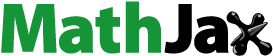
Abstract
We constructed a liquid-phase colorimetric biosensor to detect Triglycerides (TG) contamination in water using a Porcine Pancreatic Lipase Immobilized Zinc – chitosan nanocomposite matrix with Phenolsulfonphthalein as the chromophore. The nanoparticle-augmented matrix was characterized using an SEM—the change in the chromophore colour from red to yellow indicated vegetable oil hydrolysis and fatty acid release. The biosensor showed a LOQ between 25–500 µg/mL at an operating temperature between 25–45 °C, over a pH range of 4–9 within 5 minutes. The biosensor showed resilience to samples contaminated up to 50 times the BIS permissible limits for iron, copper and manganese. The sensor showed repeatability of nearly 100% with the LOD 3 µg/mL, sensitivity 47.1 U/µg/cm2 and retained up to 80% of its activity till the 10th day when stored at 10 °C. A fully adept version of the biosensor can be applied to detect TG in water and wastewater.
1. Introduction
Recent studies suggested that unchecked and unmitigated lipid accumulation beyond the permissible limits (10–100 mg/L for wastewater and 2 mg/L in packaged drinking water [Citation1,Citation2]), in turn, results in the bioaccumulation of nano-plastics and Persistent Organic Pollutants (POPs) in water. Nano-plastics (smaller than 1000 nm) in marine ecosystems undergo photocatalysis in sunlight and become lipophilic by extending out hydrophobic branches. This transformation makes them chemically similar to compounds such as POPs that last several years before breaking down [Citation3,Citation4]. Hence, early detection of TG in water is vital to address environmental problems.
Nanoconjugates (NCj) are hybrid particles formed when a biomolecule, such as an enzyme, is immobilized onto a nanocomposite [Citation5]. A nanocomposite (NCp) is a polymer loaded with nanofillers like metal nanoparticles (NP) or carbon-based nanomaterial and more [Citation6]. They combine macro, micro and nanometric particle characteristics such as direct immobilization, increased temperature and pH stability while retaining a small size and high surface-to-volume ratio [Citation7]. Introducing nanoparticles strengthens the matrix, enhancing biocatalytic efficiency and immobilization loading efficiency [Citation8,Citation9]. Chitosan was chosen to fabricate nanocomposites due to its selective hydrophilicity, high permeability, rigidity, nontoxicity, compatibility, inexpensiveness, abundance, and biodegradability [Citation10]. Immobilization can enhance activity, pH and thermal tolerance, stability, sensitivity and selectivity of enzymes [Citation11]. Since enzymes usually show specificity towards a particular substrate, these catalysts can detect analytes selectively that trigger a noticeable change and signal. This signal is translated by different types of transducers to colour, light, sound, or graph and detected by respective detectors [Citation12,Citation13].
The biosensing methods reported for the detection of TG can be categorized into optical, colorimetric, amperometric, potentiometric, spectrophotometric, fluorometric, chemical, and electrochemical [Citation14]. The conventional method is amperometry, with three electrodes: a reference, a control, and a working electrode. When coupled with a suitable recognition element, it shows fast, accurate, and precise results [Citation15]. One study reported the development of an amperometric system using three enzymes, Lipase, Glycerol Kinase, and Glycerol-3-Phosphate Oxidase, co-immobilized on a three-electrode system, and described earlier [Citation16].
Zhou and their team [Citation17] have reported an SPR biosensor composed of lipase immobilized onto magnetic beads. It uses fibre optics with a dual probe system for TG detection ranging from 0 to 8 mg/mL, with 1.25 nm/(mg/mL) sensitivity and a biosensing probe of 125 µm diameter. Breger and their colleagues in 2020 [Citation18] reported the development of a FRET biosensor that follows the kinetics under the Michaelis – Menten mechanism composed of custom–synthetic esters consisting of one terminal with a peptide chain and the other with a dye acceptor, attached by metal affinity coordination on to Quantum Dots composed of luminescent semiconductor. Lipase catalysis influences the ester–peptide bond, displacing the dye-accepting terminal and altering the rate of FRET. A paper-based device (PBD) was studied for detecting chlorpyrifos, a commonly used pesticide based on inhibiting enzymes and Image Processing through MATLAB [Citation19]. Lipase from the Rhizopus niveus was immobilized on Whatman paper No. 40 using p-nitrophenol for the substrate. The functionalized PBD responded significantly over a broad sample pH and temperature range. In this case, the Limit of Detection (LOD) was 0.065 mg/L, with a Limit of Quantitation (LOQ) of 0.198 mg/L.
Despite groundbreaking advances in lipase-immobilized biosensors for TG detection, there have been some recurring challenges. A common trait in TG biosensors is their dependency on circuitry or complex enzyme cocktails, especially visual biosensors. This makes them complex and fragile. Moreover, such biosensors have mainly been assessed using p-nitrophenol or tripalmitate, minimally emphasizing authentic TG or TG-derived samples. Moreover, in the case of amperometric or voltammetric biosensors, the method is fast, precise, and accurate; the sensor is complex to fabricate and needs operational expertise [Citation20]. Colorimetric biosensors are preferred to overcome such problems. They are specific, fast, easy to operate, cost-effective, and equally sensitive [Citation21]. This method is more intuitive since the obtained results can be observed with the naked eye without sophisticated instruments. Combining nano-scaled materials in the form of NCj and NCp and traditional colorimetry makes the technique more sensitive, precise, accurate, and cost-effective [Citation22]. With the involvement of enzymes, colorimetric biosensors become highly specific and fast in detection [Citation23].
The present study explores a novel approach for the colorimetric detection of TG in water using Lipase Immobilized Zinc-Chitosan NCj. The study focuses on validating the linear catalytic ability of the Lipase immobilized nanocomposite as a biosensing preparation in the liquid phase. With the hydrolysis of TG by lipase, fatty acids are generated, which cause a change in the colour shown by phenolsulfonphthalein from red to yellow. This prototype was designed so that the change in colouration occurs only when the NCj matrix interacts with any TG sample, making it a suited approach for fabricating colorimetric TG biosensors.
2. Materials
Porcine Pancreatic Lipase (PPL) was obtained from Sigma Aldrich, Germanyco. Olive Oil (99%), Chitosan (Ch) (Low Molecular Weight), Glacial Acetic Acid (99%), Zinc Acetate, NaOH, Polyvinyl alcohol (PVA), Phenolsulfonphthalein, Sodium Phosphate Monobasic, and Sodium Phosphate Dibasic were acquired from Sisco Research Laboratories India. Whatman Paper (No. 1) (WP-1) was purchased from GE Healthcare, India.
3. Methods (as summarized in Figure )
3.1. Synthesis of Zinc-oxide nanoparticles (ZnO NPs)
Zinc Acetate salt (5.5 g) was dissolved in distilled water (DW) (100 mL) under constant stirring and brought to 90 ℃ on a hot plate. In this solution, 16 ml NaOH (0.5 M) was added at first with constant stirring. After 5 min, 84 mL of NaOH (0.5 M) was gradually added and stirred for 2 h on heat to complete the displacement reaction. The precipitates were allowed to settle, and the liquid was decanted. The particles were centrifuged and washed with DW 5 times before drying at 100 ℃ for 30 min [Citation24].
3.2. Zinc – chitosan nanocomposite (Zn-Ch NCp) synthesis
Chitosan (2% w/v) solution was added to acetic acid (2% v/v, 25 mL) and stirred until complete dissolution. The solution was then diluted with ZnO NPs suspension (25 mL, 0.5 mg/mL) and stirred. The prepared Zn-Ch NCp was cast onto WP-1, with Petri dishes as mould and dried at 48℃ for 12 h [Citation25].
3.3. Functionalization of the NCp
Phenolsulfonphthalein (20 mL, 1% v/v in 50 mM, pH 8 phosphate buffer) was added to the Zn-Ch NCp to functionalize the NCp and dried at 48 ℃ for 8 h before enzyme immobilization [Citation26].
3.4. Enzyme immobilization
PPL (15 mL, 20 mg/mL) dissolved in Phosphate Buffer (50 mM, pH 8) was added to the NCp to develop NCj and incubated at 37°C for 6 h. Lipase was immobilized onto the NCp through physical adsorption [Citation27]. The resulting Zn-Ch–Lipase NCj was then cut into strips (2 cm × 0.5 cm) for biosensor studies. Post immobilization, the mould was washed with buffer and tested for enzyme activity to check for enzyme leakage.
3.5. Enzyme activity assay
To determine the lipase activity, a mixture of 1 mL emulsion (9:1, 2% PVA and Substrate), 0.8 mL phosphate buffer (50 mM, pH 8), and 0.2 mL enzyme solution (20 mg/mL) was incubated at 37°C for 30 min and titrated with NaOH (0.1 M) in the presence of phenolphthalein indicator [Citation28]. The activity was expressed regarding the amount of free fatty acids released per minute under assay conditions.
3.6. Characterization
The synthesized ZnO NPs were characterized for size and stability through Dynamic Light Scattering via Malvern Instruments Nano ZS, UK, as an average of 10 scans employing a 4 mW, 633 nm laser. Morphological and topological observations under enhanced magnification were made using a Jeol JSM 6390 LV, Japan, for Scanning Electron Microscopy (SEM). The NCj was chemically assessed to check for its efficacy in some primary colorimetric biosensor characteristics, like Limits of Quantitation (LOQ) and Detection (LOD) Sensitivity, Repeatability, Stability and, Shelf life.
3.7. Colorimetric bio-sensing
Entire colorimetric estimations were performed at 440 nm using UV–Vis. Spectrophotometer (LAB INDIA 3100-XE, India) based on full-spectral scanning was chosen as the λmax for the analyses. The experiments were performed in triplicates, and the reaction time was fixed at 6 min. The immobilized enzyme strips were dipped into the TG spiked water samples simulated in the lab and processed through different conditions for biosensor usage [Citation13,Citation14]. The transition of colour from red to yellow was assessed, and the intensity of the colour was quantitatively estimated to analyse the bio-sensing potential of the Zn-Ch–Lipase NCj biosensor for colorimetric detection of triglycerides. The TG contaminant levels across the experiments were maintained at 100 µg/mL (except for LOQs determination), the standard pH (except for pH assessment) was set at 8, and the standard temperature for incubation was set at 37 ℃.
3.7.1. Limit of quantitation (LOQ)
To determine the lowest and highest LOQ or the concentration of TG that can be detected by the developed biosensor [Citation21], the experiments were carried out with samples containing different concentrations of TG ranging from 10 to 1000 µg/mL.
3.7.2. Impact of sample temperature and pH
The intention of manufacturing this biosensor is to determine the TG in actual environmental samples. For that, the effect of the temperature and pH of the sample was checked [Citation29,Citation30]. For the experiment, the selected temperature ranged from 5 to 55℃ while the pH was tested from 4 to 9.
3.7.3. Impact of metal contamination
Some metal contaminants (Iron (Fe), Copper (Cu), and Manganese (Mn)) commonly found in the local water were used for the study. This was performed to assess the ability of the biosensor to perform in the presence of interfering metal solids [Citation31]. Metal contaminants were introduced to the sample with varying concentrations starting from 0 ppm to 30 ppm for Fe (BIS permissible level is 0.3 ppm), 0 ppm to 150 ppm for Cu (BIS permissible level is 1.5 ppm) and 0 ppm to 30 ppm for Mn (BIS permissible level is 0.3 ppm).
3.7.4. Storage stability and shelf life
Determining the biosensor's lifespan is crucial to identifying its stability and durability for usage. The shelf life of the sensor was evaluated for ten days by storing the biosensor strips in different temperatures and storage conditions (0, 10°C, room temperature, and 37°C).
3.7.5. Repeatability and sensitivity determination
The repeatability of the biosensor was assessed by randomly selecting 10 identical strips produced in a single batch to evaluate the consistency of their sensing potential. From this experiment, the Limit of Detection (LOD) and Sensitivity of the biosensor were calculated as described in the equations [Citation21,Citation32]:
Here, y0 = mean value of a control blank, and 3SD =Three times the standard deviation from y0
Where,
4. Results and discussion
4.1. Characterization of ZnO nanoparticles
The DLS data indicate that the size of the synthesized ZnO nanoparticles was 80.74 nm in length with a zeta potential of –20.4 mV. Literature suggests that the obtained size and charge make them suitable for biosensor applications [Citation14].
SEM images (Figure ) show distinct surface morphology and topological changes between a generic chitosan film, a functionalized Zn-Ch NCp matrix and a Zn-Ch–Lipase NCj biosensor. The surface of the chitosan film appears to be smooth and consistent. At the same time, we could observe crystalline formations in the Zn-Ch NCp, and a layer-like deposition could be observed on the Zn-Ch–Lipase NCj biosensor surface. Similar topological modifications have been reported in earlier studies [Citation33,Citation34]. The matrix was enriched with Zinc Oxide nanoparticles as they enhanced the catalytic potential and resilience to different conditions of the immobilized lipase. When in contact with lipase, Zinc nanoparticles exhibit a binding affinity towards the residues of the catalytic triad Ser-His-Asp, resulting in a partially unfolded or unfolded conformation of the enzyme and a consequent increase of the exposure of the active site. This does not directly affect the molecule's stability; it creates a bridge to balance the positively charged nitrogen from lysine and arginine with the negatively charged oxygen in glutamate and aspartate residues [Citation35].
4.2. Colorimetric biosensing
To evaluate the applicability of the Zn-Ch–Lipase NCj as a biosensor, we performed a detailed profiling of its working and chemical nature concerning the LODs, LOQs, sensitivity and stability, specificity, and the reproducibility of the biosensor through experiments and assays. It started with estimating the appropriate concentration of lipase needed (20 mg/mL) to be immobilized onto the NC Matrix to develop a biosensor that generates a significant response visible to the user as colour changes. The eluate from the washed moulds showed zero activity, confirming no significant enzyme leakage during immobilization. After full-spectrum scanning, the optimum wavelength was identified as 440 nm and chosen as the λmax for the analyses. All the experimental outcomes were consistent, and the Standard Error margins were negligible where the p-value was found to be smaller than 0.05.
4.2.1. Limit of quantitation (LOQ) of the biosensor
The biosensor was assayed with varying concentrations of TG, as outlined in Figure . The first instant of colorimetric response was recorded at a TG concentration of 25 µg/mL, and the last instant of colorimetric response was recorded at 500 µg/mL, making them the lowest and highest LOQs of TG in any given sample and the operating range to be 25–500 µg/mL. It was observed that for TG concentration below 25 µg/mL and beyond 500 µg/mL, the biosensor did not generate a measurable response, which may be due to the inhibitory effect of substrate concentration on the enzyme due to the shortage or excess in substrate concentration, as observed in earlier studies as well [Citation21].
4.2.2. Impact of sample temperature
The lipase immobilized as a biosensor showed enhanced temperature tolerance compared to the free enzyme and chromophore combination. The biosensor exhibited overall detection capabilities from 15–50 ℃ with an optimal working range between 25–45 ℃ (Figure ). The intensity is very high compared to the free enzyme, which shows minimal detection over the same temperature range. Earlier studies [Citation29,Citation36] have indicated that when immobilized onto Nano-MOFs and Nanocomposites, PPL tends to catalyse reactions in temperatures between 20 and 60°C with the best performance between 25 and 55°C.
4.2.3. Impact of pH
The biosensor was exposed to samples over a broad pH range (pH 4–9) based on the generally available pH spectrum of water samples across India [Citation30]. The outcomes were compared with free enzyme coupled with the chromophore, as observed in Figure . The signal intensity with biosensors was significantly high in all pH, and the maximum response was obtained near pH 8. Shifts in optimum pH after immobilization are standard for many enzymatic reactions. A similar observation for lipase biosensors for TG detection for samples in the pH range of 5–9 has been reported earlier [Citation12].
4.2.4. Impact of metal contamination
It has been reported by several studies that some metals, such as iron, copper, calcium, and manganese, among others, are commonly found in high quantities in regular potable water streams in Jharkhand [Citation37]. In our present study, the presence of calcium in the sample did not influence the biosensor's detection potential. The biosensor's ability to detect TG in samples contaminated with metals at varying concentrations based on multiples of Bureau of Indian Standards (BIS) permitted standards was assessed, as shown in Figure . It was observed that the biosensor retained up to 70% of its detection capabilities, up to 10 times the permissible limits approved by BIS. As we approached metal concentrations up to 50 times the permissible limit, the detectability began to fall. We observed that the biosensor lost its detection potential at 100 times the BIS limit. The concentration of metal contaminants has been expressed in percentage multiples of the permitted limits. Our biosensor showed resilience to up to 300 ng/L of metal contaminant interferent, while Swain and their team [Citation31] reported that their biosensor handled 50 ng/L of metal contaminant.
4.2.5. Storage stability and shelf life
The shelf life of the sensor was evaluated for 10 days by storing the biosensor strips in different storage temperatures such as 0, 10, 37°C, and room temperature (RT). The biosensors stored at 10°C showed maximum stability. At this temperature, the biosensor retained up to 90% of its original activity for eight days. After that, the retention was nearly 80% after ten days. At 0°C for the freezer temperature, the stability was retained at 70% for up to 10 days. Reduced activity compared to 10°C might be due to partial denaturation of the enzyme due to ice formation. The stability of the sensor stored at RT was 90% for the first two days and fell to almost 40% over the next eight days. Temperature fluctuation due to environmental conditions may lead to a sharp decline in enzyme activity. For the strips stored at 37 ℃, the stability was decreased to 30% by the 10th day. Hence, the maximum response was shown by the strips stored at a refrigeration temperature of 10°C, as shown in Figure . Retention of 70–80% activity over the first 10–15 days when stored between 4 and 10°C was reported earlier [Citation14,Citation21]. Recent studies have elaborated upon various strategies, such as introducing stabilizers or employing Metal–organic frameworks (MOFs) that can improve the product's shelf life when translated from the prototype [Citation38].
4.2.6. Repeatability and sensitivity
The repeatability of a biosensor is an important parameter as it establishes its consistency and precision. Ten identical strips were chosen at random from a batch and assayed. All ten strips showed nearly 100% consistency toward TG detection, as highlighted in Figure . The LOD or Limit of Detection and Sensitivity of the biosensor were calculated to be three µg and 47.1 U/µg/cm2, respectively. Comparable observations have also been made in studies reported earlier [Citation39]. Sankar and team [Citation19] have reported the importance of repeatability in a disposable biosensor. They observed their biosensor to have LOD ranging from 0.2 to 10 µg/mL.
Conclusion
NCs have been acting as a bridge between micro and nanometric studies, with the advantages of both scales and almost no compromises on their prime characteristics. Chitosan, a natural polymer, is preferred due to its biodegradability and broad range compatibility with organic and inorganic NPs, generating a grand spectrum of conjugates and composites. It can support a wide range of biological interactions as a matrix, and bio-molecular immobilization (antibody–antigen and enzyme immobilization) has shown great promise in research and commercialization. Our study shows the potential of Lipase–Zinc–Chitosan Nanoconjugate as a colorimetric biosensor for detecting Triglyceride in water. The prototype showed promising resilience towards commonly found metal contaminants and variable environmental conditions, which are properties akin to that of a potentially marketable biosensor. Our prototype biosensor exhibited a low response time of 6 min, high activity in enzyme catalysis for the free enzyme, high specificity towards the sample, and a broad working range in terms of detectability of 25–500 µg/mL over a local temperature range of 25–45 ℃, across 4–9 pH. It showed promising resilience to metal interferents such as iron, copper and manganese in the sample using PPL alone. In its prototype state, the biosensor exhibited an exemplary standard as a novel approach for colorimetric biosensing of TG based on its specificity, reproducibility, and stability. With deeper probing, better options for solid supports and inputs may be picked that improve the responding time and the shelf life while enhancing the other corresponding properties of the biosensor. Further studies on the solid phase of the biosensor may present room for improvement and result in a marketable TG biosensor.
Acknowledgement
The authors are grateful to the Department of Bioengineering and Biotechnology at the Birla Institute of Technology (BIT), Ranchi, for an engaging environment. The authors acknowledge the support from the Central Instrumentation Facility, BIT and BIT, Ranchi, for their support. MBI acknowledges BIT, Ranchi, for their Institutional Research Fellowship.
Data availability
Not Applicable
Disclosure statement
No potential conflict of interest was reported by the author(s).
References
- Jamwal R, Kumari S, Sharma S, et al. Recent trends in the use of FTIR spectroscopy integrated with chemometrics for the detection of edible oil adulteration. Vib Spectrosc. 2021;113:103222. doi:10.1016/j.vibspec.2021.103222
- Kaur J, Kaur V, Pakade YB, et al. A study on water quality monitoring of Buddha Nullah, Ludhiana, Punjab (India). Environ Geochem Health. 2021;43:2699–2722. doi:10.1007/s10653-020-00719-8
- Gaylarde CC, Neto JAB, da Fonseca EM. Nanoplastics in aquatic systems-are they more hazardous than microplastics? Environ Pollut. 2021;272:115950. doi:10.1016/j.envpol.2020.115950
- Baumert BO, Goodrich JA, Hu X, et al. Plasma concentrations of lipophilic persistent organic pollutants and glucose homeostasis in youth populations. Environ Res. 2022;212:113296. doi:10.1016/j.envres.2022.113296
- Sun Z, Cai M, Hübner R, et al. Tailoring particle-enzyme nanoconjugates for biocatalysis at the organic-organic interface. ChemSusChem. 2020;13(24):6523–6527. doi:10.1002/cssc.202002121
- Roy S, Kim HC, Zhai L, et al. Preparation and characterisation of synthetic melanin-like nanoparticles reinforced chitosan nanocomposite films. Carbohydr Polym. 2020;231:115729. doi:10.1016/j.carbpol.2019.115729
- Thakkar JB, Aghera DJ, Trivedi B, et al. Design and characterisation of a biosensor with lipase immobilized nanoparticles in polymer film for the detection of triglycerides. Int J Biol Macromol. 2023;229:136–145. doi:10.1016/j.ijbiomac.2022.12.281
- Wahab RA, Elias N, Abdullah F, et al. On the taught new tricks of enzymes immobilization: an all-inclusive overview. React Funct Polym. 2020;152:104613. doi:10.1016/j.reactfunctpolym.2020.104613
- Wu Y, Tilley RD, Gooding JJ. Challenges and solutions in developing ultrasensitive biosensors. J Am Chem Soc. 2018;141(3):1162–1170. doi:10.1021/jacs.8b09397
- Abd El-Hack ME, El-Saadony MT, Shafi ME, et al. Antimicrobial and antioxidant properties of chitosan and its derivatives and their applications: a review. Int J Biol Macromol. 2020;164:2726–2744. doi:10.1016/j.ijbiomac.2020.08.153
- Noori R, Ahmad R, Sardar M. Nanobiosensor in health sector: the milestones achieved and future prospects. Nanobiosensors Agric Med Environ Appl. 2020;63–90. doi:10.1007/978-981-15-8346-9_4
- Zhang H, Wu S, Zhang L, et al. High-resolution colorimetric detection of lipase activity based on enzyme-controlled reshaping of gold nanorods. Anal Methods. 2019;11(17):2286. doi:10.1039/C9AY00410F
- Wang Z, Ma B, Shen C, et al. Direct, selective and ultrasensitive electrochemical biosensing of methyl parathion in vegetables using Burkholderia cepacia lipase@ MOF nanofibers-based biosensor. Talanta. 2019;197:356–362. doi:10.1016/j.talanta.2019.01.052
- Purohit B, Vernekar PR, Shetti NP, et al. Biosensor nanoengineering: Design, operation, and implementation for biomolecular analysis. Sens Int. 2020;1:100040. doi:10.1016/j.sintl.2020.100040
- Bollella P. Enzyme-based amperometric biosensors: 60 years later … Quo Vadis? Anal Chim Acta. 2022;340517. doi:10.1016/j.aca.2022.340517
- Narwal V, Pundir CS. Development of glycerol biosensor based on co-immobilization of enzyme nanoparticles onto graphene oxide nanoparticles decorated pencil graphite electrode. Int J Biol Macromol. 2019;127:57–65. doi:10.1016/j.ijbiomac.2018.12.253
- Zhou S, Li X, Zhang J, et al. Dual-fiber optic bioprobe system for triglyceride detection using surface plasmon resonance sensing and lipase-immobilized magnetic bead hydrolysis. Biosens Bioelectron. 2022;196:113723. doi:10.1016/j.bios.2021.113723
- Breger JC, Susumu K, Lasarte-Aragonés G, et al. Quantum dot lipase biosensor utilising a custom-synthesised peptidyl-ester substrate. ACS Sens. 2020;5(5):1295–1304. doi:10.1021/acssensors.9b02291
- Sankar K, Lenisha D, Janaki G, et al. Digital image-based quantification of chlorpyrifos in water samples using a lipase embedded paper based device. Talanta. 2020;208:120408. doi:10.1016/j.talanta.2019.120408
- Hooda V, Gahlaut A, Gothwal A, et al. Recent trends and perspectives in enzyme based biosensor development for the screening of triglycerides: a comprehensive review. Artif Cells Nanomed Biotechnol. 2018;46(sup2):626–635. doi:10.1080/21691401.2018.1465946
- Hasanah U, Sani NDM, Heng LY, et al. Construction of a hydrogel pectin-based triglyceride optical biosensor with immobilized lipase enzymes. Biosensors. 2019;9(4):135. doi:10.3390/bios9040135
- Liu B, Zhuang J, Wei G. Recent advances in the design of colorimetric sensors for environmental monitoring. Environ Sci Nano. 2020;7(8):2195–2213. doi:10.1039/D0EN00449A
- Chen F, Chen D, Deng T, et al. Combination of alkaline phosphatase/graphene oxide nanoconjugates and D-glucose-6-phosphate–functionalised gold nanoparticles for the rapid colorimetric assay of pathogenic bacteria. Biosens Bioelectron. 2022;216:114611. doi:10.1016/j.bios.2022.114611
- Shah E, Mahapatra P, Bedekar AV, et al. Immobilization of Thermomyces lanuginosus lipase on ZnO nanoparticles: mimicking the interfacial environment. RSC Adv. 2015;5(33):26291–26300. doi:10.1039/C5RA02249E
- Dananjaya SHS, Kumar RS, Yang M, et al. Synthesis, characterisation of ZnO-chitosan nanocomposites and evaluation of its antifungal activity against pathogenic Candida albicans. Int J Biol Macromol. 2018;108:1281–1288. doi:10.1016/j.ijbiomac.2017.11.046
- Iyer MB, Chattopadhyay S. Colourimetric detection of triglycerides using porcine pancreatic lipase immobilized nanocomposite biosensor. Biomass Convers Biorefinery. 2023;1; doi:10.1007/s13399-023-04458-5
- Rafiee F, Rezaee M. Different strategies for the lipase immobilization on the chitosan based supports and their applications. Int J Biol Macromol. 2021;179:170–195. doi:10.1016/j.ijbiomac.2021.02.198
- Chattopadhyay S, Sen R. A comparative performance evaluation of jute and eggshell matrices to immobilise pancreatic lipase. Process Biochem. 2012;47(5):749–757. doi:10.1016/j.procbio.2012.02.003
- Yue JY, Ding XL, Wang L, et al. Novel enzyme-functionalised covalent organic frameworks for the colorimetric sensing of glucose in body fluids and drinks. Mater Chem Front. 2021;5(10):3859. doi:10.1039/D1QM00314C
- Dabhade A, Jayaraman S, Paramasivan B. Colorimetric paper bioassay by horseradish peroxidase for the detection of catechol and resorcinol in aqueous samples. Prep Biochem Biotechnol. 2020;50(8):849–856. doi:10.1080/10826068.2020.1760883
- Swain KK, Balasubramaniam R, Bhand S. A portable microfluidic device-based Fe3O4–urease nanoprobe-enhanced colorimetric sensor for the detection of heavy metals in fish tissue. Prep Biochem Biotechnol. 2020;50(10):1000–1013. doi:10.1080/10826068.2020.1780611
- Arya SS, Dias SB, Jelinek HF, et al. The convergence of traditional and digital biomarkers through AI-assisted biosensing: a new era in translational diagnostics? Biosens Bioelectron. 2023:115387. doi:10.1016/j.bios.2023.115387
- Alshammari FH. Physical characterisation and dielectric properties of chitosan incorporated by zinc oxide and graphene oxide nanoparticles prepared via laser ablation route. J Mater Res Technol. 2022;20:740–747. doi:10.1016/j.jmrt.2022.07.046
- Al-Ghamdi A, Indumathi T, Kumar ER. Green synthesised zinc oxide nanoparticles: effect of polyethylene glycol and chitosan on structural, optical and morphological analysis. Ceram Int. 2022;48(13):18324–18329. doi:10.1016/j.ceramint.2022.03.091
- Fahim YA, El-Khawaga AM, Sallam RM, et al. A review on lipases: sources, assays, immobilization techniques on nanomaterials and applications. BioNanoScience. 2024;1; doi:10.1007/s12668-024-01319-x
- Misra N, Rawat S, Goel NK, et al. Radiation facilitated-immobilized lipase (RaFIL) mini biocatalytic reactor based on epoxy decorated PP tubes: rapid colorimetric estimation of pesticide Chlorpyrifos. J Macromol Sci, Part A. 2022;59(9):575–586. doi:10.1080/10601325.2022.2098143
- Tirkey P, Bhattacharya T, Chakraborty S. Arsenic and other metals in the groundwater samples of Ranchi city. Jharkhand: Current Science; 2016, p.76.
- Yezer I, Demirkol DO. Cellulose acetate–chitosan based electrospun nanofibers for bio-functionalised surface design in biosensing. Cellulose. 2020;27(17):10183–10197. doi:10.1007/s10570-020-03486-y
- Pohanka M, Zakova J, Sedlacek I. Digital camera-based lipase biosensor for the determination of paraoxon. Sens Actuators, B. 2018;273:610–615. doi:10.1016/j.snb.2018.06.084