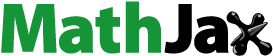
ABSTRACT
Introduction
There has been an active discussion on the sustainability of inhaler therapy in respiratory diseases, and it has cast a shadow on pMDIs which rely on propellant with high global warming potential (GWP). DPIs offer a lower GWP and effective alternative, but there has been concern whether all patients can generate sufficient inspiratory effort to disperse the drug. This review focuses on airflow resistance of DPIs and its clinical relevance.
Areas covered
For this narrative review, we searched the literature for studies comparing flow patterns with different devices. We also included a section on clinical trials comparing reliever administration with DPI, pMDI with spacer, and nebulizer during exacerbation.
Expert opinion
The evidence supports the efficacy of DPIs irrespective of respiratory condition or age of the patient even during acute exacerbations. Air flow resistance does not limit the use of DPIs and the patients were able to generate sufficient inspiratory flow rate with almost any device studied. None of 16 identified clinical trials comparing reliever administration via DPIs to other types of devices during exacerbation or bronchial challenge showed statistically significant difference between the device types in FEV1 recovery. DPIs performed as well as other types of inhaler devices even during asthma or COPD exacerbation.
1. Introduction
1.1. Search strategy
Medline, biosis, ipa, ddfu, hcaplus, embase, and scisearch were searched for peer-reviewed articles published during current millennium with key words ‘inhaler resistance,’ ‘inspiratory flow rate,’ ‘DP,I’ ‘dry powder inhaler’ written in English. Authors also identified relevant articles from their own files for this review. Additionally, relevant articles from the references of identified articles were also included to allow citing original works when discussed in the review.
1.2. Introduction to dry powder inhalers and fluid mechanics
The current inhaler types on the market have vastly different operating methods and inhalation technique. Correct use of inhaler devices is of paramount importance for successful therapy and ease of use has been associated with better treatment adherence [Citation1,Citation2]. Dry powder inhalers (DPIs) are passive devices which rely on patients’ inspiratory effort for drug dispersion and delivery. Propellant containing pMDIs use pressurized gas for dispersion but require coordination between inhaler actuation and inhalation. Soft mist inhalers (SMIs) disperse the formulation mechanically. Generally, pMDIs and SMIs require slow and deep inhalation against low device resistance while DPIs require fast and deep inhalation against higher resistance. Due to the propellant released into the atmosphere, pMDIs may have a significantly greater carbon footprint than the other devices [Citation3].
In clinical practice, the needs of patients vary. Therefore, when considering the clinical evidence not all the patient groups are equal. While patients with asthma not experiencing exacerbation usually have similar pulmonary function compared to healthy volunteers this is not the case for a patient with COPD who typically have limited pulmonary function. In addition, paediatric patients are also considered to be in risk of insufficient inspiratory effort.
shows the components of the patient-inhaler system. Figures describing lower flow characteristics of airways are based on computational modeling by Zarei et al [Citation4]. The basic components of current commercial DPI formulations usually consist of coarse lactose carriers and fine particles of active pharmaceutical ingredient (API). A DPI is a device that allows the patient to disperse the formulation into respirable particles by detaching the small API particles from large lactose carriers. This is achieved by directing the inspiratory airflow generated by the patient to the drug formulation, as well as usually increasing velocity and turbulence of the airflow by introducing orifices and meshes, for example, into the device design. Detachment is caused by drag and lift forces acting on the particles as well as collisions of particles to each other and against the inhaler inside walls.
To understand device air flow resistance, we must first look into basic fluid mechanics. There has been a plethora of discussion whether the internal airflow resistance of DPIs is beneficial or detrimental, but very little of the importance and relevance of device resistance to the device design. The resistance results from manipulation of airflow patterns inside the device. For example, shows a computational fluid dynamics simulation of a DPI flow channel, where an orifice is placed in front of the dose cup similarly when the end of a gardening hose is squeezed. From the figure, it is seen that the warmer colors indicating higher flow are concentrated near the orifice and the closely positioned dose cup. Constriction of the flow channel accelerates the flow due to Bernoulli’s effect and provides high velocity flow facilitating deagglomeration of the formulation. At the same time, squeezing the flow through the restrictive orifice increases the device resistance and limits the total flow rate. Resistance in inhalers is therefore not a passive design property, but purposefully introduced to achieve a specific goal in the design of the device.
Figure 2. Flow rate is accelerated for the formulation dispersion by orifice in the standard Easyhaler DPI as shown by computational fluid dynamics simulation.
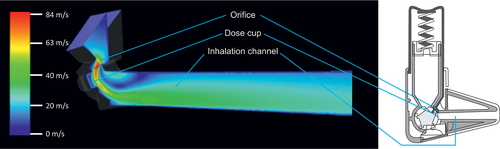
Energy wise, the inhaler device converts the available energy from the airflow to the deaggregation of the small API particles from the large carrier particles. Clarck & Hollingworth [Citation5] provide the relationship of pressure and flow rate within a DPI for turbulent flow:
where ∆p is the pressure drop between mouth cavity and surrounding air (inspiratory pressure), Q is the volumetric flow rate and R is the intrinsic flow resistance of the inhaler. To consider the energy available for deaggregation of formulation, we can study vacuum power of the airflow. Power is defined as energy per unit of time and is associated with inspiratory pressure and flow rate. Harris and Dunbar [Citation6–8] derived equations for vacuum power P of the airflow
and by substituting ∆p with EquationEq. (1(1)
(1) )
The Equationequations (2)(2)
(2) and (Equation3
(3)
(3) ) give the total power output generated by the inspiratory effort of the patient for the deagglomeration of the drug powder in DPI, but it is up to the device engineers to use the energy in an efficient way. Hira et al[Citation9] studied the effect of device resistance using simulated real-life flow profiles and three variants of the same inhaler with varying resistances. They measured the respirable API content and found out that with equal power level, the highest resistance device produced largest amount of respirable particle indicating it used the energy provided by patient’s inspiratory effort in the most efficient way [Citation9].
DPIs with higher intrinsic resistances are less prone to oropharyngeal deposition than devices with lower resistance due to lower flow rate in the upper airways [Citation10,Citation11]. Particles with too large aerodynamical diameter are unable to follow the streamlines of the air flow and are deposited in the upper respiratory track, mainly oropharyngeal region. This is called inertial impaction. From the work of Stokes [Citation12], a property called impaction factor is derived. It is a mathematical expression of the most important factors affecting the deposition to the upper respiratory track and can be expressed as [Citation13]
where IF is the impaction factor and da is the aerodynamical diameter of the drug particle. From this equation, it can be seen that the probability of impaction increases with aerodynamical diameter and flow rate. Therefore, the energy available for powder deagglomeration increases with increasing flow rate, but so does the drug losses in oropharyngeal region due to impaction [Citation14]. Assuming constant aerodynamical diameter for the drug particles, the Equationequation (4)(4)
(4) suggests that higher resistance resulting in lower flow rate would lead to lower impaction in upper respiratory tract while maintaining sufficient energy for dispersion. In real-life varying device, geometries and flow patterns at the inhaler mouthpiece are suggested to play an important role also in the deposition patterns [Citation13,Citation15]. A schematic representation of patient-device system is represented in . As seen from the figure, the lowest crossectional area and, therefore, the highest flow rate are in the bronchus-level.
While increase in deagglomeration counterbalances the oropharyngeal losses with most devices, it is possible that the oropharyngeal filtering increases faster than the powder deagglomeration and it will result in decrease of lung dose. This is called negative flow dependence [Citation16]. For the commercial devices, it is a concern with at least budesonide Airmax, the Onbrez Breezhaler, and Spiriva HandiHaler as reviewed by Clark et al [Citation16]. This reflects to the Handihalers inhalation instructions in the patient instructions leaflet, which does not encourage as forceful inhalation as other medium-high to high-resistance inhalers [Citation17].
In addition to flow rate, inspiratory pressure has been used to describe the inhalation effort during the drug administration. Clark et al. argue that for most DPIs adequate lung deposition occurs when Peak inspiratory pressure (PIP) is > 1 kPa [Citation16]. Similarly to the flow rates, the inspiratory pressure is not device independent, but is heavily influenced by the inhaler resistance. For example, Sahay et al [Citation18]. used the same device design modified to induce different resistance levels. In a crossover study involving 18 patients with pulmonary arterial hypertension, PIP increased from 3.7 kPa to 6.5 kPa when device resistance was increased from 0.019 to 0.050
(i.e. from low to high) [Citation18]. Similarly, in the study by Altman et al., patients with COPD used Breezehaler, Ellipta, and Handihaler. PIP increased with the device resistance from 3,37 kPa with resistance of 0.017 to 5,30 kPa with resistance of 0.047
[Citation19]. However, none of these studies addressed the disease outcomes. Currently, there is no universal variable to reflect patients’ inspiratory capabilities independently from the device. From clinical point of view, it means that the patients capability for sufficient inspiratory effort needs to be measured for each resistance level separately and commonly recognized pressure drop standards such as 4 kPa and 2 kPa describe different kinds of inhalation in different devices and are not in any way comparable.
Flow acceleration and inhalation volume are often regarded as factors of quality of the inhalation maneuver, but there is little evidence on their effect on drug dose and deposition patterns. However, it seems that they do not limit the use of DPIs among patients and there does not seem to be a clear association with the device resistance [Citation20–24].
2. Inspiratory flow patterns in stable patients
The ability of patients to generate sufficient flow rates to operate DPIs has been extensively studied using various methods. A summary of the results reviewed for this work is presented in . There are several ways to assess the PIF of the patient. The most common experimental setup is to use an inhaler training device, the In-Check Dial, which has adjustable resistance and can be tuned to resemble most of the DPIs using one of the pre-set resistance levels (R0-R5) ranging from pMDI to high resistance DPI. As it is the only commercially available device on the market, it has been extensively used both in research and real life. The other option is to use customized spirometer setup or pressure sensors and measure flow rates or pressure drops directly from the inhaler. With In-Check dial, the resistance can be adjusted independently from other factor making it easier to study the effect of resistance. However, the resistance does not exactly match the actual inhaler devices and other features such as mouthpiece geometry is omitted. Also, the wording of the inhalation instructions plays a large role on patients’ inspiratory effort and therefore, the measurements with the In-Check Dial are only applicable to an inhaler with similar instructions. This is evident from a study by Sahay et al. where using a high resistance device with a resistance similar to R5 of the In-Check Dial the patients were asked to inhale with maximum effort their peak inspiratory effort was 51 L/min and 29 L/min when they were asked to inhale comfortably [Citation18]. Additionally, the audible feed backs of capsule vibration or inhaler clicks are missing when it is used to reflect capsule-based devices such as Aerolizer, Handihaler, and Novolizer.
Table 1. Resistances, flow requirements, and In-Check Dial resistance levels for various commercial inhalers [Citation25,Citation27–30,Citation34].
There is significant variation in the results of studies which can be at least partly addressed to different definitions of sufficient or optimal flow rates. For the purposes of this work, we have tried to calculate comparable numbers wherever sufficient data are available. There are few literature reviews on inhaler devices and their inspiratory flow requirements [Citation25–27]. A synthesis of device resistances, lower limit for optimal flow rates, corresponding In-Check Dial resistance levels, and inhalation instructions are presented in the . The flow requirements in the are based on the work by Pohlman et al. [Citation28] However, the interpretation of the data to find limits of optimal inhalation is not straightforward and often depends on which endpoint the author emphasizes. For example, Haidl et al. provide set of device requirements that differ from those presented here [Citation26]. Also, the manufacturers provide their own recommendations for their devices. The conclusions drawn using the data from are, therefore, open to interpretation. The optimal flow rate should not be considered as best possible flow rate for given inhaler, but as a lower limit from where the device will reliably work. Due to different instructions and device-specific flow rate requirements, many of the results need to be interpreted differently with each specific device in mind. For the purpose of this work, the pressure drops have been converted to flow rates and vice versa when needed using EquationEq. (1)(1)
(1) and . The resistance ranges according to ISAM/ERS taskforce [Citation10] and In-Check Dial resistance ratings are used somewhat interchangeably in the discussion in this work. However, it must be noted that the ISAM/ERS taskforce defines resistance ranges for device classifications while In-Check Dial features only single unpublished resistance from each class.
Table 2. Percentage of patients who successfully performed the inhalation manoeuvre with flow rate requirements according to Table 2.
Medium-low (R2) resistance inhalers such as Ellipta and Diskus generally require inspiratory flow of ≥60 L/min to operate properly. In a population of 213 patients studied by Mahler et al, 19% were unable to produce sufficient inspiratory effort [Citation31]. In a similar setting reported by Ghosh et al., the prevalence of insufficient inspiratory effort was 44% [Citation32]. In a striking contrast to Mahler et al and Ghosh et al, in a study by Chen et al, 138 patients with COPD at resistance level R2 were generally able to generate sufficient flow rate, but over 40% of the patients generated flow rate of > 90 L/min, which is considered excessive and may lead to increased oropharyngeal deposition [Citation33]. While the reasons for differences in results cannot be deducted from the reports of these works, a possible explanation may lie in how the patients are instructed and encouraged to inhale on the investigation site. As seen before, inhalation instructions play large role in the performance of the patients and in clinical practice the importance of encouragement on respiratory performance is evident when performing e.g. spirometries and PEF-measurements.
The flow rate requirements range from 30 L/min for center slot Easyhaler to 60 L/min for Turbuhaler Pulmicort in medium-high resistance group (R4 for InCheck Dial). In the aforementioned study by Chen et al., success rates for these inhalers were 97% for Easyhaler and 66% for Turbuhaler. The high dependence of delivered dose to inspiratory flow rate below 60 L/min is a property which Turbuhaler has been criticized for [Citation26,Citation34]. If the inspiratory flow rate of the patient decreases due to a worsening clinical condition, it may lead to reduced drug delivery in the flow rates below 60 L/min when using Turbuhaler. Similar results are seen in a study of 47 patients hospitalized for acute exacerbation of COPD where the mean PIFR at R5 was approximately 40 L/min and at R4 approximately 50 L/min at the time of discharge showing that the variation in R4 flow requirements is crucial for patient performance, but low flow rate requirement of R5 group is satisfied by most patients even after hospitalization for exacerbation [Citation35].
Haughney et al studied a population of over 900 asthmatic patients using the In-Check Dial with a flow rate requirement of 30–90 L/min for successful inhalation with instruction to breath in strongly and deeply [Citation36]. With R5 resistance level 94% of patients were able to perform successful inhalation. The success rate with pMDI resistance R0 (20–60 L/min for successful inhalation) was only 71%. With the fact that 20% of those currently used pMDI failed with R0 in study indicates that a high resistance DPI may be more intuitive to use for the patient than a pMDI.
Ghosh et al. also studied InCheck Dial resistance R5 with instructions to inhale as from Handihaler and insufficient PIFR was present with 32% of the users [Citation32]. The large difference in failure rate compared to 6% in Haughney et al. highlights the importance of inhaling instructions. It is likely that the high failure rate with R5 is largely associated with Handihalers instructions, which promote gentler inhalation. The direct effect of Handihalers inhaling instructions is seen in a crossover study by Jõgi et al. where the mean PIFs with In-Check Dial resistance R5 were 50.1 L/min with Easyhaler’s instructions promoting strong inhalation and 40.7 L/min with Handihaler’s instructions suggesting gentler inhalation. However, even with Handihaler’s instructions, the results show a healthy margin to required 30 L/min with only one patient failing to achieve it [Citation37].
In addition to the In-Check Dial, the inspiratory effort of the patients has been studied also with marketed inhalers directly. A recent study Malmberg et al. pooled inspiratory profiles of 102 children with asthma, 185 adults with asthma and 96 patients with COPD through medium-high (R4) resistance center slot Easyhaler. In all groups, only one subject failed to achieve sufficient peak inspiratory flow rate of 30 L/min [Citation38]. Similarly, in the crossover study by Jõgi et al., 100 healthy volunteers and 100 COPD patients were examined with three inhalers: a medium-high resistance center slot Easyhaler and high resistance standard Easyhaler and Handihaler. Only 1 patient and 1 healthy volunteer failed to achieve sufficient inspiratory flow rate of 30 L/min with Handihaler [Citation37].
The patients seem to handle the devices in the lower end of the resistance range as well, since in a study examining patients with moderate-to-severe COPD with three different inhalers mean PIF values were 108 L/min for Breezhaler (low resistance, R1), 78 L/min for Ellipta (medium-low, R2), and 49 L/min for HandiHaler (high, R5) which are all more than sufficient for the studied inhalers [Citation19]. Especially with Breezhaler, the PIF is more likely to be excessive than insufficient leading to deposition in upper airways.
Paediatric populations have also been represented in studies. 60 children and 29 adults with cystic fibrosis used devices ranging from medium low to high. Aside from 1 subject in 6–10-year-old group, all the patients were able to generate PIFR of >30 L/min even with the high resistance devices [Citation39]. In a study including 3- to 6-year-old children who were able to reliably inhale from DPI 87% achieved an PIFR of > 60 L/min with R2 simulating Diskus, and 72% generated PIFR of > 60 L/min with R4 simulating Turbuhaler [Citation40]. If the flow rate limit of >30 L/min would have been used 96% of the children would have succeeded in the inhalation. For Turbuhaler, the numbers are similar to those observed with adults by Chen et al. [Citation33] and relaxing flow requirement to that of most medium-high resistance DPIs (>30 L/min) resulted in dramatic increase of success rate as was seen with the adults [Citation40]. It is also supported by study by Amirav et al. where inspiratory flow rates are reported instead of success rates allowing more detailed analysis [Citation41]. When 100 children between ages of 3 and 15 years and previous DPI use experience were using either Turbuhaler or Diskus, all of them were able to generate more than 30 L/min with both of the devices. However, only 68% were able to generate over 60 L/min with Turbuhaler. The proportion of the patients able to perform successful inhalation with medium-high resistances is rather stable across the studies indicating that 30 L/min with medium-high resistance is easily achievable for all patient groups while roughly third of the patients will fail to achieve 60 L/min regardless of the age or disease.
From these studies, we can conclude that the vast majority of stable patients are able to use DPIs and the required inspiratory flow rates are not a clinical concern. As seen from when patients are using medium-high to high resistance device with clear instructions to use maximum inspiratory effort they can consistently generate flow rate of > 30 L/min. The next question is the whether the patients experiencing exacerbations can use their device effectively. Fortunately, there are several studies with similar methodology on hospitalized patients.
3. Inhaler use during exacerbations
3.1. Inspiratory flow patterns during exacerbations
GINA[Citation42] and GOLD[Citation43] guidelines do not currently recommend any delivery device over others in treatment of acute exacerbations. GINA states that delivery of short acting β-agonists via a pMDI and spacer or a DPI leads to a similar improvement in lung function as delivery via nebulizer. Broeders et al. studied 15 patients hospitalised for asthma or COPD exacerbation [Citation44]. All of these patients were able to produce inspiratory flow rate of 30 L/min with both Diskus and Turbuhaler. However, again only approximately 60% of the patients were able to generate 60 L/min flow rate using Turbuhaler. The problem was especially prevalent during the first five days of hospitalization. Interestingly, only 14% of patients with pMDI and 87% of patients with pMDI and spacer performed adequate inhalations indicating that especially pMDI alone is unsuitable for treatment of exacerbations. Further, it was shown that salbutamol administration via pMDI alone provided significantly less protection against methacholine induced obstruction than salbutamol administered via DPI or pMDI with spacer [Citation45].
PIFR was measured among 44 patients admitted to hospital for acute exacerbation of COPD. Upon admission 90.9%, 100%, 95.5%, and 81.8% of the patients were able to generate PIFR of >30 L/min for R2, R3, R4 and R5, respectively. Upon discharge the success rates were 100%, 100%, 97.7%, and 95.5%, respectively [Citation46]. It has to be noted that at R5 the patients were instructed to inhale with Handihalers instructions which has been shown to provide lower inspiratory flow rates than with more prompt instructions and is not directly applicable to other inhalers in R5 high resistance range [Citation37]. Also, if the results are to be viewed in context of Diskus and Turbuhaler with PIFR requirement of 60 L/min 25% of patients fail with Diskus upon admission and with Turbuhaler it is even worse. As the results of individual subjects are not reported more detailed analysis of the study is not possible. Still, the vast majority of the patients were able to use any DPI even when hospitalized. As with adults, there is very limited data on patient performance during disease exacerbations in pediatric population. In a study of thirty-three 6–18-year-old children with acute asthma exacerbation generated more than sufficient mean PIFR of 62.1 L/min with R4 and 72.6 L/min with R2, which increased to 74.4 L/min and 91.1 L/min, respectively, upon discharge [Citation47]. To .conclude, the patients are generally able to generate PIFR of 30 L/min with any device and most can reach 60 L/min with medium-low resistance devices even during exacerbations.
The literature on relevance of device resistance to clinical outcomes is scant. The device resistance is a property which is difficult to isolate from the general design of the inhaler device or from how it aerosolizes the dry powder formulation. Therefore, designing a formal study to address resistance only is difficult if not impossible.
3.2. Clinical trials
While flow patterns are useful and indicate correct use of the inhalers in the end the important thing is how the patients fare especially during exacerbations and therefore, clinical end points have to be considered as well. A Cochrane review in 2016 comparing delivery methods of bronchodilators during exacerbations of COPD found the evidence to be both heterogenous and insufficient [Citation48]. For the purpose of this work, we identified 16 clinical trials comparing DPIs against either pMDI with spacer or nebuliser as they are the standard of care in centers treating acute exacerbations of asthma and COPD and there is a persisting concern among clinicians whether the DPI is a suitable administration method in these events. Studies where the different devices included different APIs were omitted, since it is not possible to differentiate between devices if the APIs differ. Turbuhaler, Aerolizer, Diskus and NEXThaler have been studied mainly in adult asthmatic patients using both salbutamol and formoterol as reliever therapy. No significant difference was found between DPI and pMDI and/or nebuliser when recovery of FEV1 was considered as the clinically relevant outcome measure [Citation49–59]. Tiotropium administration via Rotahaler DPI has also been compared against pMDI with similar efficacy [Citation60]. In ER-setting, there was no difference between pMDI or nebuliser and NEXThaler, Aerolizer, or Rotahaler in asthmatic adults or Rotahaler or Easyhaler in asthmatic children compared to pMDI with spacer or nebulizer [Citation61–64]. For the purpose of this work, only clinical trials on bronchodilators were considered since the effect of those can be directly measured. As the mechanism of action is different with other drug classes, it is possible that the results cannot be directly extrapolated to cover all drug classes used as inhaled medications.
4. Conclusions
The literature on flow patterns of DPIs show that the patients can generate sufficient inspiratory effort regardless of the internal airflow resistance of the device. Especially with the higher end of the resistance spectrum with clear instructions for maximum inspiratory effort the patients can consistently fulfil the flow rate requirements. As a result, it could be beneficial to use higher resistance devices to also avoid oropharyngeal deposition of the drugs due to excessive flow rates. However, when interpreting success rates with different devices it needs to be noted that each study was carried out in a different study subject population and the results are not directly comparable. For the purpose of this work, the authors identified 16 clinical studies comparing inhaled reliever therapy via DPI to either pMDI with spacer or nebuliser. None of the studies showed statistically significant difference between the administration methods. Based on the literature GINA and GOLD guidelines could be updated to include DPI is a delivery method also during exacerbations. From these results in clinical trials as well as from the flow characteristics presented earlier, we can conclude that in general resistance of an inhaler does not limit its use among patients despite their clinical condition. In clinical practice, the patients can rely on their DPIs and trust them to perform well even during exacerbations of their respiratory diseases.
5. Expert opinion
Increasing awareness of environmental impact of the inhaler treatment among both patients and clinicians is likely to shift the focus from pMDIs to DPIs as the carbon footprint of DPIs is only marginal (approximately 95% less) compared to that of pMDIs. DPIs are passive devices which rely on the patient’s inspiratory effort for drug dispersion and delivery. Propellant containing pMDIs instead use pressurized propellant gas for dispersion. Some companies are investigating more environmentally friendly propellants for pMDIs. If the development programs are successful, we are likely to see research programs aiming for their market authorization. However, the new programs are likely to be bioequivalence studies focusing in showing non-inferiority compared to the originator product and therefore, are not likely provide any new insights into inhaler device technology, inhaler use or patient training, or inhaler treatment in general.
As some key patents for medications used for treatment of chronic respiratory disease (such as tiotropium, indacaterol-glycopyrronium fixed combination, and vilanterol-umeclidinium fixed combination) are expiring or about to expire during the next five years, we are likely to see new generic devices entering the markets. These new entries will increase the number of different devices on the market and make the treatment more affordable due to generic competition. The new devices will increase the flexibility of the inhaler treatment in terms of device choices but also increase the amount of devices health care providers need to be familiar with and pose challenges to adequate patient training, adherence and finally achieving the desired treatment outcomes.
Lack of treatment adherence and inhaler use errors are both long-standing problems in of inhaler treatment among patients with chronic respiratory disease, and either may lead to treatment failure. Mixing different inhaler types or switching patients from one inhaler to another may induce confusion among patients and increase the probability of inhaler use errors. Instructions for correct use vary substantially among different inhalers and health care providers prescribing the devices and instructing the patients must be familiar with each device in use and have sufficient time to instruct the patient properly to ensure that the patient can use the inhaler device correctly. This poses a challenge for health care providers as they are increasingly pressed with time in their clinical practice. Hence, it would be beneficial if the patient could use one type of inhaler device with similar instruction and technique of use for the delivery of different inhaled medications. Products on the market, as per the market authorization process and related studies, are safe and effective, but this only if the inhaler treatment is performed correctly. This expert opinion paper aims to clarify these firstly, by increasing the understanding of basic fluid mechanics and the technical features of different inhaler devices with a step-by-step approach, and, secondly, by reviewing the overwhelming amount of evidence supporting the usability and efficacy of DPIs irrespective of the patient’s respiratory condition or age and even during the patient’s acute exacerbations. In clinical use, DPIs perform as well as other types of inhaler devices even during asthma or COPD exacerbation. A substantial body of evidence has shown that, given the proper instructions and training, patients are able to use different inhaler device types, the most used being dry powder inhalers (DPIs), and pressurized metered dose inhalers (pMDIs), despite the patient’s characteristics or disease severity. More importantly, patients’ inspiratory performance does not limit the use of any inhaler type. There are beliefs and misconception of high-resistance inhaler devices (DPIs) being difficult to use.
Article highlights
There is an increasing pressure to shift from pressurised metered dose inhalers to more environmentally friendly devices.
Inhaler resistance is a designed property of the inhaler.
Nearly all patients can generate sufficient inspiratory flow for the use of DPIs.
Exacerbation of obstructive pulmonary diseases do not seem to limit the use of DPIs.
During exacerbations DPIs performed as well as pMDIs and nebulizers in clinical trials.
This box summarizes key points contained in the article.
Glossary of terms and abbreviations
∆p, pressure change over the inhaler; API, active pharmaceutical ingredient; COPD, chronic obstructive pulmonary disease; da, Aerodynamical diameter; GWP, global warming potential; DPI, dry powder inhaler; IF, impaction factor; PIP, peak inspiratory pressure drop; P, power of the airflow; PIF, Peak inspiratory flow; PIP, Peak inspiratory pressure; pMDI, pressurized metered dose inhaler; Q, volumetric airflow; R, inhaler resistance
Declaration of interest
V Vartiainen has received lecture and consultant fees from Orion Pharma and was an employee of Orion Pharma during 2019–2021. F Lavorini has received grants for research or fees for speaking and advisory boards from AstraZeneca, Boehringer Ingelheim, Chiesi, GlaxoSmithKline, Menarini International, Novartis, Orion, and Trudel Medical International. A Murphy has received funding for research studies, consultancy agreements, and honoraria for presentations from AstraZeneca, Boehringer Ingelheim, Chiesi, GlaxoSmithKline, Orion, Sanofi, and Trudell Medical International. K Rabe has received Consultancy and speaker fees from Boehringer Ingelheim, AstraZeneca, Novartis, Chiesi, Sanofi, Roche, Regeneron, and Orion as well as grants from Boehringer Ingelheim and AstraZeneca. The authors have no other relevant affiliations or financial involvement with any organization or entity with a financial interest in or financial conflict with the subject matter or materials discussed in the manuscript apart from those disclosed.
Reviewer disclosures
Peer reviewers on this manuscript have received an honorarium from Expert Opinion on Drug Delivery for their review work, but have no other relevant financial relationships to disclose.
Additional information
Funding
References
- Price D, Harrow B, Small M, et al. Establishing the relationship of inhaler satisfaction, treatment adherence, and patient outcomes: a prospective, real-world, cross-sectional survey of US adult asthma patients and physicians. World Allergy Organ J. 2015;8:26.
- Gillette C, Rockich-Winston N, Kuhn JA, et al. Inhaler technique in children with asthma: a systematic review. Acad Pediatr. 2016;16(7):605–615.
- Benedick. Montreal protocol on substances that deplete the ozone layer medical and chemical technical options committee. 2018 Assessment report (2018) doi:10.1163/15718069620847781.
- Zarei S, Mirtar A, Andresen B, et al. Modeling the airflow in a lung with cystic fibrosis. J Non-Equilib Thermodyn. 2013;38(2). DOI:10.1515/jnetdy-2012-0019
- Clark ARR, Hollingworth AMM. The relationship between powder inhaler resistance and peak inspiratory conditions in healthy volunteers — implications for in vitro testing. J Aerosol Med. 1993;6:99–110.
- Harris D. Testing inhalers. Pharmaceutical technology Europe. 2007;29–35.
- Harris D. What are the implications of human lung power on dpi design? Respir Drug Delivery. 2006;2006:537–540.
- Dunbar CA, Morgan B, Van Oort M, et al. A comparison of dry powder inhaler dose delivery characteristics using a power criterion. PDA J Pharm Sci Technol. 2000;54(6):478–484.
- Hira D, Okuda T, Ichihashi M, et al. Influence of peak inspiratory flow rates and pressure drops on inhalation performance of dry powder inhalers. Chem Pharm Bull (Tokyo). 2012;60(3):341–347.
- Laube BL, Janssens HM, de Jongh FHC, et al. What the pulmonary specialist should know about the new inhalation therapies. Eur Respir J. 2011;37(6):1308–1417.
- Chrystyn H, Price D. What you need to know about inhalers and how to use them. Prescriber. 2009;20:47–52.
- Stokes GG. On the effect of the internal friction of fluids on the motion of pendulums. Mathematical and Physical Papers. Cambridge University Press. 2009; 1–10. doi: 10.1017/CBO9780511702266.002.
- Weers JG, Son Y-J, Glusker M, et al. Idealhalers versus realhalers: is it possible to bypass deposition in the upper respiratory tract? J Aerosol Med Pulm Drug Deliv. 2019;32(2):55–69.
- Cheng YS. Aerosol deposition in the extrathoracic region. Aerosol Sci Technol. 2003;37(8):659–671.
- de Boer AH, Hagedoorn P, Hoppentocht M, et al. Dry powder inhalation: past, present and future. Expert Opin Drug Deliv. 2017;14(4):499–512.
- Clark AR, Weers JG, Dhand R. The confusing world of dry powder inhalers: it is all about inspiratory pressures, not inspiratory flow rates. J Aerosol Med Pulm Drug Deliv. 2020;33(1):1–11.
- Spiriva Patient information leaflet.
- Sahay S, Holy R, Lyons S, et al. Impact of human behavior on inspiratory flow profiles in patients with pulmonary arterial hypertension using AOSTM dry powder inhaler device. Pulm Circ. 2021;11(1):1–9.
- Altman P, Wehbe L, Dederichs J, et al. Comparison of peak inspiratory flow rate via the Breezhaler®, Ellipta® and HandiHaler® dry powder inhalers in patients with moderate to very severe COPD: a randomized cross-over trial. BMC Pulm Med. 2018;18(1):100.
- Azouz W, Chetcuti P, Hosker HSR, et al. The inhalation characteristics of patients when they use different dry powder inhalers. J Aerosol Med Pulm Drug Deliv. 2015;28(1):35–42.
- Haikarainen J, Vahteristo M, Lähelmä S, et al. Patient inspiratory maneuver performance; peak lungpower, acceleration and volume. J Aerosol Med Pulm Drug Deliv. 2020;33(6):305–313.
- Delvadia RR, Wei X, Longest PW, et al. In vitro tests for aerosol deposition. IV: simulating variations in human breath profiles for realistic DPI testing. J Aerosol Med Pulm Drug Deliv. 2016;29(2):196–206.
- Lexmond AJ, Hagedoorn P, Frijlink HW, et al. Prerequisites for a dry powder inhaler for children with cystic fibrosis. PLoS One. 2017;12(8):e0183130.
- Bagherisadeghi G, Larhrib EH, Chrystyn H. Real life dose emission characterization using COPD patient inhalation profiles when they inhaled using a fixed dose combination (FDC) of the medium strength Symbicort® Turbuhaler®. Int J Pharm. 2017;522(1–2):137–146.
- Krüger P, Ehrlein B, Michael Zier RG, et al. Inspiratory flow resistance of marketed dry powder inhalers (DPI). Eur Respir J. 2014;44(Supp: 58, 4635).
- Haidl P, Heindl S, Siemon K, et al. Inhalation device requirements for patients’ inhalation maneuvers. Respir Med. 2016;118:65–75.
- Pohlmann G, Hohlfeld JM, Haidl P, et al. Assessment of the power required for optimal use of current inhalation devices. J Aerosol Med Pulm Drug Deliv. 2018;31(6):339–346.
- Ghosh S, Ohar JA, Drummond MB. Peak inspiratory flow rate in chronic obstructive pulmonary disease: implications for dry powder inhalers. J Aerosol Med Pulm Drug Deliv. 2017;30(6):381–387.
- Malmberg LP, Everard ML, Haikarainen J, et al. Evaluation of in vitro and in vivo flow rate dependency of budesonide/formoterol Easyhaler(®). J Aerosol Med Pulm Drug Deliv. 2014;27:329–340.
- Delvadia R, Hindle M, Longest PW, et al. In vitro tests for aerosol deposition II: IVIVCS for different dry powder inhalers in normal adults. J Aerosol Med Pulm Drug Deliv. 2013;26(3):138–144.
- Mahler DA, Waterman LA, Gifford AH. Prevalence and COPD phenotype for a suboptimal peak inspiratory flow rate against the simulated resistance of the Diskus ® dry powder inhaler. J Aerosol Med Pulm Drug Deliv. 2013;26(3):174–179.
- Ghosh S, Pleasants RA, Ohar JA, et al. Prevalence and factors associated with suboptimal peak inspiratory flow rates in COPD. Int J Chron Obstruct Pulmon Dis. 2019;14:585–595.
- Chen S-Y, Huang C-K, Peng H-C, et al. Inappropriate peak inspiratory flow rate with dry powder inhaler in chronic obstructive pulmonary disease. Sci Rep. 2020;10(7271).
- Buttini F, Brambilla G, Copelli D, et al. Effect of flow rate on in vitro aerodynamic performance of NEXThaler ® in comparison with Diskus ® and Turbohaler ® dry powder inhalers. J Aerosol Med Pulm Drug Deliv. 2016;29(2):167–178.
- Price DB, Yang S, Wan Yau Ming S, et al. Physiological predictors of peak inspiratory flow using observed lung function resultS (POROS): evaluation at discharge among patients hospitalized for a COPD exacerbation. Int J Chron Obstruct Pulmon Dis. 2018;13:3937–3946.
- Haughney J, Lee AJ, McKnight E, et al. Peak inspiratory flow measured at different inhaler resistances in patients with asthma. J Allergy Clin Immunol Pract. 2021;9(2):890–896.
- Jõgi R, Mattila L, Vahteristo M, et al. Inspiratory flow parameters through dry powder inhalers in healthy volunteers and patients with Chronic Obstructive Pulmonary Disease (COPD): device resistance does not limit use in COPD. Int J Chron Obstruct Pulmon Dis. 2021;16:1193–1201.
- Malmberg LP, Pelkonen AS, Vartiainen V, et al. Patients with asthma or chronic obstructive pulmonary disease (COPD) can generate sufficient inspiratory flows via Easyhaler® dry powder inhaler: a pooled analysis of two randomized controlled trials. J Thorac Dis. 2021;13(2):621–631.
- Tiddens HA, Geller DE, Challoner P, et al. Effect of dry powder inhaler resistance on the inspiratory flow rates and volumes of cystic fibrosis patients of six years and older. J Aerosol Med. 2006;19(4):456–465.
- ADACHI YS, ADACHI Y, Itazawa T, et al. Ability of preschool children to use dry powder inhalers as evaluated by in-check meter. Pediatr Int. 2006;48(1):62–65.
- Amirav I, Newhouse MT, Mansour Y. Measurement of peak inspiratory flow with in-check dial device to simulate low-resistance (Diskus) and high-resistance (Turbohaler) dry powder inhalers in children with asthma. Pediatr Pulmonol. 2005;39(5):447–451.
- Global Initiative for Asthma. Global strategy for asthma management and prevention. 2021.
- Global Initiative for Chronic Obstructive Lung Disease. Global strategy for the diagnosis, management, and prevention of chronic obstructive pulmonary disease. 2020.
- Broeders MEAC, Molema J, Hop WCJ, et al. The course of inhalation profiles during an exacerbation of obstructive lung disease. Respir Med. 2004;98(12):1173–1179.
- Broeders MEAC, Molema J, Hop WCJ, et al. Salbutamol pMDI gives less protection to methacholine induced airway obstruction than salbutamol via spacer or DPI. Eur J Clin Pharmacol. 2005;60:837–841.
- Papaioannou AI, Herodotou Y, Tomos I, et al. Ability of using different dry powder inhalers during COPD exacerbations. Pulm Pharmacol Ther. 2018;48:211–216.
- Bentur L, Mansour Y, Hamzani Y, et al. Measurement of inspiratory flow in children with acute asthma. Pediatr Pulmonol. 2004;38(4):304–307.
- van Geffen WH, Douma WR, Slebos DJ, et al. Bronchodilators delivered by nebuliser versus pMDI with spacer or DPI for exacerbations of COPD. Cochrane Database Syst Rev. 2016;2016(8). DOI:10.1002/14651858.CD011826.pub2
- Seberová E, Oxis AA. (formoterol given by Turbuhaler) showed as rapid an onset of action as salbutamol given by a pMDI. Respir Med. 2000;94(6):607–611.
- Boonsawat W, Charoenratanakul S, Pothirat C, et al. Formoterol (OXIS) Turbuhaler as a rescue therapy compared with salbutamol pMDI plus spacer in patients with acute severe asthma. Respir Med. 2003;97(9):1067–1074.
- Balanag VM, Yunus F, Yang P-C, et al. Efficacy and safety of budesonide/formoterol compared with salbutamol in the treatment of acute asthma. Pulm Pharmacol Ther. 2006;19(2):139–147.
- Rubinfeld AR, Scicchitano A, Hunt A, et al. Formoterol Turbuhaler as reliever medication in patients with acute asthma. Eur Respir J. 2006;27(4):735–741.
- Chew KS, Kamarudin H, Hashim CW. A randomized open-label trial on the use of budesonide/formoterol (Symbicort®) as an alternative reliever medication for mild to moderate asthmatic attacks. Int J Emerg Med. 2012;5(16). DOI:10.1186/1865-1380-5-16
- Morice AH, Peterson S, Beckman O, et al. Therapeutic comparison of a new budesonide/formoterol pMDI with budesonide pMDI and budesonide/formoterol DPI in asthma. Int J Clin Pract. 2007;61(11):1874–1883.
- Singh D, Berg F, Leaker B, et al. Comparison of the effect of beclometasone/formoterol in asthma patients after methacholine-induced bronchoconstriction: a noninferiority study using metered dose vs dry powder inhaler. Br J Clin Pharmacol. 2019;85(4):729–736.
- Bousquet J, Huchon G, Leclerc V, et al. A randomized, double-blind, double-dummy, single-dose, efficacy crossover trial comparing formoterol-HFA (pMDI) versus formoterol-DPI (Aerolizer) and placebo (pMDI or Aerolizer) in asthmatic patients. Respiration. 2005;72(Suppl 1):6–12.
- Beasley R, Holliday M, Reddel HK, et al. Controlled trial of budesonide–formoterol as needed for mild asthma. N Engl J Med. 2019;380(21):2020–2030.
- Brusasco V, Canonica GW, Negro RD, et al. Formoterol by pressurized metered-dose aerosol or dry powder on airway obstruction and lung hyperinflation in partially reversible COPD. J Aerosol Med Pulm Drug Deliv. 2011;24(5):235–243.
- Lavorini F, Geri P, Luperini M, et al. Clinical and functional responses to salbutamol inhaled via different devices in asthmatic patients with induced bronchoconstriction. Br J Clin Pharmacol. 2004;58(5):512–520.
- Brashier B, Dhembare P, Jantikar A, et al. Tiotropium administered by a pressurized metered dose inhaler (pMDI) and spacer produces a similar bronchodilator response as that administered by a Rotahaler in adult subjects with stable moderate-to-severe COPD. Respir Med. 2007;101(12):2464–2471.
- Najafizadeh K, Sohrab Pour H, Ghadyanee M, et al. A randomised, double-blind, placebo-controlled study to evaluate the role of formoterol in the management of acute asthma. Emerg Med J. 2007;24(5):317–321.
- Direkwatanachai C, Teeratakulpisarn J, Suntornlohanakul S, et al. Comparison of salbutamol efficacy in children–via the metered-dose inhaler (MDI) with Volumatic spacer and via the dry powder inhaler, Easyhaler, with the nebulizer–in mild to moderate asthma exacerbation: a multicenter, randomized study. Asian Pac J Allergy Immunol. 2011;29(1):25–33.
- Raimondi AC, Schottlender J, Lombardi D, et al. Treatment of acute severe asthma with inhaled albuterol delivered via jet nebulizer, metered dose inhaler with spacer, or dry powder. Chest. 1997;112(1):24–28.
- Lodha R, Gupta G, Baruah BP, et al. Metered dose inhaler with spacer versus dry powder inhaler for delivery of salbutamol in acute exacerbations of asthma: a randomized controlled trial. Indian Pediatr. 2004;41(1):15–20.