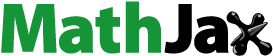
Abstract
This study employed particle-size analysis on twenty friable sandstone beds from two outcrops of the Biyadh Formation, which acts as a water aquifer, petroleum reservoir, and CO2 capturing reservoir. Hydrodynamic processes and depositional environments were identified using bivariate plots, Visher diagrams, Passega diagrams, and linear discriminant functions (LDF). The results reveal that the beds of Outcrop A mainly consist of medium- to well-sorted, medium-grained sandstone that is near-symmetrical and mesokurtic. However, Outcrop B comprises medium-sorted, medium-grained sandstone beds that are near-symmetrical and leptokurtic. All the beds in Outcrop A and B are unimodal. Furthermore, Visher and Passega diagrams indicate that the particles of Outcrop A were mostly transported by rolling, saltation, and suspension. In contrast, the particles of Outcrop B were transported by rolling, saltation, suspension, and graded suspension. The LDF technique shows that the depositional environments were beach, aeolian, fluvial, and shallow marine.
1 Introduction
One of the most significant methods to analyze hydrodynamic processes and depositional environments is particle-size analysis (grain-size analysis). It is also utilized for sediment classifications [Citation1–3]. It provides considerable evidence of sedimentary origins, modes of sediment transit, and depositional environments [Citation4]. Due to the unique characteristics of depositional environments, particle-size analysis may distinguish such environments by indicating the sedimentary conditions [Citation1,Citation4–11].
There are three approaches for quantifying particle sizes: the grade scale method, quantitative analysis using statistical and graphical methods, and the data genetic method. The most significant method for evaluating depositional environments is the graphical and statistical technique [Citation12]. It includes plotting weight percentage versus particle sizes in phi (∅) units in addition to calculating statistical particle-size parameters, including inclusive particle size mean, standard deviation, kurtosis, and skewness. Sedimentary environments can be distinguished by utilizing bivariate plots of particle-size parameters [Citation13] in addition to log-probability diagrams of particle sizes [Citation8]. Log-probability diagrams can also indicate the transportation mechanisms of the sediments, such as traction, saltation, and suspension [Citation8].
The Biyadh Formation is a Lower Cretaceous sandstone that was first reported by Koch and Brown in 1934 as large unnamed clastic units [Citation14], which were then designated as “Biyadh Sandstone” in the Sila’at Biyadh area, Ar Riyadh quadrangle, by Steineke et al. [Citation15]. According to Powers et al. [Citation14], that the subsurface Biyadh Sandstone is Barremian, while the outcrops ranges from Barremian to Albian. The Biyadh Formation outcropped for approximately 650 km, from Wadi ad Dawasir to Wadi al Atk, with two breaks: (I) the syncline of Wadi as Sabha, and (II) the Al Aflaj gravel sheet [Citation14]. It is worth noting that the Biyadh Formation is the only one in Saudi Arabia that can be traced over a huge distance [Citation16].
In Saudi Arabia, the Biyadh and Wasia are the only clastic formations deposited from the Lower Jurassic to the Eocene [Citation17]. It is conformably and gradationally underlain by thin beds of marine limestone (the Buwaib Formation) and is unconformably overlain by coarse-grained sandstone (the Wasia Formation) and in some locations by marine carbonate (the Shu’aiba Formation) [Citation14,Citation18–20]. Its thickness ranges from 300 to 700 m at several locations [Citation14,Citation18,Citation20]. The majority of the lower seventy percent of the formation is mostly non-marine cross-bedded friable sandstone with some shale beds [Citation14,Citation21–23]. According to the lithology difference, the Biyadh Sandstone was subdivided into three members by Vaslet et al. [Citation24] (from bottom to top): (I) Dughum Member, approximately 264 m of mostly sandstone; (II) Sallah Member, about 30 m of claystone; and (III) Huraysan Member, around 90 m of sandstone-siltstone. However, these members are undifferentiated at the outcrops [Citation21] and only the Dughum Member corresponds to the Biyadh Sandstone subsurface [Citation20].
The Biyadh Formation is one of the most important aquifers in Saudi Arabia [Citation22,Citation25]. Its water quality varies from fresh meteoric water, 300-900 ppm (of dissolved solids) toward the west, to connate water with up to 35,000 ppm (of dissolved solids) toward the east. At Ain Dar, the non-potable water is injected into oil reservoirs [Citation14]. In addition, the Biyadh Formation (Biyadh Reservoir) also contains petroleum in Saudi Arabia [Citation26,Citation27] in Safaniya (Biyadh-Zubair), and in the south-east of Iraq (Zubair) [Citation14,Citation19,Citation20] as well as in Yemen in Kharir Field (Masila Basin) [Citation28]. The porosity and permeability (non-anisotropy) of the Biyadh Formation range from 1-36% to 2100–6500 mD, respectively [Citation22,Citation28]. Furthermore, Khan et al. [Citation29] proved the significance of the Biyadh Formation in capturing 8–20 Gt (billion tons) of carbon dioxide (CO2). This is due to sufficient reservoir depth in the Biyadh Formation, which stores the CO2 in supercritical form, in addition to the non-fractured sedimentary cap rocks of the Shu’aiba, Wasia, and Arum Formations, which prevent CO2 leakage to the surface or portable water aquifers. The novelty of this research is investigating and evaluating the depositional processes and environments of the Biyadh Formation through the generation, integration, and plotting of particle-size analyses. It is a comprehensive study that focuses on the hydrodynamic processes and depositional environments of the Biyadh Formation of Saudi Arabia.
2 Materials and methods
This research examines the two Biyadh Formation outcrops (locations A and B) located north-east of Riyadh city. Outcrop A is located at latitude 24°55′11.4″ N and longitude 46°57′4.8″ E, while Outcrop B is 3.7 km away at latitude 24°54′6.5″ N and longitude 46°58′34.7″ E (Figure ). Each outcrop was divided into ten beds based on their structures and grain sizes, all of which are poorly cemented friable sandstone. The bed sequence is ordered based on the outcrop stratigraphic deposition, where bed A1 is the base bed of Outcrop A.
Figure 1. A geological map and stratigraphic columns of the study areas. (A) a satellite map of Saudi Arabia; (B) a geological map of the AR Riyad Quadrangle (modified from https://ngd.sgs.gov.sa, accessed on May 16, 2023); (C) a stratigraphic column of Outcrop A; and (D) a stratigraphic column of Outcrop B.

From each bed in each outcrop, a fresh representative sample was collected (bottom to top). To prepare a representative dry fraction of 350 g from each bed, a riffle sample splitter was employed. This fraction was sieved by a stacked sieve set composed of 38, 63, 125, 250, 500, 1000, 2000, and 4000-micron mesh in addition to a receiving pan. Each sample was shaken for fifteen minutes after being poured into the very top sieve. The particles’ weight in each sieve and the receiving pan was recorded. Then, the particle diameters were converted from millimeters () to ∅ units using equation Equation1
(1)
(1) , which was introduced by Krumbein and Monk [Citation30].
(1)
(1) The cumulative percentage was plotted against the particle size in ∅ units to detect the 1st, 5th, 16th, 25th, 50th, 75th, 84th, and 95th percentiles. The particle-size parameters were then calculated, including inclusive graphic mean (
), inclusive graphic standard deviation (
), inclusive graphic skewness (
), and graphic kurtosis (
) using equations 2–5 proved by Folk and Ward [Citation5] as the follows:
(2)
(2)
(3)
(3)
(4)
(4)
(5)
(5)
Bivariate plots were generated to differentiate depositional environments based on their particle texture variations. To distinguish between depositional environments, the linear discriminant functions (LDF) technique was implemented, introduced by Sahu [Citation31]. LDF determines the fluidity and fluctuations of the medium energy during deposition [Citation31,Citation32]. Four linear discriminant functions may be utilized for determining depositional environments (equations 6-9) as follows: is differentiating beach from aeolian;
is distinguishing beach from shallow agitated (marine) water;
is differentiating fluvial from shallow marine; and
is distinguishing the process of turbidity from fluvial current.
(6)
(6)
(7)
(7)
(8)
(8)
(9)
(9) To determine the depositional process, sedimentation method, and transportation mechanism, particle-size analysis was used in addition to the Passega diagram (C–M plot) [Citation6] and Visher plot (log-probability plot) [Citation8].
3. Results and discussion
3.1. Particle-size statistics
The bed thickness of the Biyadh Formation studied outcrops (A and B) ranges from 0.8–6 m and 0.45–6 m, respectively. The particle-size analysis results are shown in Table S1. Most beds are composed of sand-size particles with an average of 99.6% and 97.7%, respectively. The average gravel percentage of Outcrop A is lower than that of Outcrop B. Thus, its coarser one-percentile (C) is also low (1,050 microns) compared to outcrop B (1,635 microns). Additionally, the median particle size of Outcrop B (302 microns) is lower than Outcrop A due to the increase in mud-size particles compared to Outcrop A (349 microns).
The volume-percentage frequency curves (Figure (a)) demonstrate that every bed in Outcrop A is unimodal, with 90% of the beds are peaking at 2∅ (250 microns) and only Bed 7 is peaking at 3∅ (125 microns), indicating that Bed 7 contains finer particles than the other beds. This is also indicated by having the lowest coarser one-percentile and median particle size of 495 and 227 microns, respectively.
Figure (b) indicates that all the beds in Outcrop B are unimodal, 30% of which have broad unimodal peaks. Four (B5, B7, B8, and B9) beds peak at 3∅, while three beds (B1, B3, and B6) peak at 2∅. This agrees with the results of the coarser one-percentile and median particle sizes. The 2∅ peak beds (coarse particles) have a coarser one-percentile and median particle size of 1,236 and 381 microns, respectively, while the 3∅ peak beds (finer particles) have a coarser one-percentile and median particle sizes of 791 and 314 microns, respectively. Moreover, Outcrop B's three broad unimodal beds reach peaks at 2.5∅ (two beds) and 1.5∅ (one bed).
3.2. Particle-size parameters
The size of the particles and their transportation methods are controlled by the conditions of the depositional environments [Citation12,Citation33–37]. Using the cumulative particle-size distribution curves, the four particle-size parameters were calculated (Table S2). One of the significant parameters is the particle-size mean, which is used in different study areas, including groundwater management [Citation38] and hydraulic conductivity of sandstone reservoirs [Citation39]. A particle-size mean of less than 2∅ (coarse to medium particles) is an indication of high to medium energy in depositional environments [Citation2]. A slight mean variation of particle sizes indicates low energy fluctuations in depositional environments or a single particle source. Generally, particle size is inversely related to sorting [Citation5]. Because high-energy mediums transport coarse particles with finer grains, coarse particles sorting poorly, whereas low-energy mediums transport only fine particles, resulting in better sorting. The difference in the kinetic-energy regime may be interpreted by the standard deviation as showing the velocity of the transportation medium.
The results of the mean size show variations between the two outcrops (Figure (a)). The results from Outcrop A range from 1.13 to 2.12∅ with an average of 1.62∅. This is an indication that these beds are composed mostly (90%) of medium-particle sandstone and a single fine-particle sandstone bed. Such a narrow variation indicates low energy fluctuations in the depositional environment or a single source of particles. The results of the particle mean size from Outcrop B, however, vary from 0.70 to 2.87∅ with an average of 1.90∅ showing that Outcrop B is composed of medium-particle sandstone (50%), fine-particle sandstone (40%), and a single coarse-grained sandstone (10%). The resultant variation of the mean particle size of Outcrop B shows multiple particle sources or high energy fluctuations in the depositional environments.
The variation in the particle-size standard deviation (sorting) between the outcrops is another indicator of the difference (Figure (b)). The majority (seven) of the sandstone beds in Outcrop A are composed of medium-well-sorted particles, in addition to single sandstone beds having very-well-sorted, well-sorted, and moderately-sorted particles. This represents a slight change in the kinetic energy regime. However, Outcrop B has a wider sorting variation, which ranges from 0.42–1.12∅ with an average of 0.77∅. Its sandstone beds are composed of 30% poorly-sorted, 20% moderately-sorted, 10% well-sorted, and 40% moderately-well-sorted. Unlike Outcrop A, the wide sorting variation indicates velocity fluctuations in the transportation medium of Outcrop B.
The ranges of particle-size skewness differ between Outcrops A and B (Figure (c)). The skewness of the Outcrop A sandstone beds varies from −0.16 to 0.23∅ with an average of 0.02∅. These beds are 30% coarse-skewed, 40% near-symmetrical, and 30% fine-skewed. On the other hand, Outcrop B has a wider variation, from −0.32 to 0.25∅ with an average of −0.07∅. It is strongly-coarse-skewed, coarse-skewed, near-symmetrical, and fine-skewed with 10%, 30%, 40%, and 20%, respectively. Both leptokurtic and mesokurtic kurtosis values indicate fine-particle supply after a drop in the transportation energy of the particles [Citation1]. The kurtosis of Outcrop A is mesokurtic (60%) and platykurtic (40%). Outcrop B, in contrast, has a wider range (Figure (d)), mesokurtic (60%), leptokurtic (30%), and very-leptokurtic (10%). It is worth nothing that the results of all particle-size parameters show differences between the two outcrops.
3.3. Bivariate plots of statistical parameters
The standard deviation versus mean particle-size bivariate plot (Fig. S1A) shows that the sandstone beds of Outcrop A are mostly composed of moderately-well-sorted, medium-size particles. Most (90%) of these beds are medium-grained, with only one bed that is fine-medium-grained with an average mean particle size of 1.62∅. Their sorting ranges from moderately-sorted to very-well-sorted, with an average of 0.56∅. This is an indication of stable energy levels for most of the Outcrop A beds during the depositional process. On the other hand, the mean particle size of the beds of Outcrop B ranges from coarse-grained to fine-grained and from poorly to moderately-well-sorted. The variance in mean and standard deviation is produced by fluctuations in energy level during the depositional process.
The inclusive graphic skewness describes the distribution symmetry of the particle sizes, where positive skewness indicates leaning toward coarse particles and negative skewness shows leaning toward fine particles. The bivariate plot of the skewness against the mean particle size (Fig. S1B) shows an inverse relationship: when negative skewness (leaning towards fine-particles) decreases, the mean particle size increases (decreases in microns). The skewness of the sandstone beds of Outcrops A and B ranges from −0.16-0.23∅ and −0.32-0.25∅, respectively. This variation is another indication of energy-level fluctuation during the depositional process of Outcrop B. The kurtosis of the particle distribution of Outcrop A has a narrow range from mesokurtic (0.83∅) to platykurtic (1.04∅), an indication of energy stabilization of the transportation medium. In contrast, Outcrop B has a wider variation from mesokurtic (0.91∅) to very-leptokurtic (1.70∅ (very peaked)) (Fig. S1C). It is worth noting that this wide variation is another indication of changing the energy level during the deposition process.
The standard deviation against skewness bivariate plot (Fig. S1D) shows an inverse relationship between particle-size sorting and their skewness. During high energy transport medium, mostly coarser-size particles were deposited with a higher level of standard deviation (poor sorting) and negative skewness. However, most of the moderately- to well-sorted beds are fine-skewed. The kurtosis bivariate plot against the skewness (Fig. S1E) shows the sandstone beds of Outcrop A are (60%) mesokurtic with fine-skewed to near-symmetrical skewness and (40%) platykurtic that are mainly coarse-skewed. Outcrop B, on the other hand, is composed of sandstone beds that are (60%) mesokurtic fine-skewed to near-symmetrical; (30%) leptokurtic coarse-skewed; and (10%) very-leptokurtic strongly coarse-skewed.
3.4. Linear discriminant functions (LDF)
To distinguish between depositional environments, the LDF technique may be implemented. The LDF may be utilized to distinguish between beach and aeolian environments;
differentiates between marine and beach environments;
discriminates between shallow marine and fluvial;
may be utilized to differentiate between turbidity currents and fluvial processes. The results of the LDF analysis are shown in Table S3. The results of
for the outcrops imply beach environments for the vast majority (90 and 70%, respectively) of the sandstone beds (Figure (a)). The
of Outcrop A suggests that it is 60% marine and 40% beach. However, the results of Outcrop B have a wider range, indicating a predominant (90%) marine environment (Figure (b)). The depositional environments of Outcrops A and B are shallow marine (100 and 80%, respectively), with turbidity currents, according to the results of
and
(Figure (c)).
3.5. Facies analysis
The depositional environments are identified using statistical particle-size analysis. Other approaches are anticipated to be utilized to support these findings, including sedimentary structures, textures, facies, fossils, and bed thickness. The environments of the studied outcrops were determined using particle-size analysis and sedimentary facies.
The lithofacies of the ten sandstone beds in Outcrop A were classified as follows (from bottom to top): medium-grained (two beds), coarse- to medium-grained (two beds), medium-grained (two beds), fine-grained, and medium- to fine-grained (three beds) (Table S4). On the other hand, the lithofacies of the ten sandstone beds in Outcrop B were recognized as follows (from bottom upward): coarse- to medium-grained, coarse-grained, medium- to fine-grained (two beds), fine-grained, medium- to fine-grained, fine-grained, medium, fine- to very fine-grained, fine-grained, and medium- to fine-grained (Table S4).
According to the particle-size analysis and the common environmental characteristics, three different environments were distinguished in Outcrop A, including beach, aeolian, and shallow marine environments (Table S2). In addition to these three environments, an additional environment (fluvial) was recognized only in Outcrop B (Table S5). Aeolian environment features include medium- to well-sorted particles and large-scale cross-bedding. Fluvial environments are characterized by poorly-sorted and coarse-particles, whereas marine environments are characterized by carbonate and fine particles. The depositional environments of Outcrop A were identified as follows (from bottom upwards): (A1-6) beach; (A7) aeolian; (A8) beach; (A9) aeolian; (A10) fluvial. On the other hand, the depositional environments of Outcrop B were identified as follows (from bottom upwards): (B1) aeolian; (B2) fluvial; (B3-4) beach; (B5) aeolian; (B6) fluvial; (B7-8) aeolian; (B9) shallow marine; (B10) fluvial. It is worth noting that the fluvial, beach, and dune environments were recognized in the Biyadh Formation by Powers et al. [Citation14], Moshrif [Citation18], Soliman and Al Shamlan [Citation19], Le Nindre et al. [Citation20] and Keller et al. [Citation22]. Additionally, Le Nindre et al. [Citation20] claimed that the Biyadh Formation is composed of coastal-plain clastics, which were deposited during transgressive and regressive sequences.
3.6. Passega diagram (C–M plot)
The Passega diagram, also known as the C–M (coarser one-percentile versus median) plot, was proposed by Passega [Citation6,Citation7] and Passega and Byramjee [Citation40] to estimate the predominant hydrodynamic transportation prior to the deposition of the particles. Such a geological approach is essential for determining the depositional conditions of ancient and modern particles. The Passega diagram is a log–log bivariate plot of the coarser one-percentile versus the particles’ median on the plot (Figure ). The determined transportation mode is classified as follows: N-O is rolling, O-P is bottom suspension and rolling, P-Q is rolling and suspension, Q-R is saltation, and R-S is uniform suspension. Most of the beds’ particles in Outcrop A and half of the beds’ particles in Outcrop B fall in the N-O segment of type I, indicating rolling transportation with no to little suspension due to their high level of coarser one-percentile and median. However, the other half of the beds’ particles in Outcrop B were transported by a higher level of suspension, with little to no rolling falling in segment P-Q sector II (in five beds). This is due to their smaller particle sizes, represented by the coarser one-percentile and median sizes.
Figure 5. Passega diagram (C-M plot) of the outcrops’ beds (modified from [Citation6,Citation7,Citation40]) where, Cr is the maximum grain size of rolling transported, Cs is maximum grain size of graded suspension, and Cu is the maximum grain size of uniform suspension.
![Figure 5. Passega diagram (C-M plot) of the outcrops’ beds (modified from [Citation6,Citation7,Citation40]) where, Cr is the maximum grain size of rolling transported, Cs is maximum grain size of graded suspension, and Cu is the maximum grain size of uniform suspension.](/cms/asset/58e7071c-9421-4520-99ff-20ec1460eb47/tusc_a_2354570_f0005_oc.jpg)
3.7. Visher diagram (Log-probability plot)
The Visher diagram is a significant technique to determine the methods of particle transportation and depositional processes [Citation8]. Figure shows that the beds’ particles in both outcrops were transported by a single level of saltation and suspension. However, the suspension method in Outcrop A starts at 2∅ (250 microns), while it begins at 3∅ (125 microns) in Outcrop B. This is an indication that the medium energy of Outcrop A was higher due to the suspension of larger particle sizes. The particle-size parameters also support the finding. The mean and median of the particle sizes reveal that the particles of Outcrop A are coarser than the particles of Outcrop B. The variation of the saltation curves of Outcrop B (Figure (b)) is another indication of the low medium energy of transporting the particles.
Figure 6. Visher diagram (log-probability plot) representing suspension and saltation modes of (A) Outcrop A and (B) Outcrop B (modified from [Citation8]).
![Figure 6. Visher diagram (log-probability plot) representing suspension and saltation modes of (A) Outcrop A and (B) Outcrop B (modified from [Citation8]).](/cms/asset/28493867-3bba-453d-9afd-1c77d763a1b0/tusc_a_2354570_f0006_oc.jpg)
4. Conclusions
The particle-size analysis was implemented on two Biyadh Formation outcrops, where fresh representative friable sandstone samples were collected from ten beds from each outcrop.
The results of the particle-size analysis show a variation between the outcrops. The average results indicate that Outcrop A is composed of near-symmetrical and mesokurtic, moderately-well-sorted, medium-grained sandstone (with a narrow variation). While Outcrop B consists of near-symmetrical to leptokurtic moderately-well-sorted medium- to fine-grained sandstone (with a wide variation).
All the sandstone beds of Outcrop A and B are unimodal, which peaks mostly between 2∅ and 3∅.
Since the particles in Outcrop B are finer, it was expected to find a higher suspension transportation level than rolling compared to Outcrop A, which was supported by the Visher and Passega diagrams.
Three main depositional environments were recognized in both outcrops, including beach, aeolian, and fluvial environments, which were confirmed by the LDF methods.
Supplemental Material
Download MS Word (2 MB)Disclosure statement
No potential conflict of interest was reported by the author(s).
References
- Baiyegunhi C, Liu K, Gwavava O. Grain size statistics and depositional pattern of the Ecca Group sandstones, Karoo Supergroup in the Eastern Cape Province, South Africa. Open Geosci. 2017;9(1):554–576.
- Khalil R. Grain-Size Analysis of Middle Cretaceous Sandstone Reservoirs, the Wasia Formation, Riyadh Province, Saudi Arabia. Sustainability. 2023;15(10):7983.
- Kasim SA, Ismail MS, Ahmed N. Grain size statistics and morphometric analysis of Kluang-Niyor, Layang-Layang, and Kampung Durian Chondong Tertiary Sediments, Onshore Peninsular Malaysia: Implications for paleoenvironment and depositional processes. J King Saud Univ Sci. 2023;35(2):102481.
- Blott, S., Grain size distribution and statistics package for the analysis of unconsolidated sediments by sieving or by laser granulometer. Grandistat 2000, 1-6.
- Folk RL, Ward WC. Brazos River bar [Texas]; a study in the significance of grain size parameters. J Sediment Res. 1957;27(1):3–26.
- Passega R. Texture as characteristic of clastic deposition. AAPG Bulletin. 1957;41(9):1952–1984.
- Passega R. Grain size representation by CM patterns as a geologic tool. J Sediment Res. 1964;34(4):830–847.
- Visher GS. Grain size distributions and depositional processes. J Sediment Res. 1969;39(3.
- Khanam S, Khan K, Quasim M, et al. Proterozoic sandstone of Rajgarh Formation, Alwar sub-basin, Northeastern Rajasthan: sedimentological and paleo-hydrodynamical implications. J Sediment Environ. 2022;7(2):261–282.
- Ahmad F, Quasim M, Ahmad A, et al. Depositional mechanism of fort member sandstone (early-late bathonian), jaisalmer formation, western rajasthan: Insights from granulometric analysis. Geol Ecol Landsc. 2021;5(2):119–135.
- Quasim MA, Ghosh SK, Ahmad AHM, et al. Integrated approach of lithofacies and granulometric analysis of the sediments of the Proterozoic Upper Kaimur Group of Vindhyan Supergroup, Son Valley, India: Palaeo-environmental implications. Geol J. 2020;55(9):5991–6012.
- Boggs S. Principles of sedimentology and stratigraphy. 2012.
- Friedman GM. Distinction between dune, beach, and river sands from their textural characteristics. J Sediment Res. 1961;31(4):514–529.
- Powers R, Ramirez L, Redmond C, et al. Geology of the Arabian Peninsula. United States Department of the Interior, Geological Survey; 1966.
- Steineke, M.; Bramkamp, R.; Sander, N., Stratigraphic relations of Arabian Jurassic oil: Middle East. 1958, In: Weeks, L.G. (Ed.), Habitat of Oil. American Association of Petroleum Geologists Special Publication 18, 1294-1329.
- Abdulrazzak MJ. Ground water system evaluation for Wadi Nisah, Saudi Arabia. 1976.
- Entsminger, L. D. In Sedimentary response to tectonic and eustatic changes: an example from the mid-Cretaceous Wasia Formation, Saudi Arabia, Middle East Technical Conference and Exhibition, 1981; OnePetro: 1981.
- Moshrif MA. Recognition of fluvial environments in the Biyadh-Wasia sandstones (Lower-Middle Cretaceous) as revealed by textural analysis. J Sediment Res. 1980;50(2):603–612.
- Soliman F, Al Shamlan A. Review on the geology of the Cretaceous sediments of the Rub'al-Khali, Saudi Arabia. Cretaceous Res. 1982;3(1-2):187–194.
- Le Nindre Y-M, Vaslet D, Maddah SS, et al. Stratigraphy of the Valanginian? to Early Paleocene succession in central Saudi Arabia outcrops: Implications for regional Arabian sequence stratigraphy. GeoArabia. 2008;13(2):51–86.
- Alsharhan A, Nairn A. A review of the Cretaceous formations in the Arabian peninsula and gulf: Part II. mid-Cretaceous (Wasia Group) stratigraphy and paleogeography. J Pet Geol. 1988;11(1):89–112.
- Keller M, Bohnsack D, Koch R, et al. Outcrop Analog Studies of the Wasia—Biyadh and Aruma Aquifers in the Kingdom of Saudi Arabia. 2019.
- Gomaah M, Al-Bassam A. Geochemistry and origin of the hyper salinity of groundwater in Wasia-Biyadh Aquifer, Saudi Arabia. J Geosci Environ Prot. 2021;9(11):1–14.
- Vaslet, D.; Al-Muallem, M.; Maddeh, S.; Brosse, J.; Fourniquet, J.; Breton, J.; Le Nindre, Y., Explanatory notes to the geologic map of the Ar Riyad Quadrangle, Sheet 24 I, Kingdom of Saudi Arabia. Saudi Arabian Deputy Ministry for Mineral Resources, Jeddah, Geosciences Map, GM-121 1991, 54.
- Alshehri F, Mohamed A. Analysis of groundwater storage fluctuations using GRACE and remote sensing data in Wadi As-Sirhan, northern Saudi Arabia. Water (Basel). 2023;15(2):282.
- Al-Ghamdi, N. M. Facies, Sequence Framework, and Evolution of Rudist Buildups, Shu'aiba Formation, Saudi Arabia. Virginia Tech, 2006.
- Hughes G. Saudi Arabian Late Jurassic and Early Cretaceous agglutinated foraminiferal associations and their application for age, palaeoenvironmental interpretation, sequence stratigraphy, and carbonate reservoir architecture. Grzybowski Foundation Special Publication. 2000;7:149–165.
- Hakimi MH, Shalaby MR, Abdullah WH. Diagenetic characteristics and reservoir quality of the Lower Cretaceous Biyadh sandstones at Kharir oilfield in the western central Masila Basin, Yemen. J Asian Earth Sci. 2012;51:109–120.
- Khan AM, Al-Juhani SG, Abdul S. Digital viscoelastic seismic models and data sets of central Saudi Arabia in the presence of near-surface karst features. J Seism Explor. 2020;29:15–28.
- Krumbein W, Monk G. Permeability as a function of the size parameters of unconsolidated sand. Trans. AIME. 1943;151(01):153–163.
- Sahu BK. Depositional mechanisms from the size analysis of clastic sediments. J Sediment Res. 1964;34(1):73–83.
- Baiyegunhi TL, Liu K, Gwavava O, et al. Textural characteristics, mode of transportation and depositional environment of the Cretaceous sandstone in the Bredasdorp Basin, off the south coast of South Africa: Evidence from grain size analysis. Open Geosci. 2020;12(1):1512–1532.
- Friedman GM. Dynamic processes and statistical parameters compared for size frequency distribution of beach and river sands. J Sediment Res. 1967;37(2):327–354.
- Yang H, Shi C. Sediment grain-size characteristics and its sources of ten wind-water coupled erosion tributaries (the Ten Kongduis) in the Upper Yellow River. Water (Basel). 2019;11(1):115.
- Yadav S, Kanhaiya S, Singh S, et al. Facies architecture and textural attributes of the Late Quaternary cliff embankment sections of the Sai River, Central Ganga Plain, India. Geosys Geoenviron. 2023;2(4):100216.
- Ahmad F, Quasim M, Ghaznavi A, et al. Depositional environment of the Fort Member of the Jurasic Jaisalmer Formation (western Rajasthan, India), as revealed from lithofacies and grain-size analysis. Geologica Acta. 2017;15(3):0153–0167.
- Ghaznavi AA, Quasim M, Ahmad A, et al. Granulometric and facies analysis of Middle–Upper Jurassic rocks of Ler Dome, Kachchh, western India: an attempt to reconstruct the depositional environment. Geologos. 2019;25(1):51–73.
- Moiola R, Weiser D. Textural parameters; an evaluation. J Sediment Res. 1968;38(1):45–53.
- Tijani MN, Nton ME, Kitagawa R. Textural and geochemical characteristics of the Ajali Sandstone, Anambra Basin, SE Nigeria: implication for its provenance. CR Geosci. 2010;342(2):136–150.
- Passega R, Byramjee R. Grain-size image of clastic deposits. Sedimentology. 1969;13(3-4):233–252.