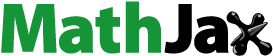
Abstract
Insects represent a promising alternative protein source as food and feed. This study evaluated the use of lactoferrin as a hydration source in Tenebrio molitor larvae rearing, on animal performance, nutritional and functional characteristics of insect meals. Seven-week-old larvae were reared for 14 days and randomly divided into two experimental groups: control group (CTRL, n = 8) reared on wheat bran; treatment group (TRT, n = 8) reared on wheat bran and supplemented with lactoferrin solution as a hydration source at days 0, 4, 8, 12. Larvae weight and feed consumption were recorded weekly to calculate growth performance. At the end of the trial, larvae were collected for meal production, whose main nutritional characteristics and colour were analysed as well as antibacterial activity against O138 Escherichia coli. The results showed a lower larval mortality in the TRT group compared to the CTRL (p < 0.05). The nutritional composition of the insect meals revealed higher content of proteins (p < 0.05) in the TRT group. After 3 h (p < 0.05), the meals hydrated with lactoferrin demonstrated a higher E. coli inhibitory activity compared to the CTRL group. In conclusion, the functionality of insect meals was enhanced by using innovative ingredients as hydration sources capable of transferring bioactive properties.
Insects are a sustainable alternative source of protein with nutritional and functional properties.
Water supplemented with lactoferrin as a hydration source for Tenebrio molitor larvae reduces the mortality rate.
Lactoferrin supplementation increases the antimicrobial activity of meal obtained from Tenebrio molitor.
HIGHLIGHTS
Introduction
The demand for food from animal origin is expected to increase exponentially in line with the predicted global population growth. In order to sustain zootechnical production, increasing livestock sustainability will be crucial (Food and Agriculture Organization of the United Nations (FAO) Citation2018). Alternative solutions to traditional protein sources are urgently needed. Over the last decade, the consumption of edible insects has steadily expanded in Europe, and insects are considered as an alternative to soybean and fish meals that have traditionally been used in animal feed as protein sources (Paula et al. Citation2018; Egerton et al. Citation2020).
The advantages of insects lie in their capacity to bioconvert waste materials into high protein biomass (Ites et al. Citation2020) and their relatively simple rearing technique, thus allowing the use of detached rearing modules. According to the Food and Agriculture Organisation (FAO), in order to tackle the demand for animal-origin proteins, insects could be a valuable source of food (FAO Citation2021). In fact, insects are already used in animal feed, and for some animals, such as chickens, they could constitute the main source of proteins.
Insects are also an important opportunity for feeding livestock, where alternative high-biological value protein sources to soybean are required (Hawkey at al. Citation2021; Parrini et al. Citation2023). Insect rearing is considered a valuable opportunity for future livestock and environmental sustainability and waste reduction according to the 2030 Agenda goals.
Although different animal species can be reared using waste and by-products, insects require less water and land, thus resulting in a higher efficiency and sustainability of their production system (van Huis et al. Citation2013). Tenebrio molitor is an insect species with four stages of development: egg, larva, pupa and adult. Larvae have a high protein content with a concentration comparable to soybean and a profile of essential amino acids that works well for animal nutrition purposes (Jantzen da Silva Lucas et al. Citation2020). T. molitor larvae are also nutritious due to their fat, vitamin and mineral content and could be considered a functional feed source due to their antioxidant and antibacterial properties (Shin et al. Citation2019; Baek et al. Citation2019).
The growing substrate has been recognised to play a key role in insect performance and the functional properties of the meal obtained that could valorise the final product (Pinotti and Ottoboni Citation2021). The intake of carbohydrates, lipids, and proteins is essential to improve the final chemical and physical characteristics of the insect biomass. As with other organisms, hydration is key to maintaining the physiological homeostasis of insects. In fact, a correct water supply to the larvae ensures the production of bioactive molecules responsible for reducing oxidative stress, thus preventing the production of reactive oxygen species (McCluney and Date Citation2008).
To ensure optimal performance, moistened feed should be always available during the growth cycle. Some vegetables, such as carrots chunks, are regularly used for this purpose. The administration of moistened feed is more difficult than dry feed due to the spoilage rate of vegetables due to their high water activity. Vegetables generally need to be replaced on a daily basis, and ensuring a standardised distribution in each crate is challenging. Due to the complexity of the task and time required for the administration of moistened feed, small-scale insect farms usually provide moistened substrate manually (Deruytter et al. Citation2021).
Ingredients with functional characteristics administered during the water supply could further enhance the characteristics of the final product, i.e. the content of bioactive compounds with beneficial properties in insect meals (Antonopoulou et al. Citation2022). Among the bioactive ingredients used as nutraceutical additives, lactoferrin is a binding iron glycoprotein present in milk with antimicrobial, antioxidant, immunomodulator and antihypertensive effects (Manzanares et al. Citation2015; Niaz et al. Citation2019; Luna-Castro et al. Citation2022). Several studies have shown that the oral administration of lactoferrin in animals improves immune responses (Sfeir et al. Citation2004; Jahan et al. Citation2017). In addition, the protein intake from the rearing substrate positively affects the growth and functional characteristics of the final biomass of the insects. However, to date, no studies have investigated the effect of directly administrating the protein solution through hydration to insects.
Considering the importance of the final yield in terms of insect biomass obtained and the functional activities of the insects in relation to the rearing conditions, the aim of this study was to evaluate the effects of hydration supplemented with lactoferrin on T. molitor larvae performance and survival, as well as the nutritional and functional characteristics of insect meals.
Materials and methods
Insect rearing conditions, substrates, insect meal production and sampling
T. molitor larvae were reared at the Italian Cricket Farm (Turin, Italy). From birth, larvae were fed with wheat bran as a growing substrate. Fruits and vegetables were used as a source of hydration for the growing period before the experimental trial. For the following study, a total of 16 containers (27×39×14 cm) were randomly divided into two groups: control group (CTRL, n = 8) and treatment group (TRT, n = 8). A total of 1.6 kg of approximately 7-week-old T. molitor larvae were randomly divided to each container (100 g/container; n = 500 larvae) and reared under controlled conditions (26 ± 2 °C, 60–75% relative humidity) using the same growing substrates as with wheat bran (50 g/container). Seven-week old larvae were selected since they are in the last stage of development and thus larvae can best exploit functional compounds in growing substrates prior to meal production (Yu et al. Citation2021). The adopted ratio between larvae and substrate was chosen according to data reported by van Broekhoven et al. (2015) and adapted to the conditions of our studies.
In order to ensure adequate humidity (∼70%) for guaranteeing proper larval growth and feed intake, both groups received sprayed water on day 0, 2, 4, 6, 8, 10 and 12 (10 mL/day). The TRT was differentiated from the CTRL group by the administration of lactoferrin solution directly to the insect as a source of hydration. Specifically, a total of 40 ml of a solution containing 12.7% of pure lactoferrin (dry matter: 14.2%; ash: 0.12%) (Candioli Farmaceutici S.r.l., Turin, Italy) was sprayed on the TRT larvae at days 0, 4, 8 and 12 (10 mL/day). This dose ensures a supplementation of 0.02 mL of solution per larva, about 2.54 mg of lactoferrin/larva.
The rearing experiment was conducted for 14 days in order to obtain fully-grown mealworms, i.e. edible insects at the last period of the larval phase before becoming pupae. The development of larvae was monitored daily.
Substrates were collected and replaced with 50 g of fresh wheat bran seven days after the beginning of the trial in order to guarantee sufficient and ad libitum feed for insects and to maintain a ratio of larvae to grams of substrate of 2:1. Samples of residual substrate were collected at days 7 and 14, and stored at −20 °C for further analysis. The weight of the larvae per container was measured weekly by separating the growing substrates from the larvae using a sieve (300 μm) (Kröncke and Benning Citation2022), and total insect biomass was weighed on a scale with an accuracy of 0.01 g (B2002-S, Mettler Toledo, Milan, Italy).
At the end of the experimental trial (day 14), larvae and substrates were separated using a sieve, and the growing substrates were collected for laboratory analysis. All the larvae in each container were cooked by drying in a microwave (model CMG2071M, Candy Hoover Group S.r.l., Brugherio, Italy), using the maximum input power of 120 W with a frequency of 2450 MHz for five minutes (Mancini et al. Citation2021). Dead larvae were then ground with a flour mill to obtain insect meals for a total of eight replicates per experimental group.
Growth performance and feed conversion efficiency
In order to determine the feed consumption (%), residual substrates were separated from the larvae and collected at days 7 and 14. Using a sieve (square mesh 300 μm), the residual substrates were comminuted into faeces and non-ingested feed. At the end of the trial, the difference between the total administered substrate and the leftover diet was used to calculate the feed consumption. The growth rate (%) was obtained from the difference of the total gain after 14 days divided by the initial biomass weight (100 g).
The efficiency of conversion of ingested food (ECI) was expressed on a dry matter basis, and calculated as the percentage of the ratio between the weight gained and weight of the ingested food (Oonincx et al. Citation2015). The feed conversion efficiency was evaluated as the feed conversion ratio (FCR) expressed on a fresh matter basis and calculated as the ratio between the weight of the ingested food and weight gained.
The number of dead larvae was weekly registered, along with the separation of the insects and residual substrates. The overall survival rate was calculated as the percentage of live larvae at the end of the trial (Jamaa et al. Citation2021). The initial number of larvae was estimated based on the average weight of T. molitor larvae at the age of 7 weeks (∼0.2 g) with reference to the total weight of each container, and the weight of dead larvae was subtracted from the recorded weight after 7 and 14 days. The survival rate was then calculated based on the number of live larvae at 14 days in relation to the total number of larvae at the beginning of the experimental period.
Chemical composition of rearing substrate and larvae of Tenebrio molitor
The chemical composition of the substrates (administered on day 0, and collected on day 14) was determined after milling using a 1 mm screen-grid following the official methods of analysis (AOAC International Citation2019). Dry matter was determined by drying samples in previously weighed aluminium bags in a forced-air oven at 65 °C for 24 h (AOAC method 930.15). Lipid content (ether extract, EE) was obtained using ethyl ether extraction (AOAC 2003.05). Total ash content was determined after incinerating samples at 550 °C for 3 h (AOAC method 942.05). Crude proteins (CPs) were determined with the Kjeldahl method, using 6.25 as the average nitrogen conversion coefficient for vegetable growing substrates (AOAC method 2001.11). Crude fibre (CF) was determined using the filtering bags technique (AOCS method Ba 6a-05). Non-structural carbohydrates (NSC) were calculated by subtracting all evaluated nutrients from 100 as follows: [100-(moisture%+ash%+EE%+CP%+CF%)].
The same methods were adopted to evaluate the chemical composition of larvae meals, except for CPs. In fact, to determine the protein content and to exclude the nitrogen of chitin content, 4.76 was used as the nitrogen-to-protein conversion factor, as reported by Janssen et al. (2017). All trays of insects and residual substrates were analysed separately as independent replicates for each group.
Colour analysis of Tenebrio molitor larvae meals
Insect meals colour was measured to evaluate the pigmentation of insects using a colorimeter (Minolta Croma-Meter CR-400, Minolta Camera Co., Ltd., Osaka, Japan). Data were registered by direct contact between the sensing head of the colorimeter and samples. Insect meals were characterised by three parameters: L* (lightness scale of 100; 0 matches with black and 100 with white), a* (greenness/redness scale) and b* (blueness/yellowness scale). The colorimetric difference ΔE between two samples was determined with the following formula:
If ΔE is between 1 and 2, then only an experienced observer will notice the difference (Mokrzycki and Tatol Citation2011). All determinations were performed in triplicate per sample.
Extraction of functional components from Tenebrio molitor meal and substrates
Methanol and deionised water were used to extract dried T. molitor larvae meal from the control and treatment groups (Frazzini et al. Citation2022). Briefly, 225 mg of insect meals, randomly selected from replicates (n = 8/group), was diluted in 1.5 mL of a solution of methanol and deionised water (50:50, v/v). The mixture was vortexed and then stirred at room temperature for 30 min. Samples were centrifuged for 15 min at 10,000 rpm at 4 °C. Supernatants were collected and filtered through a 0.45 μm syringe filter. Each sample from a single replicate was extracted three times, and the resulting supernatants were merged into a single tube, and stored at −20 °C. The same procedure was adopted for the extraction of residual substrates.
Evaluation of antioxidant properties (ABTS assay)
The antioxidant activity of the residual substrates collected at day 14 was evaluated by adopting an ABTS assay following Frazzini et al. (Citation2022). The working solution of ABTS•+ was diluted in deionised water to obtain an absorbance of 0.700 ± 0.02 OD at 734 nm at room temperature. Trolox stock solution (2.5 mM in deionised water) was used to produce the standard curve. Substrate extracts were diluted in relation to the solvents used for the extraction (deionised water and methanol, 50:50, v/v) and tested in the following concentrations: 100 vol%, 50 vol%, and 25 vol%. The assay was performed by adding 10 μL of each prepared concentration to 1 mL of working solution (ABTS•+). Absorbances were recorded after 6 min of incubation in the dark, and all determinations were performed in triplicate. The total antioxidant capacity after six minutes of reaction was expressed as the percentage inhibition (PI%), according to the following equation:
Bacterial growth inhibitory activity
The antibacterial activity of insect meals was evaluated using the O138 Escherichia coli strain belonging to the strain collection of the Department of Veterinary Medicine and Animal Sciences of the University of Milan. The strain was genetically characterised for genes encoding two virulence factors: adhesive fimbria F18 and verocytotoxin (VT2e) (Reggi et al. Citation2020). To evaluate the ability of the bioactive compound extracts to inhibit bacterial growth, a liquid culture growth inhibition assay was performed with O138 E. coli. An overnight culture of O138 E. coli in Luria-Bertani (LB) broth was used as the inoculum for the experiments.
The growth inhibition assay was performed as follows: extracts were diluted in LB liquid medium to obtain concentrations of 25 vol% and 12.5 vol% in order to test two different extract dilutions. A total of 100 μL of the diluted extract was added to a 96-well plate and 30 μL of E. coli inoculum were added. Positive controls were prepared by adding 30 μL of E. coli inoculum to methanol/distilled water solution (50:50, v/v) to evaluate bacterial growth without any external influence. To correct for the background colour, negative controls were prepared by adding 30 μL of LB without the E. coli inoculum. All samples were then incubated at 37 °C in a shaking incubator for six hours. The growth rate of E. coli was estimated hourly for six hours (T0, T1, T2, T3, T4, T5, and T6) by measuring the absorbance with a microplate spectrophotometer at an optical density (OD) of 620 nm. The measured OD was converted to log10 of the number of cells/mL, considering 1 OD = 1 × 109 cells/mL (Dell’Anno et al. Citation2020).
Extraction and one-dimensional sodium dodecyl sulphate polyacrylamide gel electrophoresis (SDS-PAGE) of soluble proteins from insect meals and residual substrates
Proteins from insect meals and residual substrates at 14 days were extracted with mortar and pestle homogenisation using a buffer for the extraction of soluble proteins consisting of 50 mM Tris-HCl (pH 8), 200 mM NaCl, 5 mM EDTA. After homogenisation, the samples were centrifuged at 15,000 rpm for 15 min at 4 °C and the supernatant was collected for protein quantification. The extracted proteins were quantified by the Bradford method, using bovine serum albumin (BSA) as the standard (Bonjoch and Tamayo Citation2001).
To determine the apparent molecular mass, SDS-PAGE was performed using a 10% (w/v) polyacrylamide gel (BioRad Mini-PROTEAN TGX Precast Gels, Bio-Rad, Hercules, CA, USA). The electrophoresis run was performed under denaturing conditions at 200 V until the loading dye reached 0.5 cm from the bottom of the gel (Mini-PROTEAN Tetra Vertical Electrophoresis Cell, Bio-Rad, Hercules, CA, USA). The gel was then stained with Coomassie brilliant blue R-250 (Sigma, Saint Louis, MO, USA) and images were acquired using iBright FL 1500 Imaging System (Invitrogen Corporation, Thermo Fisher Scientific Inc., Waltham, Massachusetts, USA).
Statistical analysis
All data were analysed using GraphPad Prism (Version 9.1.1). Data on growth performance and microbial growth inhibition were analysed using a generalised linear model. The model included the fixed effects of treatment and time (day or hour) and their interaction. Regarding the antioxidant activity, data were analysed using one-way analysis of variance (ANOVA). The average weight of insects for each group, chemical composition of growing substrates, residual substrates, T. molitor meal, L, a, b parameters and survival rate were statistically analysed using Student’s t-test for unpaired samples comparing the CTRL with the TRT group. Data were presented as mean ± standard error. Differences were considered statistically significant for p ≤ 0.05.
Results
Feed conversion efficiency and growth performance
All the growth parameters were not affected by the treatments. T. molitor larvae showed an average weight of 104.5 ± 4.08 g and 104.9 ± 7.6 g (n larvae at the end of the trial = 481) for the CTRL group, and 103.2 ± 3.23 g and 110.5 ± 3.57 g (n larvae at the end of the trial = 494) for the TRT group registered after 7 days and 14 days, respectively. No significant differences in growth rate (%) were observed between the CTRL group and the TRT group during the trial, registering 6.10% and 10.48%, respectively. Despite the wide variability in terms of feed conversion index, a slightly numerically higher ECI (%) was found in our experimental conditions involving larvae whose feed is hydrated with lactoferrin ().
Table 1. Substrate consumed per gram of diet on a fresh matter (DM) basis, feed conversion efficiency (ECI) on a dry matter basis and feed conversion ratio (FCR) on a fresh matter basis.
The treatment group showed a higher survival rate compared to the control group after 14 days (p < 0.05) ().
3.2. Chemical composition of growing substrates
shows the chemical composition of the growth substrates at day 0 and the residual substrates at day 14 in terms of specific nutrient composition. Regarding the residual substrates, which consisted of faeces and uneaten feed, chemical composition analysis showed significant differences between the two groups for the content of DM, EE and ash content that were higher in CTRL compared to TRT (p < 0.05).
Table 2. Chemical analysis of control (CTRL) and treatment (TRT) group growing substrates administered at day 0 of the trial and residual growth substrates collected at day 14.
3.3. Antioxidant activity of residual growing substrates
The antioxidant properties of the residual growing substrates extracted were evaluated at three different concentrations: 100 vol%, 50 vol%, and 25 vol%.
An analysis of the antioxidant activity revealed a dose-related antioxidant effect with maximum values at a concentration of 100% of growing substrate extracts after 6 min of reaction for the CTRL and TRT group (p < 0.05). In particular, increased antioxidant activity was observed in the extracts of the TRT growing substrates compared to the CTRL group for the three concentrations tested (100%; 50%; 25%). We observed the following results for the concentration of µmol Trolox Equivalent: 3078.00 ± 56.98 (TRT, 100%), 2619.17 ± 6.37 (TRT, 50%) and 1903.00 ± 24.25 (TRT, 25%) and 2680.33 ± 261.10 (CTRL, 100%), 2162.17 ± 30.89 (CTRL, 50%), 1243.50 ± 54.50 (CTRL, 25%) (p < 0.05) ().
Figure 2. Antioxidant activity of residual growing substrates extracts at three different concentrations (100%; 50%; 25%) in the treatment (TRT) and the control (CTRL) groups. All values are listed as mean ± standard deviations. * Asterisks indicate statistically significant differences between tested groups (p < 0.05).

Chemical composition of Tenebrio molitor meal
The results of the nutritional composition of T. molitor meal showed significant differences between the control and treatment groups for the content of CP and EE (p < 0.05; ). The lipid content of insect meals was different registering values of 30.75 ± 2.33% (on DM) and 27.49 ± 0.94% (on DM) for the CTRL and TRT groups (p = 0.003), respectively. In addition, the CP was lower in CTRL group with a percentage of 44.02 ± 3.12% (on DM) compared to the TRT group with a CP content of 46.66 ± 1.34% (on DM) (p = 0.045).
Table 3. Nutritional composition of insect meals in the control (CTRL) and the treatment (TRT) groups.
Table 4. Lightness (L*), red/green (a*) and yellow/blue (b*) results of control and treatment insect meals groups.
Colorimetric analysis of Tenebrio molitor meal
A colorimeter was used to measure L*, a*, b* and ΔE was calculated between groups (). Samples from the CTRL group had a higher average of a* and b* indexes compared to TRT (p < 0.05); these data demonstrate that meals obtained by insects grown on lactoferrin resulted in a darker colour compared to the control meals. Indeed, the variation in colour between CTRL and TRT meals resulted in 1.66 of ΔE.
Antimicrobial activity of Tenebrio molitor meal
The growth inhibition activity was evaluated for each extract considering the dilution 1:4 against O138 E. coli. The antimicrobial capacity, i.e. the growth inhibitory activity, decreased by increasing the dilution factor. The TRT group showed a higher inhibitory capacity compared to the CTRL group at a dilution of 1:4 (p < 0.01) (). A time-related effect was also observed for the inhibitory activity with a significant difference between the two groups from the third to the sixth hour after bacterial inoculation (p < 0.0001).
Figure 3. Evaluation of growth inhibition of O138 E. coli of insect meals in the control (CTRL) and the treatment (TRT) groups for six hours after the inoculation. Data were analysed using two-way ANOVA, and shown as means and standard deviations. * Asterisks indicate statistically significant differences among tested groups (p < 0.01). T0: 0 hours; T1: 1 hour; T2: 2 hours; T3: 3 hours; T4: 4 hours; T5: 5 hours; T6: 6 hours.

SDS-PAGE of soluble proteins from insect meals and residual substrates
The extracted proteins were 0.68 µg/µL for residual substrates and 2.215 µg/µL for insect meals, respectively.
One-dimensional denaturing gel revealed clearly separate protein bands, although there were qualitative differences in the intensity of the bands. Compared to the marker, the polypeptide profile for residual substrates ranged from 5 to 75 kDa, and 50–250 kDa for insect meals ().
Figure 4. SDS-PAGE of soluble proteins extracted from insect meals and residual substrates at day 14 of the control (CTRL) and treatment (TRT) groups. (A) Soluble proteins extracted from insect meals. M: Molecular weight marker (Precision Plus Protein, Bio-Rad, Hercules, CA, USA); Lane 1: 8 µg of soluble proteins of the CTRL group; Lane 2: 4 µg of soluble proteins of the CTRL group; Lane 3: 8 µg of soluble proteins of the TRT group; Lane 4: 4 µg of soluble proteins of the TRT group. (B) Soluble proteins extracted from residual substrates after 14 days of trial. M: Molecular weight marker (Precision Plus Protein, Bio-Rad, Hercules, CA, USA); Lane 1: 5 µg of soluble proteins of the CTRL group; Lane 2: 2.5 µg of soluble proteins of the CTRL group; Lane 3: 5 of soluble proteins of the TRT group; Lane 4: 2.5 of soluble proteins of theTRT group.

Discussion
Insects are considered excellent bioconverters that can transform a wide range of substrates into high-quality biomass (Fowles and Nansen Citation2020; Ites et al. Citation2020; Bordiean et al. Citation2022). In this study, we have shown that wheat bran used as a growing substrate (a by-product of the milling industry commonly used in animal feed as a source of fibre) supplemented with a hydration source with lactoferrin as a functional ingredient could result in insect meals with higher inhibitory activity. We used lactoferrin to investigate the effects of hydration source and substrate interaction on the growth and functional properties of reared insects. The small amount of lactoferrin did not affect the costs of production significantly and it can be used routinely. In particular, bovine lactoferrin is priced on the European market between €100 and €3200 per kilogram, depending on its intended use and level of purity. In addition, given that the substrate in which insects are reared influences the performance and the functional properties of the animals, the ingredient to administer to the insects could be selected specifically to reduce the costs of farming thereby enhancing the final yield and functional characteristics of insect meal obtained. Considering the encouraging results, it would be interesting to evaluate the interaction of lactoferrin with other types of substrates.
Although insect rearing is relatively recent, and optimal growing conditions are still not well-defined, the environment, diet and hydration, can directly influence animal performance and the nutritional characteristics of the final product (Antonopoulou et al. Citation2022). The growth performance, chemical composition and functional properties of edible insects also depend on the species and developmental stage (Meyer-Rochow et al. Citation2021).
We evaluated the impact of a hydration source - a water solution containing 12.7% of lactoferrin - on the insects’ growth, and the nutritional and functional value of the T. molitor meal obtained. When the macro- and micro-climatic conditions recommended by the Italian Cricket Farm (the company involved in this study) for rearing T. molitor larvae are followed, the larval stage is completed in two months. During the first phase, there is exponential growth, while in the second phase the growth rate decreases, and the chemical composition (e.g. chitin and protein content) of the larvae can be modified as they prepare for the pupae step. During the last two weeks of the larval phase, the nutritional and functional characteristics of the final product can be influenced by the rearing conditions (Yu et al. Citation2021). This is why we focused on this period in order to valorise the effect of lactoferrin on functional properties.
We found no significant differences in growth performance between groups during the 14 days of the trial. Wheat bran used as a growing substrate, alone or supplemented with a functional source of hydration represented by lactoferrin, did not influence the growth and feed conversion efficiency of T. molitor larvae. To the best of our knowledge, no other studies have assessed hydration with lactoferrin supplementation on the growth performance of larvae.
Although the larvae were maintained under the same environmental conditions, with the same moistening and feeding protocol, except for the presence of lactoferrin in the water administered to TRT, our results demonstrated that the larvae of TRT group showed a higher survival rate than CTRL group.
In line with Bordiean et al. (Citation2022), we found that the survival rate is influenced by the protein content of the growing substrate. In our study, the protein concentration of the TRT growing substrate was comparable to the substrate of the CTRL group, but the hydration with a protein source in small doses may have positively influenced the reduction in larval mortality, particularly given the antimicrobial activity of lactoferrin and derived bioactive peptides (Malone et al. Citation2022). We found that the insect meals from larvae fed with wheat bran had a lighter colour than insects that consumed the same substrate hydrated with the lactoferrin solution.
Lee et al. (Citation2008) reared larvae on substrates with low-quality protein made up of zein (from corn), and found that such substrates exhibited less melanisation of cuticles than casein used as a high-quality protein source. Their results demonstrated a positive correlation between the quantity of protein ingested by insects and the cuticular melanisation.
Stronger melanisation is known to directly increase the immune function through the stimulation of the prophenoloxidase system (Binggeli et al. Citation2014). Therefore, since both our groups received the same growing substrate with a protein content of approximately 15%, these conditions of growing, equal for both groups, could indicate that it was the hydration with lactoferrin that positively influenced the melanisation of the larvae cuticles in the TRT group thus making the insects more resistant to pathogens with a higher chance of survival. Resuming, larvae given supplementation of high-quality protein as a hydration source had a higher survival rate than larvae that received only water, conferring a darker colour in the resulting meal probably due to increased melanisation of cuticles. As reported by Lee et al. (Citation2008), balancing nitrogen quantity available for larvae, the quality of administered proteins is a crucial factor able to determine the survival of insects. Obtained findings suggest that the administration of a functional hydration source through the supplementation of approximately 10 mg of pure lactoferrin in 14 days may have influenced the nitrogen availability for melanin production and immune function, resulting in lower mortality and darker colour of meals compared to the insects of control group.
Other authors have found that higher survival rates directly correlated with a higher protein content (21–26% of CP on DM) administered to insects (van Broekhoven et al. Citation2015; Oonincx et al. Citation2015), could also be related to the melanisation process. In addition, the antimicrobial properties of lactoferrin act against a variety of pathogens, including bacteria, viruses, and parasites, and lactoferrin is recognised for its immunomodulatory and antioxidant activities (Garas et al. Citation2016). Lactoferrin thus seems to have a direct protective potential due to its antimicrobial effect, and it is likely that its supplementation in the water supply could stimulate insect melanisation.
In our study, residual substrates collected at day 14 were analysed in terms of chemical composition and showed significant differences for the content of dry matter, ash, lipids. Since residual substrates are largely composed of insects faeces, the differences in chemical composition of residual substrates could be due to a different activation of gut digestive enzymes and microbial-derived enzymes present in the larvae after lactoferrin administration (Li et al. Citation2013). However, the antioxidant activity of residual substrates could be exerted by the presence of undigested substrate, and the presence of insect faeces and cuticles. van Broekhoven et al. (Citation2015) observed that larvae fed a diet rich in protein content excreted more uric acid than larvae reared with a substrate with a low protein concentration (∼10% CP), thus increasing the uric acid level in faeces. In fact, insect faeces are mainly made up of uric acid, the end-product of purine metabolism that is excreted predominantly through urine in mammals which is an excellent scavenger (Glantzounis et al. Citation2005; Weihrauch and O'Donnell Citation2021).
Cuticles contain chitin, a major component of the insects’ exoskeleton which is also present in minor quantities in the peritrophic matrix (Zhu et al. Citation2016). This glycoprotein modulates, enhances and promotes physiological functions of insects, such as the antioxidant effect (Ngo and Kim Citation2014; Güneş et al. Citation2018). The reduction in insect mortality could be due to the protective effect of lactoferrin in the TRT hydration showing a higher bioactivity of lactoferrin. These data thus suggest an enrichment of the content of antioxidant compounds in the growing substrate, likely due to uric acid and cuticles present in the larvae rearing environment (Weihrauch and O'Donnell Citation2021).
In our study, differences were observed in the protein and lipid content of insect meals (on a dry matter basis) in the CTRL and TRT groups. The crude protein content of the insect meals obtained was calculated using a conversion factor for nitrogen of 4.76 to exclude the chitin content (Janssen et al. Citation2017). The control and treatment groups were 44.02 ± 3.12% (on DM) and 46.66 ± 1.34% (on DM) of CP, respectively, which are in line with protein contents reported for T. molitor larvae (CP: 43-51% on DM) (Hong et al. Citation2020). This result confirms previous studies showing that T. molitor meal could replace soybean meal (CP 44% on FM), and be a partial substitute for fishmeal (National Research Council Citation2012). In addition, the higher percentage of protein in the TRT group compared to the CTRL group demonstrated how the substrate affects the chemical characterisation of insects, even when the lactoferrin dose was very low and was administered through a method not jet tested to date. Even if the amino acid profile was not evaluated in this study, as reported by Janssen et al. (Citation2017), T. molitor can be considered a valid protein source with a balanced amino acid profile suitable for animal nutrition.
T. molitor larvae could thus become a sustainable protein source for livestock farming. The protein content of insect meals grown on wheat bran hydrated with lactoferrin solution compared to the control group suggests that insects are able to adjust the protein content by exploiting the available nutrients depending on their specific physiology. Although some studies have reported that substrate with an increased protein content could influence the body composition and growth performance (van Broekhoven et al. Citation2015), insects are able to adapt their final protein content by exploiting different growing substrates and thus reaching a similar final nutritional composition even when received different diets in terms of nutrients (Pinotti and Ottoboni Citation2021). Nevertheless, insects exhibit a significant plasticity in protein and lipid accumulation according to the diet administered, the developmental stage (larval or adult) and environmental conditions (Kröncke et al. Citation2023). Our results demonstrated that the protein and lipid content of insect meals could be influenced by the administration of different hydration sources due to their strong relationship in the insects composition.
Using bioactive ingredients in mealworm larvae rearing may lead to improve functional properties of insect meals (Andreadis et al. Citation2021). It is still unclear which technologies are the best at safeguarding the functional properties of larvae during the storage and subsequent processing stages. There is still limited information on the most appropriate processing system for drying insects in order to maintain the stability of their nutritional components. In our experiment, a microwave was used during meal production, which is efficient for drying insects according to Keil et al. (Citation2022). Mancini et al. (Citation2021) reported that unlike other techniques, microwaving does not affect the nutritional components.
In this study, the supplementation of lactoferrin during the water supply showed the increased capacity of insect meal extracts to inhibit the O138 E. coli growth, a representative model of gastrointestinal disorders. Lactoferrin is a glycoprotein of the transferrin family that binds to iron and has antimicrobial and antibacterial properties. Lactoferrin also regulates the immune response, and protects against infection and septic shock in animals (Lu et al. Citation2022).
E. coli is a gram-negative bacterium belonging to the Enterobacteriaceae family that harbours different pathogenic strains, and these strains can lead to multifactorial diseases in livestock farming, leading to economic losses and antibiotic use (Rossi et al. Citation2021). Results from E. coli inhibitory activity have demonstrated that the TRT meal extracts, at concentrations of 1:4 and from 3 to 6 h of incubation, inhibited the growth of O138 E. coli with compared to the CTRL group meal extract. Several studies have also confirmed that insect meals possess antimicrobial activity (Wu et al. Citation2018; Veldkamp et al. Citation2022).
The delayed inhibition effect of the different extracts could be due to the time required for the antimicrobial compounds contained in insect meals to exert their disrupting activity against bacteria. These data suggest that lactoferrin positively influences the bioactive compounds in insects. The delayed inhibition effect of the different treatment extracts may be due to the time required for the antimicrobial compounds contained in insects to act and disrupt the integrity of the bacterial cell wall. This inhibitory activity implies a potential functional role for the insect meals obtained, and their supplementation in animal feed could enhance animal health thus decreasing pathology occurrence and antibiotic use.
In terms of swine farming, the dietary inclusion of lactoferrin could be difficult due to the high doses required to achieve a sufficient antimicrobial effect, and the cost of the additive itself. The use of insects grown with functional hydration may thus be an interesting source of antimicrobial compounds. Finally, one of the most important future goals for the development of a sustainable livestock, will concern the development of specific functional diets that improve animal health, in compliance with the principles of One Health.
The SDS-page analysis showed a similar composition in terms of the soluble polypeptides extracted from the growing substrates and insect meals of the CTRL and the TRT groups. This suggests that hydration with the lactoferrin solution did not influence the protein profile of insects, thus confirming that they are able to bioconvert and metabolise dietary ingredients even in low doses (Pinotti and Ottoboni Citation2021).
Conclusions
T. molitor larvae rearing can be considered an excellent sustainable production according to a One Health approach. We found that when a lactoferrin solution was used as a functional hydration source in T. molitor larvae, it enhanced the larvae survival rate and did not impair the growth performance. The nutritional characteristics of the insect meals were also mainly influenced by the substrate and growth conditions as reported in other studies. In vitro evaluation of bacterial growth inhibition showed that insect meals hydrated with lactoferrin showed a higher antimicrobial capacity compared to the control group hydrated only with water.
The antioxidant activity of residual substrates of TRT group was higher than in the residual substrates in the CTRL group. The administration of innovative ingredients as hydration sources could thus be beneficial in the production of functional T. molitor meal with a high content of bioactive molecules. The use of functional insect meals in animal nutrition has the potential to enhance animal welfare and reduce pathologies, thus decreasing antibiotic treatments. It is important to underline that a large-scale evaluation of innovative hydration system could produce a different impact on insects rearing, however our findings may be interesting for increasing the survival rate and functionality of insects in this innovative farming system.
Further studies are needed to evaluate different strategies for applying functional ingredients in insect rearing.
Acknowledgments
The authors acknowledge the Italian Cricket Farm for providing the rearing facility, substrates and insects for the in vivo trial.
Authors’ contributions
Conceptualisation, L.R.; methodology, I.F., M.D., and B.P; formal analysis, I.F., M.D., B.C. and B.P.; investigation, I.F., M.D., S.M.; data curation, I.F.; writing—original draft preparation, I.F; writing—review and editing, M.D. and L.R.; visualisation, I.F.; supervision, L.R.; project administration, L.R.; funding acquisition, L.R. All authors have read and agreed to the published version of the manuscript.
Discloure statement
Dr. Stefano Magnaghi works as Chief of the Research and Development department for Italian Cricket Farm S.r.l. Other authors declare no conflict of interest.
No potential conflict of interest was reported by the author(s)
Data availability statement
All data are available within the manuscript.
References
- Andreadis SS, Panteli N, Mastoraki M, Rizou E, Stefanou V, Tzentilasvili S, Sarrou E, Chatzifotis S, Krigas N, Antonopoulou E. 2021. Towards functional insect feeds: agri-food by-products enriched with post-distillation residues of medicinal aromatic plants in Tenebrio molitor (coleoptera: tenebrionidae) breeding. Antioxidants. 11(1):68. doi: 10.3390/ANTIOX11010068/S1.
- Antonopoulou E, Panteli N, Feidantsis K, Mastoraki M, Koutsogeorgiou EI, Grivaki E, Papagrigoriou T, Christias SP, Chatzifotis S, Lazari D, et al. 2022. Carob (Ceratonia siliqua) as functional feed is beneficial in Yellow Mealworm (Tenebrio molitor) Rearing: evidence from growth, antioxidant status and cellular responses. Antioxidants. 11(9):1840. doi: 10.3390/ANTIOX11091840/S1.
- AOAC International. 2019. Official Methods of Analysis, 21nd Edition. Washington, DC: AOAC.
- Baek M, Kim MA, Kwon YS, Hwang JS, Goo TW, Jun M, Yun EY. 2019. Effects of processing methods on nutritional composition and antioxidant activity of mealworm (Tenebrio molitor) larvae. Entomol Res. 49(6):284–293. doi: 10.1111/1748-5967.12363.
- Binggeli O, Neyen C, Poidevin M, Lemaitre B. 2014. Prophenoloxidase activation is required for survival to microbial infections in Drosophila. PLOS Pathog. 10(5):e1004067. doi: 10.1371/JOURNAL.PPAT.1004067.
- Bonjoch NP, Tamayo PR. 2001. Protein content quantification by Bradford method. In: Reigosa Roger MJ, editor. Handbook of Plant Ecophysiology Techniques. Dordrecht: Springer. p. 283–295. doi: 10.1007/0-306-48057-3_19.
- Bordiean A, Krzyżaniak M, Stolarski MJ. 2022. Bioconversion potential of agro-industrial byproducts by Tenebrio molitor—long-term results. Insects. 13(9):810. doi: 10.3390/INSECTS13090810.
- Deruytter D, Coudron CL, Claeys J. 2021. The influence of wet feed distribution on the density, growth rate and growth variability of Tenebrio molitor. J Insects Food Feed. 7(2):141–149. doi: 10.3920/JIFF2020.0049.
- Dell’Anno M, Sotira S, Rebucci R, Reggi S, Castiglioni B, Rossi L. 2020. In vitro evaluation of antimicrobial and antioxidant activities of algal extracts. Ital J of Anim Sci. 19(1):103–113. doi: 10.1080/1828051X.2019.1703563.
- Egerton S, Wan A, Murphy K, Collins F, Ahern G, Sugrue I, Busca K, Muller N, Whooley J, McGinnity P, et al. 2020. Replacing fishmeal with plant protein in Atlantic salmon (Salmo salar) diets by supplementation with fish protein hydrolysate. Sci Rep. 10(1):4194. doi: 10.1038/s41598-020-60325-7.
- Food and Agriculture Organization of the United Nations (FAO). 2018. Transforming the livestock sector through the Sustainable Development Goals. Rome, Italy: FAO. doi: 10.4060/ca1201en.
- Food and Agriculture Organization of the United Nations (FAO). 2021. The need for guidance on alternative proteins highlighted to codex Alimentarius Commission | Sustainable and circular bioeconomy for food systems transformation | Food and Agriculture Organization of the United Nations. https://www.fao.org/in-action/sustainable-and-circular-bioeconomy/resources/news/details/en/c/1459357/.
- Fowles TM, Nansen C. 2020. Insect-based bioconversion: value from food waste. Food waste management: solving the Wicked Problem. 1st Edition. Cham: Palgrave Macmillan. p. 321–346. doi: 10.1007/978-3-030-20561-4_12/FIGURES/3.
- Frazzini S, Scaglia E, Dell’Anno M, Reggi S, Panseri S, Giromini C, Lanzoni D, Sgoifo Rossi CA, Rossi L. 2022. Antioxidant and antimicrobial activity of algal and cyanobacterial extracts: an In Vitro Study. Antioxidants. 11(5):992. doi: 10.3390/ANTIOX11050992/S1.
- Garas LC, Feltrin C, Hamilton MK, Hagey JV, Murray JD, Bertolini LR, Bertolini M, Raybould HE, Maga EA. 2016. Milk with and without lactoferrin can influence intestinal damage in a pig model of malnutrition. Food Funct. 7(2):665–678. doi: 10.1039/c5fo01217a.
- Glantzounis G, Tsimoyiannis E, Kappas A, Galaris D. 2005. Uric acid and oxidative stress. Curr Pharm Des. 11(32):4145–4151. doi: 10.2174/138161205774913255.
- Güneş E, Nizamlioğlu HF, Aydin H. 2018. Antioxidant activity of chitin obtained from the insect. J Int Environ Appl Sci. 13(4):213–216. https://dergipark.org.tr/en/pub/jieas/issue/42086/481991.
- Hawkey KJ, Lopez-Viso C, Brameld JM, Parr T, Salter AM. 2021. Insects: a potential source of protein and other nutrients for feed and food. Annu Rev Anim Biosci. 9(1):333–354. doi: 10.1146/annurev-animal-021419-083930.
- Hong J, Han T, Kim YY. 2020. Mealworm (Tenebrio molitor Larvae) as an alternative protein source for monogastric animal: a Review. Animals. 10(11):2068. doi: 10.3390/ANI10112068.
- van Huis A, Van Itterbeeck J, Klunder H, Mertens E, Halloran A, Muir G, Vantomme P. 2013. Edible insects: future prospects for food and feed security. Rome. https://www.fao.org/3/i3253e/i3253e.pdf.
- Ites S, Smetana S, Toepfl S, Heinz V. 2020. Modularity of insect production and processing as a path to efficient and sustainable food waste treatment. J Clean Prod. 248:119248. doi: 10.1016/j.jclepro.2019.119248.
- Jahan M, Kracht S, Ho Y, Haque Z, Bhattachatyya BN, Wynn PC, Wang B. 2017. Dietary lactoferrin supplementation to gilts during gestation and lactation improves pig production and immunity. PLOS One. 12(10):e0185817. doi: 10.1371/JOURNAL.PONE.0185817.
- Jamaa ZIM, Lhomme P, Takhim A, Sarehane M, Bouharroud R. 2021. Growth performance, conversion and survival rates of Tenebrio molitor (Coleoptera: tenebrionidae) reared on various livestock diets. Moroccan J Agri Sci. 2(4):161–164. https://www.researchgate.net/publication/357168518.
- Janssen RH, Vincken JP, Van Den Broek LAM, Fogliano V, Lakemond CMM. 2017. Nitrogen-to-protein conversion factors for three edible insects: tenebrio molitor, Alphitobius diaperinus, and Hermetia illucens. J Agric Food Chem. 65(11):2275–2278. doi: 10.1021/ACS.JAFC.7B00471.
- Jantzen da Silva Lucas A, Menegon de Oliveira L, da Rocha M, Prentice C. 2020. Edible insects: an alternative of nutritional, functional and bioactive compounds. Food Chem. 311:126022. doi: 10.1016/J.FOODCHEM.2019.126022.
- Keil C, Grebenteuch S, Kröncke N, Kulow F, Pfeif S, Kanzler C, Rohn S, Boeck G, Benning R, Haase H. 2022. Systematic studies on the antioxidant capacity and volatile compound profile of Yellow Mealworm Larvae (T. molitor L.) under different drying regimes. Insects. 13(2):166. doi: 10.3390/INSECTS13020166/S1.
- Kröncke N, Benning R. 2022. Self-selection of feeding substrates by Tenebrio molitor larvae of different ages to determine optimal macronutrient intake and the influence on larval growth and protein content. Insects. 13(7):657. doi: 10.3390/insects13070657.
- Kröncke N, Neumeister M, Benning R. 2023. Near-infrared reflectance spectroscopy for quantitative analysis of fat and fatty acid content in living Tenebrio molitor larvae to detect the influence of substrate on larval composition. Insects. 14(2):114. doi: 10.3390/insects14020114.
- Lee KP, Simpson SJ, Wilson K. 2008. Dietary protein-quality influences melanization and immune function in an insect. Funct Ecol. 22(6):1052–1061. doi: 10.1111/j.1365-2435.2008.01459.x.
- Li L, Zhao Z, Liu H. 2013. Feasibility of feeding yellow mealworm (Tenebrio molitor L.) in bioregenerative life support systems as a source of animal protein for humans. Acta Astronaut. 92(1):103–109. doi: 10.1016/j.actaastro.2012.03.012.
- Lu Y, Li Y, Lin Y, Wang J, Ma M, Guo H. 2022. Effects of heat treatment and simulated digestion on the properties and osteogenic activity of bovine lactoferrin. LWT. 162:113475. doi: 10.1016/j.lwt.2022.113475.
- Luna-Castro S, Ceballos-Olvera I, Benavides-González F, Blanco-Martínez Z, Sánchez-Martínez G, Vázquez-Sauceda M de la L, de la Garza M. 2022. Bovine lactoferrin in fish culture: current research and future directions. Aquac Res. 53(3):735–745. doi: 10.1111/are.15621.
- Malone A, Clark RF, Hoskin DW, Power Coombs MR. 2022. Regulation of macrophage-associated inflammatory responses by species-specific lactoferricin peptides. Front Biosci. 27(2):43. doi: 10.31083/J.FBL2702043/2768-6698-27-2-043/FIG9.JPG.
- Mancini S, Mattioli S, Paolucci S, Fratini F, Dal Bosco A, Tuccinardi T, Paci G. 2021. Effect of cooking techniques on the in vitro protein digestibility, fatty acid profile, and oxidative status of Mealworms (Tenebrio molitor). Front Vet Sci. 8:675572. doi: 10.3389/FVETS.2021.675572/BIBTEX.
- Manzanares P, Salom JB, García-Tejedor A, Fernández-Musoles R, Ruiz-Giménez P, Gimeno-Alcañíz JV. 2015. Unraveling the mechanisms of action of lactoferrin-derived antihypertensive peptides: ACE inhibition and beyond. Food Funct. 6(8):2440–2452. doi: 10.1039/C5FO00580A.
- McCluney KE, Date RC. 2008. The effects of hydration on growth of the house cricket, Acheta domesticus. J Insect Sci. 8(32):1–9. doi: 10.1673/031.008.3201.
- Meyer-Rochow VB, Gahukar RT, Ghosh S, Jung C. 2021. Chemical composition, nutrient quality and acceptability of edible insects are affected by species, developmental stage, gender, diet, and processing method. Foods. 10(5):1036. doi: 10.3390/FOODS10051036.
- Mokrzycki WS, Tatol M. 2011. Color difference ΔE : a survey. Mach Graph Vis. 20(4):383–411. https://www.infona.pl//resource/bwmeta1.element.baztech-acbebd17-40d3-40db-860d-58d8876202d7.
- National Research Council. 2012. Nutrient Requirements of Swine: eleventh Revised Edition. Washington, DC: The National Academies Press. doi: 10.17226/13298.
- Ngo DH, Kim SK. 2014. Antioxidant effects of chitin, chitosan, and their derivatives. Adv Food Nutr Res. 73:15–31. doi: 10.1016/B978-0-12-800268-1.00002-0.
- Niaz B, Saeed F, Ahmed A, Imran M, Maan AA, Khan MKI, Tufail T, Anjum FM, Hussain S, Suleria HAR. 2019. Lactoferrin (LF): a natural antimicrobial protein. Int J Food Prop. 22(1):1626–1641. doi: 10.1080/10942912.2019.1666137.
- Oonincx DGAB, Van Broekhoven S, Van Huis A, Van Loon JJA. 2015. Feed conversion, survival and development, and composition of four insect species on diets composed of food by-products. PLOS One. 10(12):e0144601. doi: 10.1371/JOURNAL.PONE.0144601.
- Parrini S, Aquilani C, Pugliese C, Bozzi R, Sirtori F. 2023. Soybean replacement by alternative protein sources in pig nutrition and its effect on meat quality. Animals. 13(3):494. doi: 10.3390/ani13030494.
- Paula EM, Broderick GA, Danes MAC, Lobos NE, Zanton GI, Faciola AP. 2018. Effects of replacing soybean meal with canola meal or treated canola meal on ruminal digestion, omasal nutrient flow, and performance in lactating dairy cows. J Dairy Sci. 103(2):1463–1471. doi: 10.3168/JDS.2017-13392.
- Pinotti L, Ottoboni M. 2021. Substrate as insect feed for bio-mass production. J Insects Food Feed. 7(5):585–596. doi: 10.3920/JIFF2020.0110/SUPPL_FILE/JIFF2020.0110_ESM.PDF.
- Reggi S, Giromini C, Dell’Anno M, Baldi A, Rebucci R, Rossi L. 2020. In Vitro digestion of chestnut and Quebracho tannin extracts: antimicrobial effect, antioxidant capacity and cytomodulatory activity in swine intestinal IPEC-J2 Cells. Animals. 10(2):195. doi: 10.3390/ANI10020195.
- Rossi L, Turin L, Alborali GL, Demartini E, Filipe JFS, Riva F, Riccaboni P, Scanziani E, Trevisi P, Dall’Ara P, et al. 2021. Translational approach to induce and evaluate verocytotoxic E. coli O138 based disease in piglets. Animals . 11(8):2415. doi: 10.3390/ANI11082415.
- Sfeir RM, Dubarry M, Boyaka PN, Rautureau M, Tomé D. 2004. The mode of oral bovine lactoferrin administration influences mucosal and systemic immune responses in mice. J Nutr. 134(2):403–409. doi: 10.1093/JN/134.2.403.
- Shin CS, Kim DY, Shin WS. 2019. Characterization of chitosan extracted from Mealworm Beetle (Tenebrio molitor, Zophobas morio) and Rhinoceros Beetle (Allomyrina dichotoma) and their antibacterial activities. Int J Biol Macromol. 125:72–77. doi: 10.1016/J.IJBIOMAC.2018.11.242.
- van Broekhoven S, Oonincx DGAB, van Huis A, van Loon JJA. 2015. Growth performance and feed conversion efficiency of three edible mealworm species (Coleoptera: tenebrionidae) on diets composed of organic by-products. J Insect Physiol. 73:1–10. doi: 10.1016/J.JINSPHYS.2014.12.005.
- Veldkamp T, Dong L, Paul A, Govers C. 2022. Bioactive properties of insect products for monogastric animals – a review. J InsFood Feed. 8(9):1027–1040. doi: 10.3920/JIFF2021.0031.
- Weihrauch D, O'Donnell MJ. 2021. Mechanisms of nitrogen excretion in insects. Curr Opin Insect Sci. 47:25–30. doi: 10.1016/J.COIS.2021.02.007.
- Wu Q, Patočka J, Kuča K. 2018. Insect antimicrobial peptides, a mini review. Toxins. 10(11):461. doi: 10.3390/TOXINS10110461.
- Yu X, He Q, Wang D. 2021. Dynamic analysis of major components in the different developmental stages of Tenebrio molitor. Front Nutr. 8:689746. doi: 10.3389/fnut.2021.689746.
- Zhu KY, Merzendorfer H, Zhang W, Zhang J, Muthukrishnan S. 2016. Biosynthesis, turnover, and functions of chitin in insects. Annu Rev Entomol. 61(1):177–196. doi: 10.1146/ANNUREV-ENTO-010715-023933.