Abstract
Drug delivery systems (DDSs) have been developed to carry an appropriate payload into the tumour’s area, accomplishing the goals of minimising potential bodily harm and enhancing therapeutic efficacy. Here, we fabricated a one-pot method for encasing docetaxel (DTX) in zeolitic imidazolate framework-8 (ZIF-8) composites called DTX@ZIF-8. The DTX@ZIF-8/HA nanoplatform is then formed by coating the DTX@HA with a hyaluronic acid (HA). Notably, the loading rate increased to 43.0% at a DTX dose of 1 mg/mL. The findings confirmed that HA could prolong blood flow and improve the tumour-specific formation of DDS. It may also be an innovative ‘switch’ and tumour-targeted ‘guider’. Hyaluronidase (HAase) in the tumour microenvironment (TME) may break down the HA shell, exposing the wrapped DTX@ZIF-8 and causing ZIF-8 to break down in the acidic tumour microenvironment, releasing the loaded DTX. The apoptosis mechanism was investigated using various biochemical staining and flow cytometric analysis. As a result, the DTX@ZIF-8/HA nanoplatforms were developed as an on-demand, tumour-specific drug delivery system, increasing the effectiveness of treatment.
1. Introduction
Cancer is a category of disorders characterised by the uncontrolled development of aberrant cells into tumours, which may either stay put in their original site of creation (termed primary cancer) or spread to neighbouring organs and produce new tumours (termed metastatic cancer) [Citation1]. Different cell genes and proteins are turned up or down throughout this process. These include genes and proteins that control cell growth and death, cell adhesion and motility, tumour suppression, and many more [Citation2–4]. General causes have been recognised, such as environmental and genetic factors, hormones, food, or even infectious agents, although specific causes vary greatly depending on the kind of cancer. In 2020, cancer is expected to kill nearly 10 million people worldwide, making it the third most significant cause of death [Citation5]. It is projected that the number of new cases of cancer will rise to 30 million by 2040 from the estimated 19.3 million in 2020. Indeed, cancer remains a significant societal burden despite significant progress in the development of new technology for detection and treatment. Leukaemia is a malignancy caused by unchecked cell division in the lymphatic system and bone marrow, two organs responsible for producing blood cells [Citation6–8]. The normal function of white blood cells, which combat infections, is often disrupted by leukaemia. In a healthy body, these cells multiply and increase by what’s required, but the bone marrow produces abnormally large numbers of white blood cells that are defective in function in cases of leukaemia. Functionalised NPs as biosensors, CRISPR/Cas9 systems, DNA conjugation, ultra-resolution imaging equipment, etc., can help with the sensitive and accurate diagnostics needed to effectively treat leukaemia [Citation9–11].
Nanocarriers, including liposomes, polymers, and inorganic nanomaterials, have emerged as a versatile method for delivering drugs to specific tissues, such as tumours [Citation12]. The properties of a promising drug delivery system (DDS) are (1) the absence of leakage into the bloodstream, (2) enhanced accumulation in tumour tissues, and (3) an optimal location for drug release in response to release triggers [Citation13–15]. The increased permeability and retention (EPR) effect makes it simple for nanoscale DDSs to be taken up by cancer cells, and they also prevent the drugs from leaking out into circulation [Citation16]. The excellent biocompatibility of chitosan-based nanomedicines has led to their widespread use in drug delivery. Self-assembled nanoparticles made from amphiphilic chitosan derivatives are attractive drug carriers because their hydrophobic regions can store insoluble pharmaceuticals. Carboxymethyl chitosan (CMCS) doped with ionic liquids can also provide sufficient adsorption groups (–OH, –COOH, and –NH–) to boost adsorption capacity [Citation17]. Using amino protonation, CMCS nanoparticles are pH-sensitive. Furthermore, cancer cells have significant reactive oxygen species (ROS) levels, which can oxidise benzylboronic acid/ester [Citation18].
Metal-organic frameworks (MOFs) include the zeolite imidazole framework (ZIF). Due to its high specific surface area and thermal stability, ZIF-8, mostly of zinc ions and 2-methylimidazole, has been the subject of much research. Crystals of ZIF-8, a cage-like coordination complex, take the shape of rhombic dodecahedrons [Citation19]. ZIF-8-based nanoplatforms have shown promise in biomedical imaging techniques like MRI, CT, photoacoustic imaging, immunotherapy, starvation therapy (ST), phototherapy, chemotherapy, and gene therapy (GT) for cancer treatment [Citation20]. Using ZIF-8 in a nanoreactor can prevent drug leakage and provide a self-sacrificial model. ZIF-8, on the other hand, tends to polymerise and has a low solubility in water. Hyaluronic acid (HA) and polyethylene glycol (PEG) are commonly used to alter the surface of ZIF-8. Common ligands were folic acid (FA), HA, and lactobionic acid (LA), and targeting ligand alteration on the ZIF-8 surface facilitated active delivery to tumour cells [Citation21]. For example, coating the cancer cell or erythrocyte membrane with a bionic mineralised nanodrug surface can achieve long-term blood circulation and immune evasion. However, ZIF-8's features, such as the mechanisms behind its effects on tumour development and the long-term toxicity in vivo, have yet to receive enough attention in the available investigations [Citation22].
The chemotherapeutic drug docetaxel (DTX) is used alone and in combination to treat a wide range of malignancies [Citation14]. Recurrent ovarian cancer, gastric cancer, head and neck, squamous cell carcinoma, breast cancer, and non-small cell lung cancer are only some of the cancers that have responded well to DTX's antitumor effects. Large volumes of distribution, binding to plasma proteins, and cytochrome CYP3A4 metabolism characterise DTX after intravenous injection [Citation23]. Inhibiting proper cell division, DTX is an antimicrotubular agent that primarily exerts a cytotoxic effect by disrupting the microtubule network in cells (it supports and stabilises microtubule formation and prevents microtubule depolymerization). The toxicity and systemic side effects of DTX constitute a significant issue that prevents the drug from being used effectively in people. Up to 80% of individuals receiving the recommended starting dosage of 100 mg/m2 have neutropenia as an adverse effect [Citation24]. Hypotension, bronchospasm, a broad rash/erythema, skin toxicity, the danger of a severe infection, fluid retention, hypoalbuminemia, and cardiac, renal, or hepatic dysfunction are all symptoms of hypersensitivity reactions (HSRs). Neurotoxicity (40% incidence of motor and sensory neuropathies), asthenia (60 to 70%), stomatitis, and diarrhoea have all been linked to DTX [Citation25]. Despite premedication with corticosteroids (prednisolone 50 mg, betamethasone 8 mg, or dexamethasone 8 mg) and the prophylactic use of granulocyte colony-stimulating factors (G-CSF), the DTX dose must often be reduced to 30–75 mg/m2 (in 5–25%) due to toxicity during treatment [Citation26].
Surface conjugation with highly biocompatible organic materials is commonly used to increase blood circulation and tumour cell accumulation of different DDSs; typical nanomaterials include red cell membrane, PEG-derivatives, poly(ethylene glycol) (PEG), etc. Hyaluronic acid (HA) is a negatively charged natural polysaccharide found in high concentrations in the extracellular matrix and has the additional benefits of being biocompatible, nontoxic, and biodegradable. Furthermore, hyaluronidase (HAase), abundant in the tumour microenvironment (TME), can break down HA chains, exposing the encased DDS and allowing for various cancer therapies. Encapsulating DTX in ZIF-8 compounds in a single step during the ZIF-8 synthesis process (DTX@ZIF-8) and then coating it with an HA shell. The apoptosis mechanism was investigated using various biochemical staining and flow cytometric analysis.
2. Experimental section
2.1. Chemicals and characterizations
Docetaxel (DTX) was obtained from Xin Ding Pengfei Technology Development Co., Ltd (Beijing, China). Zinc chloride, dimethyl sulfoxide (DMSO), and phosphate buffer saline (PBS) were purchased from Sigma-Aldrich Co. (St Louis, MO, USA). 2-Methylimidazole was purchased from Ruixi Biological Technology Co., Ltd (Xian, China). Dulbecco’s modified Eagle medium (DMEM), foetal bovine serum (FBS), 0.25% trypsin-EDTA, and 1% penicillin-streptomycin were purchased from Gibco Co., Ltd. (Carlsbad, CA, USA). The AO/EtBr kit (Rhodamine-123), 4′,6-diamidino-2-phenylindole (DAPI), was purchased from Beyotime (Shanghai, China) and used according to the protocols provided by the manufacturers. MTT was purchased from Dalian Meilun Biotechnology Corporation (Dalian, China).
Scanning electron microscopy (SEM) (ZEISS SIGMA and Zeiss Merlin compact) was implemented to examine nanoparticle surface morphology and dispersion. The morphology of the nanoparticles was verified with TEM (JEM-1400plus, Japan). Hydrodynamic sizes and zeta potentials were measured on a Zetasizer instrument (Nano ZS, Malvern). A BRUKER D8-discover XRD system characterised by powder X-ray diffraction (XRD). Thermal gravimetric analysis (TGA) was accomplished in the thermogravimetric Setaram analyzer. The nanoparticles’ Fourier transform infrared spectroscopy (FTIR) spectra were recorded using an FTIR spectrophotometer (Bruker, Germany) in the scan range of 400–4000 cm−1. Ultraviolet–visible (UV–vis) absorption spectra were obtained on a UV-2600 spectrometer (Shimadzu Ltd., Japan).
2.2. Fabrication of DTX@ZIF-8
A simple yet adaptable procedure was employed to develop DTX@ZIF-8 with high reproducibility, consistent with previous literature [Citation27]. To be more specific, 7.5 g of 2-methylimidazole (2-Mim), an organic linker, was immersed in 200 mL of MeOH solvent with vigorous stirring at RT to develop a colourless, odourless, tasteless, and odourless homogenous solution. A mixture of Zn(NO3)26H2O (2.9 g) and DTX (0.30 g) immersed in 100 mL of MeOH was ultrasonically processed for about 5 min before being added drop by drop to the 2-methylimidazole solution while being stirred continually for 20 min. The resultant DTX@ZIF-8 was rinsed three times with MeOH and water, after which the UV–vis spectrum of the supernatant solution was used to calculate the DTX loading capacity.
2.3. Fabrication of DTX@ZIF-8/HA
The steps taken to acquire HA-functionalized DTX@ZIF-8 (DTX@ZIF-8/HA) are as follows: Ultrasonication was used to dissolve 50 mL of deionized (DI) water into a moderate quantity of HA. Drop by drop, DTX@ZIF-8 developed during the preceding stage was added to the HA solution while stirring vigorously at room temperature for 48 h. The resulting product was then centrifuged for collection.
2.4. DTX controlled release from DTX@ZIF-8
For exploring the cumulative release rates of DTX in NPs, the same volume of NPs solutions (100 μg/mL DTX) was immersed in two pH values (pH 7.4 and pH 5.0) at indicated time points (200 μL release samples were collected, and the release medium was supplemented with the same volume. Then, the UV–vis spectrophotometer was detected under the Varioskan Flash Reader (Thermofisher, USA) [Citation28].
2.5. In vitro cytotoxicity
K562 cells and Human umbilical vein endothelial cells (HUVECs) cells were respectively cultured in Dulbecco’s Modified Eagle’s Medium supplemented with 100 U/mL penicillin, 100 μg/mL streptomycin, 10% foetal bovine serum (FBS) at 37 °C with 5% CO2.
HUVE cells were plated in 96-well plates (NEST Biotechnology) at a density of 1 × 104 cells per well for 24 h. After incubating with DTX@ZIF-8/HA for 24 h, then washed with PBS and cultured for another 24 h. The cell culture medium was further replaced with fresh medium containing 20 μL 5 mg mL−1 3-(4,5-dimethylthiazol-2-yl)-2,5-diphenyltetrazolium bromide (MTT) solution for another 4 h. Finally, absorbance at 490 nm was recorded on a Huadong, DG-5031, NJ microplate reader. Cell viability (%) = (Asample − Acontrol)/(Asample − Acontrol) × 100% [Citation29–32].
Cell cytotoxicity analysis was conducted on K562 cells. Cells were seeded in 96-well plates (5000 cells per well) and incubated overnight. Free DTX, ZIF-8/HA, DTX@ZIF-8, and DTX@ZIF-8/HA solutions suspended in PBS at different concentrations were added to the cell culture medium. After incubation for another 24 h, the cells were treated with freshly prepared working solution (180 μL cell culture medium plus 20 μL 5 mg/mL of MTT solution). After incubation for another 4 h, the supernatants were removed, and 150 μL of DMSO solution was added to each well. A microplate reader (Huadong, DG-5031, NJ) was used to read the optical density at 490 nm. Cell viability was determined as the ratio of the OD values of cells treated with NPs to those treated with PBS. The cell cytotoxicity analysis of NPs followed the same protocol.
2.6. Morphological, nuclear changes, and apoptosis investigations
K562 cells (2 × 105 cells in DMEM medium each well) were planted in a 6-well plate. After 24 h incubation, the culture medium was changed, and fresh culture medium having pure DTX, ZIF-8/HA, DTX@ZIF-8, and DTX@ZIF-8/HA was added (IC50 concentration of medium per well). After 24 h, the cells were collected and stained by an AO/EtBr staining [Citation33–36]. Finally, the fluorescence was observed by a fluorescence microscope (Olympus CKX53).
K562 cells (2 × 105 cells in DMEM medium each well) were planted in a 6-well plate. After 24 h incubation, the culture medium was changed, and fresh culture medium having pure DTX, ZIF-8/HA, DTX@ZIF-8, and DTX@ZIF-8/HA was added (IC50 concentration of medium per well). After 24 h, the cells were collected and stained by a DAPI staining) [Citation37–40]. Finally, the fluorescence was observed by a fluorescence microscope (Olympus CKX53).
K562 cells (2 × 105 cells in DMEM medium each well) were planted in a 6-well plate. After 24 h incubation, the culture medium was changed, and fresh culture medium having pure DTX, ZIF-8/HA, DTX@ZIF-8, and DTX@ZIF-8/HA was added (IC50 concentration of medium per well). After 24 h, the cells were collected, stained by an Annexin V-FITC/PI Staining Assay Kit (4 A Biotech Co. Ltd., Beijing, China) [Citation41], and investigated by flow cytometry (Dakewe EXFLOW-206, Shenzhen, China).
3. Results and discussion
3.1. Fabrication and characterisation of NPs
It depicts a straightforward synthetic technique for the DTX@ZIF-8/HA nanoplatforms and its potential use in cancer therapy. This example used a one-step self-assembly technique to fabricate DTX@ZIF-8 nanoparticles (NPs). To get the final DTX@ZIF-8/HA, a HA shell sensitive to HAase was applied onto the surface of DTX@ZIF-8. Since HA had several carboxyl groups, many of which were negatively charged, they interacted strongly with Zn2+ ions, allowing HA to increase and fabricate the HA/@ZIF-8 or DTX@ZIF-8/HA complexes. A complex cascade of chemical reactions in cancer cells led to the gradual release of encapsulated DTX from the ZIF-8 nanocarrier. To combine the advantages of DTX, ZIF-8, and HA in leukaemia cancer therapy and to illuminate the effect of the HA coating, DTX@ZIF-8/HA were fabricated using a technique based on the pH-responsive solubility of DTX, and then HA was self-assembled on the particle surfaces via electrostatic interactions between carboxyl groups and Zn ions to obtain DTX@ZIF-8/HA based on previous reports [Citation42–44]. ZIF-8 NPs easily decompose under acidic conditions, so they are suitable for constructing pH-responsive drug delivery systems for the drug release of drug delivery applications [Citation45–47].
Particle sizes of the evenly dispersed DTX@ZIF-8 were 40–60 nm, as shown in transmission electron microscopy (TEM) images (). A pale shell developed on the DTX@ZIF-8 nanoparticles’ outer surface after being encircled by HA through the mixing method; the resulting material was dubbed DTX@ZIF-8/HA (). The as-obtained DTX@ZIF-8/HA was more dispersed and had a more consistent size than DTX@ZIF-8.
Figure 1. Morphological characterisation of DTX@ZIF-8 and DTX@ZIF-8/HA. TEM images DTX@ZIF-8 and DTX@ZIF-8/HA. Scale bar 20 nm.
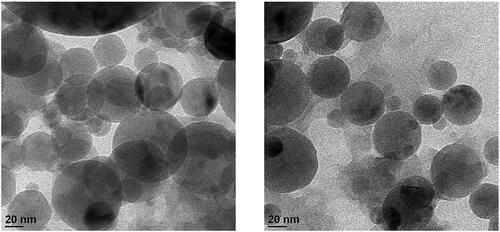
ZIF-8, DTX@ZIF-8, HA, and DTX@ZIF-8/HA crystal structures were further characterised by powder X-ray diffraction (XRD) analysis. shows that the pattern of the distinctive peaks based on the known ZIF-8 structure correlates well with the diffractions. DTX@ZIF-8 and ZIF-8 peaks coincided at their highest points. However, when comparing the diffraction peak of DTX@ZIF-8 to the ZIF-8 reference (2θ = 7.28°, 12.65°, and 17.97°), a minor divergence was shown toward lower values at 2θ = 7.21°, 12.63°, and 17.96°. As predicted by Bragg’s Law, this resulted in a growth of available space within the crystal. DTX molecules within the nanocarrier’s micropores may account for the discrepancy. Not only was no peak associated with DTX detected, but this also suggested that DTX molecules were sequestered within ZIF-8. However, once HA was applied as a coating, the diffraction profile of DTX@ZIF-8/HA was slightly obscured, which might be taken as evidence for the effective shield of the HA. Next, we used FT-IR spectroscopy, as shown in , to qualitatively ascertain the alteration processes of the nanoplatform. Pure DTX and HA FT-IR spectra were submitted for evaluation. The vibrational spectra of the as-obtained DTX@ZIF-8 were shown to be like those of ZIF-8, excluding faint features at 1765 cm−1 and 1725 cm−1 from DTX (), suggesting the presence of DTX molecules in the DTX@ZIF-8 materials. When assessing the FT-IR spectral of DTX@ZIF-8/HA to those of DTX@ZIF-8 and HA, the band at 1560 cm−1 of DTX@ZIF-8/HA has broadened, perhaps because of the presence of the amide bonds of the HA. C–H and –OH stretching are possible for the 2880 and 3435 cm−1 peaks. The example signals proved that DTX was successfully incorporated into ZIF-8, and HA was successfully modified onto the surface of DTX@ZIF-8.
Figure 2. A) XRD patterns of the simulated ZIF-8, pure HA, as-fabricated ZIF-8, DTX@ZIF-8, and DTX@ZIF-8/HA. B) FT-IR spectra of as-prepared ZIF-8, DTX, DTX@ZIF-8, HA, and DTX@ZIF-8/HA.
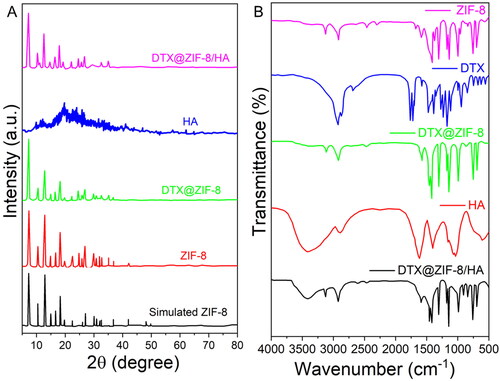
Moreover, the HA coating on DTX@ZIF-8 was ascribed to a difference in the mean size of the samples (), with the unmodified sample having a diameter of around 143 nm and the DTX@ZIF-8/HA sample having a diameter of roughly 165 nm. In addition, the size distribution of DTX@ZIF-8/HA was narrower than that of DTX@ZIF-8, with a PDI value as low as 0.016 (). The outcomes showed that the DTX@ZIF-8/HA had a homogenous structure of the necessary diameter and negatively charged surface area, giving the DTX@ZIF-8/HA nanoplatforms a good foundation for further in vitro biological studies. shows that zeta potential measurement in water was used to identify the developed components at various synthesis phases. Zeta potential measurements showed that DTX was indeed encapsulated ZIF-8, with a drop from the pure ZIF-8 value of +21.9 mV to +19.8 mV for DTX@ZIF-8. After HA-functionalization, the zeta potential shifted significantly from +19.8 mV (DTX@ZIF-8) to −16.5 mV (DTX@ZIF-8/HA). The experiment proved that the zeta potential of DTX@ZIF-8 shifted from the positively charged ZIF-8 to the negatively charged HA coating.
Figure 3. Size distributions measured by DLS of DTX@ZIF-8 and DTX@ZIF-8/HA. DTX@ZIF-8 and DTX@ZIF-8/HA dispersed in pH 7.4 PBS within 12 h and the varies of hydrodynamic diameters of DTX@ZIF-8/HA within 7 days.
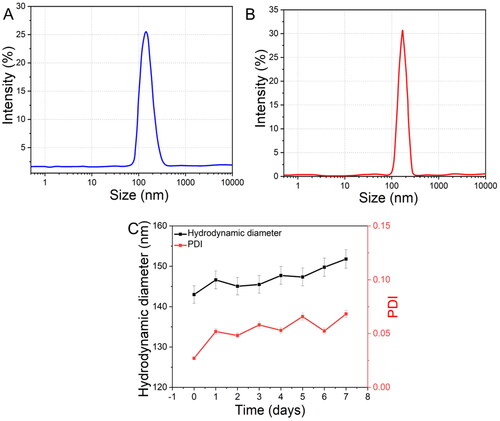
shows that the stability and dispersity of the developed DTX@ZIF-8/HA nanocomposites were assessed under physiological circumstances. In a phosphate buffer saline (PBS) solution (pH 7.4), it was discovered that DTX@ZIF-8 lacked HA coating, resulting in poor stability and dispersions and a rapid onset of settlement after 10 min. On the other hand, DTX@ZIF-8/HA maintained stability for almost 24 h under the same conditions. Within a week of being suspended in water, the DTX@ZIF-8/HA NPs’ size distributions were analysed using DLS. Hydrodynamic size and polydispersity index variations of the examined DTX@ZIF-8/HA were negligible. Furthermore, in Dulbecco’s modified eagle medium (DMEM) and phosphate-buffered saline (PBS) at 37 °C for 48 h, DTX@ZIF-8/HA remained relatively stable, demonstrating that the physiological circumstances did not affect it. The nanoplatform would, therefore, be able to circulate in vivo for an adequate amount of time, leading to a beneficial therapeutic impact.
In addition, the UV–vis absorbance spectra of ZIF-8, DTX, DTX@ZIF-8, and DTX@ZIF-8/HA NPs were tracked in real-time, as shown in . The UV–vis spectral analysis showed that the products developed the critical peak of DTX at around 281 nm as the synthesis progressed. Still, this peak was progressively attenuated in the DTX@ZIF-8 and DTX@ZIF-8/HA. This finding demonstrated that DTX could be successfully encapsulated within ZIF-8, and DTX@ZIF-8/HA compounds could be synthesised stepwise.
Figure 4. A) UV–vis absorption spectra of ZIF-8, DTX, DTX@ZIF-8 and DTX@ZIF-8/HA. B) N2 absorption-desorption isotherms of ZIF-8 and DTX@ZIF-8. C) A) ZIF-8/HA, DTX, DTX@ZIF-8, and DTX@ZIF-8/HA were dispersed in PBS (pH = 7.4) at different concentrations and did not affect the MTT reading.
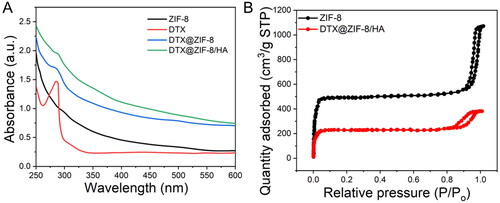
The porosity of the DTX@ZIF-8 nanostructures was determined using the Brunauer-Emmett-Teller (BET) method. Pure ZIF-8 served as a comparator and was tested in the same way. One of the hallmarks of micro-porous materials is an N2 absorption-desorption isotherm, and both materials displayed this isotherm, as shown in . ZIF-8 had a BET surface area of 1485 m2/g, while DTX@ZIF-8 reduced it to 703 m2/g. Comparing the pore volumes of pure ZIF-8 nanostructures (0.25%) and DTX@ZIF-8 (0.88%), we find that the former is much larger. The drastic decrease in BET surface area and pore volume proved that several DTX molecules were housed within DTX@ZIF-8 NPs.
3.2. DTX loading efficiency and release
We performed experiments and adjusted the loading efficacy and release actions of DTX from ZIF-8. Using a UV–vis spectrophotometer, the calibration curve for DTX was displayed in . As shown in , when the concentration of DTX rises, the loading efficiency first improves, before gradually declining. The estimated loading rate of DTX was 43.0 wt% at a concentration of 1 mg/mL. The absorption spectra of DTX before and after the DTX@ZIF-8 synthesis were also shown in . It was demonstrated that a portion of the DTX molecules had been lodged inside the ZIF-8 crystals, as shown by a weakening of the characteristic absorption peak (about 282 nm) of DTX@ZIF-8 linked to pure DTX solution. The controlled drug-release test was performed to observe the behaviour of as-prepared DTX@ZIF-8 NPs regarding DTX release (). After 25 h in a PBS buffer, the encapsulated DTX showed no sign of leaking out, demonstrating that the as-prepared DTX@ZIF-8 nanocomposites were stable under the body’s natural conditions. ZIF-8's coordination connections between its metallic and organic active sites might be disrupted in an acidic environment, releasing as much as 74.0% DTX in 25 h. Based on these findings, ZIF-8 is a promising candidate for loading hydrophobic DTX and facilitating pH-triggered drug release.
Figure 5. A) The standard curve for DTX solutions was identified at 282 nm by UV–vis. B) Difference in the loading efficacy of DTX@ZIF-8 versus concentrations of DTX. C) The absorbance spectral analysis of the initial DTX solution and the supernatant achieved after the DTX@ZIF-8. D) DTX release profiles from DTX@ZIF-8 in PBS at pH = 5.0 and 7.4.
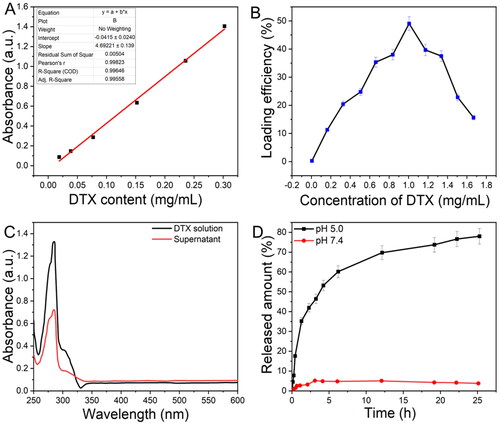
3.3. Biocompatibility and cytotoxicity
Human leukaemia cancer cells (K562 cells) and human umbilical vein endothelial cells (HUVECs) were subjected to a 3-(4,5-dimethylthiazol-2-yl)-2,5-diphenyl-tetrazolium bromide (MTT) experiment, the results of which are shown in . Since unmodified ZIF-8 is highly unstable and poisonous, we prefabricated the ZIF-8/HA variant to address these issues [Citation16]. We initially examined the UV–vis spectral analysis of ZIF-8/HA, DTX, DTX@ZIF-8, and DTX@ZIF-8/HA NPs at different concentrations in PBS () to exclude material-specific influences on the MTT assay outcome. The MTT reading was found to be unchanged.
Figure 6. A) In vitro cytotoxicity of K562 cells incubated with samples at different concentrations (0–120 μg/mL of ZIF-8 or 0–90.6 μg/mL of DTX) for 24 h. B) Cell viabilities of HUVECs with incubation of DTX@ZIF-8/HA for 24 h. C) ZIF-8/HA, DTX, DTX@ZIF-8, and DTX@ZIF-8/HA were dispersed in PBS (pH = 7.4) at different concentrations and did not affect the MTT reading.
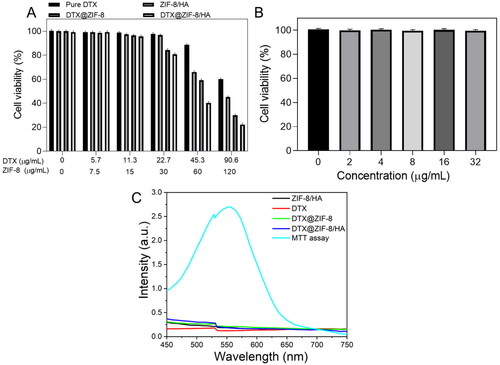
Viabilities of K562 cells revealed a concentration-dependent pattern, and pure DTX, ZIF-8/HA, DTX@ZIF-8, and DTX@ZIF-8/HA NPs all displayed more cytotoxicity to cancerous cells than the control group, as experimental concentration increased. When the HA on the surface was broken down, as shown in , over 59% of the cells treated with ZIF-8/HA NPs at the highest concentration were killed after 24 h of incubation. This indicated that the exposed ZIF-8 had a specific toxicity for K562 cells. Meanwhile, in the DTX@ZIF-8 subjected group at the maximum concentrations, cell viability was further reduced to 25%, likely due to the synergistic cytotoxicity of DTX and ZIF-8. Furthermore, when the concentrations of ZIF-8 in DTX@ZIF-8/HA were as high as 120 µg/mL, the NPs significantly reduced the survival of cancer cells to just 19.3%. Due to the larger DTX loading space of ZIF-8 and the HA on the K562 cells, the final nanomaterial displayed better cancerous cell inhibitory efficacy than the other NPs. However, as shown in , HUVECs treated with DTX@ZIF-8/HA had less cytotoxicity than K562 cells, suggesting the cell-killing effects of DTX@ZIF-8/HA while preventing cytotoxicity to non-cancerous cells.
3.4. Morphological changes
This study investigated whether apoptosis or necrosis is the mechanism of free DTX, ZIF-8/HA, DTX@ZIF-8, and DTX@ZIF-8/HA-mediated cell death using the AO/EtBr staining test in conjunction with the MTT assay [Citation38]. A nuclear staining technique, which includes detecting morphological characteristics such as nucleus disintegration, fragmented chromatin, nuclear envelope destruction, and cell blebbing, can be used to quantify the occurrence of apoptosis [Citation48–50]. We stained the nuclei using AO and EtBr to observe the apoptotic cell population following treatment with IC50 concentration of free DTX, ZIF-8/HA, DTX@ZIF-8, and DTX@ZIF-8/HA. Our findings verified that in K562 cells, the fabricated NPs caused maximal chromatin condensation and cell shrinkage. DTX@ZIF-8/HA treated cells increased nuclear fragmentation and cytoplasmic shrinkage in the K562 cell line. According to our research, nuclear fragmentation signals early death, while green fluorescence and homogeneous chromatin with an unbroken cell membrane are signs of living cells (). Late-apoptotic cells showed constricted or fragmented chromatin and reddish-coloured fluorescence in their nuclei. The study results showed that the fabricated NPs mainly caused apoptosis to cause cell death, highlighting DTX@ZIF-8/HA’s great potential as an efficient anticancer drug.
Figure 7. Dual acridine orange/ethidium bromide (AO/EB) staining shows the induction of apoptosis in the K562 cells after being treated with the IC50 concentration of DTX, ZIF-8/HA, DTX@ZIF-8 and DTX@ZIF-8/HA. Scale bar 100 µm.
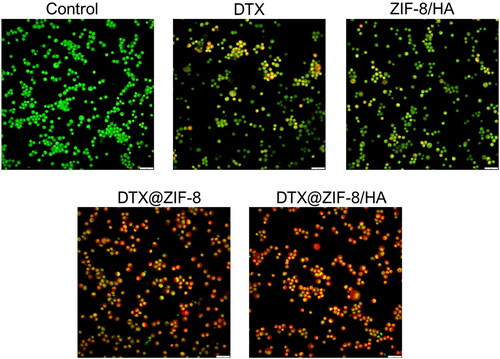
The study’s findings also showed that, compared to IC50 concentration of free DTX, ZIF-8/HA, and DTX@ZIF-8, K562 cells showed variable susceptibilities to DTX@ZIF-8/HA. Therefore, it can be concluded that DTX@ZIF-8/HA induced apoptosis more successfully than free DTX, ZIF-8/HA, and DTX@ZIF-8, perhaps because of their specific action on leukaemia cancer cells, based on the results of the AO/EtBr double staining investigation. More significant apoptosis was shown in K562 cells in response to DTX@ZIF-8/HA, as opposed to free DTX, ZIF-8/HA, and DTX@ZIF-8 and DTX@ZIF-8/HA. The loaded free DTX, ZIF-8/HA, and DTX@ZIF-8 were explicitly delivered to the cancer cells.
3.5. Nuclear condensation analysis
Changes in cellular shapes, such as chromatin condensation, shrinkage, and blebbing of the cells, indicate apoptosis [Citation35]. Thus, we next used the DAPI staining approach to assess the IC50 concentration of free DTX, ZIF-8/HA, DTX@ZIF-8, and DTX@ZIF-8/HA on chromatin condensation in treated cells. The damaged or compacted nucleus can be stained using the nuclear dye DAPI. The brilliant blue fluorescent nuclei prominently emitted by the treated cells indicate compacted apoptotic nuclei. K562 cells from the untreated, free DTX, ZIF-8/HA, DTX@ZIF-8, and DTX@ZIF-8/HA-treated groups had intact nuclei with good shape, resulting in slight or minimal fluorescence, as shown in . On the other hand, cells that were treated to IC50 concentration of free DTX, ZIF-8/HA, DTX@ZIF-8, and DTX@ZIF-8/HA showed clear blue fluorescent nuclei with aberrant nuclei, indicating condensation and chromatin damage. Overall, our findings show that K562 cells treated with free DTX, ZIF-8/HA, DTX@ZIF-8, and DTX@ZIF-8/HA showed characteristic signs of apoptosis, including the formation of apoptotic bodies and nuclear material condensation. to more thoroughly verify DTX@ZIF-8/HA ‘s inductive function in the K562 leukaemia cancer cell line’s apoptosis.
3.6. Annexin V-Alexa fluor/Propidium iodide staining assay
Flow cytometry is an advanced tool that simultaneously measures several physical properties of a single cell, such as size and granularity. In contrast, the cell is suspended and passes through a measurement apparatus [Citation51–53]. It operates based on the light-scattering characteristics of the studied cells, which can be obtained via dyes or monoclonal antibodies that target intracellular chemicals inside the cell or extracellular molecules on the surface. Based on size and granularity (complexity), forward vs. side scatter (FSC vs. SSC) gating frequently identifies cells of interest. Forward scatter is frequently used to represent cell size, whereas side scatter is associated with the granularity or complexity of the cell. To quantify the effects of free DTX, ZIF-8/HA, DTX@ZIF-8, and DTX@ZIF-8/HA on causing apoptosis, these compounds were treated with K562 cells for 24 h at an IC50 concentration. The impact of treating K562 cells with IC50 concentrations of free DTX, ZIF-8/HA, DTX@ZIF-8, and DTX@ZIF-8/HA for 24 h at equal drug doses, is shown in , which also shows the findings of the flow cytometric analysis. Annexin V-Alexa fluor and propidium iodide are used for dual labelling. analysis showed the proportion of total apoptotic cells treated with free DTX, ZIF-8/HA, DTX@ZIF-8, and DTX@ZIF-8/HA. The necrotic populations within the cell population were found to be following treatment with free DTX, ZIF-8/HA, DTX@ZIF-8, and DTX@ZIF-8/HA. According to these results, DTX@ZIF-8/HA is more likely than free DTX, ZIF-8/HA, and DTX@ZIF-8 to cause apoptosis and have cytotoxic effects on human leukaemia cancer cells (K562).
4. Conclusions
In the present scenario, we demonstrate that encapsulating hydrophobic DTX molecules in ZIF-8 compounds yields DTX@ZIF-8, a solution to providing the payload in a physiological milieu and a quest for outstanding pH-responsiveness of DDS. The DTX@ZIF-8/HA obtained was a smart nanoframeworks with distinctive features, including prolonged drug release, high biocompatibility, and pH responsiveness. This new idea provides new insight into the potential of nanomaterials in modulating cytotoxicity and modifying cell death patterns to enhance the therapy of human leukaemia cancer cells.
Ethics declarations
Not applicable.
Disclosure statement
No potential conflict of interest was reported by the authors.
Data availability
All data generated or analysed during this research are included in this published article.
Additional information
Funding
References
- Ashoub MH, Amiri M, Razavi R, et al. Induction of ferroptosis cell death in acute promyelocytic leukemia cell lines (NB4 and HL-60) using hydrothermally synthesized ZnO NPs in the presence of black cardamom extract. Results Eng. 2023;20:1. doi: 10.1016/j.rineng.2023.101479.
- Elderdery AY, Alzahrani B, Alabdulsalam AA, et al. Synthesis of nickel cobalt-codoped tin oxide nanoparticles from Psidium guajava with anticancer properties. Arab J Chem. 2023;16(2):104481. doi: 10.1016/j.arabjc.2022.104481.
- Janker S, Doswald S, Schimmer RR, et al. Targeted large-volume lymphocyte removal using magnetic nanoparticles in blood samples of patients with chronic lymphocytic leukemia: a proof-of-concept study. Int J Mol Sci. 2023;24(8):7523. doi: 10.3390/ijms24087523.
- Zahariev N, Draganova M, Zagorchev P, et al. Casein-based nanoparticles: a potential tool for the delivery of daunorubicin in acute lymphocytic leukemia. Pharmaceutics. 2023;15(2):471. doi: 10.3390/pharmaceutics15020471.
- Pourmadadi M, Ghaemi A, Shamsabadipour A, et al. Nanoparticles loaded with Daunorubicin as an advanced tool for cancer therapy. Eur J Med Chem. 2023;258:115547. doi: 10.1016/j.ejmech.2023.115547.
- Khademi R, Mohammadi Z, Khademi R, et al. Nanotechnology-based diagnostics and therapeutics in acute lymphoblastic leukemia: a systematic review of preclinical studies. Nanoscale Adv. 2023;5(3):571–17. doi: 10.1039/d2na00483f.
- Wang Y, Yang Y, Zheng X, et al. Application of iron oxide nanoparticles in the diagnosis and treatment of leukemia. Front Pharmacol. 2023;14:1177068. doi: 10.3389/fphar.2023.1177068.
- Arredondo-Beltrán IG, Ramírez-Sánchez DA, Zazueta-García JR, et al. Antitumor activity of bovine lactoferrin and its derived peptides against HepG2 liver cancer cells and Jurkat leukemia cells. Biometals. 2023;36(3):639–655. doi: 10.1007/s10534-022-00484-4.
- Wang W, Lyu C, Wang F, et al. Identification of potential signatures and their functions for acute lymphoblastic leukemia: a study based on the cancer genome atlas. Front Genet. 2021;12:656042. doi: 10.3389/fgene.2021.656042.
- Shen C, Sheng Y, Zhu AC, et al. RNA demethylase ALKBH5 selectively promotes tumorigenesis and cancer stem cell self-renewal in acute myeloid leukemia. Cell Stem Cell. 2020;27(1):64–80.e9. doi: 10.1016/j.stem.2020.04.009.
- Molina O, Abad MA, Solé F, et al. Aneuploidy in cancer: lessons from acute lymphoblastic leukemia. Trends Cancer. 2021;7(1):37–47. doi: 10.1016/j.trecan.2020.08.008.
- Llinàs MC, Martínez-Edo G, Cascante A, et al. Preparation of a mesoporous silica-based nano-vehicle for dual DOX/CPT pH-triggered delivery. Drug Deliv. 2018;25(1):1137–1146. doi: 10.1080/10717544.2018.1472678.
- Parker JP, Ude Z, Marmion CJ. Exploiting developments in nanotechnology for the preferential delivery of platinum-based anti-cancer agents to tumours: targeting some of the hallmarks of cancer. Metallomics. 2016;8(1):43–60. doi: 10.1039/c5mt00181a.
- Han W, Shi L, Ren L, et al. A nanomedicine approach enables co-delivery of cyclosporin a and gefitinib to potentiate the therapeutic efficacy in drug-resistant lung cancer. Signal Transduct Target Ther. 2018;3(1):1–10. doi: 10.1038/s41392-018-0019-4.
- Tabatabaei Rezaei SJ, Amani V, Nabid MR, et al. Folate-decorated polymeric Pt(ii) prodrug micelles for targeted intracellular delivery and cytosolic glutathione-triggered release of platinum anticancer drugs. Polym Chem. 2015;6(15):2844–2853. doi: 10.1039/C5PY00156K.
- Zhou S, Shang Q, Wang N, et al. Rational design of a minimalist nanoplatform to maximize immunotherapeutic efficacy: four birds with one stone. J Control Release. 2020;328:617–630. doi: 10.1016/j.jconrel.2020.09.035.
- Shi S, Chen X, Wei J, et al. Platinum(IV) prodrug conjugated Pd@Au nanoplates for chemotherapy and photothermal therapy. Nanoscale. 2016;8(10):5706–5713. doi: 10.1039/c5nr09120a.
- Begines B, Ortiz T, Pérez-Aranda M, et al. Polymeric nanoparticles for drug delivery: recent developments and future prospects. Nanomaterials. 2020;10(7):1403. doi: 10.3390/nano10071403.
- Taheri M, Ashok D, Sen T, et al. Stability of ZIF-8 nanopowders in bacterial culture media and its implication for antibacterial properties. Chem Eng J. 2021;413:127511. doi: 10.1016/j.cej.2020.127511.
- Barani M, Hajinezhad MR, Shahraki S, et al. Preparation, characterization, and toxicity assessment of carfilzomib-loaded nickel-based metal-organic framework: evidence from in-vivo and in-vitro experiments. J Drug Deliv Sci Technol. 2023;81:104268. doi: 10.1016/j.jddst.2023.104268.
- Ibrahim M, Sabouni R, Husseini GA. Anti-cancer drug delivery using metal organic frameworks (MOFs). Curr Med Chem. 2017;24(2):193–214. doi: 10.2174/0929867323666160926151216.
- Liu W, Yan Q, Xia C, et al. Recent advances in cell membrane coated metal–organic frameworks (MOFs) for tumor therapy. J Mater Chem B. 2021;9(22):4459–4474. doi: 10.1039/d1tb00453k.
- Kim J, Pramanick S, Lee D, et al. Polymeric biomaterials for the delivery of platinum-based anticancer drugs. Biomater Sci. 2015;3(7):1002–1017. doi: 10.1039/c5bm00039d.
- Singh N, Sallem F, Mirjolet C, et al. Polydopamine modified superparamagnetic iron oxide nanoparticles as multifunctional nanocarrier for targeted prostate cancer treatment. Nanomaterials. 2019;9(2):138. doi: 10.3390/nano9020138.
- Viswanadh MK, Agrawal N, Azad S, et al. Novel redox-sensitive thiolated TPGS based nanoparticles for EGFR targeted lung cancer therapy. Int J Pharm. 2021;602:120652. doi: 10.1016/j.ijpharm.2021.120652.
- Sanna V, Sechi M. Nanoparticle therapeutics for prostate cancer treatment. Maturitas. 2012;73(1):27–32. doi: 10.1016/j.maturitas.2012.01.016.
- Bi J, Lu Y, Dong Y, et al. Synthesis of folic acid-modified DOX@ ZIF-8 nanoparticles for targeted therapy of liver cancer. J Nanomater. 2018;2018:1–5. doi: 10.1155/2018/1357812.
- Abbasi Z, Shamsaei E, Fang X-Y, et al. Simple fabrication of zeolitic imidazolate framework ZIF-8/polymer composite beads by phase inversion method for efficient oil sorption. J Colloid Interface Sci. 2017;493:150–161. doi: 10.1016/j.jcis.2017.01.006.
- Feng T, Ai X, Ong H, et al. Dual-responsive carbon dots for tumor extracellular microenvironment triggered targeting and enhanced anticancer drug delivery. ACS Appl Mater Interfaces. 2016;8(29):18732–18740. doi: 10.1021/acsami.6b06695.
- Wang G, Li R, Parseh B, et al. Prospects and challenges of anticancer agents’ delivery via chitosan-based drug carriers to combat breast cancer: a review. Carbohydr Polym. 2021;268:118192. doi: 10.1016/j.carbpol.2021.118192.
- Zhou N, Wang W, Li H, et al. Development and investigation of dual potent anticancer drug-loaded nanoparticles for the treatment of lung cancer therapy. Process Biochem. 2021;106:42–49. doi: 10.1016/j.procbio.2021.03.018.
- Di Ianni T, Bose RJC, Sukumar UK, et al. Ultrasound/microbubble-mediated targeted delivery of anticancer microRNA-loaded nanoparticles to deep tissues in pigs. J Control Release. 2019;309:1–10. doi: 10.1016/j.jconrel.2019.07.024.
- Memariani Z, Abbas SQ, Ul Hassan SS, et al. Naringin and naringeninin as anticancer agents and adjuvants in cancer combination therapy; efficacy and molecular mechanisms of action, a comprehensive narrative review. Pharmacol Res. 2020;171:105264. doi: 10.1016/j.phrs.2020.105264.
- Singh A, Dilnawaz F, Mewar S, et al. Composite polymeric magnetic nanoparticles for co-delivery of hydrophobic and hydrophilic anticancer drugs and MRI imaging for cancer therapy. ACS Appl Mater Interfaces. 2011;3(3):842–856. doi: 10.1021/am101196v.
- Pillai SA, Sharma AK, Desai SM, et al. Characterization and application of mixed micellar assemblies of PEO-PPO star block copolymers for solubilization of hydrophobic anticancer drug and in vitro release. J Mol Liq. 2020;313:113543. doi: 10.1016/j.molliq.2020.113543.
- Lu Y, Low PS. Folate-mediated delivery of macromolecular anticancer therapeutic agents. Adv Drug Deliv Rev. 2002;54(5):675–693. doi: 10.1016/s0169-409x(02)00042-x.
- Pathak S, Ghosh MK, Mandal M, et al. Synthesis of a new acetate bridged Cu(ii) building block generated 1D polymer and studies on structural, magnetic, antibacterial and anticancer properties. New J Chem. 2019;43(4):2019–2029. doi: 10.1039/C8NJ04937H.
- Bayón-Cordero L, Alkorta I, Arana L. Application of solid lipid nanoparticles to improve the efficiency of anticancer drugs. Nanomaterials. 2019;9(3):474. doi: 10.3390/nano9030474.
- Tricot O, Mallat Z, Heymes C, et al. Relation between endothelial cell apoptosis and blood flow direction in human atherosclerotic plaques. Circulation. 2000;101(21):2450–2453. doi: 10.1161/01.cir.101.21.2450.
- Takeda T, Tsubaki M, Kino T, et al. Mangiferin induces apoptosis in multiple myeloma cell lines by suppressing the activation of nuclear factor kappa B-inducing kinase. Chem Biol Interact. 2016;251:26–33. doi: 10.1016/j.cbi.2016.03.018.
- Sjöblom T, Shimizu A, O’Brien KP, et al. Growth inhibition of dermatofibrosarcoma protuberans tumors by the platelet-derived growth factor receptor antagonist STI571 through induction of apoptosis. Cancer Res. 2001;61:5778–5783.
- Pan K, Luo Y, Gan Y, et al. pH-driven encapsulation of curcumin in self-assembled casein nanoparticles for enhanced dispersibility and bioactivity. Soft Matter. 2014;10(35):6820–6830. doi: 10.1039/c4sm00239c.
- Pan K, Chen H, Baek SJ, et al. Self-assembled curcumin-soluble soybean polysaccharide nanoparticles: physicochemical properties and in vitro anti-proliferation activity against cancer cells. Food Chem. 2018;246:82–89. doi: 10.1016/j.foodchem.2017.11.002.
- Yu S, Wang S, Xie Z, et al. Hyaluronic acid coating on the surface of curcumin-loaded ZIF-8 nanoparticles for improved breast cancer therapy: an in vitro and in vivo study. Colloids Surf B Biointerfaces. 2021;203:111759. doi: 10.1016/j.colsurfb.2021.111759.
- Ma Y, Zhao R, Shang H, et al. pH-responsive ZIF-8-based metal–organic-framework nanoparticles for termite control. ACS Appl Nano Mater. 2022;5(8):11864–11875. doi: 10.1021/acsanm.2c02856.
- Ding Y, Yuan J, Mo F, et al. A pH-responsive essential oil delivery system based on metal–organic framework (ZIF-8) for preventing fungal disease. J Agric Food Chem. 2023;71(47):18312–18322. doi: 10.1021/acs.jafc.3c04299.
- Yang C, Xu W, Meng X, et al. A pH-responsive hydrophilic controlled release system based on ZIF-8 for self-healing anticorrosion application. Chem Eng J. 2021;415:128985. doi: 10.1016/j.cej.2021.128985.
- Michalak M, Lach MS, Antoszczak M, et al. Overcoming resistance to platinum-based drugs in ovarian cancer by salinomycin and its derivatives-an in vitro study. Molecules. 2020;25(3):537. doi: 10.3390/molecules25030537.
- Mantawy EM, Said RS, Abdel-Aziz AK. Mechanistic approach of the inhibitory effect of chrysin on inflammatory and apoptotic events implicated in radiation-induced premature ovarian failure: emphasis on TGF-β/MAPKs signaling pathway. Biomed Pharmacother. 2019;109:293–303. doi: 10.1016/j.biopha.2018.10.092.
- Abu-Tahon MA, Ghareib M, Abdallah WE. Environmentally benign rapid biosynthesis of extracellular gold nanoparticles using Aspergillus flavus and their cytotoxic and catalytic activities. Process Biochem. 2020;95:1–11. doi: 10.1016/j.procbio.2020.04.015.
- Li H, Tian J, Wu A, et al. Self-assembled silk fibroin nanoparticles loaded with binary drugs in the treatment of breast carcinoma. Int J Nanomedicine. 2016;11:4373–4380. doi: 10.2147/IJN.S108633.
- Wang X, Zhang Q, Zou L, et al. Facile-synthesized ultrasmall CuS nanocrystals as drug nanocarriers for highly effective chemo–photothermal combination therapy of cancer. RSC Adv. 2016;6(25):20949–20960. doi: 10.1039/C5RA23652E.
- Sun S, White RR, Fischer KE, et al. Inducible aging in Hydra oligactis implicates sexual reproduction, loss of stem cells, and genome maintenance as major pathways. GeroScience. 2020;42(4):1119–1132. doi: 10.1007/s11357-020-00214-z.