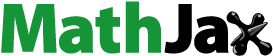
Abstract
This study was conducted to investigate the effects of dietary supplementation of active dry yeast (Saccharomyces cerevisiae) on growth performance, circulating ghrelin, fatty acid concentrations in the gastrointestinal tract, and trace mineral elements utilisation of finishing bulls. Twenty Yanbian cattle (bulls) [body weight (BW) 485 ± 38 kg] were randomly divided into two groups (control and treatment groups) and participated in a 100-day finishing trial. The control group (CON) was fed a basal diet (total mixed ration, TMR). The treatment group (active dry yeast, ADY) was fed a basal diet supplemented with ADY (Levucell SC, Saccharomyces cerevisiae CNCM I-1077, viable count ≥8.0 × 109 CFU/g, 1.0 g/bull/day). ADY supplementation significantly increased the dry matter intake (DMI) of bulls (p < .05); significantly increased the concentration of ghrelin O-acyl transferase (GOAT) (p < .05), significantly decreased the concentration of corticotropin-releasing hormone (CRH) (p < .05), tended to increase the concentration of acyl ghrelin (AG) (p = .053) in serum; significantly increased the content of C12:0 in duodenal digesta, the apparent digestibility of Zn, the concentrations Zn and Cu in serum and the deposition of Cu in the liver (p < .05), significantly decreased the deposition of Fe in muscle in finishing bulls (p < .05). In conclusion, ADY supplementation improved DMI of finishing bulls may be related to the change of AG, and a potential mechanism for the change of AG by ADY supplementation is via its effects on the metabolism of C12:0, Zn, and Cu in finishing bulls.
Active dry yeast (ADY) supplementation increased the dry matter intake (DMI) and tended to increase the concentration of acyl ghrelin (AG) in the serum of finishing bulls.
ADY supplementation increased the content of C12:0 in duodenal digesta and improved the bioavailability of Zn and Cu of finishing bulls.
A potential mechanism for the change of AG by ADY supplementation is via its effects on the metabolism of C12:0, Zn, and Cu in finishing bulls.
HIGHLIGHTS
Introduction
Supplementation of livestock feed with, for example, probiotics have been exploited to improve livestock production, lower production costs, and reduce the negative environmental impacts of livestock production (Al-Khalaifah Citation2018). Active dry yeast (ADY), such as Saccharomyces cerevisiae (CNCM I-1077 and ActisafSc47, etc.) has been widely used as a feed additive for ruminants to optimise rumen fermentation characteristics and prevent health problems. Moreover, it can also improve growth performance including dry matter intake (DMI), average daily gain (ADG), and feed efficiency (McAllister et al. Citation2011; Geng et al. Citation2016b; Liu et al. Citation2021; Maamouri and Ben Salem Citation2022), and improved DMI is one of the important reasons for improving growth performance (Geng et al. Citation2016b). At present, the mechanism of ADY on DMI of beef cattle has not been fully elucidated, but at least, circulating ghrelin concentration is considered to be involved (Geng et al. Citation2016a, Citation2016b, Citation2019). However, the relevant mechanisms for the effect of ADY on ghrelin concentration are still unclear. Ghrelin is a 28-amino acid peptide that exists in two forms, acylated and deacylated, and its acylated state can stimulate animal appetite (Schellekens et al. Citation2012). Some studies have shown that the acylation process of ghrelin requires the participation of fatty acids (Nishi et al. Citation2012; Auclair et al. Citation2019), and not only the amount of fatty acids but also the length of fatty acids (C6–C14) may affect the acylation process of ghrelin (Heppner et al. Citation2012). It has been found that supplementing medium-chain fatty acid calcium (MCFAs) in diets significantly increased the level of acyl ghrelin (AG) of dairy cows (Fukumori et al. Citation2013), which indicates that ingested or increased MCFAs in the gastrointestinal tract are directly involved in the acyl modification process of ghrelin (Nishi et al. Citation2012).
In addition, some trace mineral elements, such as Cu and Zn also have a certain effect on the concentration of ghrelin in piglets (Yin et al. Citation2009; Yang et al. Citation2012), and the effects on ghrelin are related to their bioavailability in animals (Gonzalez-Esquerra et al. Citation2019). Studies have shown that the organic forms of trace mineral elements are considered more bioavailable than the inorganic forms, and the yeast can convert inorganic forms of trace mineral elements into organic forms (Blackwell et al. Citation1995; Zhang et al. Citation2014), thereby increasing the bioavailability of trace mineral elements. These studies above implied that the increased plasma ghrelin concentration stimulated by ADY supplementation in beef cattle also may be related to the improvement of the bioavailability of Cu and Zn. Until now, the effects of ADY supplementation on the concentration of MCFAs in the gastrointestinal tract and the bioavailability of Cu and Zn in beef cattle are limited. Therefore, as a foundation for investigating the mechanism of improving feed intake via live yeast supplementation, this study evaluated the effects of ADY supplementation on growth performance, circulating ghrelin, fatty acid (C6–C14) concentrations in the gastrointestinal tract, and trace mineral elements utilisation of finishing bulls.
Materials and methods
The experiment was carried out from November 2020 to February 2021 in Longjing Mule Animal Husbandry Co., Ltd., Jilin Province, China. All procedures involving animals were performed with the approval of the Yanbian University Institutional Animal Care and Use Committee.
Animals and treatment diets
Twenty Yanbian cattle (bulls) [body weight (BW) 485 ± 38 kg] were selected and randomly divided into two groups (control and treatment groups, 10 bulls in each). The control group (CON) was fed a basal diet (total mixed ration, TMR). The treatment group (ADY) was fed a basal diet supplemented with ADY (Levucell SC, Saccharomyces cerevisiae CNCM I-1077, viable count ≥8.0 × 109 CFU/g), and yeast preparation product was top-dressed for each treatment diet for each bull. ADY was offered at the manufacturers’ recommended inclusion levels of 1.0 g/bull/day during the trial. The ingredients and chemical composition of the basal diets are shown in Table .
Table 1. Ingredient and nutritional composition of basal diets (%, dry matter basis).
Feeding and management
The trial lasted over 100 days including 10 days for adaptation to basal diets and 90 days for data collection. Before the start of the trial, all bulls were ear-tagged, dewormed, and tethered in a tie stall using neck straps. The bulls were individually fed twice a day (05:00 and 17:00 h) and had free access to fresh water. Dry matter intake was individually measured based on the differences between the amount of diet offered and refused daily. Initial and final BW measurements were repeated on two consecutive days before morning feeding, and then the average of the two days was used. The average daily gain was calculated as a difference between initial and final live weight. Gain:feed (G:F) was calculated through the ratio of individual ADG to DMI.
Samples collection
Feed and faecal samples collection
Feed samples were collected for determination of chemical analysis including acid insoluble ash (AIA) and other nutritional compositions shown in Table . Faecal grab samples were collected once a day for seven consecutive days on days 93–99 of the experimental period by spot sampling after the morning feeding. The faeces collected from each bull within 7 days were mixed and stored at −20 °C for measuring the contents of AIA and trace mineral elements Fe, Cu, Zn, Mn, and Se.
Blood and hair samples collection
On the last day of the trial, about 8 mL of blood was collected from each bull before morning feeding by venipuncture with vacuum tubes without anticoagulant and were centrifuged at 3000 × g for 20 min at 4 °C to harvest serum. The serum samples were immediately stored at −20 °C for measurement of the concentrations of intake-related hormones including total ghrelin (TG), deacyl ghrelin (DAG), ghrelin O-acyl transferase (GOAT), proopiomelanocortin (POMC), corticotropin-releasing hormone (CRH), agouti-related protein (AGRP), orexins and neuropeptide Y (NPY), and the contents of trace mineral elements Fe, Cu, Zn, Mn, and Se. Meanwhile, the hairs on the back of the loin of each bull were collected on the last day of the trial for measurement of the contents of trace mineral elements above.
Rumen fluid and duodenal digesta samples collection
At the end of the trial, the bulls were slaughtered following a 12 h fast, all bulls were shipped to a commercial slaughterhouse (Yanji, Jilin Province, China) by a truck for slaughter. After slaughter, the rumen fluid (filtered by four layers of gauze) and duodenal digesta were collected and stored in sterile frozen tubes at −80 °C for measurement of the contents of MCFAs (C6:0, C8:0, C10:0, C12:0) and fatty acids C13:0, C14:0, and C14:1. Among them, duodenal digesta was prepared into freeze-dried powder by a freeze-drying machine (FDU-1200, EYELA, Tokyo, Japan).
Meat and liver samples collection
After slaughter, about 50 g liver samples of each bull were collected, and the surface dirt was removed and put into a clean sealing bag to freeze at −80 °C for measurement of the contents of Fe, Cu, Zn, Mn, and Se. Meanwhile, after 24 h refrigeration, about 100 g longissimus dorsi muscle samples (sirloin) were collected and stored at −80 °C for measurement of the contents of trace mineral elements above.
Analysis of indexes
Hormones and fatty acids
The blood total ghrelin, DAG, GOAT, POMC, CRH, AGRP, orexins, and NPY concentrations were determined using commercial ELISA Kits—ml446287V, ml233015V, ml623074V, ml550452V, ml063545V, ml958711V, ml037849V, and ml061569V, respectively—according to the manufacturer’s instructions (Shanghai Enzyme-linked Biotechnology Co., Ltd., Shanghai, China), and a microplate reader (CMax Plus, Molecular Devices, SV, USA). Briefly, standards or samples are added to microtiter plate wells with a biotin-conjugated antibody specific to the related hormones. Following incubation with avidin conjugated to horseradish peroxidase, substrates A and B were added to act simultaneously with the enzyme conjugate to produce colour. Finally, the optical density (OD) value of each hole was read on the microplate reader under 450 nm. The concentrations of the samples are determined by comparing the OD value of the sample to a standard curve. The intra-assay and inter-assay coefficients of variation (CV) were <10 and 15%, respectively.
The fatty acids contents of basal diet, rumen fluid, and freeze-dried powder of duodenal digesta were determined by capillary gas chromatography according to the method described by O’Fallon et al. (Citation2007). The gas chromatograph-mass spectrometer (GC-MS 7890B-5977A, Agilent, CA, USA) with a capillary column DB-23 (30 m × 320 μm × 0.25 μm film thickness) and the injector and detector temperatures were maintained at 250 and 230 °C, respectively. The oven temperature was programmed at 50 °C for 1 min and increased to 175 °C at 25 °C/min, and increased to 230 °C at 4 °C/min and at 230 °C for 24.75 min. The carried gas was helium, injection volume 1 μL, and the split ratio was 5:1.
Trace mineral elements
The contents of Fe, Cu, Zn, Mn, and Se in basal diet, faeces, hair, liver, serum, and muscle samples were determined in a service corporation (Beijing Sino-UK of Biological Technology, Beijing, China). Among them, kits used to measure the contents of Fe (HY-N0023, Beijing Sino-UK of Biological Technology, Beijing, China), Cu (70541, Shandong BIOBASE Biological Industry Co., Ltd., Shandong, China), and Zn (70543, Shandong BIOBASE Biological Industry Co., Ltd., Shandong, China) by a method of colorimetry and an automatic biochemical analyser (BS-420, Mindray Bio-Medical Electronics Co., Ltd., Shenzhen, China). Kits used to measure the contents of Mn (HY-60011, Beijing Sino-UK of Biological Technology, Beijing, China) by a method of atomic absorption spectroscopy (AAS) and an atomic absorption spectrometer (BH5300, Beijing Bohui Innovation Photoelectric Technology Co., Ltd., Beijing, China). Kits used to measure the contents of Se (HY-60012, Beijing Sino-UK of Biological Technology, Beijing, China) by a method of atomic fluorescence spectrometry and an atomic fluorescence photometer (AF-230E, Beijing Haiguang Instrument Co., Ltd., Beijing, China). For Cu and Zn, the intra-assay and inter-assay CV were <10%. For Fe, Mn, and Se, the intra-assay CV was <7.3%, and the inter-assay CV was <10%.
Apparent digestibility, intake, absorption, and excretion of trace mineral elements
The apparent digestibility of Fe, Cu, Zn, Mn, and Se was calculated by the endogenous indicator method according to Diao et al. (Citation2016). The endogenous indicator was AIA and the equation of apparent digestibility of trace mineral elements (ADTME) is as follows:
where A1 is the AIA content of the feed; A2 is the AIA content of the faeces; B1 is the trace mineral elements content of the feed; B2 is the trace mineral elements content of the faeces.
The absorption and excretion of Fe, Cu, Zn, Mn and Se were calculated according to the following equations: absorption of trace mineral elements (mg/d) = DMI × content of trace mineral elements in the diet × ADTME. Excretion of trace mineral elements (mg/d) = trace mineral elements intake-absorption of trace mineral elements. Where trace mineral elements intake (mg/d) = DMI × content of trace mineral elements in the diet.
Statistics and analysis
Data of this study were analysed by one-way analysis of variance using SPSS 21.0 (SPSS Inc., Chicago, IL, USA) according to the model: Yij = μ + Di + eij, where Yij is the observation of dependent variables, μ is the overall mean, Di represents the fixed effect of treatment, and eij is the residual error of the model. In particular, the final BW, ADG, DMI, and G:F were analysed by the covariance analysis model in the general linear model of SPSS, and the initial BW was taken as the covariate. When the model is significant, the average adopts the estimated value, while when the model is not significant, the average adopts the true value. p < .05 means significant difference, while differences with p > .05 to p < .10 are considered as a trend.
Results
Growth performance
The effects of ADY supplementation on the growth performance of finishing bulls are shown in Table . The average initial BW of bulls in the CON group and ADY group was about 485.50 kg (485.70 and 485.09 kg), and the average final BW of bulls in the CON group and ADY group was 591.41 and 597.78 kg, respectively. ADY significantly increased the DMI of finishing bulls (p < .05), but did not significantly affect the final BW, ADG, and G:F (p > .05).
Table 2. Effects of active dry yeast (ADY) supplementation on growth performance of finishing bulls.
Blood hormone indexes
The effects of ADY supplementation on blood intake-related hormones of finishing bulls are shown in Table . ADY tended to increase the concentration of AG (p = .053) and AG/TG in the serum (p = .092), significantly decreased the concentration of CRH (p < .05), significantly increased the concentration of GOAT in the serum (p < .05), and increased numerically relevant hormones, such as NPY, orexins and AGRP in the serum (p > .05).
Table 3. Effects of active dry yeast (ADY) supplementation on blood intake-related hormones of finishing bulls.
Fatty acids content
The effects of ADY supplementation on fatty acids content in rumen fluid and duodenal digesta of finishing bulls are shown in Table . C8:0 and C10:0 were not detected in the rumen fluid of finishing bulls. ADY did not significantly affect the content of C12:0, C13:0, C14:0, and C14:1 in rumen fluid (p > .05). Furthermore, C8:0 was not detected in duodenal digesta of finishing bulls. ADY did not significantly affect the content of C10:0, C14:0, and C14:1 (p > .05), but significantly increased the content of C12:0 in duodenal digesta (p < .05), and tended to increase the content of C13:0 in duodenal digesta (p = .054).
Table 4. Effects of active dry yeast (ADY) supplementation on selected fatty acids content in rumen fluid and duodenal digesta of finishing bulls.
Apparent digestibility of trace mineral elements
The effects of ADY supplementation on ADTME of finishing bulls are shown in Table . ADY significantly increased the apparent digestibility of Zn (p < .05) but did not significantly affect the apparent digestibility of Fe, Cu, Mn, and Se (p > .05).
Table 5. Effects of active dry yeast (ADY) supplementation on apparent digestibility of trace mineral elements of finishing bulls.
Intake, absorption, and excretion of trace mineral elements
The effects of ADY supplementation on the intake, absorption, and excretion of trace mineral elements of finishing bulls are shown in Table . ADY tended to increase the intake of Fe, Cu, Zn, Mn, and Se (p = .064). ADY significantly increased the absorption of Zn (p < .05), but did not significantly affect the absorption of Fe, Cu, Mn, and Se (p > .05). Meanwhile, ADY did not significantly affect the excretion of Fe, Cu, Zn, Mn, and Se (p > .05).
Table 6. Effects of active dry yeast (ADY) supplementation on intake, absorption, and excretion of trace mineral elements of finishing bulls.
Serum trace mineral elements content
The effects of ADY supplementation on the serum trace mineral elements content of finishing bulls are shown in Table . ADY significantly increased the serum concentrations of Cu and Zn (p < .05), but did not significantly affect the serum concentrations of Fe, Mn, and Se (p > .05).
Table 7. Effects of active dry yeast (ADY) supplementation on serum trace mineral elements content of finishing bulls.
Trace mineral elements content in tissues
The effects of ADY supplementation on trace mineral element contents in tissues of finishing bulls are shown in Table . ADY significantly increased the Cu content in the liver (p < .05), significantly reduced the Fe content in the muscle (p < .05) and tended to reduce the Zn content in the muscle (p = .099). Furthermore, ADY significantly increased the overall deposition of Cu in finishing bulls (p < .05).
Table 8. Effects of active dry yeast (ADY) supplementation on trace mineral elements contents in tissues of finishing bulls.
Discussion
ADY (CNCM I-1077) used in our study, as a commercialised yeast preparation, has been widely used in ruminant production. At present, many studies have shown that ADY supplementation can improve DMI and growth performance, such as ADG and feed efficiency in beef cattle (Geng et al. Citation2016b; Liu et al. Citation2021; Ma et al. Citation2021). Meanwhile, some studies have also shown that ADY supplementation does not significantly improve the DMI and growth performance of beef cattle (Sartori et al. Citation2017; Williams et al. Citation2021). In the present study, ADY supplementation significantly improved the DMI of finishing bulls and did not affect other growth performances of beef cattle, which is similar to the previous studies (Geng et al. Citation2016b; Ran et al. Citation2018). However, the increase in DMI but no difference in G:F may have a negative impact on feed costs. The physiological status of the animal, the types of yeast preparation and the nutrients of the basal diets all may be the factors that lead to variable results in the production performance of beef cattle (Buntyn et al. Citation2016). Additionally, adding yeast to a diet may enhance the population of fibrolytic bacteria, particularly Ruminococcus and Fibrobacter, improve fibre digestion in the rumen, and thus improve DMI (Phesatcha et al. Citation2021; Suntara et al. Citation2021).
At present, although the mechanism of ADY on the feed intake of beef cattle has not been fully elucidated, may be related to its effect on circulating ghrelin concentration (Geng et al. Citation2016a, Citation2016b, Citation2019). Ghrelin is a 28-amino acid acylated peptide, mainly released by specialised gastrointestinal endocrine cells, and it acts as an entero-brain hormone that stimulates feed intake (Kojima et al. Citation1999; Currie et al. Citation2005). Ghrelin exists in two forms, acylated and deacylated, and its acylated state can stimulate animal appetite through orexins and NPY/AGRP in mammals (Gualillo et al. Citation2003; Schellekens et al. Citation2012). The acylation process of the ghrelin requires the ligation of the fatty acid side chain to its serine 3 residue, a rare post-translational modification (acylation) achieved by GOAT. CRH is the most dominant regulator on the systemic hypothalamic-gland-pituitary-adrenocortical (HPA) axis, which will be reduced the activity through the action of ghrelin (Dos-Santos et al. Citation2018). In the present study, ADY supplementation tended to increase the concentration of AG and AG/TG in the serum, significantly decreased the concentration of CRH, significantly increased the concentration of GOAT in the serum, and increased numerically relevant hormones, such as NPY, orexins, and AGRP in the serum. It was reported that exogenous AG can increase time spent eating and DMI in beef cattle (Wertz-Lutz et al. Citation2006). In addition, AG concentration and AG/TG were positively correlated with DMI in beef cattle (Foote et al. Citation2014), and it has been reported that ADY could increase plasma ghrelin concentrations (Geng et al. Citation2018). Therefore, the results of this study further verified that ghrelin is involved in the regulation mechanism of ADY on the feed intake of beef cattle, and the increased concentration of GOAT, the change of AG concentration and decreased concentration of CRH in serum may explain the increased DMI of finishing bulls in this study.
It has been shown that dietary fatty acids can be used for ghrelin acylation in rodents (Nishi et al. Citation2005; Kirchner et al. Citation2009), and not only the amount of fatty acids but also the length of fatty acids can impact the efficiency of ghrelin acylation, fatty acids derived from C6:0 to C14:0 may affect the acylation process of ghrelin (Heppner et al. Citation2012). Moreover, an adequate supply of medium-chain fatty acids (C6:0, C8:0, C12:0) is essential and more effective for ghrelin acylation and GOAT expression (Kirchner et al. Citation2009; Fukumori et al. Citation2013). The length of fatty acids used for ghrelin acylation seems to be important for the metabolic effects of ghrelin, study has shown that C12-acylated ghrelin could promote feed intake in mice, and had a strong effect on adiposity in rodents (Heppner et al. Citation2012). The results of this study showed that ADY supplementation significantly increased the content of C12:0 in duodenal digesta, which may be a reason for the change of AG concentration in serum. It was reported that ADY supplementation can change microflora in the rumen and enhance dietary fat metabolism (Geng et al. Citation2021; Zhang et al. Citation2022), which may be a key reason for the increased concentration of C12:0 in the gastrointestinal tract of beef cattle. In addition, in the process of metabolism in vivo in ruminants, microorganisms could degrade macromolecular nutrients, such as lipids into small molecules, such as fatty acids. And some studies have shown that the addition of ADY increased the number or relative abundance of certain microorganisms, such as Ruminococcus, Fibrobacter, and Selenomonas ruminantium in the rumen (Geng et al. Citation2021; Phesatcha et al. Citation2021; Suntara et al. Citation2021), which may also be the reason for the increase of C12:0 in the gastrointestinal tract. For that, more experimental data are needed to verify these hypotheses. Moreover, fasting before slaughter may cause differences in the results of ruminal components. Therefore, we need to conduct more experiments to enrich relevant data in the future.
Trace mineral elements, such as Fe, Cu, Zn, Mn, and Se in ruminants perform multiple functions including regulating gene expression, nutritional metabolism, immune function, and health (Clegg et al. Citation2005; Bruno et al. Citation2007; Nwosu Citation2019). Mineral utilisation by animals depends not only on the mineral content in their diets but also on the potential bioavailability and mineral absorption in the gastrointestinal tract (Spears Citation2003). It was reported that the biological value of trace elements increased when yeast is used to treat inorganic trace elements (such as Se-enriched yeast) in vitro (Pehrson et al. Citation1999). However, it is unknown whether direct feeding ADY affects the utilisation of trace elements in beef cattle. In this trial, we explored the effect of ADY on the utilisation of trace mineral elements Fe, Cu, Zn, Mn, and Se in finishing bulls. We found that ADY supplementation has a positive effect on the utilisation of trace mineral elements, which significantly increased the apparent digestibility of Zn and the serum concentrations of Cu and Zn, and increased the content of Cu in the liver and overall deposition of Cu in finishing bulls. The organic forms of trace elements are considered more bioavailable than the inorganic forms (Zhang et al. Citation2014). And yeast can convert inorganic forms of trace elements into more easily absorbed by animals in the form of organic matter (Blackwell et al. Citation1995; Zhang et al. Citation2014). Therefore, the increase of Zn apparent digestibility in this trial may be due to the conversion of inorganic zinc into organic zinc in the diet by ADY, which promoted the absorption of Zn by beef cattle. Studies have shown that Cu and Zn can bind to specific sites of metallothionein (MT) in intestinal cells and are absorbed by the small intestine, and MT may be involved in the homeostatic control of Zn and Cu levels in the body (Kägi Citation1991; Irato et al. Citation2001). Moreover, Zn acts by increasing intestinal MT which binds Cu with high affinity (Irato et al. Citation2001). Therefore, the increase in the apparent digestibility of Zn in this trial may increase the content of intestinal MT, thereby promoting the absorption of copper in finishing bulls, thus increasing the concentrations of Cu in serum and liver.
In addition, we found that ADY supplementation significantly decreased the deposition of Fe in muscle and have no significant effect on its overall deposition in this study, which indicated that ADY can change the distribution of trace mineral elements Fe in the tissues of bulls. Some studies have shown that, compared to the direct addition of trace mineral elements in the inorganic form [such as zinc sulphate (ZnSO4), sodium selenite (Na2SeO3), etc.] or no trace mineral elements in diets, the distribution of trace mineral elements in different tissues of lambs, broilers and growing pigs is different after feeding yeast enriched with trace mineral elements (Juniper et al. Citation2008; Azad et al. Citation2017; Falk et al. Citation2018), the results of this trial are similar to the above results. In addition, Storey and Greger (Citation1987) found that high levels of zinc reduced the absorption of iron, and reduced the contents of iron in the liver and tibia in rats. In this trial, ADY supplementation increased the apparent digestibility of Zn, which may also cause the decreased deposition of Fe in the muscle tissue. However, the deeper mechanisms still need to be further explored.
Many studies have shown that Zn and Cu could regulate animals’ appetite and increase their feed intake (Ward and Spears Citation1997; Abdollahi et al. Citation2020), and for growing pigs, their stimulation to ghrelin mRNA expression or secretion in the stomach is the main reason for the increase in feed intake (Yin et al. Citation2009; Yang et al. Citation2012; Gonzalez-Esquerra et al. Citation2019). Therefore, in this trial, the promoting effect of ADY on feed intake may be related to the changes in circulating ghrelin concentration caused by improved bioavailability of Zn and Cu in finishing bulls. However, for ruminants, the mechanism by which Zn and Cu regulate ghrelin has not been revealed, and more experimental data are needed to verify their regulatory mechanism on ghrelin. All in all, the results of this trial provide a new idea for revealing the effect of ADY on the serum ghrelin concentration of beef cattle by trace mineral elements, but more experimental data are needed to verify these hypotheses.
Conclusions
Under the dietary conditions of our study, ADY supplementation increased the DMI of finishing bulls, the concentration of GOAT in serum, and the content of C12:0 in duodenal digesta, improved bioavailability of Zn and Cu, and tended to increase the concentration of AG and AG/TG in the serum of finishing bulls. This improvement in DMI is related to the effect of ADY on ghrelin. A potential mechanism for the change of AG by ADY supplementation is via its effects on the metabolism of C12:0, Cu, and Zn in beef cattle.
Ethical approval
All procedures involving animals were performed with the approval of the Yanbian University Institutional Animal Care and Use Committee.
Disclosure statement
No potential conflict of interest was reported by the author(s).
Data availability statement
The data analysed during the current study are available from the corresponding author on reasonable request.
Additional information
Funding
References
- Abdollahi M, Rezaei J, Fazael H. 2020. Performance, rumen fermentation, blood minerals, leukocyte and antioxidant capacity of young Holstein calves receiving high-surface ZnO instead of common ZnO. Arch Anim Nutr. 74(3):189–205.
- Al-Khalaifah HS. 2018. Benefits of probiotics and/or prebiotics for antibiotic-reduced poultry. Poult Sci. 97(11):3807–3815.
- Auclair N, Patey N, Melbouci L, Ou Y, Magri-Tomaz L, Sané A, Garofalo C, Levy E, St-Pierre DH. 2019. Acylated ghrelin and the regulation of lipid metabolism in the intestine. Sci Rep. 9(1):17975.
- Azad SK, Shariatmadari F, Torshizi MK, Ahmadi H. 2017. Effect of zinc concentration and source on performance, tissue mineral status, activity of superoxide dismutase enzyme and lipid peroxidation of meat in broiler chickens. Anim Prod Sci. 58(10):1837–1846.
- Blackwell KJ, Singleton I, Tobin JM. 1995. Metal cation uptake by yeast: a review. Appl Microbiol Biotechnol. 43(4):579–584.
- Bruno RS, Song Y, Leonard SW, Mustacich DJ, Taylor AW, Traber MG, Ho E. 2007. Dietary zinc restriction in rats alters antioxidant status and increases plasma F2 isoprostanes. J Nutr Biochem. 18(8):509–518.
- Buntyn JO, Schmidt TB, Nisbet DJ, Callaway TR. 2016. The role of direct-fed microbials in conventional livestock production. Annu Rev Anim Biosci. 4:335–355.
- Clegg MS, Hanna LA, Niles BJ, Momma TY, Keen CL. 2005. Zinc deficiency-induced cell death. IUBMB Life. 57(10):661–669.
- Currie PJ, Mirza A, Fuld R, Park D, Vasselli JR. 2005. Ghrelin is an orexigenic and metabolic signaling peptide in the arcuate and paraventricular nuclei. Am J Physiol Regul Integr Comp Physiol. 289(2):R353–R358.
- Diao H, Gao Z, Yu B, Zheng P, He J, Yu J, Huang Z, Chen D, Mao X. 2016. Effects of benzoic acid (VevoVitall®) on the performance and jejunal digestive physiology in young pigs. J Anim Sci Biotechnol. 7:32.
- Dos-Santos RC, Grover HM, Reis LC, Ferguson AV, Mecawi AS. 2018. Electrophysiological effects of ghrelin in the hypothalamic paraventricular nucleus neurons. Front Cell Neurosci. 12:275.
- Falk M, Bernhoft A, Framstad T, Salbu B, Wisløff H, Kortner TM, Kristoffersen AB, Oropeza-Moe M. 2018. Effects of dietary sodium selenite and organic selenium sources on immune and inflammatory responses and selenium deposition in growing pigs. J Trace Elem Med Biol. 50:527–536.
- Foote AP, Hales KE, Lents CA, Freetly HC. 2014. Association of circulating active and total ghrelin concentrations with dry matter intake, growth, and carcass characteristics of finishing beef cattle. J Anim Sci. 92(12):5651–5658.
- Fukumori R, Sugino T, Shingu H, Moriya N, Kobayashi H, Hasegawa Y, Kojima M, Kangawa K, Obitsu T, Kushibiki S, et al. 2013. Ingestion of medium chain fatty acids by lactating dairy cows increases concentrations of plasma ghrelin. Domest Anim Endocrinol. 45(4):216–223.
- Geng CY, Ji S, Jin YH, Li CY, Xia GJ. 2018. Comparison of blood immunity, antioxidant capacity and hormone indexes in finishing bulls fed active dry yeast (Saccharomyces cerevisiae) and yeast culture. Int J Agric Biol. 20(11):2561–2568.
- Geng CY, Meng QX, Ren LP, Zhou ZM, Zhang M, Yan CG. 2016a. Comparison of ruminal fermentation parameters, fatty acid composition and flavour of beef in finishing bulls fed active dry yeast (Saccharomyces cerevisiae) and yeast culture. Anim Prod Sci. 58(5):841–847.
- Geng CY, Meng QX, Zhang M. 2019. Correlations between circulating ghrelin concentrations and growth performance, carcass traits, meat quality indices in finishing bulls fed high-concentrate diets. IOP Conf Ser Earth Environ Sci. 346(1):012088.
- Geng CY, Ren LP, Zhou ZM, Chang Y, Meng QX. 2016b. Comparison of active dry yeast (Saccharomyces cerevisiae) and yeast culture for growth performance, carcass traits, meat quality and blood indexes in finishing bulls. Anim Sci J. 87(8):982–988.
- Geng CY, Yang LY, Ji S, Jin YH, Zhang M. 2021. Effect of ADY and YC on concentration of ruminal medium chain fatty acid, lactic acid, ethanol and relative abundance of bacteria in beef cattle. IJAB. 25(2):455–459.
- Gonzalez-Esquerra R, Araujo RB, Haese D, Kill JL, Cunha AF, Monzani PS, Lima CG. 2019. Effect of dietary copper sources on performance, gastric ghrelin-RNA expression, and growth hormone concentrations in serum in piglets. J Anim Sci. 97(10):4242–4247.
- Gualillo O, Lago F, Gómez-Reino J, Casanueva FF, Dieguez C. 2003. Ghrelin, a widespread hormone: insights into molecular and cellular regulation of its expression and mechanism of action. FEBS Lett. 552(2–3):105–109.
- Heppner KM, Chaudhary N, Müller TD, Kirchner H, Habegger KM, Ottaway N, Smiley DL, Dimarchi R, Hofmann SM, Woods SC, et al. 2012. Acylation type determines ghrelin’s effects on energy homeostasis in rodents. Endocrinology. 153(10):4687–4695.
- Irato P, Santon A, Ossi E, Albergoni V. 2001. Interactions between metals in rat liver and kidney: localization of metallothionein. Histochem J. 33(2):79–86.
- Juniper DT, Phipps RH, Ramos-Morales E, Bertin G. 2008. Selenium persistency and speciation in the tissues of lambs following the withdrawal of dietary high-dose selenium-enriched yeast. Animal. 2(3):375–380.
- Kägi JH. 1991. Overview of metallothionein. Methods Enzymol. 205:613–626.
- Kirchner H, Gutierrez JA, Solenberg PJ, Pfluger PT, Czyzyk TA, Willency JA, Schürmann A, Joost HG, Jandacek RJ, Hale JE, et al. 2009. GOAT links dietary lipids with the endocrine control of energy balance. Nat Med. 15(7):741–745.
- Kojima M, Hosoda H, Date Y, Nakazato M, Matsuo H, Kangawa K. 1999. Ghrelin is a growth-hormone-releasing acylated peptide from stomach. Nature. 402(6762):656–660.
- Liu S, Shah AM, Yuan M, Kang K, Wang Z, Wang L, Xue B, Zou H, Zhang X, Yu P, et al. 2021. Effects of dry yeast supplementation on growth performance, rumen fermentation characteristics, slaughter performance and microbial communities in beef cattle. Anim Biotechnol. 3:1–11.
- Ma J, Wang C, Wang Z, Cao G, Hu R, Wang X, Zou H, Kang K, Peng Q, Xue B, et al. 2021. Active dry yeast supplementation improves the growth performance, rumen fermentation, and immune response of weaned beef calves. Anim Nutr. 7(4):1352–1359.
- Maamouri O, Ben Salem M. 2022. The effect of live yeast Saccharomyces cerevisiae as probiotic supply on growth performance, feed intake, ruminal pH and fermentation in fattening calves. Vet Med Sci. 8(1):398–404.
- McAllister TA, Beauchemin KA, Alazzeh AY, Baah J, Teather RM, Stanford K. 2011. Review: the use of direct fed microbials to mitigate pathogens and enhance production in cattle. Can J Anim Sci. 91(2):193–211.
- Nishi Y, Hiejima H, Hosoda H, Kaiya H, Mori K, Fukue Y, Yanase T, Nawata H, Kangawa K, Kojima M. 2005. Ingested medium-chain fatty acids are directly utilized for the acyl modification of ghrelin. Endocrinology. 146(5):2255–2264.
- Nishi Y, Mifune H, Kojima M. 2012. Ghrelin acylation by ingestion of medium-chain fatty acids. Methods Enzymol. 514:303–315.
- Nwosu O. 2019. A systematic review of the impact of minerals on pregnant sheep and goats and their offspring in the African continent [Ph.D. thesis]. University of Pretoria.
- O’Fallon JV, Busboom JR, Nelson ML, Gaskins CT. 2007. A direct method for fatty acid methyl ester synthesis: application to wet meat tissues, oils, and feedstuffs. J Anim Sci. 85(6):1511–1521.
- Pehrson B, Ortman K, Madjid N, Trafikowska U. 1999. The influence of dietary selenium as selenium yeast or sodium selenite on the concentration of selenium in the milk of Suckler cows and on the selenium status of their calves. J Anim Sci. 77(12):3371–3376.
- Phesatcha K, Phesatcha B, Chunwijitra K, Wanapat M, Cherdthong A. 2021. Changed Rumen fermentation, blood parameters, and microbial population in fattening steers receiving a high concentrate diet with Saccharomyces cerevisiae improve growth performance. Vet Sci. 8(12):294.
- Ran T, Shen YZ, Saleem AM, AlZahal O, Beauchemin KA, Yang WZ. 2018. Using ruminally protected and nonprotected active dried yeast as alternatives to antibiotics in finishing beef steers: growth performance, carcass traits, blood metabolites, and fecal Escherichia coli. J Anim Sci. 96(10):4385–4397.
- Sartori ED, Canozzi MEA, Zago D, Prates ÊR, Velho JP, Barcellos JOJ. 2017. The effect of live yeast supplementation on beef cattle performance: a systematic review and meta-analysis. JAS. 9(4):21–37.
- Schellekens H, Finger BC, Dinan TG, Cryan JF. 2012. Ghrelin signalling and obesity: at the interface of stress, mood and food reward. Pharmacol Ther. 135(3):316–326.
- Spears JW. 2003. Trace mineral bioavailability in ruminants. J Nutr. 133(5 Suppl 1):1506S–1509S.
- Storey ML, Greger JL. 1987. Iron, zinc and copper interactions: chronic versus acute responses of rats. J Nutr. 117(8):1434–1442.
- Suntara C, Cherdthong A, Uriyapongson S, Wanapat M, Chanjula P. 2021. Novel Crabtree negative yeast from rumen fluids can improve rumen fermentation and milk quality. Sci Rep. 11(1):6236.
- Ward JD, Spears JW. 1997. Long-term effects of consumption of low-copper diets with or without supplemental molybdenum on copper status, performance, and carcass characteristics of cattle. J Anim Sci. 75(11):3057–3065.
- Wertz-Lutz AE, Knight TJ, Pritchard RH, Daniel JA, Clapper JA, Smart AJ, Trenkle A, Beitz DC. 2006. Circulating ghrelin concentrations fluctuate relative to nutritional status and influence feeding behavior in cattle. J Anim Sci. 84(12):3285–3300.
- Williams MS, Mandell IB, Bohrer BM, Wood KM. 2021. The effects of feeding benzoic acid and/or live active yeast (Saccharomyces cerevisiae) on beef cattle performance, feeding behavior, and carcass characteristics. Transl Anim Sci. 5(4):txab143.
- Yang W, Wang J, Zhu X, Gao Y, Liu Z, Zhang L, Chen H, Shi X, Yang L, Liu G. 2012. High lever dietary copper promote ghrelin gene expression in the fundic gland of growing pigs. Biol Trace Elem Res. 150(1–3):154–157.
- Yin J, Li X, Li D, Yue T, Fang Q, Ni J, Zhou X, Wu G. 2009. Dietary supplementation with zinc oxide stimulates ghrelin secretion from the stomach of young pigs. J Nutr Biochem. 20(10):783–790.
- Zhang X, Dong X, Wanapat M, Shah AM, Luo X, Peng Q, Kang K, Hu R, Guan J, Wang Z. 2022. Ruminal pH pattern, fermentation characteristics and related bacteria in response to dietary live yeast (Saccharomyces cerevisiae) supplementation in beef cattle. Anim Biosci. 35(2):184–195.
- Zhang XG, Peng YN, Li XR, Ma GD, Wang MG. 2014. Higher bioavailability of organic bound zinc from high zinc-enriched fungi. Int J Vitam Nutr Res. 84(5–6):277–285.