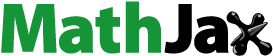
Abstract
This study was conducted to assess the effects of dietary monoglycerides and tributyrin on performance, blood metabolites, and intestinal health function in nursery piglets. A total of 96 crossbred entire male piglets (7.70 ± 0.49 kg) were allotted for 35 d in a complete block design to one of four treatments: (1) negative control (NC): no feed additive, (2) positive control (PC) containing 60 mg halquinol/kg diet, (3) diet containing 2 g monoglyceride blend/kg diet (MGD), and (4) diet containing 2 g tributyrin/kg diet (TBT). The growth phases were defined as pre-starter (day 0–20), and starter (day 20–35). No treatment effect on performance was observed. Pre-starter piglets fed NC showed higher diarrhoea occurrence (DO) than those fed PC and TBT (p < .05), and a lower DO was observed in starter piglets fed PC, MGD, and TBT compared to NC (p < .001). Pre-starter piglets fed TBT showed lower alkaline phosphatase concentration than in PC, and a lower aspartate aminotransferase concentration compared to PC and MGD (p < .05). Plasma urea concentration was higher in starter piglets fed MGD than in NC and TBT (p < .05). Piglets fed TBT showed higher jejunum villous height:crypt depth ratio than other treatments (p < .05). Piglets on MGD had higher ileum cellular infiltrate than in PC but lower Enterobacteriaceae family in the piglets jejunum compared to other treatments (p < .05). Our results suggest that dietary tributyrin improves intestinal function structures, without affecting the microbial population as exhibited in nursery piglets fed monoglyceride-based diets.
The use of antimicrobial growth promoters has been widely banned in piglet diets.
The feed additives tested appeared to be potential alternatives to antimicrobial growth promoters.
Piglets fed a tributyrin diet showed improvements in the structure of the intestinal epithelium.
Diets containing a blend of monoglycerides reduced the abundance of Enterobacteriaceae in nursery piglets.
HIGHLIGHTS
Introduction
The gastrointestinal tract (GIT) of piglets is dramatically altered post-weaning (Genova et al. Citation2020), undergoing marked growth, high protein turnover, and intensive intestinal changes that affect the digestive and the immune system of piglets (Pluske Citation2016). Consequently, piglets post-weaning development and growth performance are affected by changes in GIT biometry and intestinal histology (Pluske et al. Citation2018), and imbalances in GIT microbial population that compromise intestinal maturation (Genova et al. Citation2022).
In this sense, nutritional and/or feeding strategies for nursery piglets should reduce post-weaning stress effects (Campbell et al. Citation2013). Driven by the European Union policies on antimicrobial growth promoters (AGP) used in pig production, several countries have also banned these substances from animal production. Thus, many companies and researchers have searched for alternative ingredients or solutions to replace AGP in pig production (Berto et al. Citation2020; Nguyen et al. Citation2020).
Therefore, functional ingredients that do not reduce growth performance and comply with feed quality and safety regulations have been studied, improving intestinal health function (Liu et al. Citation2018; Modina et al. Citation2019). Monoglycerides derived from medium-chain fatty acids (e.g. monolaurin or glycerol monolaurate from lauric acid) have also been studied (Anacarso et al. Citation2018; Jackman et al. Citation2020). In addition, tributyrin is a compound produced via esterification, in which three butyric acids molecules are attached to glycerol that can improve piglets performance (Sotira et al. Citation2020; Zhang et al. Citation2020). Indeed, tributyrin have been reported to increase colon mucosa cell turnover to improve intestinal trophic state in nursery pigs (Sakdee et al. Citation2016), antibacterial activity against different bacteria (e.g. Escherichia coli and Salmonella) in weaned piglets fed tributyrin and essential oil (Zhang et al. Citation2020) or monoglycerides (Jackman et al. Citation2020), and modulation of blood metabolites (e.g. glucose and urea) in weaned piglets fed tributyrin (Sotira et al. Citation2020).
However, few studies have been conducted to assess the effects of dietary monoglycerides or tributyrin on the ability to reduce histopathological damage, stimulation of digestive-immune function through changes in organ weights (e.g. weight of liver and spleen), and bactericidal-bacteriostatic effect on pathogenic bacteria such as Clostridium sulfite-reducer in nursery piglets. Here, we hypothesised that dietary monoglycerides and tributyrin would improve intestinal health and promote optimal growth performance in weaned piglets, with the potential as complementary additives to replace AGP. Therefore, this study was conducted to assess the effects of dietary monoglycerides and tributyrin on growth performance, blood metabolites, and intestinal health in nursery piglets.
Materials and methods
The animal procedures were approved by the Ethics Committee on the Use of Animals in Experimentation at the Universidade Estadual do Oeste do Paraná (Unioeste) (protocol no. 02/2022). The study was conducted in an experimental farm belonging to Unioeste.
Experimental design, animals, housing, and diets
A total of 96 crossbred entire male piglets (7.70 ± 0.49 kg and weaned at 25 d old) from a commercial lines (Landrace × Large White) were allotted to one of four dietary treatments in a completely block design based on the initial body weight (BW) categorised into light (up to 6.8 kg BW) or heavy (over 6.8 kg BW) piglets. Pigs were allotted to four treatments, in which had eight replicates pens (n = 4 replicates pens per block) of three piglets of the same BW category per pen as experimental unit. The blocks (n = 2) consisted of 16 pens (experimental unit) and 48 piglets in each block, totalling 32 pens.
Dietary treatments were as follows: (1) negative control (NC): diet without feed additive or AGP, (2) positive control (PC): a diet containing 60 mg halquinol (Farmabase company, Jaguariúna, SP, Brazil)/kg diet, (3) diet containing 2 g monoglyceride blend/kg diet (MGD), and (4) diet containing 2 g tributyrin/kg diet (TBT) (Table ). The dosage of MGD and TBT was based on the manufacturer’s recommendations. The monoglyceride blend contained 33% of monolaurate and 41.3% of C8 and C10 monoglycerides (Sauvet Company, Campinas, SP, Brazil). The tributyrin contained 55.8% tributyrin and silica as an excipient (Sauvet company, Campinas, SP, Brazil).
Table 1. Composition of dietary treatments offered to nursery piglets (as-fed)a.
All diets were corn and soybean meal-based with industrial amino acids, and mixed for 15 min in a vertical mixer. Diets were formulated to meet nutritional requirements for nursery piglets according to Rostagno et al. (Citation2017) (Table ) and offered as mash.
Table 2. Calculated and analysed chemical composition of diets offered to nursery piglets (as-fed).
At the beginning of the experiment, animals were weighed and identified with numbered ear tags. Piglets were housed in a nursery room with two rows (with a central aisle) of slatted floor pens (1.5 m2) equipped with front gutter feeders and back nipple drinkers. The experiment lasted 35 d, performed only in one series, without an adaptation period. Animals were fed for ad libitum intake and had free access to water throughout the experiment.
Room temperature and relative humidity were recorded by a data logger (UT330B digital USB; UNI-T,Vketech, Beijing, China). The nursery room ventilation was provided with the aid of tilt-and-turn glass windows. The heating of the experimental pens was controlled using one heat lamp in each pen and side heaters. A combination of daylight and artificial light was used, with a 12-h light/dark cycle. Room temperature and relative humidity in the middle of nursery room averaged 26.7 °C and 67.5%, respectively.
Diet analysis
The chemical composition of diets samples (≅200 g) was analysed as described by AOAC (Citation2019). Then, samples were pooled by dietary treatment, and dried in a forced-air oven (Tecnal brand, SF-325 NM model; Piracicaba, SP, Brazil) at 55 °C for 72 h. All samples were ground through a 1 mm screen in a Wiley mill, and stored in identified plastic containers. Dry matter was determined according to method no. 930.15. The diets were analysed for crude protein by the Kjeldahl procedure following method no. 984.13. The ether extract was determined according to method no. 920.39. Neutral detergent fibre, acid detergent fibre, and crude fibre were determined following methods no. 2002:04, 973.18, and 978.10, respectively. The gross energy was determined in the animal nutrition laboratory using a bomb calorimeter (IKA®, model C200, Wilmington, North Carolina, USA).
Growth performance and diarrhoea occurrence
Animals were weighed on days 0, 20, and 35 of the experimental period with a digital scale (Rinnert digital scale, model BPW-5000; Braço do Trombudo, SC, Brazil). Offered diet and leftovers were monitored daily throughout the experiment. The average daily feed intake (ADFI, kg), the average daily BW gain (ADG, kg), and feed-to-gain ratio (F:G, kg:kg) were calculated. The experimental growth phases were set as follows: (1) pre-starter (day 0–20) and (2) starter (day 20–35), and the piglets were fed different dietary regimen in each phase (Rostagno et al. Citation2017).
Diarrhoea occurrence (DO) was recorded daily (09:00 am) during the cleaning of each experimental unit. Faeces were scored on a 4-point scale as follows: 0 = normal faeces; 1 = soft faeces; 2 = watery/pasty faeces, and 3 = liquid faeces (Pérez-Calvo et al. Citation2019). Scores 2 and 3 were considered diarrhoeal faeces. Diarrhoea was measured at pen level and the DO was considered when it was observed 2 consecutive days with a faecal score of 2 or 3.
Blood sampling and analyses
On day 0, all piglets were submitted to blood collection to monitor their initial blood profile prior to feeding to the dietary treatments. All piglets fasted for 8 h; however, 64 piglets (16 piglets/treatment, 2 piglets/pen, and 8 piglets/block) were chosen at the end of each phase for blood collection based on the nearest BW to the average BW in each dietary treatment on day 20. The same animals selected at the end of the pre-starter phase were sampled at the end of the starter phase. Blood samples (≅10 mL, 08:00 am) were withdrawn from the anterior cranial vena cava using 0.7 × 30 mm needles. After sampling, blood samples were transferred to four different sterile glass tubes, each one containing one type of anticoagulant (heparin, EDTA, or potassium fluoride) or stored for serum. All samples were transported to the lab in a thermal box (4°C). Then, plasma or serum were isolated by centrifugation (Centrilab centrifuge, model 80-2B, Maringá, Brazil) at 3000 g for 10 min at room temperature.
Plasma was transferred (≅3 mL) to identified eppendorf tubes (duplicates) and frozen at −20 °C for further analyses of glucose (enzymatic-colorimetric method, Trinder), urea (enzymatic-colorimetric method), total protein (colorimetric-biurete method), cholesterol (colorimetric-enzymatic method), alkaline phosphatase (colorimetric-kinetic method), alanine aminotransferase (kinetic-UV method), aspartate aminotransferase (kinetic-UV method), and albumin (enzymatic-colorimetric method), using Gold Analisa Diagnostic kits (Belo Horizonte, MG, Brazil). Analyses were performed via spectrophotometry (Bel SPECTRO S05, Ramos, RJ, Brazil) in technical duplicate.
Slaughter procedures and pH of gastrointestinal tract contents
On day 36, 6 piglets/treatment (1 piglet/pen and 3 piglets/block) were chosen based on the BW closest to the average weight of their respective dietary treatment on day 35. The animals were not the same blood donors. Animals were fasted for 8 h and electrically stunned (240 volts for 3 s), followed by exsanguination. The viscera were exposed by a central incision. The sections of the GIT were aseptically isolated with double ligation and pH was measured in the homogenised content of the medial region of the stomach, jejunum (150 cm from the ileocaecal junction), ileum (15 cm from the ileocaecal junction), caecum, and medial colon using a digital pHmeter (HI 99163, Hanna Instruments Inc., Rhodes Island, USA) as previously described by Manzanilla et al. (Citation2006).
Relative organ weight
After measuring pH, digestive organs (empty stomach, liver with gallbladder, empty small intestine, caecum, and colon) and non-digestive organs (heart, spleen, and kidneys) were weighed (digital scale, model 9094, Toledo, Brazil) on day 36 (6 piglets/treatment, 1 piglet/pen and 3 piglets/block). The length of the small intestine was measured using a measuring tape. Relative organ weight (ROW) to slaughter was then calculated as follows (Genova et al. Citation2021):
Intestinal morphometry and histopathology
Segments (≅3 cm length) of jejunum and ileum were taken on day 36 (6 piglets/treatment, 1 piglet/pen and 3 piglets/block) as previously described by Guo et al. (Citation2001). Fragments collected were washed with distilled water and saline (0.9% sodium chloride) and then stored in 50 mL sterile plastic containers with a prepared solution of 10% neutral buffered formalin (100 mL 37.5% formaldehyde, 900 mL distilled water, 4 g monobasic sodium phosphate, and 6.5 g dibasic sodium phosphate) for 48 h (Genova et al. Citation2021). Afterward, samples were placed in a 70% alcohol solution and then sent to a commercial lab (Mercolab, Cascavel, PR, Brazil). Samples were washed in saline solution, fixed in Bouin’s solution, dehydrated in a graded series of ethanol, and cleared with xylol. Tissues were embedded in paraffin wax to prepare slides. Five-micrometer sections were cut from the paraffin blocks using a rotary microtome for subsequent staining with haematoxylin and eosin.
Slides images were captured by a digital camera (Pro Series, Silver Spring, MD, USA) coupled with an Olympus Bx 40 microscope (Olympus, Tokyo, Japan). The histopathological and morphometric exam of images were performed using imaging software (Image-Pro plus 4.1, Media Cybernetics®, Silver Spring, Maryland). Two intestine fragments per animal were separated for intestine histopathology analysis. A total of 15 villous height (VH) and their crypt depth (CD) were measured (Genova et al. Citation2021). Samples were analysed using an optical microscope (Olympus, Tokio, Japan) at 4× magnification. The presence of infiltrate, congestion, desquamation, coccidiosis, bacterial lumps, rods, cystic crypts, mucus, tissue necrosis and oedema were recorded as previously described by Kraieski et al. (Citation2017). Histopathology description was scored as 0 = absent, 1 = mild, 2 = moderate, and 3 = intense (Genova et al. Citation2022).
Intestinal microbial population
Samples of jejunum content were collected for isolation on day 36 using the classic plating technique. Counting and characterisation of the microbial population were performed with culture plates. After evisceration, the jejunum mucosa was exposed using a scalpel. Jejunum contents were placed in sterile plastic containers in a thermal box (4°C) and then transported to a commercial laboratory (Cascavel, PR, Brazil). Then, at the commercial laboratory, 1 g of each sample was serially diluted in 1% peptone water. Each dilution was vortexed (Phoenix brand, AP 56 model; Araraquara, SP, Brazil) for 30 s. A 100 μL aliquot of each diluted sample was spread evenly on the surface of the plates containing bacteria-specific agar. Samples were analysed according to procedures of ISO 16649-2:2001 (ISO Citation2006). The microbial populations assessed were Clostridium sulfite-reducer (tryptose-sulfite-cycloserine agar, neogen brand; São Paulo, SP, Brazil), Enterobacteriaceae family (eosin methylene blue levine agar, Kasvi brand; São José dos Pinhais, PR, Brazil), and lactic acid bacteria (de Man, Rogosa, and Sharpe agar, Acumedia brand; São Paulo, SP, Brazil) (Genova et al. Citation2022). Plates were incubated at 37 °C (EL 202; Eletrolab, São Paulo, SP, Brazil) aerobically for 24 h for Enterobacteriaceae counting, and anaerobically for 48 h for Clostridium sulfite-reducer and lactic acid bacteria counting.
Statistical procedures
Statistical analyses were performed using the MEANS, GLM, UNIVARIATE, GENMOD, FREQ procedures of SAS University Edition software (SAS Inst. Inc., Cary, NC, USA). Prior to variance (ANOVA) or covariance (ANCOVA) analysis, data were evaluated for outliers. The pen was considered the experimental unit, except for slaughtering data. Data were analysed considering treatment and BW block as fixed and random effects, respectively. The initial BW and pre-trial blood variables (baseline sampled on day 0) were included in the model as covariates (p < .05). All other data were analysed according to the model previously described with no covariates. Data on bacteria counts were log10 colony-forming unit (CFU)/g transformed to be analysed. Treatment effect on the dependent variables were evaluated via ANOVA or ANCOVA. The Tukey’s post hoc test was used to compare treatment averages. Significant differences were set at p < .05. All normally distributed data were reported as averages and pooled standard error of the mean.
A generalised linear model was fitted for DO (binomial distribution and logit link function) and intestinal histopathology. The results of DO were presented as observed proportions (relative frequency in %). Treatment and time were considered as fixed effects and BW block as a random factor. The treatment effect was verified via type III analysis. The Akaike information criteria was used to test the model fitting for the intestinal histopathology. Significant differences were set at p < .05, and estimates for DO and intestinal histopathology were compared using a test of the difference between the lsmeans, through the χ2 statistic.
Results
Growth performance and diarrhoea occurrence
No effects of dietary treatments on growth performance were observed (Table ). However, pre-starter piglets fed the NC diet showed a higher DO than those fed PC and TBT diets (p < .05) (Table ), and a lower DO was observed in starter piglets fed PC, MGD, and TBT diets than in those fed NC diet (p < .001).
Table 3. Growth performance and diarrhoea occurrence (DO) in nursery piglets fed monoglycerides blend and tributyrinTable Footnoted.
Blood metabolites
Pre-starter piglets fed the TBT diet showed lower concentration of alkaline phosphatase than those fed the PC diet (p < .05) (Table ). Pre-starter piglets fed the TBT diet showed lower aspartate aminotransferase concentration than those fed PC and MGD diets (p < .05). In addition, the plasma urea concentration was higher in starter piglets fed the MGD diet than in those fed NC and TBT diets (p < .05).
Table 4. Blood metabolites profile in nursery piglets fed monoglycerides blend and tributyrinTable Footnotec.
Digestive and non-digestive organ biometry, and pH of gastrointestinal tract contents
No treatments effect on ROW, small intestine length, and GIT contents pH were observed (Table ).
Table 5. Weight of relative digestive and non-digestive organs (expressed as a percentage of body weight) and gastrointestinal tract contents pH in nursery piglets fed monoglycerides blend and tributyrin at day 35Table Footnotea.
Intestinal morphometry and histopathology
Piglets fed the TBT diet showed higher jejunum VH:CD ratio than piglets fed other dietary treatments (p < .05) (Table ).
Table 6. Villous height (VH), crypt depth (CD) and VH:CD ratio of the jejunum and ileum in piglets fed monoglycerides blend and tributyrin at day 35Table Footnotec.
All animals showed an absence for the histopathological description of coccidiosis, bacterial lumps, rods, cystic crypts, mucus, tissue necrosis and oedema (Table ). However, piglets fed the MGD diet had higher ileum cellular infiltrate scores than piglets fed the PC diet (p < .05) (see Table and Figure ).
Figure 1. Cell infiltrate of the ileum in piglets fed monoglycerides blend and tributyrin at day 35 (n = 6 piglets per treatment). dietary treatments: (A) negative control: diet without feed additive or antimicrobial growth promoters; (B) positive control: diet containing 60 mg halquinol/kg diet; (C) diet containing 2 g monoglyceride blend/kg diet (MGD); and (D) diet containing 2 g tributyrin/kg diet (TBT). the monoglyceride blend contained 33% monolaurate and 41.3% C8 and C10 monoglycerides. The tributyrin contained 55.8% tributyrin and silica (excipient).

Table 7. Intestinal histopathology of the jejunum and ileum in piglets fed monoglycerides blend and tributyrin at day 35Table Footnotec.
Microbial population of the intestinal contents
Lower Enterobacteriaceae family counts were observed in the jejunum content of piglets fed the MGD diet compared to other dietary treatments (p < .05) (Table ).
Table 8. Bacterial counts (log10 CFU/g) in the jejunum content of piglets fed monoglycerides blend and tributyrin at day 35Table Footnotec.
Discussion
We observed an absence of effect of the additives tested on growth performance that could be explained by the complexity of diets provided and the controlled experimental conditions (Genova et al. Citation2022). However, our results agree with those previously observed in studies (Araujo et al. Citation2016; Sakdee et al. Citation2016; Grecco et al. Citation2018), where no effects of dietary acidifier blends on the growth performance of newly weaned piglets were observed. In addition, the animals fed AGP had no improved performance during the experimental period. This unexpected response may be attributed to the lower amount of halquinol administered via diet (0.06 g/kg diet). This fact is confirmed in studies conducted by Grecco et al. (Citation2018) when piglets were fed 0.2 g of halquinol/kg diet, and Habib et al. (Citation2020), when chickens were fed 0.25–1 g of halquinol/kg diet.
This indicates that the NC diet fed to piglets in the present study may have been effective in developing and maturing the GIT, favouring enzyme activity (Adewole et al. Citation2016; Pluske et al. Citation2018). Thus, we hypothesised that the dose of the additives tested failed to promote improvements in piglet performance, as reported by Sakdee et al. (Citation2016) who evaluated the effects of supplementing 0.4% TBT in piglet diets. In addition, the experimental conditions under which the animals were housed (e.g. experimental farm, lower microbial load, low sanitary pressure) could have contributed to the absence of favourable responses or even compromised the effectiveness in animals fed feed additives compared to those fed NC (Sakdee et al. Citation2016).
Abrupt weaning of piglets promotes reduced growth performance because it causes a stress condition that occurs when piglets are moved from maternity to the nursery room, impairing enzyme activity that hinders intestinal health (Pluske et al. Citation2018; Jackman et al. Citation2020). These digestive disorders lead to reduced diet intake and diarrhoea occurrence (Genova et al. Citation2021). Thus, the feeding efficiency rate is reduced due to an extra energy expenditure to support the immune system (Bauer et al. Citation2006; Pluske et al. Citation2018). Therefore, discrepant results related to biological responses in piglets fed dietary MGD and TBT than NC and PC diets may be related to an interaction between diet composition and associated with the absence of challenges, due to the fact that the effectiveness of these feed additives is more observed in critical periods (Araujo et al. Citation2016; Grecco et al. Citation2018).
During weaning, diarrhoea events are worsened due to dehydration and solute losses (Zlotowski et al. Citation2008; Grecco et al. Citation2018). Previous studies (Wang et al. Citation2017; Anacarso et al. Citation2018) have reported that dietary MGD and TBT can reduce or eliminate most pathogenic bacteria and multidrug-resistant intestinal strains by making the intestine an inhospitable environment for pathogen growth, and post-weaning diarrhoea is reduced. However, in the present study, a reduction of pathogenic bacteria was not observed in piglets consuming diets with TBT. On the other hand, the fact that piglets fed MGD showed lower abundance of Enterobacteriaceae is attributed to the bacteriostatic effect or bacterial cell lysis and cell death, although MGD exhibits more potent inhibitory activity against gram-positive bacteria (Jackman et al. Citation2020).
The results of the present study do not agree with those observed by Costa et al. (Citation2011), who tested a sodium butyrate-based additive in weaned piglets diets and observed no effects on the diarrhoea occurrence, due to the high digestibility of the diet that left no indigestible substrates for pathogenic microorganisms. Although TBT is composed of three butyric acid molecules broken down into hydrogen ions and butyrate with bactericidal function, the effect of these compounds was not observed on the cell growth of pathogenic bacteria (e.g. Enterobacteriaceae), in agreement with the study of Sotira et al. (Citation2020), when feeding 0.2% TBT to the piglets.
In agreement with our study, Fang et al. (Citation2014) reported that dietary sodium butyrate was able to reduce stress in weaned piglets based on the release of organic molecules from salts in the intestinal epithelium. In our study, the reduction on DO in piglets fed TBT is supported by the improved intestinal mucosa and villous regeneration rate (Zhang et al. Citation2020). This would promote mitosis and cell proliferation, reflecting in a higher absorptive area in the intestinal mucosa observed by the VH:CD ratio in piglets fed the TBT diet.
Here, we reasoned that although a significant reduction was observed in DO by both improved intestinal morphometry in piglets fed TBT and reduced Enterobacteriaceae abundance in piglets fed MGD, these results suggested a targeted effect on intestinal parameters (e.g. intestinal architecture and barrier, microbial populations, nutrient digestibility), but which does not always reflect in performance improvements in non-challenged or healthy animals.
Factors like diet composition, stressors, and health should be considered in the evaluation of haematological and metabolites blood results in piglets (Cooper et al. Citation2014). The above-mentioned factors probably influenced our results. Indeed, during the experimental period, piglets showed good health, received the same dietary management, and were from the same herd. The results of total protein, plasma albumin, total cholesterol, alanine aminotransferase, and glucose we observed are within the reference range for piglets which is considered the ideal physiological range (Perri et al. Citation2017).
That piglets fed the MGD diet had higher plasma urea concentrations compared to TBT or NC diets suggests a reduction in the efficiency of nitrogen compounds usage (Nowak et al. Citation2019) and lower protein availability (Fang et al. Citation2014). Although it is worth mentioning that urea concentrations were within the reference range (4.2–55.86 mg/dL) for piglets (Perri et al. Citation2017), our result agrees to that reported by Sotira et al. (Citation2020), who also observed an improvement in protein utilisation due to lower urea concentration in piglets fed TBT, but is discrepant to the finding of Jackman et al. (Citation2020), when piglets were fed medium-chain fatty acid-based diets and observed lower urea concentration.
While circulating urea in the blood is a residue from dietary protein breakdown in the liver, high concentrations may indicate renal and/or hepatic injury (Sotira et al. Citation2020), as well as inefficient use of dietary proteins. These lesions can be observed via histological, haematological, or biochemical analyses as in the present study where blood metabolites tests were performed to verify aspartate aminotransferase (AST) and alanine aminotransferase (ALT) concentrations. Notably, AST concentrations were altered in pre-starter piglets fed MGD diet suggesting physiological changes in the liver (e.g. liver damage); however, histological lesions in the liver were not assessed in the present study. Changes in the metabolic process of the liver such as a higher AST concentration can also increase urea concentration. Thus, this result suggested that piglets fed MGD at the tested dosage may have metabolic and hepatic-renal functioning alterations.
Nowak et al. (Citation2019) evaluated the effects of combined additives (eubiotic blend) on blood metabolites and reported promising effects on health, metabolism, and nutrient absorption. Such effects can be observed via blood analysis (high total proteins, albumins, glucose, and triglycerides concentration coupled with low urea concentration). However, in the present study, these variables were little affected by dietary treatments.
We used blood alkaline phosphatase (ALP) to investigate intestinal disorders such as intestinal dysbiosis (Genova et al. Citation2020). Higher ALP concentrations were observed in pre-starter piglets fed the PC diet compared to those fed the TBT diet, and this may be explained by the effect of the antibiotic fed to the piglets (Alam et al. Citation2014), due to the fact that halquinol has activity against a wide variety of gram-positive and gram-negative bacteria (Fomentini et al. Citation2016). In addition, antibiotics administration can cause intestinal dysbiosis and alter pathogenic bacteria (Saettone et al. Citation2020). An increased population of Enterobacteriaceae has previously been associated with poor intestinal health (Luise et al. Citation2019), and under these kinds of conditions, ALP is increased (Alam et al. Citation2014). However, we did not observe alterations on ALP concentrations in piglets with a higher abundance of Enterobacteriaceae.
Organ biometry was not altered by treatments, as well as the pH of GIT contents in piglets from the present study. These results agree with those previously reported by Grecco et al. (Citation2018) who reported no effects of an acidifier blend (calcium formate, calcium lactate, and medium chain fatty acids) on the stomach, small intestine, caecum, colon, liver, spleen, and kidneys of newly weaned piglets fed diets containing colistin (40 ppm) or halquinol (120 ppm). Likewise in the present study, these authors fed piglets diets containing similar amounts of nutrients.
It is reasonable to expect that dietary acidifiers would reduce gastric pH increasing gastric enzyme activity and protein digestion (Tugnoli et al. Citation2020). Although, these effects were not reported previously in similar studies (Braz et al. Citation2011; Zhang et al. Citation2020). Grecco et al. (Citation2018) reported no effects of dietary acidifiers on gastrointestinal chime which supports what we observed in piglets fed MGD and suggests this is not their main mechanism of action. This is corroborated by the partial absorption of acidifiers at the gastric level which prevents salts from getting into the small intestine and reduces possible beneficial effects. However, the tributyrin molecule is broken down in the intestine, where it exerts pH-lowering effects via hydrogen ions derived from butyrate (Sotira et al. Citation2020).
Post-weaning is marked by decreased VH and increased CD in the small intestine. This impairs intestinal epithelium and hinders nutrient digestion and absorption (Montagne et al. Citation2003; Wang et al. Citation2006). Therefore, preserving the intestinal structure is important for optimal nutrient usage in nursery piglets. The VH:CD ratio we observed in the present study suggests that dietary tributyrin improves intestinal morphometry in the jejunum and hence nutrient absorption in nursery piglets. This is supported by the action of butyrate in maintaining the integrity of the intestinal mucosa, as well as providing energy for the intestinal cells (Sotira et al. Citation2020; Zhang et al. Citation2020). Nevertheless, no better growth performance was observed. However, the benefits of these feed additives on the intestinal mucosa would also promote improvements in animal welfare due to reduced diarrhoea occurrence.
Regarding small intestine histopathological findings, we observed a greater ileum cellular infiltrate score in piglets fed the MGD diet. This suggests a greater accumulation of defence cells to provide inflammatory responses and/or infections (Satessa et al. Citation2020), but no effects on intestinal morphometry. Greater cell infiltration may occur due to the elimination of beneficial microorganisms (e.g. Bacillus, Bifidobacterium) in the small intestine which affects nutrient synthesis (Bedford and Gong Citation2018). In discrepancy to that statement, no reduction in beneficial microorganisms (e.g. lactic acid bacteria) was observed in piglets fed MGD.
The maintenance of the intestinal mucosa integrity (Plöger et al. Citation2012), the regulation of intestinal microecology (Huang et al. Citation2015; Xu et al. Citation2016), and anti-inflammatory effects (Wang et al. Citation2018) have been reported to be the mechanisms of action of MGD and organic acids. These advantageous effects were not expressed as growth performance improvement or lower histological injures suggesting inconsistent results regarding data on the intestine histopathology of animals (Satessa et al. Citation2020).
However, it is important to highlight the positive effect on the pathogenic microbial population of the jejunum, as suggested by the lower Enterobacteriaceae counts in piglets fed MGD diet, because MGD has the property to disrupt the cell membranes of bacteria and kill them (Desbois and Smith Citation2010). Therefore, an improvement in overall health is suggested (Yang et al. Citation2014; Luise et al. Citation2019).
Nevertheless, Grecco et al. (Citation2018) reported that results from studies involving feed additives in piglets are attributed by the number of dietary ingredients, diet palatability, and maturity and intestinal health prior to the experiment. In a previous study (Zhang et al. Citation2020), dietary tributyrin and methyl salicylate was beneficial to GIT histopathology and increased lactobacilli population in piglets.
Our results showed the potential of dietary MGD to reduce the Enterobacteriaceae family in experimental pig units because MGD plays a role as an antimicrobial and imunomodulator. These MGD actions create barriers to protecting the intestinal epithelium from pathogenic bacteria such as those belonging to the Enterobacteriaceae family (Luise et al. Citation2019; Jackman et al. Citation2020; Xu et al. Citation2020).
Although improvements in intestinal histology, reduced diarrhoea occurrence, and increased nitrogen use efficiency were evidenced in animals fed TBT, none of the dietary treatments positively affected piglet performance. In summary, we believe that these positive effects were not reflected in optimal performance due to the complexity of factors (e.g. digestibility of energy and nutrients, weaning age, experimental conditions, stressing factors, and strict sanitation) in nursery piglets.
Conclusions
The use of a monoglyceride blend restricted the growth of the Enterobacteriaceae family in the jejunum but a diet containing tributyrin supported intestinal histology as suggested by the positive effect on VH:CD ratio, lower diarrhoea occurrence, and efficiency of nitrogen compounds usage in starter piglets. In addition, none of the dietary treatments promoted improvements in the performance of nursery piglets. The results of our research suggest further studies involving the use of tributyrin and monoglycerides in diets for enhanced piglet performance under experimental challenge models.
Ethical approval
The animal procedures were approved by the Ethics Committee on the Use of Animals in Experimentation at the Universidade Estadual do Oeste do Paraná (Unioeste) (protocol no. 02/2022).
Acknowledgements
We thank the efforts and support in the research from the Sauvet Company, Western Paraná State University, and the Copagril Agroindustrial Cooperative.
Disclosure statement
No potential conflict of interest was reported by the author(s).
Data availability statement
The data that support the findings of this study are available from the corresponding author, J.L.G., upon reasonable request.
Additional information
Funding
References
- Association of Official Analytical Chemists [AOAC]. 2019. Official methods of analysis. Rockville (MD): Association of Official Analytical Chemists.
- Adewole DI, Kim IH, Nyachoti CM. 2016. Gut health of pigs: challenge models and response criteria with a critical analysis of the effectiveness of selected feed additives – a review. Asian-Australas J Anim Sci. 29(7):909–924. doi: 10.5713/ajas.15.0795.
- Alam SN, Yammine H, Moaven O, Ahmed R, Moss AK, Biswas B, Muhammad N, Biswas R, Raychowdhury A, Kaliannan K, et al. 2014. Intestinal alkaline phosphatase prevents antibiotic-induced susceptibility to enteric pathogens. Ann Surg. 259(4):715–722. doi: 10.1097/SLA.0b013e31828fae14.
- Anacarso I, Quartieri A, De Leo R, Pulvirenti A. 2018. Evaluation of the antimicrobial activity of a blend of monoglycerides against Escherichia coli and Enterococci with multiple drug resistance. Arch Microbiol. 200(1):85–89. doi: 10.1007/s00203-017-1419-5.
- Araujo G, Terré M, Mereu A, Ipharraguerre I, Bach A. 2016. Effects of supplementing a milk replacer with sodium butyrate or tributyrin on performance and metabolism of Holstein calves. Anim Prod Sci. 56(11):1834–1841. doi: 10.1071/AN14930.
- Bauer DC, Hunter DJ, Abramson SB, Attur M, Corr M, Felson D, Heinegard D, Jordan JM, Kepler TB, Lane NE, et al. 2006. Classification of osteoarthritis biomarkers: a proposed approach. Osteoarthritis Cartilage. 14(8):723–727. doi: 10.1016/j.joca.2006.04.001.
- Bedford A, Gong J. 2018. Implications of butyrate and its derivatives for gut health and animal production. Anim Nutr. 4(2):151–159. doi: 10.1016/j.aninu.2017.08.010.
- Berto PN, Tse MLP, Ramos DRA, Saleh MAD, Miassi GM, Yamatogi RS, Berto DA, Trindade Neto MA. 2020. Dietary supplementation with hydrolysed yeast and its effect on the performance, intestinal microbiota, and immune response of weaned piglets. An Acad Bras Ciênc. 92(Suppl 1):e20180969. doi: 10.1590/0001-3765202020180969.
- Braz DB, Costa LB, Berenchtein B, Tse MLP, Almeida VV, Miyada VS. 2011. Acidifiers as alternatives to antimicrobial growth promoter of weanling pigs. Arch Zootec. 60(231):745–756. doi: 10.4321/S0004-05922011000300062.
- Campbell JM, Crenshaw JD, Polo J. 2013. The biological stress of early weaned piglets. J Anim Sci Biotechn. 4(1):1–4.
- Cooper CA, Moraes LE, Murray JD, Owens SD. 2014. Hematologic and biochemical reference intervals for specific pathogen free 6-week-old Hampshire-Yorkshire crossbred pigs. J Anim Sci Biotechnol. 5(1):1–6.
- Costa LB, Berenchtein B, ALmeida VV, Tse MLP, Braz DB, Andrade C, Mourão GB, Miyada VS. 2011. Phytobiotic additives and sodium butyrate as alternatives to antibiotics for weanling pigs. Arch Zootec. 60(231):687–698. Portuguese. doi: 10.4321/S0004-05922011000300056.
- Desbois AP, Smith VJ. 2010. Antibacterial free fatty acids: activities, mechanisms of action and biotechnological potential. Appl Microbiol Biotechnol. 85(6):1629–1642. doi: 10.1007/s00253-009-2355-3.
- Fang CL, Sun H, Wu J, Niu HH, Feng J. 2014. Effects of sodium butyrate on growth performance, haematological and immunological characteristics of weanling piglets. J Anim Physiol Anim Nutr (Berl). 98(4):680–685. doi: 10.1111/jpn.12122.
- Fomentini M, Haese D, Kill JL, Sobreiro RP, Puppo DD, Haddade IR, Lima AL, Saraiva A. 2016. Prebiotic and antimicrobials on performance, carcass characteristics, and antibody production in broilers. Cienc Rural. 46(6):1070–1075. doi: 10.1590/0103-8478cr20150133.
- Genova JL, Melo ADB, Rupolo PE, Carvalho ST, Costa LB, Carvalho PLO. 2020. A summary of feed additives, intestinal health and intestinal alkaline phosphatase in piglet nutrition. Czech J Anim Sci. 65(8):281–294. doi: 10.17221/70/2020-CJAS.
- Genova JL, Melo ADB, Rupolo PE, Macedo REFD, Engracia Filho JR, Carvalho ST, Faucitano L, Costa LB, Carvalho PLO. 2022. New findings of intestinal alkaline phosphatase: effects on intestinal and organ health of piglets challenged with ETEC F4 (K88). R Bras Zootec. 51:e20210144.
- Genova JL, Rupolo PE, Melo ADB, Santos LBDA, Wendt GN, Barbosa KA, Carvalho ST, Oliveira NTE, Costa LB, Carvalho PLO. 2021. Biological response of piglets challenged with Escherichia coli F4 (K88) when fed diets containing intestinal alkaline phosphatase. Czech J Anim Sci. 66(10):391–402. doi: 10.17221/82/2021-CJAS.
- Grecco HAT, Amorim AB, Saleh MAD, Tse Marcos LP, Telles FG, Miassi GM, Pimenta GM, Berto DA. 2018. Evaluation of growth performance and gastro-intestinal parameters on the response of weaned piglets to dietary organic acids. An Acad Bras Cienc. 90(1):401–414. doi: 10.1590/0001-3765201820160057.
- Guo M, Hayes J, Cho KO, Parwani AV, Lucas LM, Saif LJ. 2001. Comparative pathogenesis of tissue culture-adapted and wild-type Cowden porcine enteric calicivirus (PEC) in gnotobiotic pigs and induction of diarrhea by intravenous inoculation of wild-type PEC. J Virol. 75(19):9239–9251. doi: 10.1128/JVI.75.19.9239-9251.2001.
- Habib MA, Haque MA, Islam MS, Liton MR. 2020. Effect of dietary halquinol supplementation on the productive performances, carcass traits and blood profile of Sonali chicken. Asian J Med Biol Res. 5(4):316–323. doi: 10.3329/ajmbr.v5i4.45270.
- Huang C, Song P, Fan P, Hou C, Thacker P, Ma X. 2015. Dietary sodium butyrate decreases postweaning diarrhea by modulating intestinal permeability and changing the bacterial communities in weaned piglets. J Nutr. 145(12):2774–2780. doi: 10.3945/jn.115.217406.
- ISO. 2006. ISO 4832; microbiology of food and animal feeding stuffs–horizontal method for the enumeration of coliforms—colony-count technique. Geneva: International Organization for Standardization. [accessed 2022 Jan 01]. https://www.iso.org/standard/38282.html.
- Jackman JA, Boyd RD, Elrod CC. 2020. Medium-chain fatty acids and monoglycerides as feed additives for pig production: towards gut health improvement and feed pathogen mitigation. J Anim Sci Biotechnol. 23(1):11–44.
- Kraieski AL, Hayashi RM, Sanches A, Almeida GC, Santin E. 2017. Effect of aflatoxin experimental ingestion and Eimeira vaccine challenges on intestinal histopathology and immune cellular dynamic of broilers: applying an intestinal health index. Poult Sci. 96(5):1078–1087. doi: 10.3382/ps/pew397.
- Liu Y, Espinosa CD, Abelilla JJ, Casas GA, Lagos LV, Lee SA, Kwon WB, Mathai JK, Navarro DMDL, JaworskI NW, et al. 2018. Non-antibiotic feed additives in diets for pigs: a review. Anim Nutr. 4(2):113–125. doi: 10.1016/j.aninu.2018.01.007.
- Luise D, Bertocchi M, Motta V, Salvarani C, Bosi P, Luppi A, Trevisi P. 2019. Bacillus sp. probiotic supplementation diminish the Escherichia coli F4ac infection in susceptible weaned pigs by influencing the intestinal immune response, intestinal microbiota and blood metabolomics. J Anim Sci Biotechnol. 10(1):1–16.
- Manzanilla EG, Nofrarías M, Anguita M, Castillo M, Perez JF, Martín-Orúe SM, Kamel C, Gasa J. 2006. Effects of butyrate, avilamycin, and a plant extract combination on the intestinal equilibrium of early-weaned pigs. J Anim Sci. 84(10):2743–2751. doi: 10.2527/jas.2005-509.
- Modina SC, Polito U, Rossi R, Corino C, Di Giancamillo A. 2019. Nutritional regulation of gut barrier integrity in weaning piglets. Animals. 9(12):1–15.
- Montagne L, Pluske JR, Hampson DJ. 2003. A review of interactions between dietary fibre and the intestinal mucosa, and their consequences on digestive health in young non-ruminant animals. Anim Feed Sci Tech. 108(1–4):95–117. doi: 10.1016/S0377-8401(03)00163-9.
- Nguyen DH, Seok WJ, Kim IH. 2020. Organic acids mixture as a dietary additive for pigs: a review. Animals. 10(6):952. doi: 10.3390/ani10060952.
- Nowak P, Kasprowicz-Potocka M, Zaworska A, Nowak W, Stefańska B, Sip A, Grajek W, Grajek K, Frankiewicz A. 2019. The effect of combined feed additives on growing pigs’ performance and digestive tract parameters. Ann Anim Sci. 19(3):807–819. doi: 10.2478/aoas-2019-0030.
- Pérez-Calvo E, Wicaksono AN, Canet E, Daulton E, Ens W, Hoeller U, Verlhac V, Celi P, Covington JA. 2019. The measurement of volatile organic compounds in faeces of piglets as a tool to assess gastrointestinal functionality. Biosyst Eng. 184:122–129. doi: 10.1016/j.biosystemseng.2019.06.005.
- Perri AM, O’sullivan TL, Harding JC, Wood RD, Friendship RM. 2017. Hematology and biochemistry reference intervals for Ontario commercial nursing pigs close to the time of weaning. Canad Vet J. 58(4):371–376.
- Plöger S, Stumpff F, Penner GB, Schulzke J-D, Gäbel G, Martens H, Shen Z, Günzel D, Aschenbach JR. 2012. Microbial butyrate and its role for barrier function in the gastrointestinal tract. Ann N Y Acad Sci. 1258(1):52–59. doi: 10.1111/j.1749-6632.2012.06553.x.
- Pluske JR. 2016. Invited review: Aspects of gastrointestinal tract growth and maturation in the pre- and post-weaning period of pigs. J Anim Sci. 94(Suppl 3):399–411. doi: 10.2527/jas.2015-9767.
- Pluske JR, Turpin DL, Kim JC. 2018. Gastrointestinal tract (gut) health in the young pig. Anim Nutr. 4(2):187–196. doi: 10.1016/j.aninu.2017.12.004.
- Rostagno HS, Albino LFT, Hannas MI, Donzele JL, Sakomura NK, Perazzo FG, Saraiva A, Teixeira ML, Rodrigues PB, Oliveira RFD. 2017. Tabelas brasileiras para aves e suínos: composição de alimentos e exigências nutricionais. Viçosa (MG): Universidade Federal de Viçosa.
- Saettone V, Biasato I, Radice E, Schiavone A, Bergero D, Meineri G. 2020. State-of-the-art of the nutritional alternatives to the use of antibiotics in humans and monogastric animals. Animals. 10(12):2199. doi: 10.3390/ani10122199.
- Sakdee J, Poeikhamph T, Rakangthon C, Poungpong K, Bunchasak C. 2016. Effect of tributyrin supplementation in diet on production performance and gastrointestinal tract of healthy nursery pigs. Pak J Nutr. 15(11):954–962. doi: 10.3923/pjn.2016.954.962.
- Satessa GD, Kjeldsen NJ, Mansouryar M, Hansen HH, Bache JK, Nielsen MO. 2020. Effects of alternative feed additives to medicinal zinc oxide on productivity, diarrhoea incidence and gut development in weaned piglets. Animals. 14(8):1638–1646. doi: 10.1017/S1751731120000154.
- Sotira S, Dell’Anno M, Caprarulo V, Hejna M, Pirrone F, CallegarI ML, Tucci TV, Rossi L. 2020. Effects of tributyrin supplementation on growth performance, insulin, blood metabolites and gut microbiota in weaned piglets. Animals. 10(4):726. doi: 10.3390/ani10040726.
- Tugnoli B, Giovagnoni G, Piva A, Grilli E. 2020. From acidifiers to intestinal health enhancers: how organic acids can improve growth efficiency of pigs. Animals. 10(1):134. doi: 10.3390/ani10010134.
- Wang Y, Shan T, Xu Z, Liu J, Feng J. 2006. Effect of lactoferrin on the growth performance, intestinal morphology, and expression of PR-39 and protegrin1 genes in weaned piglets. J Anim Sci. 84(10):2636–2641. doi: 10.2527/jas.2005-544.
- Wang CC, Wu H, Lin FH, Gong R, Xie F, Peng Y, Feng J, Hu CH. 2018. Sodium butyrate enhances intestinal integrity, inhibits mast cell activation, inflammatory mediator production and JNK signaling pathway in weaned pigs. Innate Immun. 24(1):40–46. doi: 10.1177/1753425917741970.
- Wang Y, Wu Y, Wang B, Cao X, Fu A, Li Y, Li W. 2017. Effects of probiotic Bacillus as a substitute for antibiotics on antioxidant capacity and intestinal autophagy of piglets. AMB Expr. 7(1):1–11. doi: 10.1186/s13568-017-0353-x.
- Xu J, Chen X, Yu S, Su Y, Zhu W. 2016. Effects of early intervention with sodium butyrate on gut microbiota and the expression of inflammatory cytokines in neonatal piglets. PLOS One. 11(9):e0162461. doi: 10.1371/journal.pone.0162461.
- Xu Y, Lahaye L, He Z, Zhang J, Yang C, Piao X. 2020. Micro-encapsulated essential oils and organic acids combination improves intestinal barrier function, inflammatory responses and microbiota of weaned piglets challenged with enterotoxigenic Escherichia coli F4 (K88+). Anim Nutr. 6(3):269–277. doi: 10.1016/j.aninu.2020.04.004.
- Yang L, Bian G, Su Y, Zhu W. 2014. Comparison of faecal microbial community of lantang, bama, erhualian, meishan, xiaomeishan, duroc, landrace, and yorkshiresows. Asian-Australas J Anim Sci. 276(6):898–906.
- Zhang WX, Zhang Y, Zhang XW, Deng ZX, Liu JX, He ML, Wang HF. 2020. Effects of dietary supplementation with combination of tributyrin and essential oil on gut health and microbiota of weaned piglets. Animals. 10(2):180. doi: 10.3390/ani10020180.
- Zlotowski P, Driemeier D, Barcellos DESN. 2008. Patogenia das diarréias dos suínos: modelos e exemplos. Acta Sci Vet. 36(1):S81–S86.