towards a certain protein target is of utmost importance in the field of drug design since it plays a crucial role. The actual execution of calculating binding free energy using formal mathematical equations grounded in statistical mechanics presents notable problems. The task of capturing solvation effects presents significant challenges, especially when the observed influence is ascribed to an individual water molecule rather than the collective behaviour shown by water in larger quantities. The ability to completely prevent these minute impacts is considered to be unachievable. When you look at the structures in the Protein Data Bank (PDB), you can see that each complex formed by a ligand and a protein has a different number of water molecules attached to it, usually between 4 and 6. Furthermore, water not only functions to sustain ligand interactions but also plays a vital role in defining specificity in biological systems. Therefore, the protein that has been augmented with water molecules was considered appropriate for performing docking analysis [Citation34].
The active site, which is where the natural ligand is bound, has been investigated here. As a prerequisite to the docking study, the inhibitor (10R)-7-amino-12-fluoro-1, 3, 10, 16-tetramethyl-1H-8, 4-(metheno) pyrazolo(4,3-h) (2,5,11) benzoxadiazacyclotetradecin-15(10H)-one was removed from the active site. The natural ligand is being studied at its binding location (called the active site). Posaconazole and its acetyl, benzoyl and benzyl derivatives, as an example, are illustrated in their docking posture between the active site and the region of ALK tyrosine kinase where the native ligand was initially bound orthosterically.
When docking with the ALK TK pdb:4cmu ligand, all of the posaconazole derivatives and posaconazole seem to have scores that are equivalent to one another (see Table ). It is interesting to note that the posaconazole exhibited a hydrophobic contact with the residues Ala1148, Leu1156, Leu1196, and Val1130 by means of its terminal 1,2,4-triazole ring substituent. Furthermore, posaconazole displayed one hydro-gen bonding connection with Lys1294 residue and one halogen bond with Lys1122 via its fluorine atom on the terminal phenyl ring directly beside the 1,2,4-triazole ring. Both of these interactions were seen in the presence of another halogen bond. In a remarkable turn of events, Leu1196 is a residue that is often mutated, and as a consequence, it has an impact on the structural stability of proteins, the affinity of proteins for small molecules, and the resistance of cancer patients to drugs [Citation36]. Due to the fact that the majority of the process takes place in an aquatic environment, the halogen bonds that forms with water would play a significant impact. It has previously been shown via quantum chemistry research that halogen bonds that are formed with water are more thermodynamically stable than other interactions that include water [Citation37].
Table 2. Presentation of the docking score for the pdb:4cmu ligand, Posaconazole, and its synthesized derivatives.
Next, compound B (acetyl posaconazole) interacted with ALK TK via many types of contacts including water hydrogen bond, conventional hydrogen bond, carbon hydrogen bind, halogen bond, pi-lone pair link, alkyl hydrophobic bond, and pi-alkyl hydrophobic interactions with a docking score of −8.5814 kcal/mol (RMSD:2.93 Å). The bioactivity profile of the acetyl derivative is influenced by electrostatic and hydrophobic interactions, as shown in this interaction. There are six water molecules, namely HOH2102, HOH2063, HOH2056, HOH2147, HOH2151, and HOH2155, involved in hydrogen bonding with water, providing substantial stability to the drug receptor complex. Additionally, residues ASP1203 and ASN1335 participated in traditional hydrogen bonding with acetyl derivatives. Additionally, acetyl posaconazole formed hydrophobic interactions with Leu1256, Ala1148, Met1290, AL1126, and Leu1122 residues via alky and pi-alkyl contacts. The chemical 172 interacts to the ALK tyrosine kinase and drug receptor complex by several interactions such as typical hydrogen bonds, carbon–hydrogen bonds, pi-pi cation contacts, alkyl hydrophobic interactions, and pi-alkyl hydrophobic interactions.
Compound C (benzyl posaconazole) bonded to the ALK TK by forming five water hydrogen bonds, two conventional hydrogen bonds, seven alkyl and pi-alkyl hydrophobic interactions, a pi-cation interaction, a pi-lone pair interaction, and carbon hydrogen bonds. Further, the water molecules namelyHOH2159, HOH2148, HOH2147, HOH2102, and HOH2056 were participated in water hydrogen bonding with benzyl posaconazole. Consequently, benzyl posaconazole depicted two hydrogen bonds with the Lys1294 and Asn1335 residues. Furthermore, the residues such as Met1329, Ala1289, Ala1126, Ala1148, Ala1256, Leu1122, and al1130 were exhibited alkyl and pi-alkyl hydrophobic contacts with the benzyl posaconazole. Next, two water molecules; HOH2102 and HOH2056 displayed pi-lone pair contacts which are a type of noncovalent interaction that involves the stabilizing association of a lone pair of a Lewis basic atom with a π system. Lone pair–π interactions are commonly attributed to electrostatic forces. This observation indicates that the bioactivity of benzyl posaconazole is significantly influenced by interactions involving water hydrogen bonds, alkyl groups, and pi-alkyl hydrophobic contacts.
In addition to this, the benzoyl derivative exhibits a fascinating alkyl hydrophobic interaction with ASP1249, which is located in the area that forms ATP binding region. The benzoyl posaconazole's hydrophobic nature disturbs the water structure and reduces entropy by promoting stronger bonding and ordering of water molecules around it. The aggregation of nonpolar solute molecules may minimize disruptions. The fluorine-substituted benzene ring that is part of the active “DFG-in” conformation of acetyl posaconazole occupies the first ATP binding pocket and forms a halogen bond with the GLU1197 residue. This conformation is responsible for the active state of the compound. Furthermore, the benzyl substituent that was in the active “DFG-in” conformation demonstrated a carbon–hydrogen bonding interaction with MET1199. According to the research that has been conducted, the residues Lys1150, Glu1167, Asp1249, and Asp1270 (the K/E/D 109 signature) in the ATP binding region are the primary sites where the catalytic activity of ALK is concentrated. Furthermore, the in-silico data are supported by the experimental validation that was performed against the A594 lung cancer cell line. Therefore, the suppression of the A549 lung cancer cell line by experimental means was shown to be predominantly connected with the inhibition of ALK TK, which was supported by in silico molecular docking (see Figure ).
Figure 10. Presentation of 2D & 3D interactions of Posaconazole (A) and its synthesized acetyl (B), benzoyl (C) and benzyl (D) derivatives.

According to the docking study, there is a clear difference between how posaconazole and its acetyl, benzyl, and benzoyl deriatiess bind to molecules. The observed binding pattern of the synthesized derivative was consistent with the IC50 value observed in the MTT assay. Similar to what was found in the ADME study, the molecular docking analysis shows that posaconazole derivatives and Leu1196 do not interact with each other. This phenomenon is often associated with genetic alterations.
3.6. Molecular dynamic and simulation analysis
Molecular dynamics (MD) simulations are used to look at ALK TK proteins in both their apo and complexed forms to see how stable, fluctuating, compact, and able to change shape they are. The apo and complexed states of ALK TK-4CMU with Posaconazole and its acetyl, benzyl, and benzoyl derivatives were simulated using molecular dynamic simulation. Both the apo and complexed states were evaluated using these two systems. A 200 ns molecular dynamics (MD) manufacturing run was successfully conducted on both 4CMU and posaconazole complexes, along with their respective derivatives.
The root mean square deviation (RMSD) is a statistical measure used to determine the typical deviation of a set of atoms from a reference frame. At 200 ns of simulation time, the c-alpha backbone of the ATK TK (PDB I.D. 4CMU) in the Apo state, also known as the uncomplex state, had a deviation value of 0–2.5 Å. Following complexation, a significant discrepancy has been observed in the reduction of the root mean square deviation (RMSD) values, specifically 0–1.9 Å and 0–2.1 Å for the benzoyl and benzyl derivative-bound ALK TK, respectively. Conversely, the acetyl derivative-bound ALK TK (PDB I.D. 4CMU) exhibited a comparable deviation of 2.5 Å to that of the APO state of ALK TK. It is noteworthy that an observation was made regarding the posaconazole-bound ALK TK (PDB I.D. 4CMU), whereby an elevation in the root mean square deviation (RMSD) value of 0–2.8 Å was identified. It is notable that, in comparison to posaconazole and its acetyl derivative, the root mean square deviation (RMSD) of the protein bound to the benzyl and benzoyl derivatives is lower, suggesting a significant increase in stability (see Figure ).
Figure 11. MD simulation trajectory analysis of Root Mean Square Divisions (RMSD) of control protein ALK TK(Apo) (red colour), Posaconazole (golden colour) and its acetyl (magenta), benzyl (parrot green), and benzoyl (teal colour) derivatives bound with 4CMU, i.e., ALK TK at 200 ns time frame.

The RMSD maps, when compared before and after simulation, show that the ALK-TK protein is stable in its bound state to posaconazole and its derivatives. In sum, the data suggest that the high degree of binding between posaconazole and its derivatives and ALK TK (PDB I.D. 4CMU) may account for the complex's remarkable stability.
It can figure out how a protein folds and how compact it is by guessing its radius of gyration (Rg) in both its apo and complexed forms. When a protein is folded, its constituent subunits are tightly packed, but when it is unfolded, its constituent subunits are more loosely packed, leading to a less stable conformation and larger radius of gyration values. Radius of gyration (Rg) was slightly increased in ALK TK proteins that were bound to posaconazole (Rg_APO_ALK TK: 23.42 Å, Rg posaconazole bound ALK TK: 26.23 Å) and its acetyl (Rg: 26.23), benzyl (Rg: 24.66) derivatives. Interestingly, the posaconazole benzoyl (Rg: 20.04) derivative has a smaller radius of gyration. A smaller Rg value suggests a more tightly packed protein–ligand complex. In comparison to posaconazole, its acetyl derivative, and its benzyl derivative, the benzoyl derivative was shown to be more compact in its complex form. We can see from the RMSD and Rg quality scores that the binding of posaconazole and its derivatives to the protein target ALK TK in the binding cavities has a big effect on how stable the proteins are. The root mean square fluctuation (RMSF) is an MD simulation parameter that may be used to determine the average variation of protein residues over an all-atom-based simulation. The RMSF was carried out on the apo and complex carbon atoms of the backbone (see Figure ).
Figure 12. MD simulation trajectory analysis of Root Mean Square fluctuation (RMSF) of control protein ALK TK(Apo) (blue colour), Posaconazole (red colour) and its acetyl (parrot green), benzyl (pink colour), and benzoyl (black) derivatives bound with 4CMU, i.e., ALK TK at 200 ns time frame.

Conformational fluctuations in the apo state (when the ALK TK (PDB:4CMU) domain does not bind to the ALK TK) were seen to be within the typical range of 3–4 Å, as shown by the figure. However, in the complex state, an alteration in the peak was observed. There were clear changes in the ALK TK protein domain's conformation and configuration when it was linked to posaconazole and its derivatives in the MD simulation study. These changes tend to happen less often in the complex state of the protein–ligand interaction. Posaconazole's RMSF plot shows that fluctuations in the c-alpha chain diminish between positions 25 and 50, 95 and 105, 180 and 200, 230 and 240, and 285 and 295, respectively. The acetyl derivative also showed reduced fluctuation in the first 25–30, 180–195, and 225–235 residues. In addition, minor alterations in the RMSF were detected at residues 50 and 100. For benzoyl derivatives, the RMSF changed for residues between 25 and 120, 90 to 120, 150 to 200, and close to 280. For residues between 25 and 100, 150 to 200, and 250 to 260, it went down for benzyl derivatives. Thus, on the basis of variations in RMSF, it can be concluded that posaconazole and its acetyl, benzyl, and benzoyl derivatives were closely connected with the c-alpha chain of ALK TK. The degree of variation was first evident around residues 150–200, according to the RMSF study as a whole.
3.7. Molecular mechanics generalized borne surface area (MMGBSA)
The MM/GBSA method computes the total binding free energy of a molecular complex. This is achieved by adding up the gas-phase energy, solvation free energy, and entropic contributions. The MM-GBSA score quantifies the total free energy associated with a certain protein–ligand interaction. The binding free energy is the difference in free energy between the bound and totally unbound states. The bound state is a clearly defined complex state, whereas the totally unbound state may include any states where interactions between a protein and ligand are minimal. MMGBSA was performed to find the binding free energy (ΔG) between posaconazole and its different forms and the ALK TK protein. The calculation of the binding free energy ΔG values was performed by using the last 50–100 nanoseconds of trajectory data. The average binding free energy values were for posaconazole: −70.36, acetyl: −61.90 kcal/mol, benzyl: 62.19 kcal/mol, and benzoyl: −69.56 kcal/mol derivatives (see Table ). Among the three derivatives examined, the benzoyl derivative showed the highest binding affinity to ALK TK based on the ΔG values, followed by the acetyl and benzyl derivatives. However, the benzoyl derivative showed the highest affinity based on the MM-GBSA calculations. The only time a noncovalent binding event occurs is when it is associated with a negative binding free energy (ΔG), which is the well-known sum of an enthalpic term (ΔH) and an entropic term (-TΔS). This is the case with any other spontaneous activity as well. An increase in affinity of 2.1 kcal/mol may be achieved in benzoyl posaconazole was achieved by the inclusion of the lipophilic benzoyl side chain. There is a −2.55 kcal/mol increase in affinity in comparison to posaconazole with respect to the ΔGbindLipo that occurs when the benzoyl group is added in posaconazole [Citation38].
Table 3. Depiction of MMGBSA results for the 4cmu-Posaconazole, 4cmu-acetyl, 4cmu-benzyl and 4cmu-benzoyl derivative complexes.
The binding energy of posaconazole and its synthetic acetyl, benzyl, and benzoyl derivatives is investigated on a residue-by-residue basis. Upon examination of the energy contributions of each residue to the binding of posaconazole, it was seen that the residue with the greatest ΔG value was ASN1178 (−63.17 kcal/mol). This was then followed by GLN1364 (−62.45 kcal/mol), ASN1353 (−62.17 kcal/mol), and GLN1188 (−61.31 kcal/mol). The amino acid residues ASN1178 and ASN1353 exhibit ΔG values of −63.37 and −60.72 kcal/mol, respectively. Notably, these residues have a substantial role in contributing to the binding free energy, especially in the context of acetyl derivatives, particularly since they provide the biggest entropic contribution. This is an important aspect of their activity. Next in line were GLN1238 and ASN1254, both of which had ΔG values of −61.44 and −61.09 kcal/mol, respectively. These residues were following in their footsteps. When it comes to benzyl derivatives, ASN1178 once again emerges as a possible energy donor to the binding free energy. It has a ΔG value of −69.34 kcal/mol, which is followed by GLN1159, ASN1254 once again, and GLN1188, which have ΔG values of −62.8 kcal/mol, −61.67 kcal/mol, and −60.67 kcal/mol, respectively. The amino acid residue ASN1178, which has ΔG values of −66.22 kcal/mol, is the primary contributor to the energy of the benzoyl derivative. This is followed by the other amino acid residues, such as GLN1159 (−63.07 kcal/mol), ASN1254 (−63.07 kcal/mol), and GLN1188 (−61.23 kcal/mol). Overall, the benzoyl derivative has a significant amount of energy. In light of the aforementioned discovery, it has been shown that the amino acid residues ASN1178, GLN1188, ASN1154, and GLN1159 play a substantial part in contributing to the binding free energy pattern of posaconazole and its acetyl, benzyl, and benzo-yl derivatives. This is the case because these residues are responsible for a considerable amount of the binding energy.
3.8. ADME evaluation
During the first phases of pharmaceutical development, it may be feasible to identify potential candidates with a less crucial ADME profile by using predictive methods to assess their absorption, distribution, metabolism, and elimination (ADME) characteristics prior to their synthesis. The preference for in vitro in-silico ADME analysis over in vivo ADME assessment is primarily driven by its superiority in terms of cost, time, and ethical considerations. In this study, the SWISS ADME webserver was used to perform an ADME analysis on the synthesized compounds with the aim of evaluating their pharmacokinetic efficacy. The predicted ADME characteristics of posaconazole and its derivative were rather promising.
The most important thing is to look at the agent's physiochemistry, pharmacokinetics (including absorption, distribution, metabolism, and excretion), and toxicity. These may be more important than just how potent it is. Contrary to expectations, it is often feasible to improve in vitro potency at the expense of other physio-chemical properties like high molecular weight and increased lipophilicity [Citation39]. Posaconazole and its acetyl derivative had the highest GI absorption of all the drugs tested. The ratio of WlogP to tPSA revealed that posaconazole and its acetyl derivative had the greatest GI absorption of all the compounds tested. It's worth noting, however, that none of these compounds can penetrate the brain's barrier. It seems that highly lyophilic substituents, such as benzyl, benzoyl, and acetyl, prevent any synthesized derivative from passing the blood–brain barrier. Although P-glycoprotein (P-gp) has been demonstrated to be overexpressed in multi-drug-resistant malignancy, benzyl and benzoyl substituted posaconazole do not function as P-gp substrates [Citation40].
Permeability glycoprotein (P-gp), commonly known as multidrug drug resistance protein (MDR), is found in the small intestine, the primary region for the epithelial absorption of many orally ingested drugs [Citation41]. Some anticancer medicines have been demonstrated to have decreased oral bioavailability due to P-gp. Concomitant dosing of some of the antineoplastic medicines produces overexpression of P-gp, reducing the bioavailability of certain of these medications, like Imatinib. The bioavailability of some antineoplastic drugs, such as paclitaxel, varies across individuals owing to differences in P-gp expression in the gut, which might be caused by genetic polymorphism or pathologic state [Citation42]. This finding has important implications for the development of new cancer drugs, since benzyl and benzoyl derivatives may be employed to combat the problem of drug resistance.
The possibility of CYP450-mediated biotransformation may also be predicted using SwissADME. Benzyl and benzoyl substitutes were shown to interact with three of the five CYP450 isoforms; acetyl-substituted compounds displayed interaction with four of the isoforms; and posaconazole interacted with all five. So, this study shows that the pharmacokinetic properties of the benzyl and benzoyl substituents were better than those of the acetyl derivative and posaconazole. Furthermore, substituents like benzyl and benzoyl may be maintained in future medication optimization.
When screening potential new drugs at an early stage in the drug discovery and development process, drug likeness is an important criterion to consider. According to its older definition, “drug-likeness” evaluates qualitatively a molecule's potential to develop into an oral medication in terms of bioavailability. Two Lipinski violations were present in each of the three derivatives, while two Lipinski violations were present in posaconazole. Before the synthesis or advance testing of compounds for bioavailability and permeability, the ABS score must be greater than 10% in the rat (see supplementary material file S5 for ADME results).
4. Conclusions
The current research identified the antifungal agent: posaconazole as a promising candidate for inhibiting ALK tyrosine kinase by molecular docking analysis, with a docking score of −8.71 kcal/mol. The in silico docking studies were confirmed using MTT testing on A549 cell lines, indicating its potential as a promising therapeutic option for targeting ALK tyrosine kinase specifically. ADME investigation revealed a problem with posaconazole acting as a Pgp substrate. Consequently, we have designed three derivatives and evaluated them for ADME analysis in which two of the derivatives did not demonstrate Pgp substrate activation. Three derivatives, namely acetyl, benzyl, and benzoyl derivatives, were synthesized and their structures were determined using IR, 1H NMR, 13C NMR, MASS spectroscopy, and elemental analysis. Docking study shows that the acetyl, benzyl, and benzoyl forms of posaconazole have unique binding properties. DFT analysis provides important insights into the spatial distribution of charges and charge-related properties inside molecules. The IC50 values from the MTT test support the docking results. The results of molecular docking and ADME investigations suggest that posaconazole derivatives do not interact with Leu1196, a residue often associated with mutations. The molecular dynamics simulation showed that the protein had lower root mean square deviation (RMSD) while interacting with benzyl and benzoyl derivatives compared to posaconazole and its acetyl derivative. The present research is focused on developing benzyl and benzoyl posaconazole, a novel derivative of posaconazole, as a potential lead therapeutic candidate for targeting ALK tyrosine kinase in cancer therapy.
Author contributions
Rahul D. Jawarkar, Praveen Sharma, Sachin Kumar Jain: conceptualization, editing and formal analysis. Umang Shah, Magdi E.A. Zaki, Sami-AL-Hussain: Conceptualization, editing and review, Formal Analysis and Review. Suraj Mali4, Chin-Hung Lai, Vijay Masand, Vivek Humane; editing, formal analysis.
Supplemental Material
Download Zip (1.2 MB)Acknowledgments
The author Rahul D. Jawarkar is thankful to Dr. R. K. Jain, Dean, Oriental university, Indore for providing extended facility during entire course of research work.
Data availability statement
Data can available upon request to the Journal.
Additional information
Funding
References
- Liang X, Yang Q, Wu P, et al. The synthesis review of the approved tyrosine kinase inhibitors for anticancer therapy in 2015–2020. Bioorg Chem. 2021;113:105011. doi:10.1016/j.bioorg.2021.105011
- Li Y, Lv Y, Zhang C, et al. Recent advances in the development of dual ALK/ROS1 inhibitors for non-small cell lung cancer therapy. Eur J Med Chem. 2023;257:115477. doi:10.1016/j.ejmech.2023.115477
- Jones GS, Baldwin DR. Recent advances in the management of lung cancer. Clin Med. 2018;18(Suppl 2):s41–s46. doi:10.7861/clinmedicine.18-2-s41
- Rodriguez-Canales J, Parra-Cuentas E, Wistuba II. Diagnosis and Molecular Classification of Lung Cancer. Lung Cancer. Cancer Treatment and Research. 2016;170:25–46. doi:10.1007/978-3-319-40389-2_2
- Golding B, Luu A, Jones R, et al. The function and therapeutic targeting of anaplastic lymphoma kinase (ALK) in non-small cell lung cancer (NSCLC). Mol Cancer. 2018;17(1). doi:10.1186/s12943-018-0810-4
- Schneider JL, Lin JJ, Shaw AT. ALK-positive lung cancer: a moving target. Nature Cancer. 2023;4(3):330–343. doi:10.1038/s43018-023-00515-0
- Gainor JF, Dardaei L, Yoda S, et al. Molecular mechanisms of resistance to first- and second-generation ALK inhibitors inALK-rearranged lung cancer. Cancer Discov. 2016;6(10):1118–1133. doi:10.1158/2159-8290.CD-16-0596
- Wu W, Haderk F, Bivona T. Non-canonical thinking for targeting ALK-fusion Onco-proteins in lung cancer. Cancers (Basel). 2017;9(12):164. doi:10.3390/cancers9120164
- Pushpakom S, Iorio F, Eyers PA, et al. Drug repurposing: progress, challenges and recommendations. Nat Rev Drug Discovery. 2018;18(1):41–58. doi:10.1038/nrd.2018.168
- Shankar E, Subramaniam V, Allimuthu D. Editorial: Adopting drug repurposing to overcome drug resistance in cancer. Front Cell Dev Biol. 2023;11:1191682. doi:10.3389/fcell.2023.1191682
- Lee W-H, Loo C-Y, Ghadiri M, et al. The potential to treat lung cancer via inhalation of repurposed drugs. Adv Drug Delivery Rev. 2018;133:107–130. doi:10.1016/j.addr.2018.08.012
- Gerber DE, Putnam WC, Fattah FJ, et al. Concentration-dependent Early Antivascular and Antitumor Effects of Itraconazole in Non–Small Cell Lung Cancer. Clin Cancer Res. 2020;26(22):6017–6027. doi:10.1158/1078-0432.CCR-20-1916
- Chen B, Trang V, Lee A, et al. Posaconazole, a second-generation Triazole antifungal drug, inhibits the hedgehog signaling pathway and progression of basal cell carcinoma. Mol Cancer Ther. 2016;15(5):866–876. doi:10.1158/1535-7163.MCT-15-0729-T
- Callaghan R, Luk F, Bebawy M. Inhibition of the multidrug resistance P-glycoprotein: time for a change of strategy? Drug Metab Dispos. 2014;42(4):623–631. doi:10.1124/dmd.113.056176
- Robinson K, Tiriveedhi V. Perplexing role of P-glycoprotein in tumor microenvironment. Front Oncol. 2020;10:265. doi:10.3389/fonc.2020.00265
- Johnson TW, Richardson PF, Bailey S, et al. Discovery of (10R)-7-Amino-12-fluoro-2,10,16-trimethyl-15-oxo-10,15,16,17-tetrahydro-2H-8,4-(metheno)pyrazolo[4,3-h][2,5,11]-benzoxadiazacyclotetradecine-3-carbonitrile (PF-06463922), a Macrocyclic Inhibitor of Anaplastic Lymphoma Kinase (ALK) and c-ros Oncogene 1 (ROS1) with Preclinical Brain Exposure and Broad-Spectrum Potency against ALK-Resistant Mutations. J Med Chem. 2014;57(11):4720–4744. doi:10.1021/jm500261q
- Daina A, Michielin O, Zoete V. SwissADME: a free web tool to evaluate pharmacokinetics, drug-likeness and medicinal chemistry friendliness of small molecules. Sci Rep. 2017;7(1). doi:10.1038/srep42717
- Bawadekji A, Imran M, Nayeem N. Posaconazole : a pharmaceutical review = : . . J North Basic Appl Sci. 2019;4(2):109–123.
- McLean AD, Chandler GS. Contracted Gaussian basis sets for molecular calculations. I. second row atoms, Z=11–18. J Chem Phys. 1980;72(10):5639–5648. doi:10.1063/1.438980
- Krishnan R, Binkley JS, Seeger R, et al. Self-consistent molecular orbital methods. XX. A basis set for correlated wave functions. J Chem Phys. 1980;72(1):650–654. doi:10.1063/1.438955
- Becke AD. Density-functional thermochemistry. III. The role of exact exchange. J Chem Phys. 1993;98(7):5648–5652. doi:10.1063/1.464913
- Lee C, Yang W, Parr RG. Development of the Colle-Salvetti correlation-energy formula into a functional of the electron density. Physical Review B. 1988;37(2):785–789. doi:10.1103/PhysRevB.37.785
- Miehlich B, Savin A, Stoll H, et al. Results obtained with the correlation energy density functionals of becke and Lee, Yang and Parr. Chem Phys Lett. 1989;157(3):200–206. doi:10.1016/0009-2614(89)87234-3
- Lee C-C, Kuo C-J, Ko T-P, et al. Structural basis of inhibition specificities of 3C and 3C-like proteases by zinc-coordinating and Peptidomimetic compounds. J Biol Chem. 2009;284(12):7646–7655. doi:10.1074/jbc.M807947200
- Bowers KJ, Chow DE, Xu H, et al. Scalable algorithms for molecular dynamics simulations on commodity clusters. ACM/IEEE SC 2006 Conference (SC'06)2006. p. 43-43.
- Jorgensen WL, Chandrasekhar J, Madura JD, et al. Comparison of simple potential functions for simulating liquid water. J Chem Phys. 1983;79(2):926–935. doi:10.1063/1.445869
- Martyna GJ, Klein ML, Tuckerman M. Nosé–Hoover chains: the canonical ensemble via continuous dynamics. J Chem Phys. 1992;97(4):2635–2643. doi:10.1063/1.463940
- Toukmaji AY, Board JA. Ewald summation techniques in perspective: a survey. Comput Phys Commun. 1996;95(2-3):73–92. doi:10.1016/0010-4655(96)00016-1
- Kagami LP, das Neves GM, Timmers LFSM, et al. Geo-measures: a PyMOL plugin for protein structure ensembles analysis. Comput Biol Chem. 2020;87:107322. doi:10.1016/j.compbiolchem.2020.107322
- Ghosh A, Mukerjee N, Sharma B, et al. Target specific inhibition of protein tyrosine kinase in conjunction with cancer and SARS-COV-2 by olive nutraceuticals. Front Pharmacol. 2022;12:812565. doi:10.3389/fphar.2021.812565
- Al-Qubaisi M, Rozita R, Yeap S-K, et al. Selective cytotoxicity of Goniothalamin against Hepatoblastoma HepG2 Cells. Molecules. 2011;16(4):2944–2959. doi:10.3390/molecules16042944
- Iwahara T, Fujimoto J, Wen D, et al. Molecular characterization of ALK, a receptor tyrosine kinase expressed specifically in the nervous system. Oncogene. 1997;14(4):439–449. doi:10.1038/sj.onc.1200849
- Morris SW, Naeve C, Mathew P, et al. ALK, the chromosome 2 gene locus altered by the t(2;5) in non-Hodgkin's lymphoma, encodes a novel neural receptor tyrosine kinase that is highly related to leukocyte tyrosine kinase (LTK). Oncogene. 1997;14(18):2175–2188. doi:10.1038/sj.onc.1201062
- Jawarkar RD, Sharma P, Jain N, et al. QSAR, molecular docking, MD simulation and MMGBSA calculations approaches to recognize concealed pharmacophoric features requisite for the optimization of ALK tyrosine kinase inhibitors as anticancer leads. Molecules. 2022: 27(15):4951. doi:10.3390/molecules27154951
- Lorén CE, Scully A, Grabbe C, et al. Identification and characterization of DAlk: a novelDrosophila melanogasterRTK which drives ERK activationin vivo. Genes Cells. 2001;6(6):531–544. doi:10.1046/j.1365-2443.2001.00440.x
- Kong X, Pan P, Sun H, et al. Drug Discovery Targeting Anaplastic Lymphoma Kinase (ALK). J Med Chem. 2019;62(24):10927–10954. doi:10.1021/acs.jmedchem.9b00446
- Xu Z, Yang Z, Liu Y, et al. Halogen bond: its role beyond drug–target binding affinity for drug discovery and development. J Chem Inf Model. 2014;54(1):69–78. doi:10.1021/ci400539q
- Bissantz C, Kuhn B, Stahl M. A medicinal chemist’s guide to molecular interactions. J Med Chem. 2010;53(14):5061–5084. doi:10.1021/jm100112j
- Hann MM. Molecular obesity, potency and other addictions in drug discovery. MedChemComm. 2011;2(5):349–355. doi:10.1039/C1MD00017A
- Thomas H, Coley HM. Overcoming multidrug resistance in cancer: an update on the clinical strategy of inhibiting P-glycoprotein. Cancer Control. 2017;10(2):159–165. doi:10.1177/107327480301000207
- Thorn M, Finnstrom N, Lundgren S, et al. Cytochromes P450 and MDR1 mRNA expression along the human gastrointestinal tract. Br J Clin Pharmacol. 2005;60(1):54–60. doi:10.1111/j.1365-2125.2005.02389.x
- Alfarouk KO, Stock C-M, Taylor S, et al. Resistance to cancer chemotherapy: failure in drug response from ADME to P-gp. Cancer Cell Int. 2015;15(1). doi:10.1186/s12935-015-0221-1