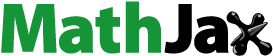
Abstract
Tea waste (TW) has a protein content of approximately 30% and the potential to replace other protein sources. However, the effect of dietary TW supplement is still ambiguous. The objective of this study was to investigate the effect of three levels of a dietary TW supplement on the performances and physiological changes in goats. Nine multiparous crossbred Saanen lactating goats were arranged in three replicates of a 3 × 3 Latin square. The three groups of goats were fed with 0, 5, or 10 by weight [wt%] dry matter [DM] basis TW, respectively, for a 21-day period. All diets were a total mixed ration (TMR) containing a 40:60 (DM basis) ratio of concentrate: roughage. The goats were weighed at 15 and 21 days. Faeces and urine were collected during the last 7 days of each period. The TW addition had no effect on the DM intake (DMI), DMI% and body weight. The milk protein content in goats on a 10 wt% TW diet was significantly higher (p < 0.05) than that of goats on the other diets. The milk lactose of both TW diets significantly decreased when compared to the control diet (p < 0.01). Goats with 5 and 10 wt% TW tended to decrease digestibility of crude protein (p = 0.10). There were no changes in the DM digestibility, blood glucose, blood urea nitrogen, triglyceride, total protein, ruminal pH, ammonia-nitrogen, total volatile fatty acids, acetic acid (A), propionic acid (P), A:P ratio, nitrogen balance, allantoin excretion and methane emission among treatments. Diets with 5 or 10 wt% TW (DM basis) could be used to replace other plant protein sources.
The tea waste replaced as protein source up to 10% in goat feed with no effect on dry matter intake, blood profile and methane production.
The 10% tea waste diet increased milk protein content in dairy goat.
Low dose of condensed tannin in tea waste provided more protein absorption in small intestine by reducing ruminal NH3–N level.
Highlights
Introduction
Goats play an important role in the rural economy of many developing countries around the globe. However, most Asian small-scale goat farmers do not have access to the relevant information and advanced technologies to increase their outputs, giving them very limited opportunities to improve their production as well as their livelihood. Another factor which plays an important role in raising goats is the cost of feed, especially the protein components.
Tea waste (TW) is a by-product of the beverage industry that can be used as a protein source with no negative environmental impact (Kondo et al. Citation2004a). Tea leaves contain a variety of amino acids, proteins, tannins, and polyphenols (Yamamoto et al. Citation1997), indicating that TW may have the potential as a protein supplementation in animal feed.
Protein-rich commercial feedstuffs (e.g. soybean meal and alfalfa hay etc.) are often used in livestock production, but they are generally expensive. Protein-rich forage became a renewed interest after the occurrence of bovine spongiform encephalopathy and some cost variations in soy products due to climate change and other factors. The protein content of TW ranges from 27.63% to 31.10% by weight (wt%) (Xu et al. Citation2007). In addition, goats fed with 200 g green (G)TW with oat silage led to an increased nitrogen (N) retention and a constant dry matter intake (DMI) (Kondo et al. Citation2004a). However, TW also contains a high level of tannin, which can reduce its nutritive value because tannin binds to the feed protein and makes it unavailable for ruminal microorganisms. Ruminants fed tannin-rich diets showed a decreased feed intake (Silanikove et al. Citation1996), rumen degradability, and feed digestibility (Kondo et al. Citation2007).
High concentrations of tannin reduce the voluntary feed intake and nutrient digestibility, whereas low to moderate concentrations may improve the digestive utilisation of feed due to the reduction in protein degradation in the rumen and a subsequent increase in amino acid flow to the small intestine (Frutos et al. Citation2004).
The objective of this study aimed to investigate the effects of different levels of TW addition (0, 5, and 10 wt%) in diets on the productive performances, feed digestibility, N balance, biochemical, and ruminal parameters in crossbred Saanen lactating goats.
Materials and methods
The trial was carried out under the relevant guidelines and regulations on the use of animals for scientific purposes certified by the Chulalongkorn University Animal Care and Use Committee (permission No. 1731050).
Animal, diet, and management
The experiment was assigned to three replicates of a 3 × 3 Latin square. Nine multiparous early-lactating crossbred Saanen goats, with a body weight (BW) of approximately 30.00 ± 3.50 kg were used in this experiment. Nine lactating goats were randomly assigned to the three replicates. Goats were raised in metabolic crates and provided with feed, water, and mineral block ad libitum. Basal diet contained corn silage mixed with concentrate in 2:3 (w/w, dry matter [DM] basis) ratio in the form of a total mixed ration (TMR). Green tea waste from local company had compositions of DM 18.00 g/kg, crude protein 227.00 g/kg DM and condensed tannins 38.26 g/kg DM. There were three dietary treatments. The first treatment was fed with a basal diet (the control group), while the two treatment groups were fed diets containing TW at 5 and 10 wt% (DM basis), respectively. All diets were isonitrogenous (16% crude protein; CP) and isocaloric (1.60 Mcal/kg of net energy lactation [NEL] on a DM basis) (NRC Citation1981). The experimental period lasted for 21 days, and was made up of a 14-day adaptation period followed by a 7-day collection period. Each goat was given the respective dietary treatment for 21 days, fed ad libitum twice a day at 07:00 h and 16:00 h.
Data collection and sampling procedure
All goats were weighed at 15 and 21 days to determine their BW. Daily feed intake was determined from the difference between the provided and remaining feed. Samples of feed, faeces, and urine were randomly collected daily by the total collection method during the last 7 days of each experimental period. Feed and faeces samples were dried in an air-oven at 103 °C for 24 h to determine the DM. Sub-samples of feed and faeces were dried at 60 °C and kept at −20 °C until analysis. They were analysed for organic matter (OM), CP, ash (AOAC Citation1995), acid detergent fibre (ADF), neutral detergent fibre (NDF) (Van Soest et al. Citation1991) levels. A 200 g of ground dried feed and tea waste sample was sent to Thailand Institute of Scientific and Technological Research (TISTR) for condensed tannin analysis (HPLC, LC-20A/PDA, Shimadzu, Japan). Total urine was collected daily using plastic containers and then sulphuric acid was added to 10% (v/v) to prevent nitrogen loss. Nitrogen and allantoin levels in the excreted urine were determined by the Kjeldahl (AOAC Citation1995) and colorimetric (Young and Conway Citation1942) methods, respectively.
Goats were manually milked at 07:00 h and 15:00 h every day, recording the milk yield. Fresh milk samples were collected and mixed with formalin [1 mL of 40% (w/v) formalin in 60 mL of fresh milk] and then kept at −20 °C until analysis. Milk samples were analysed for their lactose, protein, total solids, and fat contents using an automatic Milkoscan™ 133B (N. Foss Electric Denmark). Heparinised blood samples of goats were collected via the jugular vein at 2.5 h post-feeding (Sano et al. Citation1999) at 15 and 20 days. These samples were centrifuged at 1000 g for 10 min, the plasma (supernatant) was removed and kept at −20 °C until analysis. These plasma samples were analysed for total protein by the modified biuret method; glucose by glucose oxidase method; blood urea nitrogen (BUN) by an enzyme kinetic method; and triglyceride by an enzyme colorimetric method using ILAB 650 (Milano, Italy).
Ruminal fluid samples were collected from each goat using an oral stomach tube connected to a syringe. Fluid samples were taken before feeding and at 2 and 4 h after feeding and then the ruminal pH was immediately measured using a glass electrode pH metre (MettlerToldo, S220 Seven Compact™, Thailand). After that, they were filtered through two layers of cheese-cloth, preserved by adding 24% (v/v) metaphosphoric acid to stop fermentation, and then frozen at −20 °C prior to analysis. Volatile fatty acid (VFA) concentrations were determined by gas chromatography (GC; Agilent 7000 series, Triple quad GC/MS, Agilent Technologies, UK). Ammonium (NH3)–N concentration was measured by the micro-Kjeldahl procedure (AOAC Citation1995). Nitrogen retention was calculated from the difference between the N input (from the DMI) and the N output (from the N level in the faeces, urine, and milk). The methane (CH4) emission level was calculated from EquationEquation (1)(1)
(1) (Moss et al. Citation2000):
(1)
(1)
where C2, C3, and C4 represent acetic, propionic, and butyric acids, respectively.
Statistical analysis
The numerical results are presented as the mean value ± 1 standard deviation (mean ± SD). A 3 × 3 latin square design was performed in three replicates. The blocking factors were periods and goats. The data were analysed using the General Linear Model (GLM) procedure of the SAS version 9.4 (SAS Citation2015), and compared by the Duncan’s multiple range tests (Steel and Torrie Citation1980), accepting significance at the p < 0.05 level. The model for analysing the data was:
(2)
(2)
with h = 1, 2, 3, i = 1,…, 9, j = 1, 2, 3 and k = 1,2,3, where μ was the overall mean, Rh the random effect of replicates, Gi(h) the random effect of goats nesting the i within the h replicate, Pj the random effect of period, Tk the fixed effect of treatment, and εhijk the random residual error.
Results
Feed composition, nutrient intake and digestibility
The feed ingredients and chemical composition of the experimental diets are shown in Table . All diets were composed of a similar composition except for the condensed tannin (CT) contents. The CT concentrations in all diets were 0.90, 2.50 and 4.90 g/kg DM, respectively. There were no significant differences (p > 0.05) in the DMI and DMI/%BW between treatments and the control . However, there were significant differences (p < 0.05) in DMI (g/d) and DMI(%BW) between 5 wt% TW and 10 wt% TW (Table ). Apparently, the digestibility of DM, OM, and CP was not significantly different (p > 0.05) among the goats in the control, 5 wt% TW and 10 wt% TW dietary groups. A significant difference (p < 0.05) was found in the NDF digestibility (NDFD) and the ADF digestibility (ADFD). The NDFD showed significant difference (p < 0.05) between 10 wt% TW diet group and the remaining groups. Moreover, the differences of ADFD (p < 0.05) were found between the control and both TW groups.
Table 1. Feed ingredients and chemical compositions of experimental diets.
Table 2. Effects of TW substitution on the nutrient intake and digestibility.
Milk yield and composition
The values of the total milk yield, percentage of milk composition, and total yield of milk composition are shown in Table . There were no significant differences (p > 0.05) in the milk yield and milk composition among the three dietary groups except for the milk protein percentage and lactose percentage. Goats fed with TW at 10 wt% TW diet showed a significant difference (p < 0.05) in milk protein percentage at 3.16% compared to those fed with the control diet and 5 wt% TW diet at 2.96%. The lactose percentages showed significant differences (p < 0.01) between the control diet group and both the TW supplemented groups. The highest lactose percentage was found in the control diet group at 4.45% while the values in the 5 and 10 wt% TW groups were 4.21 and 4.22%, respectively.
Table 3. Effects of TW substitution on the milk yield and milk composition.
Ruminal fermentation and blood metabolites
The effects of the experimental diets on the ruminal changes in the pH, VFA production, and NH3–N levels are shown in Table . There were no significant differences (p > 0.05) in the average ruminal pH and VFA production level. Ruminal NH3-N levels in the two TW diets were not significantly different (p > 0.05) from that in the control diet, but it was noticeable that the average ruminal NH3–N level in both TW diets was approximately 4% less than that in the control diet. The average propionic acid productions were significantly different (p < 0.05) between the control group and 10 wt% TW diet group at 4 h after feeding. In addition, the A:P ratio at 4 h post-feeding tended to increase in the TW supplementation groups (p = 0.10).
Table 4. Effects of dietary supplementation with TW on the rumen pH, NH3–N, and VFA at 4 h post-feeding.
The concentrations of blood glucose, BUN, triglyceride, and total protein are given in Table . No significant alteration (p > 0.05) in the blood glucose, triglyceride, and total protein level was found when TW was included in the diet. Increasing the level of TW from 0 to 10 wt% decreased numerically the BUN by 3.30–11.00%, with the lowest BUN level in 10 wt% TW supplementation.
Table 5. Effects of TW substitution on the blood glucose, urea nitrogen, triglyceride, and total protein in crossbred Saanen lactating goats (mean ± SD).
Nitrogen balance, allantoin excretion and methane production
There were no significant differences (p > 0.05) in the nitrogen balance and allantoin excretion (Table ). The 5 wt% TW diet group had the lowest N intake and N absorbed compared to the control and 10 wt% TW groups (p < 0.01). The increase of TW level in the diet had no significant effect (p > 0.05) on CH4 production (Table ), which ranged from 95.50 to 112.40 mmol/L at 4 h post-feeding. The lowest CH4 emission level (84.60–94.40 mmol/L) was observed before morning feeding and it increased rapidly to the highest level at 4 h post-feeding. The average CH4 emission levels in the 0, 5, and 10 wt% TW dietary groups were 91.60, 100.80, and 101.50 mmol/L, respectively.
Table 6. Effects of TW substitution on the nitrogen retention and allantoin excretion.
Table 7. Effects of TW substitution on methane production.
Discussion
The results demonstrated that a diet supplemented with TW at 10 wt% did not affect the DMI. It was previously reported that CT can reduce the feed intake in ruminants by reducing the palatability of the diet, where this deleterious effect of dietary CT is dependent on the CT concentration in the diet. At a concentration of less than 50 g/kg DM, CT was found to be nutritious for ruminants without affecting the feed intake (Min et al. Citation2003), whereas at a moderate CT level (20–40 g/kg DM), it could increase the protein metabolism without any harmful influence on the feed intake (Aerts et al. Citation1999). In contrast, a high level of CT (more than 50 g/kg DM) could decrease the feed intake and protein digestibility (Frutos et al. Citation2004). Therefore, the diets in this study containing low level of CT did not interrupt DMI when compared to the control group without TW supplementation.
Several studies on the utilisation of TW as a replacement protein source have shown similar results to this study. No significant difference (p > 0.05) in the DMI was observed when 5–10 wt% (DM basis) of GTW was included in the diet fed to dairy cattle (Kondo, Nakano, et al. Citation2004; Eruden et al. Citation2005; Theeraphaksirinont et al. Citation2009) and wethers (Xu et al. Citation2007). The substitution of oat silage with GTW silage from 3.30% to 13.20% g/kg DM had no detrimental effect on the DMI in goats (Kondo et al. Citation2004a). In these studies, the dietary CT levels varied from 0.15 to 15.30 g/kg DM, which was broadly comparable to the TW diets in the present study containing CT at 2.50–4.90 g/kg DM. It was lower than the effective dose (50 g/kg DM) that could reduce the DMI. It can be concluded that TW can be given to crossbred Saanen lactating goats at up to 10 wt% of the total diet with no deleterious effect on the DMI.
The digestibility values of DM, OM and CP were not significantly different (p > 0.05) between the control and TW dietary groups. This is in agreement with previous observations in dairy cattle when 5–10 wt% GTW was incorporated in diet (Eruden et al. Citation2003; Theeraphaksirinont et al. Citation2009). The digestibility was not affected by TW supplementation due to the low levels of CT content (2.50–4.90 g/kg DM) in all groups, which had no influence on the feed composition or microbial fermentation. According to McMahon et al. (Citation2000), the condensed tannin concentrations over 40 to 50 g/kg DM in forages might impair the CP and DM digestibility of the forages by ruminants as a result of CT interactions with proteins in feed, saliva, and bacterial cells, with microbial exoenzymes, endogenous proteins or other feed components. Consistent with the previous reports, CT could interfere with nutrient digestibility, according to increased dietary CT levels (151.10 g/kg DM), leading to the reduced digestibility of DM, OM, and N in goats (Animut et al. Citation2008). Generally, GTW contains a lower CT level (16.60–83.50 g/kg DM) than forage (10–220 g/kg DM) (Kondo et al. Citation2004a, Citation2004b, Citation2007; Kondo, Nakano, et al. Citation2004; Nasehi et al. Citation2018; Mueller-Harvey et al. Citation2019). The addition of dietary GTW at 50–100 g/kg DM resulted in no significant differences (p > 0.05) in the OMD and CPD in dairy cattle (Eruden et al. Citation2003; Theeraphaksirinont et al. Citation2009). No differences (p > 0.05) in digestibility were observed in sheep fed with different dietary GTW levels ranging from 2.90 to 6.10 g/kg DM CT nor between sheep fed on the control and 2 wt% TW diets (Nasehi et al. Citation2018). However, nutrient digestibility decreased linearly as the dietary GTW level increased. When goats were fed with dietary GTW at 31.20 and 124.80 g/kg DM (0.60 and 2.80 g/kg DM CT), there were no differences (p > 0.05) in the DMD between the control and treatment diets (Kondo et al. Citation2004a). It should be noted that the nutrient digestibility in ruminants is affiliated with various factors i.e. the ruminal microbial population, microbial activities, limited rumination capacity to disintegrate fibre particle and the flow rate of particles through the gastrointestinal tract (Darlis et al. Citation2012). In addition, the amount of microbial protein synthesised in the gut depending on energy availability and the endogenous losses might give rise to underestimation of protein digestibility (Cantalapiedra-Hijar et al. Citation2009).
Equivalent concentrations of different CT sources were shown to have variable effects on the degradation of CP, due to differences in the molecular weights and chemical structures that influence the biological activity of CT (Min et al. Citation2003). It was postulated from these studies that there were discrepancies in the CT on CPD. It could be partly due to the difference in concentrations, chemical structures, or reactivity of the tannin present in the diet. More information is needed on how the tannins in other feedstuff sources, apart from forages, may affect the nutrient digestibility, especially in terms of the CPD.
The NDFD of highest CT group in this study was different from that of the control group possibly due to highest NDF percentage in feed composition. According to ADF content in feed analysis, both TW diet groups contained higher ADF percentage than the control group, resulting in enhanced ADF digestibility.
There were no differences (p > 0.05) in milk yield and milk composition in lactating Saanen goats fed 0, 5, or 10 wt% DM TW, except for the milk protein percentage that was significantly increased (p < 0.05) in the 10 wt% TW group and lactose percentage which was highest in the control group (p < 0.01). This is inconsistent with other studies, where the addition of GTW at 100 g/kg DM in the diet fed to dairy cattle had no influence on the milk protein percentage (Theeraphaksirinont et al. Citation2009). The higher milk protein percentage in this study might be caused by the tannin in the diet, especially in the 10 wt% TW diet group, relating to increased N intake and N absorption. The effects of dietary tannin on the milk protein percentage have been reported to be variable with no significant differences (p > 0.05) between diets containing tannin that were fed to sheep, dairy cattle, and goats (Toral et al. Citation2011; Liu et al. Citation2013; Mokhtarpour et al. Citation2017). However, in some studies, dietary tannin had an influence on the milk protein content (Mokhtarpour et al. Citation2016; Abo-Donia et al. Citation2017). The milk protein percentage was increased in dairy cows fed with tannin at 4.50 g/kg DM, while a higher level of tannin did not affect the milk protein concentration (Aguerre et al. Citation2016). The reason for this is debateable, although the tannin could potentially increase the milk protein content (Abo-Donia et al. Citation2017). In this study, 10 wt% TW containing CT at 4.90 g/kg DM in the diet was found to improve the milk protein content in these crossbred Saanen lactating goats. This study, the lactose contents were different (p < 0.01) between the TW supplementation groups and the control group. Lactose synthesis required two precursors including glucose and UDP-galactose (Mardones and Villagrán Citation2020). The primary source of glucose in ruminants is gluconeogenesis, which utilises propionic acid as a precursor. In this study, the 10 wt% TW diet group had lower propionate than the control group (p < 0.05). In contrast, the cassava silage with variated condensed tannin levels (0, 25, 50, 75 g/kg DM) in dairy goat diet did not affect lactose concentration. Thus, the concentration of condensed tannin was not directly involved in lactose component in milk (Nascimento et al. Citation2021).
The lower of propionic acid at 4 h post-feeding showed in 10 wt% TW group compared to the control and 5 wt% TW groups. The study of different molecular weights of CT in the in vitro study found that the high molecular weight of CT suppressed total volatile fatty acid especially acetic acid (Saminathan et al. Citation2015). This study found higher average acetic acid concentration when compared to the control group. In addition, the CT (0.10–0.25 g/kg DM) with four different feed types in vitro gas production showed the decreased in short chain fatty acids compared to no CT addition. However, this study did not evaluate the condensed tannin molecular weight in tea waste, then the results of volatile fatty acid may be altered by different factions of CT molecular weight.
No significant differences (p > 0.05) in the blood glucose, triglyceride, and total protein levels were found among the three experimental diets (0, 5, and 10 wt% TW), which is in agreement with previous studies in dairy cattle (Kondo, Nakano, et al. Citation2004; Eruden et al. Citation2005) and wethers (Xu et al. Citation2007). However, the BUN values in those studies were variable. When GTW was included at 5 wt% (DM basis), no difference (p > 0.05) in the BUN levels in animals was found compared to a control diet (Kondo, Nakano, et al. Citation2004; Eruden et al. Citation2005; Xu et al. Citation2008). In contrast, when GTW was included at 10 wt% DM basis, the BUN level in the animals decreased significantly (p < 0.05) compared to the control (0 wt% GTW) diet (Eruden et al. Citation2005; Xu et al. Citation2008). Dairy cows fed with CT at 3.50 g/kg DM had a suppressed BUN concentration (Min et al. Citation2015). The BUN level reflects a high protein degradation with the formation of excess ruminal NH3-N, which then enters the blood circulation and is converted to urea in the liver (Loor et al. Citation2002).
In the present study, the TW diets providing CT at 2.50–4.90 g/kg DM may have had some effect on the protein stability by reducing protein degradability. Although goats fed experimental diets in this study did not show any significant difference (p > 0.05) in the BUN level, it is likely that the TW diet decreased the ruminal NH3-N level, leading to the numerically decreased BUN.
The N intake and N absorbed were the lowest in 5 wt% TW group (p < 0.01) related to the dry matter intake result. The N excretion level is related to the level of N intake. Regardless of the type of the diet, N retention was found to be positive in all experimental diets when ruminants consumed a large amount of N (Patra Citation2010). The N retention was higher in dairy cattle fed a high protein level diet than a low protein level diet (Mulligan et al. Citation2004). Ingested N at between 60% and 90% was eliminated through faeces and urine, depending on the N intake and production levels (Flachowsky and Lebzien Citation2006). However, tannin plays a role in nitrogen retention. A decline in urinary excretion was observed in sheep and cattle when a high level of tannin was included in the diet (Deaville et al. Citation2010; Ahnert et al. Citation2015). Lactating goats fed diets containing higher levels of tannin consumed the same amount of N but showed a reduced urinary N excretion, same amount of milk N, and an increased faecal N level. This resulted in a higher N retention in goats fed with a higher tannin diet (Mokhtarpour et al. Citation2017). When fed with different levels of GTW (20–60 g/kg DM of diet), sheep consumed the same amount of N intake, but a lower nitrogen retention was found at higher dietary levels of GTW (Nasehi et al. Citation2018).
The present study revealed no significant effect (p > 0.05) of a TW diet on the N retention in crossbred lactating goats. However, the N retention in goats fed the 10 wt% TW diet was slightly higher than that in the 0 and 5 wt% TW diets, and resulted from the low level of urinary N excretion and slightly increased faecal N excretion. A low tannin concentration had some benefit by binding protein, which can then escape degradation in the rumen, reducing deamination in the rumen and in turn supplying protein to be absorbed in the intestine (McSweeney et al. Citation2001). The escaped protein would increase the faecal N and reduce the urinary N levels (Soltan et al. Citation2013). It is likely that dietary TW inclusion at 10 g/kg DM might improve the N retention in crossbred lactating goats. Allantoin is a by-product from the degradation of purines and is excreted in the urine. It is quantitatively related to the amount of microbial protein (Chen et al. Citation1990), and has been proposed as a good estimator of the rumen microbial protein level supplied to host animals (Topps and Elliott Citation1965). There was no effect of the dietary treatments on allantoin excretion in this study, which is in agreement with a previous study in dairy cattle (Kondo, Nakano, et al. Citation2004). In addition, the supplementation of rain tea pot meal provided a CT of 5.30 g/kg DM but no significant difference (p > 0.05) in allantoin excretion was found between the control and supplemented groups (Anantasook et al. Citation2013).
The ruminal pH, and concentrations of total VFA, acetic acid, butyric acid, and the acetic: propionic acid ratio were not significantly affected (p > 0.05) by TW replacement. It was previously found that ruminal fermentation was not changed by GTW addition at a low (5 wt%), medium (10 wt%), and high (15 wt%) level (on a DM basis) in the diet fed to wethers (Xu et al. Citation2008). This was also found in dairy cattle fed with GTW diets at 5–10 wt% DM basis (Kondo, Nakano, et al. Citation2004; Theeraphaksirinont et al. Citation2009). The ruminal NH3–N was slightly decreased in goats fed with the TW diets, and was the lowest in goats fed with the 10 wt% TW diet at 4 h post-feeding. This was probably due to the lower degradability of the protein content in the TW diet than that in the control diet. Previous research has reported that tannin levels of 1–5 wt% decreased the protein degradability (Salawu et al. Citation1999; Santos et al. Citation2000). However, goats fed with a GTW diet consisting of 0.90–3.10 g/kg DM CT showed a significantly (p < 0.05) decreased ruminal NH3-N (Kondo et al. Citation2004a). In this study, the level of tannin in the TW diets ranged from 0.25 wt% to 0.49 wt%, similar to that in the previous studies in goats. These tannin levels might make a complex with the feed protein, resulting in a lower degradability of the dietary protein in the rumen.
Several studies have proposed that dietary CT is a means to mitigate CH4 emission from ruminal fermentation (Carulla et al. Citation2005; Bhatta et al. Citation2009; Anantasook et al. Citation2016; Gunun et al. Citation2017). However, the efficiency of CT to reduce CH4 emission depends on the level of CT in the diet fed to the animals. Meta-analysis demonstrated that there was a relationship between the dietary concentration of CT and fermentable CH4 (Jayanegara et al. Citation2012). The effect of dietary CT on CH4 emission in goats was reported to be dependent on the level of CT in the diet. When goats were fed with dietary CT at 5 g/kg DM, the CH4 emission was 45% higher than those fed with dietary CT at 177 g/kg DM (Puchala et al. Citation2005). In addition, goats fed with dietary CT at 50 g/kg DM had a 47% decreased CH4 emission level, comparable to those fed with dietary CT at 3 g/kg DM (Animut et al. Citation2008).
In an in vitro study, TW dietary treatments containing three different levels of CT (range of 2.90–6.10 g/kg DM) decreased the level of CH4 emission (Nasehi et al. Citation2018). In this study, the highest level of dietary CT was in the 10 wt% TW diet, which was equivalent to CT at 4.90 g/kg DM, and did not significantly (p > 0.05) affect the level of CH4 emission. The higher response of TW diets in studies with a similar CT level as in this study, on the CH4 emission level in lambs might be caused by the additive effect of a high level of dietary saponin (range 6.10–11.30 g/kg DM). A number of studies have reported that dietary saponins or saponin-rich plants can reduce CH4 production (Pen et al. Citation2007; Holtshausen et al. Citation2009; Jadhav et al. Citation2018), and the inclusion of saponins at 15 g/kg DM reduced the CH4 emission (Holtshausen et al. Citation2009). Saponin extracted from tea seed showed a beneficial effect on CH4 emission in an in vitro study. Moreover, the addition of tea saponin extract at 10–40 g/kg DM significantly decreased (p < 0.05) CH4 emission (Hu et al. Citation2005). However, no data on the saponin types and concentrations in these TW diets are available in this study. It is possible that the absence of a CH4 emission response to the TW diets in this study might be caused by their low CT and saponin contents.
Conclusions
There was no influence of the substitution of tea waste as a source of dietary protein on the dry matter intake, dry matter intake per %body weight, milk yield, and milk composition in crossbred Saanen lactating goats, except that the 10 weight% tea waste diet increased the milk protein percentage but both tea waste diets decreased lactose percentage. However, the total milk protein yield was not different. Goats fed with the 5 and 10 weight% tea waste diets tended to reduce crude protein digestibility compared with those in the control diet. The addition of dietary tea waste had some benefit on nitrogen retention, but did not affect the methane emission compared to the control diet. Therefore, tea waste can be used to replace other dietary protein sources without any deleterious effect on the performance and physiological condition of crossbred Saanen lactating goats.
Acknowledgment
The authors greatly appreciate Prof. Somchai Chanpongsang for his advice and fruitful comments.
Disclosure statement
No potential conflict of interest was reported by the author(s).
Data availability statement
The data that support the findings of this study are available from the corresponding author, upon reasonable request.
Additional information
Funding
References
- [AOAC] Association of Official Analytical Chemists. 1995. Official methods of analysis of the AOAC (16th ed.). Arlington (VA): AOAC International.
- [NRC] National Research Council. 1981. Nutrient requirements of goats: angora, dairy, and meat goats in temperate and tropical countries. Washington (DC): The National Academies Press.
- Abo-Donia FM, Yang LY, Hristov AN, Wang M, Tang SX, Zhou CS, Han XF, Kang JH, Tan ZL, He ZX. 2017. Effects of tannins on the fatty acid profiles of rumen fluids and milk from lactating goats fed a total mixed ration containing rapeseed oil. Livest Sci. 204:16–24.
- Aerts RJ, Barry TN, McNabb WC. 1999. Polyphenols and agriculture: beneficial effects of proanthocyanidins in forages. Agric Ecosyst Environ. 75(1–2):1–12.
- Aguerre MJ, Capozzolo MC, Lencioni P, Cabral C, Wattiaux MA. 2016. Effect of quebracho-chestnut tannin extracts at 2 dietary crude protein levels on performance, rumen fermentation, and nitrogen partitioning in dairy cows. J Dairy Sci. 99(6):4476–4486.
- Ahnert S, Dickhoefer U, Schulz F, Susenbeth A. 2015. Influence of ruminal Quebracho tannin extract infusion on apparent nutrient digestibility, nitrogen balance, and urinary purine derivatives excretion in heifers. Livest Sci. 177:63–70.
- Anantasook N, Wanapat M, Cherdthong A, Gunun P. 2013. Effect of plants containing secondary compounds with palm oil on feed intake, digestibility, microbial protein synthesis and microbial population in dairy cows. Asian-Australas J Anim Sci. 26(6):820–826.
- Anantasook N, Wanapat M, Gunun P, Cherdthong A. 2016. Reducing methane production by supplementation of Terminalia chebula RETZ. containing tannins and saponins. Anim Sci J. 87(6):783–790.
- Animut G, Puchala R, Goetsch AL, Patra AK, Sahlu T, Varel VH, Wells J. 2008. Methane emission by goats consuming diets with different levels of condensed tannins from lespedeza. Anim Feed Sci Technol. 144(3–4):212–227.
- Bhatta R, Uyeno Y, Tajima K, Takenaka A, Yabumoto Y, Nonaka I, Enishi O, Kurihara M. 2009. Difference in the nature of tannins on in vitro ruminal methane and volatile fatty acid production and on methanogenic archaea and protozoal populations. J Dairy Sci. 92(11):5512–5522.
- Cantalapiedra-Hijar G, Yáñez-Ruiz DR, Martín-García AI, Molina-Alcaide E. 2009. Effects of forage:concentrate ratio and forage type on apparent digestibility, ruminal fermentation, and microbial growth in goats. J Anim Sci. 87(2):622–631.
- Carulla JE, Kreuzer M, Machmüller A, Hess HD. 2005. Supplementation of Acacia mearnsii tannins decreases methanogenesis and urinary nitrogen in forage-fed sheep. Aust J Agric Res. 56(9):961–970.
- Chen XB, Hovell FDD, ØRskov ER. 1990. Excretion of purine derivatives by ruminants: recycling of allantoin into the rumen via saliva and its fate in the gut. Br J Nutr. 63(2):197–205.
- Darlis NA, Liang JB, Ho YW. 2012. Effects of diets of differing fiber contents on digestibility, passage rate of digesta and heat production in lesser mouse deer (Tragulus javanicus). Mammal Biol. 77(6):385–390.
- Deaville ER, Givens DI, Mueller-Harvey I. 2010. Chestnut and mimosa tannin silages: effects in sheep differ for apparent digestibility, nitrogen utilisation and losses. Anim Feed Sci Technol. 157(3–4):129–138.
- Eruden B, Nishida T, Hosoda K, Shioya S, Cai Y. 2003. Effects of green tea grounds silage on digestibility, rumen fermentation, and blood components in lactating dairy cows. Nihon Chikusan Gakkaiho. 74(4):483–490.
- Eruden B, Nishida T, Matsuyama H, Hosoda K, Shioya S. 2005. Effect of the addition of various levels of green tea grounds silage on the feed intake and milk production in lactating cow. Nihon Chikusan Gakkaiho. 76(3):295–301.
- Flachowsky G, Lebzien P. 2006. Possibilities for reduction of Nitrogen (N) excretion from ruminants and the need for further research—a review. Landbauforschung Volkenrode. 56:19–30.
- Frutos P, Hervás G, Giráldez FJ, Mantecón AR. 2004. Tannins and ruminant nutrition review. Span J Agric Res. 2(2):191–202.
- Gunun N, Gunun P, Wanapat M, Cherdthong A, Kang S, Polyorach S. 2017. Improving the quality of sugarcane bagasse by urea and calcium hydroxide on gas production, degradability and rumen fermentation characteristics. J Anim Plant Sci. 27:1758.
- Holtshausen L, Chaves AV, Beauchemin KA, McGinn SM, McAllister TA, Odongo NE, Cheeke PR, Benchaar C. 2009. Feeding saponin-containing Yucca schidigera and Quillaja saponaria to decrease enteric methane production in dairy cows1. J Dairy Sci. 92(6):2809–2821.
- Hu WL, Liu JX, Ye JA, Wu YM, Guo YQ. 2005. Effect of tea saponin on rumen fermentation in vitro. Anim Feed Sci Technol. 120(3–4):333–339.
- Jadhav RV, Kannan A, Bhar R, Sharma OP, Gulati A, Rajkumar K, Mal G, Singh B, Verma MR. 2018. Effect of tea (Camellia sinensis) seed saponins on in vitro rumen fermentation, methane production and true digestibility at different forage to concentrate ratios. J Appl Anim Res. 46(1):118–124.
- Jayanegara A, Leiber F, Kreuzer M. 2012. Meta-analysis of the relationship between dietary tannin level and methane formation in ruminants from in vivo and in vitro experiments. J Anim Physiol Anim Nutr (Berl). 96(3):365–375.
- Kondo M, Hidaka M, Kita K, Yokota H. 2007. Ensiled green tea and black tea waste as protein supplement for goats. In: Priolo A, Biondi L, Ben Salem H, Morand-Fehr P, editors. Advanced nutrition and feeding strategies to improve sheep and goat. Options Méditerranéennes Series A. Zaragoza: CIHEAM; p. 165–169.
- Kondo M, Kita K, Yokota H-O. 2004a. Feeding value to goats of whole-crop oat ensiled with green tea waste. Anim Feed Sci Technol. 113(1–4):71–81.
- Kondo M, Kita K, Yokota H-O. 2004b. Effects of tea leaf waste of green tea, oolong tea, and black tea addition on sudangrass silage quality and in vitro gas production. J Sci Food Agric. 84(7):721–727.
- Kondo M, Nakano M, Kaneko A, Agata H, Kita K, Yokota H-O. 2004. Ensiled green tea waste as partial replacement for soybean meal and alfalfa hay in lactating cows. Asian Australas J Anim Sci. 17(7):960–966.
- Liu HW, Zhou DW, Li K. 2013. Effects of chestnut tannins on performance and antioxidative status of transition dairy cows. J Dairy Sci. 96(9):5901–5907.
- Loor JJ, Herbein JH, Jenkins TC. 2002. Nutrient digestion, biohydrogenation, and fatty acid profiles in blood plasma and milk fat from lactating Holstein cows fed canola oil or canolamide. Anim Feed Sci Technol. 97(1–2):65–82.
- Mardones L, Villagrán M. 2020. Lactose synthesis. In: Gutiérrez-Méndez N, editor. Lactose and lactose derivatives. London: IntechOpen.
- McMahon LR, McAllister TA, Berg BP, Majak W, Acharya SN, Popp JD, Coulman BE, Wang Y, Cheng KJ. 2000. A review of the effects of forage condensed tannins on ruminal fermentation and bloat in grazing cattle. Can J Plant Sci. 80(3):469–485.
- McSweeney CS, Palmer B, McNeill DM, Krause DO. 2001. Microbial interactions with tannins: nutritional consequences for ruminants. Anim Feed Sci Technol. 91(1–2):83–93.
- Min BR, Barry TN, Attwood GT, McNabb WC. 2003. The effect of condensed tannins on the nutrition and health of ruminants fed fresh temperate forages: a review. Anim Feed Sci Technol. 106(1–4):3–19.
- Min B, Hernández K, Pinchak W, Anderson R, Miller J, Valencia E. 2015. Effects of plant tannin extracts supplementation on animal performance and gastrointestinal parasites infestation in steers grazing winter wheat. OJAS. 05(03):343–350.
- Mokhtarpour A, Naserian AA, Pourmollae F, Ghaffari MH. 2016. Effect of treating alfalfa silage with pistachio by-products extract on Saanen dairy goats performance and microbial nitrogen synthesis. J Anim Physiol Anim Nutr (Berl). 100(4):758–767.
- Mokhtarpour A, Naserian A, Pourmollae F, Safa S. 2017. Effects of two sources of tannins on performance, nitrogen utilization and efficiency of microbial nitrogen synthesis in dairy goats. Iran J Appl Anim Sci. 7:61–68.
- Moss AR, Jouany JP, Newbold J. 2000. Methane production by ruminants: its contribution to global warming. Ann Zootech. 49(3):231–253.
- Mueller-Harvey I, Bee G, Dohme-Meier F, Hoste H, Karonen M, Kölliker R, Lüscher A, Niderkorn V, Pellikaan WF, Salminen J-P, et al. 2019. Benefits of condensed tannins in forage legumes fed to ruminants: importance of structure, concentration, and diet composition. Crop Sci. 59(3):861–885.
- Mulligan FJ, Dillon P, Callan JJ, Rath M, O’Mara FP. 2004. Supplementary concentrate type affects nitrogen excretion of grazing dairy cows. J Dairy Sci. 87(10):3451–3460.
- Nascimento TVC, Oliveira RL, Menezes DR, de Lucena ARF, Queiroz MAÁ, Lima AGVO, Ribeiro RDX, Bezerra LR. 2021. Effects of condensed tannin-amended cassava silage blend diets on feeding behavior, digestibility, nitrogen balance, milk yield and milk composition in dairy goats. Animal. 15(1):100015.
- Nasehi M, Torbatinejad NM, Rezaie M, Ghoorchi T. 2018. Effects of partial substitution of alfalfa hay with green tea waste on growth performance and in vitro methane emission of fat-tailed lambs. Small Rumin Res. 168:52–59.
- Patra AK. 2010. Effects of supplementing low-quality roughages with tree foliages on digestibility, nitrogen utilization and rumen characteristics in sheep: a meta-analysis. J Anim Physiol Anim Nutr (Berl). 94(3):338–353.
- Pen B, Takaura K, Yamaguchi S, Asa R, Takahashi J. 2007. Effects of Yucca schidigera and Quillaja saponaria with or without β 1–4 galacto-oligosaccharides on ruminal fermentation, methane production and nitrogen utilization in sheep. Anim Feed Sci Technol. 138(1):75–88.
- Puchala R, Min BR, Goetsch AL, Sahlu T. 2005. The effect of a condensed tannin-containing forage on methane emission by goats1. J Anim Sci. 83(1):182–186.
- Salawu MB, Acamovic T, Stewart CS, Hvelplund T, Weisbjerg MR. 1999. The use of tannins as silage additives: effects on silage composition and mobile bag disappearance of dry matter and protein. Anim Feed Sci Technol. 82(3–4):243–259.
- Saminathan M, Sieo CC, Abdullah N, Wong CMVL, Ho YW. 2015. Effects of condensed tannin fractions of different molecular weights from a Leucaena leucocephala hybrid on in vitro methane production and rumen fermentation. J Sci Food Agric. 95(13):2742–2749.
- Sano H, Takebayashi A, Kodama Y, Nakamura K, Ito H, Arino Y, Fujita T, Takahashi H, Ambo K. 1999. Effects of feed restriction and cold exposure on glucose metabolism in response to feeding and insulin in sheep1. J Anim Sci. 77(9):2564–2573.
- Santos GT, Oliveira RL, Petit HV, Cecato U, Zeoula LM, Rigolon LP, Damasceno JC, Branco AF, Bett V. 2000. Effect of tannic acid on composition and ruminal degradability of Bermudagrass and alfalfa silages. J Dairy Sci. 83(9):2016–2020.
- SAS. 2015. SAS/STAT user’s guide: version 9.4. Cary (NC): SAS Institute Inc.
- Silanikove N, Gilboa N, Nir I, Perevolotsky A, Nitsan Z. 1996. Effect of a daily supplementation of polyethylene glycol on intake and digestion of tannin-containing leaves (Quercus calliprinos, Pistacia lentiscus, and Ceratonia siliqua) by goats. J Agric Food Chem. 44(1):199–205.
- Soltan YA, Morsy AS, Sallam SMA, Lucas RC, Louvandini H, Kreuzer M, Abdalla AL. 2013. Contribution of condensed tannins and mimosine to the methane mitigation caused by feeding Leucaena leucocephala. Arch Anim Nutr. 67(3):169–184.
- Steel RGD, Torrie JH. 1980. Principles and procedures of statistics: a biometrical approach. 2nd ed. New York: McGraw-Hill.
- Theeraphaksirinont T, Chanpongsang S, Chaiyabutr N, Topanurak S. 2009. Effects of green tea waste in total mixed ration on productive performances in cross-bred lactating cows. Proceedings of 47th Kasetsart University Annual Conference: Animals, Kasetsart University, Bangkok, Thailand, 17–21.
- Topps JH, Elliott RC. 1965. Relationship between concentrations of ruminal nucleic acids and excretion of purine derivatives by sheep. Nature. 205(4970):498–499.
- Toral PG, Hervás G, Bichi E, Belenguer Á, Frutos P. 2011. Tannins as feed additives to modulate ruminal biohydrogenation: effects on animal performance, milk fatty acid composition and ruminal fermentation in dairy ewes fed a diet containing sunflower oil. Anim Feed Sci Technol. 164(3–4):199–206.
- Van Soest PJ, Robertson JB, Lewis BA. 1991. Methods for dietary fiber, neutral detergent fiber, and nonstarch polysaccharides in relation to animal nutrition. J Dairy Sci. 74(10):3583–3597.
- Xu C, Cai Y, Moriya N, Eruden B, Hosoda K, Matsuyama H. 2008. Influence of replacing brewers’ grains with green tea grounds on feed intake, digestibility and ruminal fermentation characteristics of wethers. Animal Sci J. 79(2):226–233.
- Xu C, Cai Y, Moriya N, Ogawa M. 2007. Nutritive value for ruminants of green tea grounds as a replacement of brewers’ grains in totally mixed ration silage. Anim Feed Sci Technol. 138(3–4):228–238.
- Yamamoto T, Juneja LR, Kim M. 1997. Chemistry and applications of green tea. Boca Raton (FL): CRC Press.
- Young EG, Conway CF. 1942. On the estimation of allantoin by the Rimini-Schryver reaction. J Biol Chem. 142(2):839–853.