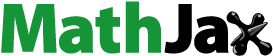
Abstract
The objective was to evaluate the effectiveness of lactic acid bacteria (LAB) inoculants and chemical additives on the fermentation quality, aerobic stability and in vitro gas production kinetics and digestibility of total mixed ration (TMR) silage. Total mixed ration (568 g/kg dry matter (DM)) was ensiled with six experimental treatments: (1) no additives (control); (2) Lactobacillus buchneri (LB; applied at 1 × 106 cfu/g fresh weight (FW)); (3) Lactobacillus casei (LC; applied at 1 × 106 cfu/g FW); (4) calcium propionate (CAP; applied at 0.5% FW); (5) sodium diacetate (SD; applied at 0.5% FW); (6) potassium sorbate (PS; applied at 0.1% FW). All silos (18 L) were opened for fermentation quality, in vitro gas production kinetics and digestibility analysis after 90 days of ensiling, and then subjected to aerobic stability test for 14 days. All the TMR silage was well-preserved with low pH (4.36 ∼ 4.66) and acceptable levels of butyric acid (1.02 ∼ 2.51 g/kg DM) and ammonia nitrogen (86.3 ∼ 107 g/kg total nitrogen). All the groups were steady during 14 days of aerobic exposure, while SD group was more stable with lower (p < 0.05) yeast (4.60 vs. 5.17 ∼ 5.77 log10 cfu/g FW) and mould (3.33 vs. 4.12 ∼ 4.64 log10 cfu/g FW) populations than other treated groups on day 14. Moreover, SD group had the highest (p < 0.05) in vitro digestibility of dry matter (67.8 vs. 56.6 ∼ 63.5%) and neutral detergent fibre (61.7 vs. 50.5 ∼ 57.4%) among all groups. Overall, SD is recommended as additive to improve fermentation quality, in vitro ruminal digestibility, and aerobic stability of TMR silage.
After 14 days of aerobic exposure, the yeast and mould populations in TMR silage can be efficiently inhibited by sodium diacetate treatment.
Sodium diacetate treatment evidently enhanced in vitro digestibility of dry matter and neutral detergent fibre compared to other treatments.
Sodium diacetate is recommended as additive to improve fermentation quality, in vitro ruminal digestibility, and aerobic stability of TMR silage.
HIGHLIGHTS
Introduction
Total mixed ration (TMR) is a type of complete diet formula that can meet the nutritional requirements of ruminants. The TMR materials are ensiled in a sealed silo to create an anaerobic environment and fermented for a long time, which is defined as TMR silage (Dai et al. Citation2022). Recently, the ruminant production industry has shown a renewed interest in TMR silage in many countries, like China, Japan, Brazil, Vietnam, South Korea, Indonesia, Thailand, Israel, Nepal, South Africa, Italy, and Argentina (Schmidt et al. Citation2017; Borreani et al. Citation2019). Several benefits have been associated with TMR silage, such as the potential for incorporating unpalatable byproducts, uniform composition during storage under farm conditions, and a reduced requirement for labour and machinery (Bueno et al. Citation2020). The TMR silage can also stabilise rumen function, avoid self-selection by animals, and increase the dry matter intake and digestibility in ruminants (Cao et al. Citation2011).
The hygienic quality of silage is important for animal health, animal production and food quality. Lactic acid bacteria (LAB) inoculants and chemical additives have been developed to help prevent or reduce the growth and activity of undesirable microorganisms in silage, and enhance the fermentation quality and aerobic stability of silage (Queiroz et al. Citation2013). Inoculants containing Lactobacillus buchneri have been used to avoid or limit the aerobic deterioration of maize silage (Kleinschmit and Kung Citation2006). The inoculation of Lactobacillus casei could decrease the biogenic amine production regardless of the silage type (Nishino et al. Citation2007). However, biological additives may not be effective, because they depend on environmental conditions and forage characteristics (Muck Citation2013). Consequently, chemical additives may be more robust than biological additives (Kung et al. Citation2003). Calcium propionate, sodium diacetate, and potassium sorbate are salts of organic acids with antifungal properties that are often used as food preservatives. Calcium propionate has been widely used as main components of preservatives in food and forage because of its ability to inhibit the growth of moulds and other microorganisms (Mills and Kung Citation2002). Sodium diacetate can easily ionise into acetic acid, inhibiting the activity of undesirable microorganisms during the fermentation process (Li et al. Citation2022). Okur et al. (Citation2022) also reported that sodium diacetate treatment of high-moisture corn silage resulted in substantial improvement in stability of the fermented biomass after air infiltration. Potassium sorbate has been applied in corn silage to improve aerobic stability due to antimicrobial activity (Kung et al. Citation2018). Knický and Spörndly (Citation2009) characterised the potassium sorbate as being effective in inhibiting spore-forming bacteria, yeasts and moulds at a pH range from 3 to 6. However, few studies have simultaneously compared several bacterial and chemical additives for improving TMR silage preservation.
Therefore, the study aimed to determine the effects of two LAB inoculants and three chemical additives on the fermentation quality, aerobic stability, and in vitro gas production kinetics and digestibility of TMR silage.
Materials and methods
Total mixed ration silage preparation and treatments
Total mixed ration consisted of common vetch, whole-crop oat, hulless barley straw, and mixed concentrate. Common vetch, whole-crop oat and hulless barley were cultivated in the experimental field of the Shigatse Grassland Station (Tibet, China: N 29°270', E 88°880'). All of the crops were harvested on 25 September 2015. Whole-crop oat was harvested at the milk ripe stage. Hulless barley straw was the residue remaining after grain harvest. Common vetch was harvested at the pod bearing stage. Mixed concentrate (New Hope Feed Co., Ltd., Lhasa, China) contained 20% rape cake meal, 7.5% crack corn, 27.5% distillers dried grains with solubles, 20% wheat bran, 20% whole cottonseed, and 5% vitamin-mineral. Vitamin-mineral supplement contained 2450 mg/kg Cu, 20 mg/kg Co, 10000 mg/kg Mn, 21500 mg/kg Fe, 620 mg/kg I, 210 mg/kg Se, 10500 mg/kg Zn, and minimum of 25 IU/g of vitamin E, 1100 IU/g of vitamin D, 3500 IU/g of vitamin A. The harvested crops were chopped to lengths of approximately 20 mm. The chemical compositions of ingredients for TMR are shown in Table . The ingredient proportions, chemical compositions and microbial populations of TMR before ensiling are presented in Table .
Table 1. Chemical compositions (g/kg DM, unless otherwise stated) of ingredients used in total mixed ration silage.
Table 2. Ingredient proportions, chemical compositions and microbial populations of pre-ensiled total mixed ration.
Five laboratory silos (18 L capacity) were individually prepared for each of the following treatments: (1) deionised water alone (control); (2) Lactobacillus buchneri (LB) applied at 1 × 106 colony-forming units (cfu)/g fresh weight (FW); (3) Lactobacillus casei (LC) applied at 1 × 106 cfu/g FW; (4) calcium propionate (CAP; food-grade additive, purity 99%, purchased from Aokai Biotechnology Development Co., Ltd., Nantong, China) applied at 0.5% FW; (5) sodium diacetate (SD; food-grade additive, purity 99%, purchased from Jirong Amino acid Co., Ltd., Jinzhou, China) applied at 0.5% FW; (6) potassium sorbate (PS; food-grade additive, purity 98%, purchased from Wanglong Science and Technology Co., Ltd., Ningbo, China) applied at 0.1% FW. Lactobacillus buchneri and L. casei strains were both provided by the Institute of Ensiling and Processing of Grass in Nanjing Agricultural University of China. Inoculants were made and enumerated in deMan, Rogosa and Sharp (MRS) broth (Shanghai Bio-way Technology Co., Ltd., Shanghai, China) to determine the amount of inoculant required to meet the targeted inoculation rate of 1 × 106 cfu/g FW for L. buchneri and L. casei. Oliveira et al. (Citation2017) reported that the most effective rates for improving the fermentation quality were 105 and 106 cfu/g FW, and the latter rate was more effective. Hence, 1 × 106 cfu/g FW was used as the inoculant application rate in this experiment. Inoculants were mixed with deionised water and uniformly sprayed onto the raw material with a manual sprayer, followed by constant mixing. The chemical additives were diluted in deionised water, and 10 mL of solution per kg of raw material was applied with a manual sprayer. The application rates of chemical additives were according to the report of Dai et al. (Citation2022). The control TMR silage was sprayed with an equivalent volume of deionised water. Each silo was packed with approximately 8 kg of raw material to achieve a packing density of about 240 kg of dry matter (DM) per m3. A total of 30 silos (6 treatments × 5 replicates) were hermetically sealed with screw tops and maintained in a barn at room temperature (21 ∼ 25 °C) for 90 days.
After 90 days of ensiling, the silos were opened. At silo opening, approximately the top 6-cm TMR silage from the surface of each experimental unit was discarded in order to avoid contamination. The whole TMR silage mass was then emptied on a polyethylene plastic film and mixed thoroughly. A portion of silage mass was collected for fermentation quality and microbiological analyses. An approximate 3-kg allotments were taken for aerobic stability test.
Aerobic stability
Approximately 3 kg of TMR silage mass was transferred in sterile polyethylene bottle (10 L capacity) without compaction and allowed to be exposed to air for 14 days at ambient temperature (18 ∼ 22 °C). The TMR silage surface was covered with a double layer of gauze to minimise evaporation and dust contamination while allowing the ingress of air. Five replicated TMR silage of each treatment were sampled to determine pH values, organic acids, water soluble carbohydrate, and ammonia nitrogen contents as well as microbial populations after 0, 6, 9 and 14 days during aerobic exposure. Due to the low ambient temperature (<20 °C) in practical production of Tibet, the monitoring-temperature method reported by Wang et al. (Citation2020) was not used to evaluate the aerobic stability in this study. Aerobic deterioration was defined as a rise in pH value of silage by 0.5 above the initial pH value (on day 0 of the aerobic stability test) according to the method of Chen et al. (Citation2017).
Chemical and microbiological analyses
At sampling, fresh forage, pre-ensiled TMR and TMR silage mass was thoroughly mixed and collected after any wastage had been removed. The first subsample (20.0 g) was homogenised with 100 mL of distilled water, and filtrated with sterile gauze. The filtrate was stored at −20 °C and used for measuring pH, buffering capacity, ammonia nitrogen (NH3-N), organic acid and ethanol contents. The second subsample (150 g) was oven-dried at 65 °C for 48 h, and ground to pass a 1-mm screen and prepared for determining DM, fibre, water soluble carbohydrate (WSC), ether extracts (EE), ash and total nitrogen (N) contents. The third subsample (10.0 g) was mixed with 90 mL sterilised saline in a 200-mL conical flask and shaken for 10 min at 120 rpm in a constant temperature shaker, followed by filtration with sterile gauze and continuously dilutions (10−1∼10−7) in sterilised saline in several 2-mL tubes. Then, 1 mL of liquid from each tube was used to count microbial populations.
The pH was measured with a pH metre (Mettler Toledo, Zurich, Switzerland). The buffering capacity was measured based on the method of Jasaitis et al. (Citation1987). The concentrations of organic acid (lactic acid, acetic acid, propionic acid, butyric acid) and ethanol contents were analysed by HPLC using an Agilent HPLC 1260 system (Agilent Technologies, Inc., Santa Clara, CA, USA) fitted with a Carbomix H-NP5 column (Sepax Technologies, Inc., Newark, DE, USA) and a refractive index detector (Agilent Technologies, Inc., Santa Clara, CA, USA). The eluent used was 2.5 mmol/L H2SO4 at 0.5 mL/min at 55 °C. Total volatile fatty acids (VFAs) were calculated as the sum of acetic acid (AA), propionic acid (PA) and butyric acid (BA), expressed on a DM basis. The concentration of NH3-N was measured by the phenol-hypochlorite method (Broderick and Kang Citation1980). Silage quality was evaluated with the fermentation end-products as described by Conaghan et al. (Citation2010). Fermentation end-products (FP) were calculated as the sum of lactic acid (LA), AA, PA, BA and ethanol, expressed on a DM basis. The LA concentration was expressed as a percentage of fermentation end-products (LA: FP). The classifications of silage quality are very bad (≤ 50% LA: FP and ≥ 25 g/kg DM of BA), bad (≤ 50% LA: FP and <25 g/kg DM of BA), medium (50–60% LA: FP), good (61–70% LA: FP) and excellent (>70% LA: FP).
The DM of raw material and TMR silage was measured by drying the samples in a forced-air oven until reaching a constant weight. The WSC concentrations were determined with the methods of Owens et al. (Citation1999). The acid detergent fibre (ADF, method 973.18), DM (method 930.15), EE (method 920.39), ash (method 942.05), and crude protein (CP, method 984.13) contents were analysed based on the standard procedures of Association of Official Analytical Chemists guidelines (AOAC Citation2000). Amylase-treated neutral detergent fibre (aNDFom) content was analysed using an Ankom200 Fibre Analyser (Ankom Technology, Macedon, NY, USA) according to the method of Reich and Kung (Citation2010), heat stable amylase and sodium sulphite were used in the aNDF procedure and the results of aNDFom and ADFom were expressed on DM basis exclusive of residual ash. Non-fibrous carbohydrate (NFC) was calculated by the formula:
(NRC Citation2000).
For enumeration of the microorganisms, 10 g sample (pre-ensiled TMR or TMR silage mass) was shaken well with 90 mL of sterilised saline solution (8.50 g/L NaCl) at 120 rpm for 2 h. Then 1 mL solution was used for 10-fold serial dilution for microorganism counting. The colonies of lactic acid bacteria (LAB) were counted on MRS agar medium after incubation in an anaerobic incubator (N2: H2: CO2 = 85:5:10, YQX-II; CIMO Medical Instrument Manufacturing Co., Ltd., Shanghai, China) at 37 °C for 3 days. Aerobic bacteria were cultured and counted on nutrient agar medium (Nissuiseiyaku Ltd., Tokyo, Japan). Yeasts and moulds were counted on potato dextrose agar (Nissui-seiyaku Ltd., Tokyo, Japan) and acidified with sterilised tartaric acid solution to pH 3.5. Yeasts were distinguished from moulds and other bacteria by colony appearance and the observation of cell morphology. These agar plates were incubated at 37 °C for 3 days. The microbial data was obtained as colony-forming units (cfu) and were transformed to a logarithmic scale on a FW basis.
In vitro gas production and digestibility
The following procedures in this study were approved by the Ethics Committee of Nanjing Agricultural University (Jiangsu, Nanjing). The TMR silage samples after 90 days of ensiling were used to measure the in vitro gas production and digestibility according to the reports of Wang et al. (Citation2020). The difference between initially incubated DM and residual DM, corrected by the blanks after the incubation, was calculated to determine in vitro DM digestibility (IVDMD). The IVDMD and in vitro neutral detergent fibre digestibility (IVNDFD) were determined and calculated using the methods described by Liu et al. (Citation2016). The organic matter digestibility (OMD), metabolisable energy (ME), net energy for lactation (NEL), short chain fatty acid contents (SCFA), and microbial crude protein (MCP) biomass production were predicted according to Wang et al. (Citation2020).
The kinetic parameters of gas production (GP) were determined by fitting gas production data to the nonlinear equation:
where, Y is the volume of gas produced at time t, b is the potential gas production (mL), c is the gas production rate constant. Parameters b and c were estimated by an iterative least square method using a non-linear regression (NLIN) option of the Statistical Analysis Systems (SAS Citation2001).
Statistical analysis
A completely randomised experimental design was used with 6 treatments and 5 replicates per treatment. All statistical procedures were performed with Statistical Packages for the Social Sciences (SPSS 13.0 for Windows; SPSS Inc., Chicago, IL, USA). Data on fermentation characteristics and in vitro characteristics of TMR silage was subjected to one-way analysis of variance (ANOVA):
where, Yi is the dependent variable; μ is the least square mean; Ti is the effect of additives; and eij is the residual error term.
Data on aerobic stability parameters of TMR silage was analysed by two-way analysis of variance according to the model for a factorial treatment design as follows:
where Yij is the dependent variable; µ is overall mean; Ti is the effect of additives; Dj is the effect of exposure days; (T × D)ij is the effect of interaction between additives and exposure days; and eij is the residual error term.
Statistical differences among means were determined using Tukey’s multiple comparison. Values of p < 0.05 were declared significant.
Results and discussion
Chemical compositions and microbial populations of pre-ensiled TMR
Common vetch had high CP contents (∼188 g/kg DM) and buffering capacity (∼320 mEq/kg DM) (Table ). High WSC (∼139 g/kg DM) and NFC contents (∼378 g/kg DM) were found in whole-crop oat. Hulless barley straw had high DM (∼968 g/kg FW) and aNDFom (∼576 g/kg DM) contents. High DM (∼904 g/kg FW), ash (∼114 g/kg DM), WSC (∼110 g/kg DM) and NFC (∼506 g/kg DM) contents and low aNDFom (∼161 g/kg DM) contents were observed in mixed concentrate.
Well-fermented silage can be produced from a material that has adequate DM content (250 ∼ 400 g/kg DM), high content of WSC, low buffering capacity, and adequate LAB population to compete against the undesirable microorganisms prior to ensiling (Chen et al. Citation2017). Herein, a relatively higher DM content (∼568 g/kg FW) was found in pre-ensiled TMR (Table ), which may inhibit the lactic acid fermentation during ensiling. The moisture content of silage material plays an important role in influencing silage fermentation, because moisture is required by LAB for metabolic reactions and has a significant effect on the initial level and transport of oxygen during ensilage process (Ni et al. Citation2014). It is possible that the high DM contents of silage limit the multiplication and metabolic activity of the indigenous LAB strains (Keshri et al. Citation2019). Thus, it is necessary to add the LAB inoculants into the pre-ensiled TMR to accelerate the lactic acid fermentation and improve the silage quality. In addition, an adequate WSC content of raw material is also an important factor for obtaining good fermentation and preservation of silage. In the present study, the pre-ensiled TMR had a WSC content of approximately 97.0 g/kg DM, and this was higher than 60 ∼ 70 g/kg DM (considered as a theoretical requirement for making good silage), which might promote the growth of favourable microorganisms, facilitate lactic acid fermentation and ensure the successful fermentation over the ensiling progress (Wang et al. Citation2017).
It is worth noting that high populations of yeasts (7.12 log10 cfu/g FW) and moulds (6.37 log10 cfu/g FW) were found in pre-ensiled TMR. During ensiling, the growth of yeasts induces a large loss of DM and energy, which are principally fermented to ethanol, CO2 and water under anaerobic condition (Carvalho et al. Citation2014). After exposure, yeasts are the main initiators of aerobic spoilage by consuming valuable sugars and lactic acid, thus raising the pH and allowing an increase of silage inner temperature (Pahlow et al. Citation2003). Finally, moulds complete the deterioration of silage (Dolci et al. Citation2011). For well-preserved silage, the populations of moulds and yeasts should not exceed 3 ∼ 4 log10 cfu/g FW (McEniry et al. Citation2006), and higher numbers of yeasts and moulds in raw material indicate higher risk of aerobic deterioration after exposure. Hence, it is necessary to use some antimicrobial additives to improve the fermentation quality and aerobic stability of TMR silage. Consequently, we hypothesised that the pre-ensiled TMR could be used to produce high-quality TMR silage with the help of LAB inoculants and antimicrobial additives.
Fermentation characteristics, chemical compositions and microbial populations of TMR silage
In the current study, the lactic acid contents in all treated groups varied from 80.5 to 114 g/kg DM (Table ), which were within the ideal range of values from 80 to 120 g/kg DM as recommended by Chamberlain and Wilkinson (Citation1996). Greater (p < 0.05) lactic acid (95.1 ∼ 114 vs. 74.1 ∼ 84.9 g/kg DM) contents were found in LB and LC groups compared to other groups. It demonstrated the efficiency of LAB inoculants in accelerating lactic acid fermentation and converting WSC into organic acids, thus lowering pH. Moreover, LC group had higher (p < 0.05) lactic acid (114 vs. 95.1 g/kg DM) contents and lower (p < 0.05) pH (4.36 vs. 4.55) than LB group. It is related to their different metabolic pathways. Holzer et al. (Citation2003) reported that L. casei belongs to facultative hetero-fermentative LAB, and usually ferments hexoses homo-fermentatively into lactic acid but, under special conditions, hetero-fermentative metabolism into lactic acid, CO2 and ethanol (or acetic acid) occurs. Regarding L. buchneri, it belongs to obligate hetero-fermentative LAB, and ferments hexoses to lactic acid, CO2 and ethanol (or acetic acid). Hence, as LAB inoculants of silage, L. casei performed better than L. buchneri in accumulating lactic acid and decreasing pH during ensilage. The highest (p < 0.05) ratio of LA to AA and LA: FP in LC group also proved an enhanced homo-fermentation in LC-treated TMR silage.
Table 3. Fermentation characteristics, chemical compositions and microbial populations of total mixed ration silage after 90 days of ensiling.
The CAP, SD and PS groups had higher (p < 0.05) lactic acid (80.5 ∼ 84.9 vs. 74.1 g/kg DM) contents, and lower (p < 0.05) yeast (5.22 ∼ 5.84 vs. 6.19 log10 cfu/g FW) and mould (3.34 ∼ 3.85 vs. 5.69 log10 cfu/g FW) populations than control. This may be due to the antimicrobial property of these chemical additives, which inhibits the growth of undesirable microorganisms and promotes lactic acid fermentation during ensiling (Dai et al. Citation2022; Han et al. Citation2022). The calcium propionate, sodium diacetate, and potassium sorbate additives can ionise to produce according organic acids (mainly propionic, acetic, and sorbic acids) and salt ions, which have acidic characteristics and antibacterial property (Wen et al. Citation2017). The un-dissociated molecules of these organic acids can pass through the plasma membrane and liberate protons to acidify the cytoplasm, thus preventing the growth of yeasts and moulds (Kleinschmit et al. Citation2005). Furthermore, the CAP and SD groups had the highest (p < 0.05) propionic acid (12.4 g/kg DM) and acetic acid (22.2 g/kg DM) contents, respectively, among all groups. It may result from the ionisation of calcium propionate and sodium diacetate to produce the propionic and acetic acids. It also partly explained the highest (p < 0.05) VFAs contents in CAP and SD groups.
The presence of butyric acid in silage is undesirable given that its generation is an energy-waste metabolism, and butyric acid >5 g/kg DM is an indicator of substantial clostridial activity reducing feed intake and causing health issues (McDonald et al. Citation1995). Herein, the butyric acid contents in all groups were less than 3.00 g/kg DM, suggesting good fermentation. The NH3-N level is also an important parameter for evaluating silage quality and reflects the degradation of crude protein via proteolysis by plant protease activity and/or clostridium fermentation (Ogunade et al. Citation2018). In the present study, the pre-ensiled TMR contained a large proportion (45% FW) of common vetch, and the NH3-N contents (<110 g/kg total N) in all groups were less than the guideline (150 g/kg total N for legume silage) of Buxton et al. (Citation2003), indicating good fermentation quality. Han et al. (Citation2022) reported that the high DM content can inhibit the growth of clostrida and enterobacteria, which may be responsible for the low butyric acid and NH3-N contents in all groups. In addition, all the chemical additives in this study decreased (p < 0.05) NH3-N contents (87.6 ∼ 93.1 vs. 104 g/kg total N) compared to control, suggesting these antimicrobial additives could effectively suppress the proteolysis during ensiling. It is noteworthy that LB group had similar (p > 0.05) or even higher (p < 0.05) levels of butyric acid and NH3-N than control. It was probably because L. buchneri was more sensitive to the high DM condition in TMR silage, resulting in its lower metabolic activity during the early stage of ensiling.
Yeasts could metabolise WSC to produce CO2 and ethanol during ensiling (Weinberg Citation2008). The growth of yeasts was inhibited by LAB inoculants and chemical additives, as indicated by lower (p < 0.05) yeast populations (5.22 ∼ 5.84 vs. 6.19 log10 cfu/g FW) and ethanol contents (0.633 ∼ 2.62 vs. 8.45 g/kg DM) compared to control. It may be because LAB inoculants promoted the lactic acid fermentation and chemical additives ionised the corresponding organic acids, which rapidly reduced the pH and restricted the growth of yeast. Interestingly, similar (p > 0.05) LAB populations were found among LB (7.97 log10 cfu/g FW), LC (7.61 log10 cfu/g FW) and control (7.78 log10 cfu/g FW) groups. Muck (Citation1988) reported that the absence of an inoculant effect on the LAB population after the fermentation process is associated with factors that limit its growth, such as nutrient shortage or the accumulation of excretory products of the organism, resulting in insufficient conditions to cause a rapid decrease in pH and to overcome the epiphytic population.
Aerobic stability
Silage is inevitably exposed to air when used for feeding, leading to undesirable microbial multiplication, decreasing nutritive value, and increasing the risk of potential pathogens (Driehuis and Oude Elferink Citation2000). Aerobic spoilage is primarily initiated by lactic acid-assimilating yeasts, which can thrive well in high lactic acid-containing silage (Carvalho et al. Citation2012). Breakdown of lactic acid leads to rise in pH, eventually providing better conditions for multiplication of opportunistic aerobic bacteria (e.g. Bacilli, Listeria monocytogenes) and moulds (e.g. Aspergillus, Fusarium, Pencillium), which degrade silage subsequently to severe extent (Woolford Citation1990; Pahlow et al. Citation2003). Herein, aerobic deterioration was defined as a rise in pH value of silage by 0.5 above the initial pH value (Chen et al. Citation2017).
The microorganisms related to aerobic deterioration can increase pH and nutrient loss (McDonald et al. Citation1995). In this experiment, the pH increased by 0.06, 0, 0.11, 0.25, 0.08, and 0.18 for control, LB, LC, CAP, SD, and PS groups, respectively, during 14 days of aerobic exposure (Table ). It indicated that all the groups were steady during 14 days of aerobic exposure. It was probably due to the sufficient acetic acid (11.5 ∼ 22.2 g/kg DM) and propionic acid (2.74 ∼ 12.4 g/kg DM) contents in TMR silage after air exposure. It has been proven that propionic acid and acetic acid can effectively restrict the growth and activity of undesirable microorganisms, thus improving the aerobic stability of silage (Kung et al. Citation1998; Danner et al. Citation2003). The lactic acid contents in treated groups decreased to varying degrees, which may be correlated with the consumption of lactate-assimilating yeasts (Carvalho et al. Citation2012). The production of ethanol from WSC was mainly dominated by yeast activity (da Silva et al. Citation2020), and high ethanol content was commonly accompanied with high yeast numbers and activity. Thus, the dramatic (p < 0.05) reduction of ethanol content in control group suggested that the metabolism of yeasts in control group was limited during aerobic exposure.
Table 4. Changes in pH and fermentation products of total mixed ration silage after exposure to air.
After 14 days of aerobic exposure, SD group had lower (p < 0.05) yeast (4.60 vs. 5.17 ∼ 5.77 log10 cfu/g FW) and mould (3.33 vs. 4.12 ∼ 4.64 log10 cfu/g FW) populations than other treated groups (Table ). It was probably because sodium diacetate can ionise to acetic acid with antimicrobial property, and the un-dissociated acetic acid can get through the bacterial plasma membrane and disrupt the microbial metabolism (Partanen and Jalava Citation2008). It also indicated that SD had superior inhibitory effect on the yeast and mould populations than other treatments during aerobic exposure. Danner et al. (Citation2003) reported that the antimicrobial effect of an organic acid depends on its pKa and pH, and organic acid shows higher antimicrobial activity when pH is lower than its pKa. Herein, the pKa of the CAP, SD, and PS was similar, and SD had greater antimicrobial effect probably due to its relatively lower pH (4.67 vs. 4.72 ∼ 4.87) during aerobic exposure.
Table 5. Changes in chemical compositions and microbial populations of total mixed ration silage after exposure to air.
Table 6. In vitro gas production kinetics and digestibility, and available energy of total mixed ration silage after 90 days of ensiling.
In vitro gas production kinetics and ruminal digestibility
In vitro culture has been conducted as a common method to predict rumen digestibility of ruminants. The IVDMD is an important parameter that reflects the rate of feed utilisation in rumen, and the digestion of DM mainly includes WSC, protein, fibre contents, and other substances (Chen et al. Citation2019). Herein, LB, CAP, SD and PS groups had numerically (p > 0.05) or significantly (p < 0.05) higher IVDMD (61.6 ∼ 67.8% vs. 59.4%) and IVNDFD (55.5 ∼ 61.7% vs. 52.3%) than control (), which may be associated with the increase of WSC contents (27.7 ∼ 46.9 g/kg DM vs. 24.6 g/kg DM). There is no significant (p > 0.05) difference in OMD, SCFA, ME, and NEL among all groups. Moreover, CAP, SD, and PS groups had numerically (p > 0.05) or significantly (p < 0.05) higher cumulative GP at incubation time 72h (GP72) (203 ∼ 209 mL vs. 195 mL), potential gas production (205 ∼ 210 mL vs. 200 mL), and gas production rate constant (0.0505 ∼ 0.0529 mL/h vs. 0.0469 mL/h) than control, which may be attributed to the inhibition effects on the growth of yeasts and moulds, thus reducing the nutrient loss and providing sufficient substrates and energy for microbial metabolism (Dai et al. Citation2022).
Conclusions
All the TMR silage was well-preserved with low pH and acceptable levels of NH3-N and butyric acid. During 14 days of aerobic exposure, all the groups were steady, while SD was more stable with lower yeast and mould populations than other treatments on day 14. SD obviously improved the IVDMD and IVNDFD of TMR silage. Hence, under the environment of Tibet (high altitude and low ambient temperature), chemical additives especially SD performed superior than biological additives in improving fermentation quality, in vitro ruminal digestibility, and aerobic stability of TMR silage.
Ethical approval
All animal experimental protocols were approved by the Animal Care and Use Committee of Nanjing Agricultural University.
Disclosure statement
No potential conflict of interest was reported by the author(s).
Data availability statement
The data that support the findings of this study are available from the corresponding author, ST, upon reasonable request.
Additional information
Funding
References
- [AOAC] Association of Official and Analytical Chemist. 2000. Official methods of analysis. 18th edn. Washington, DC: AOAC.
- Borreani G, Ferrero F, Tabacco E. 2019. Baled silage management. In: Nussio LG, Da Silva EB, Oliveira KS, Gritti VC, Salvo PAR, Salvati GGS, De Sousa DO, editors. 6th international symposium on forage quality and conservation. Piracicaba: ESALQ. p. 219–246.
- Broderick GA, Kang JH. 1980. Automated simultaneous determination of ammonia and total amino acids in ruminal fluid and in vitro media. J Dairy Sci. 63(1):64–75.
- Bueno AVI, Lazzari G, Jobim CC, Daniel JLP. 2020. Ensiling total mixed ration for ruminants: a review. Agronomy. 10(6):879.
- Buxton DR, Muck RE, Harrison JH, Mahanna B, & Chase LE. 2003. Practical Applications and Solutions to Silage Problems. Madison: ASCSSA-SSSA, Agronmy.
- Cao Y, Cai Y, Hirakubo T, Fukui H, Matsuyama H. 2011. Fermentation characteristics and microorganism composition of total mixed ration silage with local food by‐products in different seasons. Anim Sci J. 82(2):259–266.
- Carvalho BF, Avila CLS, Pinto JC, Neri J, Schwan RF. 2014. Microbiological and chemical profile of sugar cane silage fermentation inoculated with wild strains of lactic acid bacteria. Anim Feed Sci Technol. 195:1–13.
- Carvalho BF, Fernandes BT, Piza R, Nascimento LB, Teresinha BT, Andrade RR. 2012. Fermentation and aerobic stability of corn silage inoculated with Lactobacillus buchneri. Rev Bras Zootecn. 41:2369–2373.
- Chen L, Yuan XJ, Li JF, Wang SR, Dong ZH, Shao T. 2017. Effect of lactic acid bacteria and propionic acid on conservation characteristics, aerobic stability and in vitro gas production kinetics and digestibility of whole-crop corn based total mixed ration silage. J Integr Agr. 16(7):1592–1600.
- Chen L, Yuan XJ, Li JF, Dong ZH, Wang SR, Guo G, Shao T. 2019. Effects of applying lactic acid bacteria and propionic acid on fermentation quality, aerobic stability and in vitro gas production of forage-based total mixed ration silage in Tibet. Anim Prod Sci. 59(2):376–383.
- Chamberlain AT, Wilkinson JM. 1996. Feeding the dairy cow. Lincoln: Chalcombe Publications.
- Conaghan P, O'Kiely P, O'Mara FP. 2010. Conservation characteristics of wilted perennial ryegrass silage made using biological or chemical additives. J Dairy Sci. 93(2):628–643.
- Dai T, Dong D, Wang S, Zong C, Yin X, Jia Y, Shao T. 2022. The effectiveness of chemical additives on fermentation profiles, aerobic stability and in vitro ruminal digestibility of total mixed ration ensiled with Napier grass and wet distillers’ grains in southeast China. Italian J Anim Sci. 21(1):979–989.
- Danner H, Holzer M, Mayrhuber E, Braun R. 2003. Acetic acid increases stability of silage under aerobic conditions. Appl Environ Microbiol. 69(1):562–567.
- da Silva EB, Savage RM, Biddle AS, Polukis SA, Smith ML, Kung L. Jr. 2020. Effects of a chemical additive on the fermentation, microbial communities, and aerobic stability of corn silage with or without air stress during storage. J Anim Sci. 98(8):1–11.
- Dolci P, Tabacco E, Cocolin L, Borreani G. 2011. Microbial dynamics during aerobic exposure of corn silage stored under oxygen barrier or polyethylene films. Appl Environ Microbiol. 77(21):7499–7507.
- Driehuis F, Oude Elferink SJ. 2000. The impact of the quality of silage on animal health and food safety: a review. Vet Q. 22(4):212–216.
- Han Z, Xu G, Wang S, Dai T, Dong D, Zong C, Shao T. 2022. Antimicrobial effects of four chemical additives on fermentation quality, aerobic stability, and in vitro ruminal digestibility of total mixed ration silage prepared with local food by‐products. Anim Sci J. 93(1):e13755.
- Holzer M, Mayrhuber E, Danner H, Braun R. 2003. The role of Lactobacillus buchneri in forage preservation. Trends Biotechnol. 21(6):282–287.
- Jasaitis DK, Wohlt JE, Evans JL. 1987. Influence of feed ion content on buffering capacity of ruminant feedstuffs in vitro. J Dairy Sci. 70(7):1391–1403.
- Keshri J, Chen Y, Pinto R, Kroupitski Y, Weinberg ZG, Sela Saldinger S. 2019. Bacterial dynamics of wheat silage. Front Microbiol. 10:1532.
- Kleinschmit DH, Schmidt RJ, Kung L. Jr. 2005. The effects of various antifungal additives on the fermentation and aerobic stability of corn silage. J Dairy Sci. 88(6):2130–2139.
- Kleinschmit DH, Kung L. 2006. A meta-analysis of the effects of Lactobacillus buchneri on the fermentation and aerobic stability of corn and grass and small-grain silages. J Dairy Sci. 89(10):4005–4013.
- Knický M, Spörndly R. 2009. Sodium benzoate, potassium sorbate and sodium nitrite as silage additives. J Sci Food Agric. 89(15):2659–2667.
- Kung L, Jr, Sheperd AC, Smagala AM, Endres KM, Bessett CA, Ranjit NK, Glancey JL. 1998. The effect of preservatives based on propionic acid on the fermentation and aerobic stability of corn silage and a total mixed ration. J Dairy Sci. 81(5):1322–1330.
- Kung L, Jr, Stokes MR, Linc J. 2003. Silage additives. In: Buxton DR, Muck RE, and Harrison JH, editors. Silage science and technology. Madison, WI: American Society of Agronomy, Crop Science Society of America, Soil Science Society of America. p. 305–360.
- Kung L, Jr, Smith ML, da Silva EB, Windle MC, da Silva TC, Polukis SA. 2018. An evaluation of the effectiveness of a chemical additive based on sodium benzoate, potassium sorbate, and sodium nitrite on the fermentation and aerobic stability of corn silage. J Dairy Sci. 101(7):5949–5960.
- Li YF, Wang LL, Jeong EC, Kim HJ, Ahmadi F, Kim JG. 2022. Effects of sodium diacetate or microbial inoculants on aerobic stability of wilted rye silage. Anim Biosci. 35(12):1871–1880.
- Liu QH, Li XY, Desta ST, Zhang JG, Shao T. 2016. Effects of Lactobacillus plantarum and fibrolytic enzyme on the fermentation quality and in vitro digestibility of total mixed rations silage including rape straw. J Integr Agr. 15(9):2087–2096.
- McDonald P, Edwards RA, Greenhalgh JFD, Morgan CA, Sinclair LA. 1995. Animal nutrition. Harlow, Essex: Longman Scientific & Technical.
- McEniry J, O’Kiely P, Clipson NJW, Forristal PD, Doyle EM. 2006. The microbiological and chemical composition of baled and precision-chop silages on a sample of farms in County Meath. Irish J Agr Food Res. 45:73–83.
- Mills JA, Kung L. 2002. The effect of delayed ensiling and application of a propionic acid-based additive on the fermentation of barley silage. J Dairy Sci. 85(8):1969–1975.
- Muck RE. 1988. Factors influencing silage quality and their implications for management. J Dairy Sci. 71(11):2992–3002.
- Muck RE. 2013. Recent advances in silage microbiology. AFSci. 22(1):3–15.
- Ni K, Wang Y, Pang H, Cai Y. 2014. Effect of cellulase and lactic acid bacteria on fermentation quality and chemical composition of wheat straw silage. AJPS. 05(13):1877–1884.
- Nishino N, Hattori H, Wada H, Touno E. 2007. Biogenic amine production in grass, maize and total mixed ration silages inoculated with Lactobacillus casei or Lactobacillus buchneri. J Appl Microbiol. 103(2):325–332.
- NRC 2000. Nutrient Requirements of Beef Cattle: update 2000. Washington, DC: National Academy Press.
- Ogunade IM, Jiang Y, Pech Cervantes AA, Kim DH, Oliveira AS, Vyas D, Weinberg ZG, Jeong KC, Adesogan AT. 2018. Bacterial diversity and composition of alfalfa silage as analyzed by Illumina MiSeq sequencing: effects of Escherichia coli O157: h 7 and silage additives. J Dairy Sci. 101(3):2048–2059.
- Okur AA, Gozluklu K, Okur E, Okuyucu B, Koc F, Ozduven ML. 2022. Effects of apple vinegar addition on aerobic deterioration of fermented high moisture maize using infrared thermography as an indicator. Sensors. 22(3):771.
- Oliveira AS, Weinberg ZG, Ogunade IM, Cervantes AAP, Arriola KG, Jiang Y, Kim D, Li X, Gonçalves MCM, Vyas D, et al. 2017. Meta-analysis of effects of inoculation with homofermentative and facultative heterofermentative lactic acid bacteria on silage fermentation, aerobic stability, and the performance of dairy cows. J Dairy Sci. 100(6):4587–4603.
- Owens V, Albrecht K, Muck R, Duke S. 1999. Protein degradation and fermentation characteristics of red clover and alfalfa silage harvested with varying levels of total nonstructural carbohydrates. Crop Sci. 39(6):1873–1880.
- Pahlow G, Muck RE, Driehuis F, Oude Elferink SJWH, Spoelstra SF. 2003. Microbiology of ensiling. In: Buxton DR, Muck RE and Harrison HJ, editors. Silage science and technology (Agronomy Series No. 42). Madison, WI: American Society of Agronomy. p. 31–93.
- Partanen K, Jalava T. 2008. Effects of some organic acids and salts on microbial fermentation in the digestive tract of piglets estimated using an in vitro gas production technique. AFSci. 14(4):311–324.
- Queiroz OCM, Arriola KG, Daniel JLP, Adesogan AT. 2013. Effects of 8 chemical and bacterial additives on the quality of corn silage. J Dairy Sci. 96(9):5836–5843.
- Reich LJ, Kung L. 2010. Effects of combining Lactobacillus buchneri 40788 with various lactic acid bacteria on the fermentation and aerobic stability of corn silage. Anim Feed Sci Technol. 159(3-4):105–109.
- [SAS] Statistical Analysis System. 2001. Inc: SAS® User’s Guide: Statistics. ver. 8.2 ed. Cary, NC: SAS Institution.
- Schmidt P, Restelatto R, Zopollatto M. 2017. Ensiling total mixed rations –An innovative procedure. In: Nussio LG, Sousa DO, Gritti VC, Salvati GGS, Santos WP, Salvo PAR, editors. V International symposium on forage quality and conservation. Piracicaba: FEALQ. p. 7–20.
- Wang S, Yuan X, Dong Z, Li J, Shao T. 2017. Effect of ensiling corn stover with legume herbages in different proportions on fermentation characteristics, nutritive quality and in vitro digestibility on the Tibetan Plateau. Grassl Sci. 63(4):236–244.
- Wang S, Zhao J, Yu C, Li J, Tao X, Chen S, Shao T. 2020. Nutritional evaluation of wet brewers’ grains as substitute for common vetch in ensiled total mixed ration. Italian J Anim Sci. 19(1):1015–1025.
- Weinberg ZG. 2008. Preservation of forage crops by solid-state lactic acid fermentation-ensiling. New York: Springer. p. 443–467.
- Wen AY, Yuan XJ, Jian W, Desta ST, Shao T. 2017. Effects of four short-chain fatty acids or salts on dynamics of fermentation and microbial characteristics of alfalfa silage. Anim Feed Sci Tech. 223:141–148.
- Woolford MK. 1990. The detrimental effects of air on silage. J Appl Bacteriol. 68(2):101–116.