ABSTRACT
Introduction
During intraoperative neurophysiological monitoring in neurosurgery, brain electrodes are placed to record electrocorticography or to inject current for direct cortical stimulation. A low impedance electrode may improve signal quality.
Areas covered
We review here a brain electrode (WISE Cortical Strip, WCS®), where a thin polymer strip embeds platinum nanoparticles to create conductive electrode contacts. The low impedance contacts enable a high signal-to-noise ratio, allowing for better detection of small signals such as high-frequency oscillations (HFO). The softness of the WCS may hinder sliding the electrode under the dura or advancing it to deeper structures as the hippocampus but assures conformability with the cortex even in the resection cavity. We provide an extensive review on WCS including a market overview, an introduction to the device (mechanistics, cost aspects, performance standards, safety and contraindications) and an overview of the available pre- and post-approval data.
Expert opinion
The WCS improves signal detection by lower impedance and better conformability to the cortex. The higher signal-to-noise ratio improves the detection of challenging signals. The softness of the electrode may be a disadvantage in some applications and an advantage in others.
1. Introduction
Tissue resection during a neurosurgical intervention or the planning thereof requires a precise differentiation between pathological and functional tissue. More and more of the distinction is performed with modern neuroimaging. For some aspects, electrophysiological measurements remain indispensable. If an electrode is placed directly on the brain, it serves several purposes, mainly recording electrocorticography (ECoG) or injecting current for direct cortical stimulation (DCS).
During neurosurgery in the sensorimotor region, ECoG may be combined with peripheral somatosensory stimulation to detect the phase reversal in the somatosensory evoked potential (PRSEP) which localizes the central sulcus between sensory cortex and motor cortex [Citation1–3].
Conversely to ECoG recordings, an electrode may be placed directly on eloquent cortex to inject current for direct cortical stimulation (DCS) [Citation4,Citation5]. The aim may be to delineate the language areas or to elicit a direct cortical motor evoked potential (DCMEP) [Citation2,Citation6–9]. DCS carries the risk to provoke an intraoperative seizure [Citation10]. As a warning sign for an impeding a seizure, after discharges (AD) after a stimulation pulse may be recorded in ECoG [Citation11].
In patients with epilepsy, ECoG may be used to delineate epileptogenic tissue against healthy tissue. There are mainly two indications to perform ECoG recordings. First, semi-chronic ECoG can be recorded for a few weeks in the epilepsy monitoring unit [Citation12]. Second, acute ECoG can be recorded intraoperatively during resective surgery to detect spontaneous epileptiform patterns in the ongoing ECoG. The acute ECoG is also used to record cortico-cortical evoked potentials [Citation13,Citation14]. As a traditional epileptiform pattern, interictal epileptiform discharges (IED or spikes) are readily visible because of their high amplitude [Citation15,Citation16]. As a more recently proposed pattern, high-frequency oscillations (HFO) in the ECoG are used in tailoring epilepsy surgery with the aim to improve the rate of postoperative seizure freedom [Citation17,Citation18]. Among HFO, fast ripple oscillations (250–500 Hz) were tested to indicate epileptogenic tissue in ECoG [Citation19,Citation20]. However, intraoperative HFO analysis is still restrained to few centers.
From a measurement point of view, HFOs are low in amplitude, short in duration, occur rarely, and the generators of HFOs are small. They are thus difficult to detect against the background noise. Their detection requires an optimized measurement chain to obtain sufficient signal-to-noise ratio (SNR). Elements of the chain are the anesthesia settings [Citation21], a low noise amplifier [Citation22,Citation23] and a higher contact density on the electrode [Citation24,Citation25]. Importantly, higher contact density entrains a smaller contact area, increasing contact impedance and thus the thermal noise in the recording. As a remedy, low impedance contacts may reduce background noise, thereby enhancing the SNR. A low impedance electrode may therefore be desirable in particular for the detection of HFO in epilepsy surgery.
From the neurosurgeon’s point of view, an ECoG electrode should not only be easy to handle during placement but should also not slip from the optimal position. Unintentional changes in electrode position could lead to misleading measurements. However, there is a trade-off between electrode adhesion and electrode stiffness. Ideally, the electrode should be stiff enough to slide under the dura in small craniotomies and soft enough to conform to the bottom of a sulcus.
We review here a brain electrode (WISE Cortical Strip, WCS®), where a thin polymer strip embeds platinum nanoparticles to create conductive electrode contacts. The flexible strip electrode has low impedance contacts in experimental measurements [Citation26–29] and during neurosurgical interventions [Citation30,Citation31].
2. Overview of the market
There is a wide variety of electrodes, many of them first developed for animal experimentation, that have now achieved FDA clearance and/or CE mark for use in patients. An overview is given in a recent review article [Citation32]. We focus here on electrodes that are applied in intraoperative ECoG ().
Table 1. Devices that are commercially available.
2.1. Traditional ECoG electrodes
In the traditional ECoG electrode, the recording contacts are small platelets made from metal foil that are mechanically attached to a polymer strip for handling. The electrodes are commonly shaped as strips with four or more contacts embedded in a polymer base layer of thickness 800 µm. More extensive recordings use two-dimensional arrangement of contacts in a grid. The spacing between contact centers is typically 10 mm and the contact diameter ~4 mm. The metal contacts usually consist of stainless steel or platinum and have a flat surface. Suppliers with a large market share are Ad-Tech (Oak Creek, WI, U.S.A.), Dixi (Marchaux – Chaudefontaine, France), PMT (Chanhassen, MN, U.S.A.) and inomed (Emmendingen, Germany).
2.2. Recently introduced ECoG electrodes
There are several producers that have recently introduced new ECoG electrodes or are working toward introducing new electrodes. Common to many of them is the use of thin film polymer strips. The conductive contact material may be organic as in PEDOT:PSS to reduce impedance [Citation33,Citation34], a material which however has no CE mark for patient use yet. Among new ECoG electrodes with CE mark and FDA clearance are those produced by SPES (www.spesmedica.com) and by WISE (www.wiseneuro.com). A new ECoG electrode produced by Cortec has FDA clearance (www.cortec-neuro.com). An electrode that has not yet obtained a CE certificate is produced by neurosoft (neurosoft-bio.com). The thin film polymer base of these electrodes underlies their softness that assures close mechanical contact to the curvature of the brain. The softness or flexibility of an electrode is proportional to the thickness of the substrate material (). The softness, in turn, may improve also the electrical contact, i.e. reduce the electrical impedance. The integrated production of these electrodes enables, in principle, higher channel count for greater spatial coverage and higher special resolution.
3. Introduction to the device
3.1. How the device works
The device presented here is a low impedance electrode (2.7 kΩ [Citation31]). It is currently available as a 4-contact cortical strip electrode (strip thickness: 250 µm; electrode material: pure platinum; contact diameter 4 mm; inter-electrode distance 10 mm center to center, right). The fabrication process first creates a silicon substrate and then uses a beam of platinum nanoparticles to embed a thin conductive layer. The implanted platinum nanoparticles (diameter <100 nm) create nanostructure with roughness <100 nm [Citation29]. The rough surface area is larger than a flat surface by a factor ~ 70. The enlarged surface enables efficient charge exchange between the contact and the surrounding fluid, ensuring electrical charge transfer between the contact and the brain. This microscopic property of the surface results in the low impedance of the electrode contact. left shows a standard electrode (produced by Ad-Tech, strip thickness 800 µm; platinum electrode contact diameter 2.3 mm; exposed contact surface ~4 mm2; inter-contact distance 10 mm center-to-center) for comparison. For both electrodes, the inter-contact distance 10 mm and the number of four contacts amounts to a total electrode length of ~40 mm. With the Ad-Tech thicker film substrate material (800 µm), the Ad-Tech is more stiff and not all contacts show contact to the brain with their full area. The reduced area may contribute to a higher impedance of the electrode contact and the higher variability of the impedance (). With the WCS thin film substrate material (250 µm), the WCS is more flexible and fits softly to the curved brain surface.
Figure 1. ECoG electrodes in the surgical field.
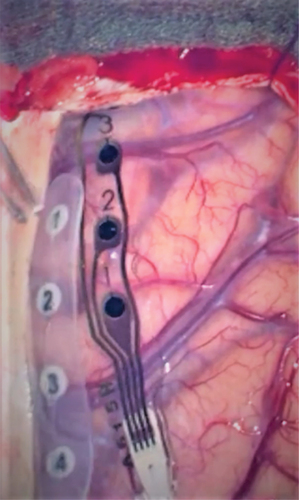
Figure 2. Impedance and noise level. (a) The median impedance was 6.9 kΩ for Ad-Tech and 3.4 kΩ for WCS measured at 140 Hz.
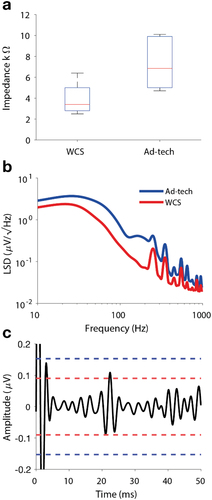
3.2. Cost effectiveness
ECoG electrodes are produced for single use. Their placement on the brain during surgery does not require specific tools. Placement is self-evident and does not require specific training of the neurosurgeon. We assume that the manufacturing cost for the WCS are higher than for the other devices, since the WCS is manufactured with a new technology and in small quantity. However, manufacturing cost contributes only little to the sales cost that may vary from country to country.
3.3. Performance standards
All ECoG electrodes must comply with the performance standards as they appear in the review publications for intraoperative neuromonitoring [Citation35] and for semi-chronic recordings [Citation12]. These comprise an acceptable impedance for neurophysiological measurements and an acceptable usability for neurosurgical placement. The WCS is intended for intraoperative use only.
In intraoperative neurophysiological recordings, the duration of recording may be critical. A low impedance of the electrode contact may reduce the noise in a recording and thereby improve the signal-to-noise ratio (SNR) of the signal of interest. () shows an example where the signal exceeds the noise floor of the WCS (red lines). This means SNR > 1 and the signal is detectable. When averaging, a lower noise floor reduces the number of averages that are needed to detect the signal with sufficient reliability. With shorter averaging duration the neurophysiologist can provide faster feedback to the neurosurgeon. This may be relevant for low amplitude signals like visual evoked potentials (VEP), cortico-cortical evoked potentials (CCEP). The surgeon can then take measures before the signal changes become irreversible.
However, in some applications, e.g. the somatosensory evoked potential (SEP), the signal amplitude of the SEP is high and a lower impedance will not further improve the SNR. For high amplitude SEP, a low impedance electrode will not provide an advantage for intraoperative recording.
The usability pertains to how the electrode can be placed, how it conforms to the brain surface, and whether it provides the desired adhesion to the brain surface.
For connection to the recording device during a neurosurgical intervention, ECoG electrodes with four recording contacts are typically equipped with 1.5 mm touch proof connectors (DIN 42,802); this holds also for the WCS electrode.
3.4. Safety and complications
The WCS has received the CE mark certification and FDA clearance after a risk analysis for design, use, and process was conducted that has mitigated all risks. Verification and validation activities have been performed during the design and development of the device and are fully documented in the documentation retained by the manufacturer and submitted and reviewed by the Notified Body for CE mark. In addition to tests related to characterization and performances, the following preclinical tests have been performed: Biocompatibility evaluation (according to ISO 10,993–1) which proves that the device is biocompatible for its intended use; Electrical safety and electromagnetic compatibility (according to the applicable parts of IEC 60,601–1, IEC 60,601-2-40, and IEC 60,601-2-26); Usability Engineering process (according to IEC 62,366).
The medical device study [Citation31] adhered to very high international standard (UNI EN ISO 14,155:2020) with standardized AE categorization and a contract research organization (CRO) so that AE were meticulously reported.
Since the WCS electrode has passed the tests for CE mark or FDA clearance, its placement on the brain carries minimum risk. It is not in itself at the cause of complications or adverse events. A typical adverse event during neurosurgery is that electrical stimulation causes an epileptic seizure [Citation10]. While the stimulation current passes through the ECoG electrode, the cause of a stimulated electric seizure lies in an inappropriate choice of stimulation parameters.
3.5. Contraindications
In some surgeries, ECoG electrode placement may require that the electrode is slid under the dura. This may be the case when the neurosurgeon creates only a small craniotomy (~40 mm diameter) to reduce the rate of adverse events and increase safety during neurosurgery [Citation36]. With this craniotomy size, ECoG electrode placement may require that the electrode is slid under the dura. The same holds for ECoG recordings in the medial temporal lobe where the electrode strip has to be advanced to hippocampus or amygdala where there is limited surgical access. Sliding the electrode to the outskirts of the surgical field is readily achieved with a traditional electrode with strip thickness ~800 µm (Section 3.1). This may be different for the WCS electrode. It was designed to be very soft for strong adhesion to the brain and for a high conformability to the brain surface, which may be an advantage for placement on widely exposed cortex in large craniotomies or placement in the bottom of a sulcus. The lack of stiffness might be disadvantageous when the electrode shall be slid horizontally on the brain in order to slide under the dura or to the hippocampus. This has been reported from neurosurgeons for surgeries where electrode placement was difficult [Citation31].
4. Clinical profile and post-marketing findings
4.1. Pre-approval
There was one pre-approval medical device study with the title ‘WIN Study’ (WIN: WISE cortical strip for Intraoperative Neurophysiological monitoring). The pre-market study was conducted 04/2019–01/2020 as a prospective, multi-center, open-label, one-arm, interventional, non-inferiority study [Citation31]. The study concluded that the impedance of the WCS electrode (median 2.7 kΩ) was significantly lower than the impedance of the Ad-Tech device (median 5.3 kΩ). This might be due to better adhesion and conformability to the brain surface and also the rough surface structure of the platinum contact surface of the WCS electrode. No limitations with respect to safety were noted. The WCS electrode was suitable for recording continuous ECoG and for recording SEP in the ECoG on the brain surface. Likewise, direct cortical stimulation of the motor cortex through the WCS electrode was suitable to elicit motor evoked potentials (MEP) in the muscles.
The impedance of the electrode did not change over time within the duration of the intraoperative measurements [Citation31].
To document the neurosurgeons perspective, questionnaires on the usability of the WCS electrode were collected. Some neurosurgeons’ comments pertained to surgeries with small craniotomies not exposing the motor cortex, where the strip electrodes had to be slid under the dura for correct positioning. In 9/32 surgeries the neurosurgeons reported difficulties when sliding the WCS under the dura because the WCS is very flexible and lacks stiffness. Thus, it was concluded at the time of the investigation that the use of the WCS might be limited when performing neurosurgery in a small surgical field.
4.2. Post-approval
A first peer-reviewed post-approval study focused on whether the lower impedance of the WCS electrode would improve the detectability of events in the HFO frequency band. The study re-analyzed a subset of the SEP data that had been recorded for the pre-approval study (Sarnthein 2022). For analysis the data were filtered in the HFO band. The SEP response is associated with and HFO at its crest [Citation37,Citation38]. This approach resulted in a recording setup where the timing of the HFO events is precisely defined [Citation30]. As a first result of this study, impedance was lower for the WCS than for the Ad-Tech also in this subset of recordings (median 6.9 kΩ for Ad-Tech and median 3.4 kΩ for WCS, ). As a second aspect, the study analyzed the noise characteristics of the continuous ECoG in the frequency domain. The linear spectral density (LSD, ) of the WCS electrode (red line) was lower than the LSD of the Ad-Tech electrode (blue line) across the whole spectral frequency range. As the main result of the study, the noise level and the SNR in the HFO band () improved from Ad-Tech (165 nV, SNR = 7.5) to WCS (90 nV, SNR = 12.8). To investigate whether the SNR improvement was actually of clinical relevance, the study quantified the rate of HFO that were detected, i.e. the number of SEP sweeps where the response in the HFO band had SNR > 1. The number of HFO detected with WCS electrode (100%) slightly exceeded the number of HFO detected with Ad-Tech electrode (91%), thus quantifying the advantage of the WCS electrode for HFO detection.
A second peer-reviewed post-approval study performed cortico-cortical evoked potentials in epilepsy surgery to identify the language tracts [Citation14]. The WCS electrode is shown in a figure where it was slid under the dura.
A series of other post-approval studies were not peer-reviewed yet because the WCS electrode has become commercially available only since 2022 [Citation39–41]. Moriconi et al. recorded spontaneous ECoG in epilepsy surgery from areas that were planned for resection including the mesial temporal lobe. They reported ‘The WCS has a better adhesion and conformability to the brain surface and also a better signal acquisition on ECoG than the traditional electrode. The higher flexibility of the WCS electrode limited its use in deeper structures (like amygdala and hippocampus) for greater handling difficulties’ [Citation41]. A single case conducted from the same clinical center has added ‘that WCS features allowed to perform high quality registration from the bottom of the sulcus, leading to surgical resection of highly epileptogenic cortex’ [Citation39]. Castelli et al. elicited MEP and recorded SEP and cortico-cortical evoked potentials in the ECoG during epilepsy surgery where they reported ‘The unique strip’ features represent a promising advancement in IOM during brain surgery, providing excellent versatility in harder condition’ and that the WCS ‘features were enhanced in particular condition, such as stimulation/recording in the context of surgical cavity, where standard and more rigid four contact strips tend to lose the adherence to the brain surface resulting in significant signal distortion’ [Citation40].
5. Alternative devices
There are several companies currently working on flexible ECoG electrodes (). Those with CE mark include SPES and WISE. The Cortec electrode has FDA clearance. Electrode development in an earlier stage include neurosoft. Common to these electrodes is their softness that assures close mechanical contact to the curvature of the brain. This, in turn may improve also the electrical contact, i.e. reduce the impedance.
6. Conclusion
Low-impedance brain electrodes for neurophysiological monitoring of the ECoG reduce noise. The improved signal-to-noise ratio improves detection of difficult signals such as those in the HFO frequency range. Improving the measurement chain may extend the diagnostic value of HFO as a biomarker for epileptogenic tissue. Placement of the soft WCS electrode remains an issue, but the above advantages of the WCS may outweigh this issue in many applications.
7. Expert opinion
Electrophysiological measurements during neurosurgical interventions are of utmost importance, not only to monitor neurological functions but also to help differentiating between pathological tissue (e.g. tumor or seizure onset zones) and healthy parenchyma. Brain electrodes are used intraoperatively mainly for recording electrocorticography (ECoG) or for injecting current for direct cortical stimulation (DCS). In daily practice, the main applications are the recording of the phase reversal in the somatosensory evoked potential (PRSEP) which localizes the central sulcus between sensory cortex and motor cortex as well as the mapping of eloquent cortical function (e.g. motor function or language areas) by injecting current for direct cortical stimulation. Furthermore, another common clinical application is the detection of epileptogenic tissue by performing ECoG for that purpose.
There are several soft thin film electrodes that have recently been introduced to the market and WCS (WISE Cortical Strip) is one of them. WCS is made of a thin polymer strip that embeds platinum nanoparticles to create conductive electrode contacts. When placed on the cortical surface in a large surgical field, soft electrodes such as WCS have advantages over traditional stiff electrodes because of their high surface adhesion and high conformability. The high conformability has also enabled recording from the bottom of the sulcus [Citation39,Citation40], in contrast to stiffer devices. However, some users have noted difficulties if the electrode has to be advanced to deep structures like the hippocampus [Citation41] or slid under the dura for small craniotomies [Citation31].
When speculating 5 years ahead, we expect several developments. For soft thin film electrodes, a mechanical placement aid may facilitate electrode placement on brain structures other than the plain cortex freely exposed in the surgical field. This is a low-tech solution that will greatly enhance the usability of soft electrodes and overcome the main challenge (lack of stiffness during placement) in clinical practice. From the measurement perspective, thin film electrodes with a dense array of recording contacts will become readily available for human use [Citation24,Citation25]. The strict regulatory requirements and the high price of the devices may slow down adoption of these devices in clinical practice.
As a challenge downstream of the electrode, dense array recording entrains the demand for amplifier systems with a large number of recording channels. The more frequent and more expanded recordings spur the demand for more reliable documentation of the recording sites with respect to the imaging obtained for surgical planning and with respect to the postsurgical imaging of the extent of the resection volume. While a very powerful interface between neurophysiological recording and anatomical imaging has been proposed [Citation42], its implementation requires highly qualified manpower with knowledge in neurophysiology, anatomy, imaging modalities, and neurosurgery. Still, we expect that this advanced documentation will spread to further neurosurgery centers together with the use of thin film ECoG electrodes. An open question for future studies is whether the improved recording qualities actually improve recording of clinically relevant patterns in the ECoG. Is the recording of high-frequency oscillations clinically relevant? Finally, we need to document whether the application of improved ECoG electrodes improves postsurgical patient outcome.
Article highlights
The ECoG electrode (WISE Cortical Strip, WCS®) is made of conductive electrode contacts where platinum nanoparticles are embedded in a thin polymer strip.
Higher flexibility and adhesiveness together with lower impedance yield improved signal-to-noise ratio in recordings.
These improvements help in detecting challenging small signals.
High-frequency oscillations are small signals that indicate epileptogenic tissue.
The softness of the WCS may hinder sliding the electrode under the dura or advancing it to deeper structures but enables recording form the bottom of a sulcus.
Declaration of interest
JS is member of the inomed scientific advisory board and has received speaker fees for giving presentations at inomed in-house courses on IONM. MCN has received a research grant from Novocure, honoraria for consulting or lectures from Osteopore, Novocure and MSD. MCN has reviewed documentation for WISE and his institution has received research funds from WISE Srl. The authors have no other relevant affiliations or financial involvement with any organization or entity with a financial interest in or financial conflict with the subject matter or materials discussed in the manuscript apart from those disclosed.
Reviewers disclosure
Peer reviewers on this manuscript have no relevant financial relationships or otherwise to disclose.
Author contributions
In the production of this manuscript, JS has contributed the neurophysiological aspects and MCN has contributed the neurosurgical aspects.
Acknowledgments
We thank V. Dimakopoulos for adapting the figures.
Additional information
Funding
References
- Gregorie EM, Goldring S. Localization of function in the excision of lesions from the sensorimotor region. J Neurosurg. 1984;61(6):1047–1054. doi: 10.3171/jns.1984.61.6.1047
- Cedzich C, Taniguchi M, Schafer S, et al. Somatosensory evoked potential phase reversal and direct motor cortex stimulation during surgery in and around the central region. Neurosurgery. 1996;38(5):962–970. doi: 10.1097/00006123-199605000-00023
- Romstöck J, Fahlbusch R, Ganslandt O, et al. Localisation of the sensorimotor cortex during surgery for brain tumours: feasibility and waveform patterns of somatosensory evoked potentials. J Neurol Neurosurg Psychiatry. 2002;72(2):221–229.
- Gordon B, Lesser RP, Rance NE, et al. Parameters for direct cortical electrical stimulation in the human: histopathologic confirmation. Electroencephalogr Clin Neurophysiol. 1990;75(5):371–377. doi: 10.1016/0013-4694(90)90082-U
- Penfield W, Boldrey E. Somatic motor and sensory representations in the cerebral cortex of man as studied by electrical stimulation. Brain. 1937;60(4):389–443. doi: 10.1093/brain/60.4.389
- Taniguchi M, Cedzich C, Schramm J. Modification of cortical stimulation for motor evoked potentials under general anesthesia: technical description. Neurosurgery. 1993;32(2):219–226.
- Seidel K, Beck J, Stieglitz L, et al. The warning-sign hierarchy between quantitative subcortical motor mapping and continuous motor evoked potential monitoring during resection of supratentorial brain tumors. JNS. 2013;118(2):287–296. doi: 10.3171/2012.10.JNS12895
- Neuloh G, Pechstein U, Schramm J. Motor tract monitoring during insular glioma surgery. J Neurosurg. 2007;106(4):582–592.
- Szelenyi A, Langer D, Beck J, et al. Transcranial and direct cortical stimulation for motor evoked potential monitoring in intracerebral aneurysm surgery. Neurophysiol Clin. 2007;37(6):391–398. doi: 10.1016/j.neucli.2007.09.006
- Szelenyi A, Joksimovic B, Seifert V. Intraoperative risk of seizures associated with transient direct cortical stimulation in patients with symptomatic epilepsy. J Clin Neurophysiol. 2007;24(1):39–43. doi: 10.1097/01.wnp.0000237073.70314.f7
- Gollwitzer S, Hopfengartner R, Rossler K, et al. Afterdischarges elicited by cortical electric stimulation in humans: when do they occur and what do they mean? Epilepsy Behav. 2018;87:173–179. doi: 10.1016/j.yebeh.2018.09.007
- Zijlmans M, Zweiphenning W, van Klink N. Changing concepts in presurgical assessment for epilepsy surgery. Nat Rev Neurol. 2019;15(10):594–606. doi: 10.1038/s41582-019-0224-y
- Prime D, Rowlands D, O’Keefe S, et al. Considerations in performing and analyzing the responses of cortico-cortical evoked potentials in stereo-EEG. Epilepsia. 2018;59(1):16–26. doi: 10.1111/epi.13939
- Giampiccolo D, Parmigiani S, Basaldella F, et al. Recording cortico-cortical evoked potentials of the human arcuate fasciculus under general anaesthesia. Clin Neurophysiol. 2021;132(8):1966–1973. doi: 10.1016/j.clinph.2021.03.044
- Alarcon G, Garcia Seoane JJ, Binnie CD, et al. Origin and propagation of interictal discharges in the acute electrocorticogram. Implications for pathophysiology and surgical treatment of temporal lobe epilepsy. Brain. 1997;120(Pt 12):2259–2282. doi: 10.1093/brain/120.12.2259
- Yu HY, Lin CF, Chou CC, et al. Outcomes of hippocampus-sparing lesionectomy for temporal lobe epilepsy and the significance of intraoperative hippocampography. Clin Neurophysiol. 2021;132(3):746–755. doi: 10.1016/j.clinph.2020.12.008
- Zweiphenning W, van’t Klooster MA, van Klink NEC, et al. Intraoperative electrocorticography using high-frequency oscillations or spikes to tailor epilepsy surgery in the Netherlands (the HFO trial): a randomised, single-blind, adaptive non-inferiority trial. Lancet Neurol. 2022;21(11):982–993.
- Chen Z, Maturana MI, Burkitt AN, et al. High-frequency oscillations in epilepsy: what have we learned and what needs to be addressed. Neurology. 2021;96(9):439–448. doi: 10.1212/WNL.0000000000011465
- van T, Klooster MA, van Klink NEC, et al. Tailoring epilepsy surgery with fast ripples in the intraoperative electrocorticogram. Ann Neurol. 2017;81(5):664–676. doi: 10.1002/ana.24928
- Fedele T, van ‘, Klooster M, et al. Automatic detection of high frequency oscillations during epilepsy surgery predicts seizure outcome. Clin Neurophysiol. 2016;127(9):3066–3074. doi: 10.1016/j.clinph.2016.06.009
- Firestone E, Sonoda M, Kuroda N, et al. Sevoflurane-induced high-frequency oscillations, effective connectivity and intraoperative classification of epileptic brain areas. Clin Neurophysiol. 2023;150:17–30. doi: 10.1016/j.clinph.2023.03.004
- Fedele T, Schonenberger C, Curio G, et al. Intraoperative subdural low-noise EEG recording of the high frequency oscillation in the somatosensory evoked potential. Clin Neurophysiol. 2017;128(10):1851–1857. doi: 10.1016/j.clinph.2017.07.400
- Fedele T, Ramantani G, Burnos S, et al. Prediction of seizure outcome improved by fast ripples detected in low-noise intraoperative corticogram. Clin Neurophysiol. 2017;128(7):1220–1226. doi: 10.1016/j.clinph.2017.03.038
- Boran E, Ramantani G, Krayenbühl N, et al. High-density ECoG improves the detection of high frequency oscillations that predict seizure outcome. Clin Neurophysiol. 2019;130(10):1882–1888. doi: 10.1016/j.clinph.2019.07.008
- Zweiphenning WJEM, van Diessen E, Aarnoutse EJ, et al. The resolution revolution: comparing spikes and high frequency oscillations in high-density and standard intra-operative electrocorticography of the same patient. Clin Neurophysiol. 2020;131(5):1040–1043. doi: 10.1016/j.clinph.2020.02.006
- Corbelli G, Ghisleri C, Marelli M, et al. Highly deformable nanostructured elastomeric electrodes with improving conductivity upon cyclical stretching. Adv Mater. 2011;23(39):4504±. doi: 10.1002/adma.201102463
- Marelli M, Divitini G, Collini C, et al. Flexible and biocompatible microelectrode arrays fabricated by supersonic cluster beam deposition on SU-8. J Micromech Microeng. 2011;21(4):045013. doi: 10.1088/0960-1317/21/4/045013
- Ravagnan L, Divitini G, Rebasti S, et al. Poly(methyl methacrylate)-palladium clusters nanocomposite formation by supersonic cluster beam deposition: a method for microstructured metallization of polymer surfaces. J Phys D Appl Phys. 2009;42(8):75.
- Gnatkovsky V, Cattalini A, Antonini A, et al. Recording electrical brain activity with novel stretchable electrodes based on supersonic cluster beam implantation nanotechnology on conformable polymers. Int j nanomed. 2019;14:10079–10089.
- Dimakopoulos V, Neidert MC, Sarnthein J. Low impedance electrodes improve detection of high frequency oscillations in the intracranial EEG. Clin Neurophysiol. 2023;153:133–140.
- Sarnthein J, Seidel K, Neidert MC, et al. Evaluation of a new cortical strip electrode for intraoperative somatosensory monitoring during perirolandic brain surgery. Clin Neurophysiol. 2022;142:44–51.
- Paulk AC, Salami P, Zelmann R, et al. Electrode development for epilepsy diagnosis and treatment. Neurosurg Clin N Am. 2023;35(1):135–149. doi: 10.1016/j.nec.2023.09.003
- Khodagholy D, Gelinas JN, Thesen T, et al. NeuroGrid: recording action potentials from the surface of the brain. Nat neurosci. 2015;18(2):310–315. doi: 10.1038/nn.3905
- Castagnola E, Maiolo L, Maggiolini E, et al. PEDOT-CNT-Coated low-impedance, ultra-flexible, and brain-conformable micro-ECoG arrays. IEEE Trans Neural Syst Rehabil Eng. 2015;23(3):342–350. doi: 10.1109/TNSRE.2014.2342880
- Stecker MM, Arle JE. Surgery for epilepsy. In: Deletis V, editor. Neurophysiology in neurosurgery. Deletis. Elsevier; 2020. p. 443–456.
- Yaşargil M. Microneurosurgery. Stuttgart: Thieme; 1984.
- Burnos S, Fedele T, Schmid O, et al. Detectability of the somatosensory evoked high frequency oscillation (HFO) co-recorded by scalp EEG and ECoG under propofol. NeuroImage Clin. 2016;10:318–325. doi: 10.1016/j.nicl.2015.11.018
- Baker SN, Gabriel C, Lemon RN. EEG oscillations at 600 hz are macroscopic markers for cortical spike bursts. J Physiol. 2003;550(2):529–534. doi: 10.1113/jphysiol.2003.045674
- Biagioli N, Morandi S, Moriconi E, et al. Role of new generation WISE cortical strip (WCS) in bottom of the sulcus focal cortical dysplasia (BOSD): a case report. In: Congresso Nazionale Società Italiana di Neurofisiologia Clinica. Bergamo; 2023.
- Castelli N, Cojazzi V, Innocenti N, et al. Adherent four contact strip for IOM in epilepsy surgery. In: European Association of neurosurgery societies. Barcelona; 2023.
- Moriconi E, Tramontano V, Morandi AE, et al. Wise cortical strip for tailored epilepsy surgery. In: European Association of neurosurgery societies. Barcelona; 2023.
- Demuru M, van Blooijs D, Zweiphenning W, et al. A practical workflow for organizing clinical intraoperative and long-term iEEG data in BIDS. Neuroinformatics. 2022;20(3):727–736. doi: 10.1007/s12021-022-09567-6