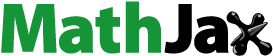
Abstract
The effects of dietary supplementation with Rumex nervosus leaves (RNL) on fatty acid (FAs) indices, organoleptic evaluation, performance index, and feed efficiency were investigated. This study consists of five treatments. Broilers in treatments 1–4 received basal feed plus RNL at doses of 0, 1, 3, and 5 g/kg, while broilers in treatment 5 received basal feed plus the antibiotic Colimex. On day 34, FAs profile and organoleptic assessment were evaluated, and performance index and feed efficiency were determined for the entire experimental period. Results showed that FAs profile of the breast meat was affected by treatments with varying degrees (p˂0.05). The 1 g RNL resulted in the highest eicosapentaenoic acid + docosahexaenoic acid, which resulted in the highest meat lipid quality, the highest elongation index, improved organoleptic characteristics, and tended to improve performance index, feed efficiency, and nutritional index. The 5 g RNL treatment reduced total saturated fatty acids (ƩSFAs), omega-6/omega-3 ratio, especially linoleic acid/α-linolenic acid ratio, alanine aminotransferase, and increased total omega-3 fatty acids, unsaturation index, and Δ9-desaturase. In conclusion, RNL can be introduced to broiler diets, especially at an incorporation rate of 1 g, and it promotes broiler health and performance because it contains phytoconstituents that can alter the content and indices of FAs meat and improve storability and sensory quality.
Oxidative chemicals increase lipid peroxidation and decrease sensory quality of broiler meat.
As antioxidants, natural RNL inhibit oxidative degradation and prevent lipid peroxidation.
RNL contains phytoconstituents that increase the fatty acid content and oxidative stability of meat.
Fat deposition and associated markers in breast meat differed between low and high RNL levels.
RNL improved nutritional index, sensory quality, performance index, and feed efficiency.
HIGHLIGHTS
Introduction
To meet the increasing customer demand for broiler meat, intensive feeding with high stocking density has developed rapidly in the last 50 years. However, under these circumstances, broilers are more susceptible to stress factors such as pathogenic diseases and oxidative stress. This stress has negatively affected meat quality (Yang et al. Citation2019; Kwiecień et al. Citation2021; Yu et al. Citation2021). As consumers desire safer, tastier, and healthier meat, meat flavour and organoleptic quality have become increasingly important (Asante-Addo Citation2020; Li et al. Citation2023). Chicken breast meat is the most popular among consumers. Consumers are particularly interested in the profile of FAs, which has become the focus of studies because of its direct link to circulatory disease (Jachimowicz et al. Citation2022).
Polyunsaturated fatty acids (PUFAs), especially omega-3 polyunsaturated fatty acids (Σn-3PUFAs), are considered as flavour carriers of fats and functional components to alleviate many lifestyle diseases such as cardiovascular risk, myocardial infarction, coronary artery disease, cerebral stroke, autoimmune diseases, and some cancers (Attia Citation2003; Oppedisano et al. Citation2020; Untea et al. Citation2023). Therefore, the consumption of Σn-3PUFAs plays an important role in the health and proper functioning of the organism through the maintenance and development of various organs, especially the brain. The positive effects of Σn-3PUFAs on human health are well known. Humans are poor at synthesising Σn-3PUFAs on their own; therefore, these compounds should be supplied through the diet (Attia et al. Citation2017; Cartoni Mancinelli et al. Citation2022).
Nutraceutical feed additives are commonly used to protect chickens from pathogenic diseases, but some also have growth-promoting effects (Rahman et al. Citation2022; Islam and Nishibori Citation2023). Antibiotic supplementation has benefits by being used as a subtherapy to promote broiler performance and health but has been withdrawn due to the high consumer demand for antibiotic-free poultry products and establishment of resistant bacteria (Smith Citation2019; Rahman et al. Citation2022). The European Union’s ban on the use of antibiotics as growth promoters has fueled the search for alternative feed additives in broiler production (Smith Citation2019). Due to the increasing use of antibiotics in broiler production, antibiotic-resistant bacteria have emerged and can be transmitted to humans through the food chain, causing major health problems (Islam and Nishibori Citation2023). Antibiotic resistance is a major public health concern because it reduces the effectiveness of antibiotics and makes it difficult to treat bacterial diseases (Al-Garadi et al. Citation2022). Antibiotics or their substitutes improve animal growth and health via a variety of mechanisms, including inhibiting subclinical infections, improving nutrient uptake and utilisation by thinning polarised epithelium and reducing growth-inhibiting microbial metabolites in the intestines, and increasing nutrient availability by reducing microbes sharing nutrients in the intestines (Rahman et al. Citation2022).
The use of natural herbs or their secondary metabolites to improve the organoleptic quality of meat through antimicrobial and antioxidant effects is a worldwide focus of investigation (Aati et al. Citation2019; Wu and Zhou Citation2021). Botanicals significantly increased the content of UFAs in broiler meat (Waheed et al. Citation2018; Attia et al. Citation2022), indicating their role in enhancing meat quality.
Rumex nervosus is a traditional medicinal plant of the Polygonaceae family. In vitro and in vivo studies have shown that Rumex nervosus contains many bioactive products such as gallic acid that possess numerous biological and pharmacological activities for instance antioxidant, antimicrobial, anti-inflammatory, and immunomodulatory effects (Qasem et al. Citation2020; Gemechu et al. Citation2021). Therefore, the hypothesis of the study is that RNL may contribute to human health by improving the desirable FAs and sensory properties of the pectoral muscle through its action. However, seemingly, there are no studies on the effects of short or long-term RNL supplementation on the taste of chicken breast meat and its lipid quality. However, a number of studies on the use of phytogenic or bioactive metabolites in broiler diets show encouraging results in improving FAs profile health indices and sensory properties of breast meat (Jachimowicz et al. Citation2022; Untea et al. Citation2023). In contrast, the researches with RNL does not yet provide evidence for the use of this shrub in this regard. The quality, nutritional, and metabolic indices may serve as a secondary surrogate for costly laboratory work following the indices of a fasting lipid panel that measures plasma triglycerides and lipoprotein cholesterol distribution in relation to a patient’s risk for cardiovascular disease. This study seeks to fill knowledge gaps on the incorporation of RNL into broiler diets and the associated health indices for FAs profile and sensory attributes.
RNL-based health-promoting broiler diets will fill research gaps and enhance the value of this research through futurity guidelines for profitable applications of this healthy product in broiler diets. Thus, the aim of this study was to investigate the effects of RNL supplementation on organoleptic properties and FAs profiles indexing of broiler breast meat, serum safety evaluation, performance index, and feed efficiency.
Materials and methods
Ethical approval
The experimental study was approved by King Saud University’s (KSU) Scientific Research Ethics Committee (Approval No. KSU-SE-20-44).
Birds and dietary treatments
A150 one-day-old Ross-308 chicks (n = 150) were randomly assigned to one of five treatments. In the first four treatments, broilers received a basal diet supplemented with RNL powder at doses of 0, 1, 3, and 5 g/kg, whereas in the five treatments in the ‘antibiotic group,’ birds received a basal diet supplemented with Colimex powder (colistin and amoxicillin at doses of 150 and 200 mg/kg, respectively). Colimex was used in this study following (Elbadawy and Aboubakr Citation2017). Some studies study natural herb such as Daniellia oliveri leaf extracts (Alagbe 2021), Syzygium aromaticum seed (Suliman et al. Citation2021), Prosopis africana essential oil (Alagbe 2023) as an alternative to antibiotic feed additives in broiler chicken diets on the studied properties. Other study used biological stimulants (probiotics (Mogotlane et al. Citation2023; Islam and Nishibori Citation2023; Al-Khalaifa et al. Citation2019) or prebiotics (Al-Khalaifa et al. Citation2019)) used as an alternative to antibiotics. Each treatment group consisted of five replicates and six chicks per replicate (3 males and 3 females). Birds were reared in an environmentally controlled, heated room in the KSU Experimental Poultry Research Unit, with a lighting schedule of 23 L: 1 D h. Feed and water were available ad libitum throughout the experiment. RNL was gathered, ground, and mixed in the lowest levels (0.1–0.5% RNL) on top of broiler basal diet. The control diet was formulated as a mash (Table ).
Table 1. Feed composition of broilers diets.
Phytochemistry, total phenolic content and macronutrients determination of RNL
Total phenol concentration in a RNL extract was quantified by the Folin-Ciocalteu colorimetric manner, and absorbance was determined using a spectrophotometer at 760 nm, based on Al-Garadi et al. (Citation2022). The assays were frequent twice, a calibration curve was constructed using gallic acid, and the results were expressed in g gallic acid equivalent per mg. High performance liquid chromatography (HPLC) was used to isolate, separate, and dose the chemical constituents of the RNL extract combination, as indicated by Qaid et al. (Citation2021). The chemical composition of RNL was measured by gas chromatography-mass spectrometry assay (GC-MS), and the macronutrients was determined by proximate analysis as described by Al-Garadi et al. (Citation2022).
Fatty acid content of the pectoral meat
On day 34 of the birds, one male bird per cage (n = 5 per treatment) was randomly selected and slaughtered for sampling of the pectoralis major muscle. The method of slaughter was as stated by Suliman et al. (Citation2021). The pectoral muscle of each chicken was frozen at −20 °C until later analysis and then thawed overnight in the refrigerator at 4 °C before analysis.
To obtain the FAs composition of the breast muscle, the frozen meat samples were freeze-dried and then ground using a grinder. Each sample was homogenised to 45 ml with a chloroform-methanol mixture (2:1, v/v). According to Agnew et al. (Citation2019), the supernatant obtained was used to prepare FAs methyl esters with methanol/sulfuric acid (95:5) and hexane mixture for 14 h and extracted by sample preparation with thin layer chromatography. The hexane extract obtained from the FAs methyl esters was used for quantification of free FAs using an Agilent 6890 GC coupled with a 5975 C Mass Selective Detector (Agilent Technologies, Palo Alto, CA, USA) equipped using a DB-5 (Agilent) and a fused silica capillary column (30 m × 0.25 mm × 0.25 μm film thickness) to detected the FAs methyl esters. The peak areas of the FAs methyl esters were calculated automatically by recording the data with an Agilent Chemstation. Mixtures were identified by comparing mass spectra with those of authentic standards and by GC retention index, characterised mixtures, and literature and library data. All quantifications were based on the compound peak areas derived from total ion current or key ion fragmentograms. FAs concentrations were expressed as a percentage of the total peaks recorded and detected for each sample. Each fatty acid value was expressed as a % of the total FAs (qualitative assessment) and average values were used to calculate ΣSFAs and ΣPUFAs (ΣMUFAs and ΣPUFAs) (Dal Bosco et al. Citation2022).
Qualitative, nutritional, and metabolic indices of fatty acids profile analysis
Qualitative indices
(Simopoulos Citation2008).
(Timmis et al. Citation2022).
(Ryman et al. Citation2017; Draycott et al. Citation2019).
(Ochi and Tsuchiya Citation2018).
Metabolic indices
These indices are calculated from the activities of the desaturating and elongating enzymes of the pectoral muscle tissue via the breast through ‘product/substrate ratio’ as described in Dal Bosco et al. (Citation2022).
whereas
(Zhang et al. Citation2007).
To assess the activities of 5- and 6-desaturase, the enzymes that catalyse the production of n-6PUFAs and n-3PUFAs from the precursors linoleic acid and α-linolenic acid, respectively (Vessby et al. Citation2002).
An activity index depends on secondary assessment of the activity of the β-oxidation pathway in breast mitochondria myocyte to estimate their energy expenditure (Failla et al. Citation2021).
Sensory assessment
For sensory evaluation of breast meat from five birds per treatment, cooked meat samples were cut into small sections of around 2 cm3. Warm samples were placed on numbered plates for assessment. Fifteen semi-trained panellists (15 men, 30–45 years old) rated juiciness, flavour, tenderness, and overall acceptability. Each panel representative received a coded meat sample, which was distributed one by one and sensory properties were explained to the testers beforehand (Suliman et al. Citation2021; Alagbe Citation2023). Before the test, panellists were not fed or smoked for 2 h. After consumption of each meat sample, each participant was given water and salted crackers to remove residual taste from the previous samples. Any type of communication between subjects during sensory evaluation was prohibited. Using an eight-point hedonic scale, the examiners assigned points for quality assessment as described in Supplementary Table S1.
Serum safety enzyme and creatinine assessment of RNL
Serum samples were collected from chickens at 34 days of age via the wing vein (1 bird per replicate). Using commercially available diagnostic kits and a spectrophotometer, serum blood samples were separated and centrifuged to measure alanine aminotransferase (ALT) and aspartate aminotransferase (AST) as indices of hepatic function and serum creatinine as an indicator of kidney function.
Performance index and feed efficiency
The following performance index formula was used to evaluate performance: ((Gain (g))2)/feed intake (g) or equal to gain/feed conversion ratio. Feed efficiency (FE) = Gain/feed intake. Body weight gain, feed intake, and feed conversion ratio (feed intake ‘g’/gain ‘g’) were calculated to determine the performance index and feed efficiency. Birds and feed were weighed on days 1 and 34.
Statistical analysis
The Statistical Analysis System (SAS (Citation2012)) was used to analyse the data, which was then submitted to one-way analysis of variance (ANOVA). The design was a completely randomised design. The equation of the statistical model is:
where Yij is the singular observation; µ is the overall mean; Ti is the treatment diets (ith) effects; and eij is the experimental error. Each treatment has five experimental units. Before beginning the analysis, the Kolmogorov–Smirnov test was employed to ensure that the chosen parameters were within a normal distribution. Means presented as Mean ± SEM and separated using the Welsch multiple comparison test at a CI of 95% (p < 0.05).
Results
Phytochemistry, total phenolic content and macronutrients of RNL
The total phenolic content in RNL extract was 171.2 mg GAE/g for RNL. As shown in Supplementary Table S2, RNL contained 1.54%, 327.33 kcal/100 g, 13.63%, 52.91%, 18.01%, 8.24%, and 5.67% lipid, nutritional value (gross energy), crude protein, total carbohydrate, crude ash (inorganic matter), total crude fibre, and moisture content, respectively, indicating that RNL can be used as a nutritional supplement.
Based on the HPLC, gallic acid was the most abundant constituent in RNL extract (Supplementary Table S3). In addition, 13 compounds were found in RNL based on the results from GC-MS, which are listed in Supplementary Table S4. These compounds in RNL are saturated fatty acids, polyunsaturated fatty acids, and other active ingredients.
Fatty acid composition
The effects of the different treatments on FAs content of breast meat are shown in Tables . Significant differences (p < 0.05) were observed in the saturated fatty acid profile (Table ) between treatment groups for C14:0 (myristic acid), palmitic acid (C16:0), stearic acid (C18:0), and arachidic acid (C20:0), ranging from 1.66 to 3.01%, 21.73 to 24.74%, 4.37 to 8.55%, and 0.00 to 0.14%, respectively. These SFAs were mainly represented by palmitic acid and stearic acid. Myristic acid content was highest in the antibiotic group compared to the other treatments. Palmitic acid was lowest in the group receiving 5 g RNL/kg diet and highest in the antibiotic group and the group receiving 3 g RNL/kg diet. Stearic acid was highest in the groups receiving 0 and 3 g RNL/kg diet (p < 0.05) and lowest in the antibiotic group. Other individual FAs did not differ significantly (p > 0.05). Compared with the other groups, the group receiving 3 g RNL/kg diet had the highest ΣSFAs and the 5 g RNL-treated group had the lowest ΣSFAs.
Table 2. Saturated fatty acids (SFAs) of breast meat of broilers at d 34 fed experimental diet.
Table 3. Monounsaturated fatty acids (MUFAs) of pectoral meat of broilers at d 34 fed experimental diet.
Table 4. Polyunsaturated fatty acids (ΣPUFAs) of pectoral meat of broilers at d 34 fed experimental diet.
Significant differences (p < 0.05) were observed between treatment groups for breast meat monounsaturated fatty acids (MUFAs; Table ) for palmitoleic acid (C16:1), 9-octadecenoic acid or trans-oleic acid (C18:1n-9), and cis-13 & 11-eicosenoic acid (C20:1n-7 & -9). Palmitoleic acid was highest in the antibiotic group and lowest in the 3 g RNL/kg diet group; the reverse was true for trans-oleic acid. C20:1n-7 and -9 and ƩMUFAs were highest in the antibiotic group and lowest in the control group, whereas the RNL-treated groups were intermediate. However, the levels of other individual MUFAs in the breast muscle were similar (p > 0.05) in the treatment groups.
The effect of the treatments on the PUFAs composition of the breast meat is shown in Table . The content of ALA, HPA, and Σn-3PUFAs was highest in the 5 g RNL and antibiotic groups and lowest in the control group. DHA content was highest in the group with 1 g RNL/kg diet compared to the other groups. The content of linoleic acid (LA) was the highest in the basal diet without RNL addition, lowest in the antibiotic group, and intermediate in the RNL-treated groups. The contents of eicosadienoic acid, arachidonic acid, adrenic acid, and osbond acid were highest in 5 g RNL compared with the other treatments. ΣUFAs were highest in the control group and lowest in the antibiotic group, while the RNL-treated groups were intermediate. However, no significant differences were found between treatment groups for other individual ΣUFAs levels in breast muscle. ΣSFAs: 31.82 − 35.07% and ΣUFAs: 64.93–68.19) were influenced by dietary RNL (p˂0.05).
Lipid quality, nutritional, and metabolic indices of fatty acid
The various quality, nutritional, and metabolic indices calculated based on FAs composition are shown in Table .
Table 5. Qualitative, nutritional, and metabolic indexing of fatty acids present in pectoral meat of broilers at d 34 fed experimental diet.
Qualitative indices
As for the index of ΣPUFAs/ΣSFAs, it is evident that the treatments did not show significant differences (p > 0.05), indicating that the unsaturation of meat was similar in all groups. For the n-6PUFAs/n-3PUFAs ratio, it was found that the five experimental groups had more significant differences when the specificity of the index was increased (between 3.98 and 7.58 for 5 g RNL/kg diet and control birds, respectively; p < 0.001). Therefore, birds fed 5 g RNL/kg diet had the lowest ratio (the best value) and the control group had the highest ratio (the worst value) and vice versa for Σn-3PUFAs/Σn-6PUFAs. Thus, meat with a lower ratio of n-6PUFAs/n-3PUFAs is more desirable to reduce the risk of various chronic diseases. This index shows that the meat of the group with 5 g RNL/kg has a balanced proportion of PUFAs, while the meat of the control group has a much higher ratio. The meat of the group with antibiotic and 5 g RNL diet is an even more fascinating and effective experimental diet with a high LA/ALA index. The ΣEPA + DHA% also provides higher values for the meat groups richer in PUFAs at 1 and 5 g RNL, as also shown by the index UI.
Nutritional indices
All treatments had similar nutritional indices (p > 0.05) except for the FLQ index, which was present only in birds fed 1 and 5 g RNL/kg diet. The pectoral meat of the 1 g RNL group had a 1.7-fold higher FLQ index (EPA + DHA, relative to total FAs) than the breast meat of the 5 g RNL group. Although the 5 g RNL group had a higher content of Σn-3PUFAs than the 1 g RNL group, the 1 g RNL/kg diet had a higher FLQ and the most desirable nutritional value than the 5 g RNL group. Although the control group had a high content of PUFAs, the FLQ index was zero because EPA and DHA were not present in this group.
Metabolic indices
Regarding metabolic indices, a significantly higher elongase index was observed in the in breast muscle tissue of the birds receiving 1 g RNL and a higher thioesterase index in the birds receiving 3 g RNL compared with the other treatments, while the birds receiving antibiotic were the lowest elongase and thioesterase. The Δ9-desaturase activity was higher in the 5 g RNL group than in the other groups. The Δ5-desaturase + Δ6-desaturase were significantly similar between groups (p > 0.05). The birds receiving the 5 g RNL group tended to be magnified by the 5 g RNL diet, with higher kinetic activity (higher locomotor activity or higher energy expenditure) and their muscles had higher oxidative than glycolytic status compared with the other treatments.
Sensory attributes
Sensory evaluation of broiler chickens receiving different RNL doses is shown in Table . The treatment groups had a significant (p ˂ 0.05) effect on the juiciness, tenderness, and flavour of breast meat. However, the overall acceptability of breast meat was comparable between treatments (p > 0.05). Juiciness and tenderness were highest and lowest in the antibiotic group and control group (p < 0.05), respectively, and were intermediate between the 1 and 3 g RNL groups (p < 0.05). The Flavour was better in the antibiotic-treated group and the RNL-treated groups than in the 0 g RNL.
Table 6. Sensory quality score assessment of breast meat of broilers at d 34 fed experimental diet.
Serum biochemistry of liver and renal profile
Table shows the effects of the feed treatments on safety enzymes and renal function of broilers on day 34. On day 34, RNL had no effect (p > 0.05) on safety enzymes or creatinine value. However, the ALT value was significantly (p < 0.05) decreased.
Table 7. Serum profile of kidney and renal functions at d 34 of broiler chickens.
Performance index and feed efficiency
Data on performance index and feed efficiency of broilers are summarised in Figures and . Treatments had no effect on performance index and feed efficiency throughout the growth period (d 1–34). Therefore, numerically (p > 0.05), the group treated with 1 g RNL had the highest performance index and feed efficiency throughout the period compared to the other groups. All broilers in all groups were healthy and showed no mortality.
Discussion
Some antibiotics can potentially alter fat metabolism in broilers, leading to changes in the fatty acid profile (Cimrin et al. Citation2020). These changes are not always desirable, as they may affect the nutritional quality of the meat. In this study, the antibiotic-treated group had lower values of fatty acids nutritionally (NVI and HPI) and metabolically (elongase, thioesterase, and activity indices) compared to the control group, which was due to a higher proportion of ƩSFAs, especially myristic acid (C14:0) and palmitic acid (C16:0), and a lower proportion of trans-oleic acid (C18:1n-9: 9). A study by Cimrin et al. (Citation2020) confirmed this viewpoint for C14:0 and C16:0 but not for total SFAs in the antibiotic-treated group. The antibiotic-treated group had a lower value of UI, indicating a lower value of healthy fat in their meat. When compared to the control, the antibiotic-treated group raised creatinine by 35.29%. Increased creatinine levels indicated compromised kidney function, whereas low creatinine levels may indicate renal protective activities (Ahmed et al. Citation2023; Haque et al. Citation2017).
Despite these undesirable effects, antibiotic as growth promoter promote growth performance index by 1.86% compared to control. In addition, antibiotic-treated group boosted qualitative fatty acids indexes such as reducing Σn-6PUFAs/Σn-3PUFA ratio and Linoleic acid/α-Linolenic acid ratio as well as reducing Σn-6PUFAs. Furthermore, antibiotic-treated group had increased Δ9-desaturase activity, which resulted in higher conversion of palmitic acid (C16:0) to palmitoleic acid (C16:1) and higher Cis-11-eicosenoic acid (C20:1n-9) levels, resulting in larger ƩMUFAs. Previous research by Cimrin et al. (Citation2020) supports this viewpoint, as palmitoleic monounsaturated fatty acid in yolk was shown to be higher in the antibiotic-treated group as compared to the control. All this qualitative improvement can be seen as a positive effect from a food safety perspective, especially a lower value of UI indicating a lower probability of autoxidation of FAs, and resulting in a higher sensory quality score and the desired organoleptic qualities. The biomarker ALT level rise indicated poor liver functioning (Ahmed et al. Citation2023). In comparison to the control group, the antibiotic-treated group lowered ALT biomarker by −38.25%, indicated good liver functioning.
Research investigated how the polyphenol-rich natural product RNL affects FAs profile and sensory evaluation. To our knowledge, there is no published evidence on the effects of dietary supplementation with RNL or its secondary metabolites in broilers on the selected parameters. For this reason, the findings of this investigation are compared with those of other natural phytogenic products.
Here, 5 g RNL treated group reduced ƩSFAs by 2.39% and increased ΣUFAs by 1.17%, especially increased Σn-3PUFAs by 33.48%, especially, increased C18:3n − 3 by 74.40% and C22:5n-3 by 1.20% compared with treatment with 0 g RNL. In addition, 5 g RNL treatment reduced Σn-6PUFAs/Σn-3PUFAs ratio by 49.56%, especially LA/ALA ratio by 74.93%, intermediate EPA + DHA%, increased UI by 8.85, and Δ9-desaturase by 7.02%, tending to the highest activity index by 4.87% compared with the control group. ΣSFAs were highest and lowest in the 3 and 5 g RNL groups, respectively and vice versa for ΣUFA. The ΣUFAs and ΣSFAs determined in this study are in agreement with those determined by Alagbe (Citation2021) and Qaid et al. (Citation2021). According to Alagbe (Citation2021), meat from birds is poor in lipids but rich in PUFAs. In poultry, the MUFAs are related to either internal anabolism or intestinal absorption from the diet, and the highest contents were represented mainly by palmitoleic acid and C20:1n-9, in the antibiotic group and mainly by oleic acid the 3 g RNL group. The low individual or total MUFA content observed mainly in the control group and lower level of RNL groups may be due to low intestinal absorption from the diet or different intramuscular fat content of the birds (Sirri et al. Citation2011). The meat of the control group was characterised by the highest percentage of PUFAs in adverse manner (the lowest n-3 and the highest n-6). Therefore, the meat of the 5 g RNL group is characterised by a higher proportion of PUFAs in the positive manner (the highest n-3 and the intermediate n-6).
The ratio of PUFAs to SFAs is a universally used indicator for analysing the effects of a given diet on cardiovascular health, assuming that all PUFAs lower low-density lipoprotein and total cholesterol, but all SFAs may increase serum cholesterol. Consequently, this is a direct indicator: higher levels indicate a greater benefit (or the opposite effect) of eating a particular meat or meat product (Simopoulos Citation2008). In this case, the groups with 0 and 5 g RNL had a higher numerical PUFAs/SFAs ratio (positive effect), so, the atherogenic index was lower and the HH ratio higher in these groups than in the other groups. A lower ratio of n-6PUFAs/n-3PUFAs is desirable to reduce the risks of chronic diseases that are prevalent in developing countries and in Western societies (Timmis et al. Citation2022). Thus, on the aforementioned index, both the 0 and 5 g RNL groups performed better than the other treatments, whereas on the basis of the n-6PUFAs/n-3PUFAs ratio, the 5 g RNL group and the other groups were more desirable than the 0 g RNL group.
Since these two FAs cannot be synthesised in the human body and must be ingested through the diet, the linoleic acid/linolenic acid ratio was used as an evaluation method for infant feeding. They also compete for the same elongase and desaturase enzymes that enable PUFA synthesis. Because of the low conversion rate ALA, a decrease in the ratio LA/ALA moderately improves the values of certain n-3PUFAs such as EPA, DHA and DPA (Das Citation2006); consequently, the index LA/ALA could be considered as a first step to estimate PUFAs. The ratio of LA/ALA in typical Western diets stated to be ∼ 10:1 or higher (Blasbalg et al. Citation2011; Simopoulos Citation2016), that is higher than the ideal ratio of ∼1–2:1 based on the amounts required for top conversion of ALA to its longer chain end product (Draycott et al. Citation2019). Supplementation of 5 g RNL or the antibiotic increased the ALA content of the meat and decreased the LA/ALA ratio. This is consistent with Ryman et al. (Citation2017), who found that supplementation of ALA increased ALA content and decreased the ratio of LA/ALA compared with linoleic acid supplementation. In contrast, lower levels of ALA in meat could be explained by the higher conversion of ALA to its longer chain derivatives (Dal Bosco et al. Citation2016).
EPA and DHA are n-3PUFAs that benefit human health by preventing cardiovascular disease, inflammation, and reproductive health (Ochi and Tsuchiya Citation2018). Here, both EPA and DHA were present only in birds fed 1 and 3 RNL/kg diet (1.61 and 0.88%, respectively). UI contains information on the degree of unsaturation of each FAs and is computed as ƩUFAs (%) multiplied by the number of double bonds, yielding different levels of the different unsaturated types. This index was similar for the omega-3 and omega-6 series; thus, it is not very specific for dietary features, although it plays a role in determining oxidative feed stability in humans and animals and in establishing certain oxidative protective measures (Crupi and Cuzzocrea Citation2022; Untea et al. Citation2023). Here, meat from broilers treated with an antibiotic and 3 g RNL/kg feed had a lower value of UI than meat from broilers treated with 5 g RNL/kg feed, indicating a lower probability of autoxidation of FAs but a lower value of healthy fat from their meat.
Although the NVI was similar for all treatments, it was numerically higher (1.93) in the group treated with1 g RNL. Because of their greater amounts, the NVI only takes into account the major FAs in animal-derived meals, namely palmitic acid, oleic acid, and stearic acid (Chen et al. Citation2016). An atherogenic index is a more specific index for determining the atherogenicity of foods than PUFAs/SFAs (Dal Bosco et al. Citation2016). With the exception of stearic acid, which is not considered proatherogenic due to the ability of humans to desaturate stearic acid to oleic acid, the atherogenic index specifically shows the link between SFAs. On the other hand, lauric, myristic, and palmitic acids promote lipocyte adherence to circulatory and immune systems cells, and the aggregation of atherogenic plaques, while decreasing esterified FAs and phospholipids content (Omri et al. Citation2019). In contrast to the PUFAs/SFAs index, a lower value indicates that the meal has better nutritional properties. In agreement with Chen et al. (Citation2004), they suggested that the HPI directly indicates that this index is just the opposite of the atherogenic index. Thus, HPI showed better numerical nutritional value of fat (2.30 and 2.22, respectively) and lower atherogenic index (0.44 and 0.45, respectively) at both 0 and 5 g RNL compared with the other treatments. Thus, the HPI is used to evaluate the nutritional value of fat and the effects of FAs on cardiovascular disease. The thrombogenicity index is used with the atherogenicity index to further describe the thrombogenic ability of FAs. In this context, they are divided into pro-thrombogenic (lauric acid ‘C12:0’, myristic acid ‘C14:0’, and ‘palmitic acid’ C16:0) and anti-thrombogenic FAs based on the effect of some derivatives (eicosanoids), e.g. MUFAs, n-3PUFAs and n-6PUFAs, although Calder (Citation2006) revealed the adverse effect of n-6PUFAs on thrombogenesis. Thus, supplementation of natural products or feeds with lower thrombogenicity is valuable for public health (5 g RNL was the group with the lowest thrombogenicity index (0.675), which indirectly means that 5 g RNL has lower thrombogenicity). The HH ratio focuses on the relationships between FAs in the diet and low-density lipoproteins in the blood terms of the hypocholesterolemic FAs (Oleic acid and PUFAs) and hypercholesterolemic FAs (lauric acid, myristic acid, palmitic acid) (Chen et al. Citation2016). In this study, both groups receiving 0 and 5 g RNL were able to increase hypocholesterolemic FAs (such as PUFAs) and decrease hypercholesterolemic FAs, resulting in an increase in HH ratio (2.46 and 2.19, respectively).
The much greater elongation and higher elongation index (C18:0:C16:0 ratio) of breast muscle tissue in the 1 g RNL group observed in this study is most likely due to the greater elongation of long C18 acyl chains compared with C16 acyl chains. In addition, the significantly higher thioesterase index (C16:0:C14:0 ratio) of breast muscle tissue in the 3 g RNL-treated group may have been associated with the lower cleavage of C14-acyl carrier proteins (ACPs) and the higher cleavage of C16-ACPs. On the other hand, the Δ9-desaturase converting stearic acid to oleic acid and palmitic acid to palmitoleic acid was lower in the 3 g RNL-treated group, indicating that the lower amounts of palmitoleic acid in the 3 g RNL-treated group may be due to lower Δ9-desaturase activity. While the higher conversion of C16:0 in the 5 g RNL-treated group and the antibiotic-treated group may be due to higher Δ9-desaturase activity. The rate of omega-3 β-oxidation in the myocyte (e.g. n-3PUFAs/ALA) or mobilisation of n-3PUFAs for energy synthesis (i.e. during exercise or locomotor activity) results in an oxidative state. The ratios of n-3PUFAs and ALA are indicative of energy consumption (β-oxidation cycle). The two carboxy-terminal carbon atoms of fatty acyl-coenzyme A are released as acetyl-coenzyme A units each time until a cycle of β-oxidation is completed in mitochondria (Houten and Wanders Citation2010). In our case, the birds receiving the 5 g RNL group tended to have higher kinetic activity (higher locomotor activity) and their muscles were higher in oxidative status than in glycolytic status compared with the other treatments. This is in agreement with other authors (Dal Bosco et al. Citation2012; Failla et al. Citation2021). They observed that chickens with higher kinetic activity had more oxidative than glycolytic muscles. Thus, the activity index is calculate the energy consumption resulting from locomotor activity throughout the life of the chicken, with all the nutritional and qualitative effects on its meat.
This study shows that the cooked breast meat has significant differences in juiciness, tenderness, flavour and overall acceptability. In agreement with those of Alagbe (Citation2023), who found that the addition of Prosopis africana essential oil to broiler feed positively influenced the sensory evaluation of broiler meat. In addition, Giannenas et al. (Citation2013) showed that feeding 200 mg/kg lemongrass and clove oil to broilers altered meat flavour perception. Krishan and Narang (Citation2014) found that chicken meat samples fed 300 mg/kg thyme oil were tastier and softer. The breast meat of broilers given RNL was juicier, more tender, tastier, and generally more acceptable than that of the control group, suggesting that the addition of RNL may improve the flavour of broiler meat. In contrast, Reda et al. (Citation2020) reported that dietary supplementation with 50 mg/kg garlic oil did not alter the sensory attributes of breast meat. The differences in these outcomes may be due to the different composition of bioactive composites in the essential oils. The meat of the 1 g RNL group was more flavourful than the other RNL doses and the control group, which might be related to the fact that 1 g RNL had the highest EPA%+DHA% content, resulting in the highest meat lipid quality. The higher phenolic contents prevented the oxidation and denaturation of myoglobin (Nuerjiang et al. Citation2023). These phenols can delay the oxidative pathway of lipids in meat, preventing spoilage and undesirable taste and odour (Al-Garadi et al. Citation2022). Phytogenic additives can accumulate healthy PUFAs and reduce lipid oxidation, which prolongs the life of meat (Gholami‐Ahangaran et al. Citation2022). This improvement in sensory quality of meat may be related to the presence of natural antioxidants, e.g. RNL, which is rich in phenols such as gallic acid (Qasem et al. Citation2020; Gemechu et al. Citation2021).
Differences in body fat deposition of broilers fed different feed additives may be ascribed to changes in lipid synthesis or lipid oxidation rates (Crespo and Esteve-Garcia Citation2001). According to Ciftci et al. (Citation2010), the antioxidant properties of RNL and antibiotics prevented lipid peroxidation of tissue lipids, especially MUFAs compared with control. As a result, the MUFA content of breast meat was significantly higher in this study. In contrast, SFAs increased in the antibiotic and RNL groups, whereas PUFA and n-6 fatty acids decreased (Ciftci et al. Citation2010). The results suggest that 1 g/kg RNL intake can be used as an alternative to antibiotics in broiler diets. Toghyani et al. (Citation2011) found that sensory evaluation of thigh meat did not reveal any abnormal odour or flavour of the meat induced by the antibiotic group receiving 3 mg/kg flavophospholipol and by the phytogenic groups receiving 2 and 4 g/kg cinnamon and 2 and 4 g/kg garlic powder as feed additives. In agreement with Toghyani et al. (Citation2011), the inclusion of antibiotics in broiler diets improved the sensory evaluation of the meat.
Oxidising chemicals have an undesirable effect on the sensory evaluation of meat from both sensory and health perspectives. The use of natural or synthetic antioxidants could successfully minimise oxidative deterioration. Antibiotics in broiler diets improved sensory evaluation of meat according to the results. Nevertheless, the use of these drugs is limited due to residues or consumer resistance to antibiotic therapy. Growing consumer awareness of the potential health consequences of using antibiotics as growth promoters has led consumer demands to establish requirements for their food to be high quality, nutritious, and free of chemical antioxidants or preservatives due to safety concerns and health risks. This has led the meat industry to use plant-based additives such as RNL instead of synthetic additives. As a result, RNL at 1 g/kg improve the sensory quality of meat and have health benefits, as they are considered an important source of bioactive compounds with antioxidant, antibacterial and other bioactive effects. Moreover, since they are responsible for the odour of aromatic herbs, they can be used to produce flavour enhancers for food (Gowe and Management 2015). In this regard, RNL, especially at 1 g/kg feed, could be used as natural additives in broiler diets or the production of meat products, not only to improve shelf life by retarding lipid oxidation and microbial growth, but also to produce highly nutritious products with good organoleptic properties
Overall, RNL is an effective product that positively affects FAs indices and organoleptic parameters of broilers. These effects, which led to these modifications in FAs indices and organoleptic quality, can be attributed to the fact that phenolic compounds are the main product of plant secondary metabolism (Song et al. Citation2020). Here, the ethanolic RNL extract had a higher total phenolic content (171.2 mg GAE/g for RNL). In agreement with Qasem et al. (Citation2020), gallic acid and other active natural phenolic compounds with different biological properties are present in RNL. In this case, 13 major constituents were identified in RNL in GC-MS analysis, and HPLC analysis revealed that gallic acid is the most abundant constituent in RNL extract. The results of the proximate analysis of RNL indicate the suitability of RNL as a dietary supplement. Thus, RNL contains lipid sources that include desirable and undesirable as well as essential and nonessential FAs in varying degrees.
In terms of safety, broilers treated with RNL showed no changes at day 34 on AST, indicating no muscle or liver damage. Safety enzymes are considered safety signs and are abnormally increase in the bloodstream when the muscles or liver are damaged (Song et al. Citation2021). Despite substantial differences in serum levels of ALT enzymes between groups, they were detected at low levels in the bloodstream. However, these results indicate that no adverse effects of RNL at the doses used on the biological safety of the tested herbs in the blood, as evidenced by no significant changes in renal and liver functions. This indicates that up to 5 g of RNL per kg of feed can be added to the broiler feed without adverse effects.
The performance index ranged from 37 to 40, which is higher than the performance index of 28 to 37 of determined by Jahan et al. (Citation2006) for the basal diet. It was found that dietary natural herbs improved the performance index and feed efficiency, especially at a level of 1 g RNL. Gallic acid, secondary water-soluble phenolic metabolites, are key components and small basic building blocks of hydrolysable gallotannins and ellagitannins, which are degraded by acid or enzymes. Gallic acid is an important basic building block of hydrolysable tannins and contributes to their antioxidant and other biological activities (Brus et al. Citation2021). In addition, it has an useful proliferative effect on nontransformed epithelial cell lines that restore, maintain, and develop the absorptive surface of the small intestine. In agreement with Samuel et al. (Citation2017), who found that 75 to 100 mg/kg of gallic acid is suitable for improved performance and health benefits in a broiler diet. In contrast, Butler (Citation1992) claims that hydrolysable tannins often have antinutritional effects that result in decreased weight gain and nutrient utilisation efficiency by systemically suppressing the metabolism of digested and absorbed nutrients, particularly proteins. Under the experimental condition, no adverse nutritional effects or side effects on growth and feed efficiency were observed compared with the antibiotic and control groups, so the safety enzyme did not cause mortality in the normal situation. Furthermore, no evidence of a negative effect of the same RNL doses on carcass parameters or even breast weight was observed in our recently published study (Qaid et al. Citation2023). In addition, the present GC-MS results of the meat samples did not show carcass residues such as gallic acid, indicating that safe meat without carcass residues was present.
Conclusion
In conclusion, the 1 g RNL treatment had the highest elongation index, the highest EPA% + DHA%, and the highest meat lipid quality, and then tended to improve the performance index, feed efficiency and nutritional index. On the other hand, the treatment with 5 g RNL showed the best lipid quality, nutritional, health and metabolic indices in terms of fatty acid composition of breast muscle. Despite the positive effects of 5 g RNL on various fatty acid profile indices, it is possible to use RNL in broiler diets at 1 g to obtain positive multifunctional benefits. The addition of RNL to broiler feed does not result in abnormal odour or flavour in the meat, but 1 g RNL increases sensory characteristics to levels comparable to antibiotics and outperforms the 0 g RNL group. RNL was found to contain a number of phytoconstituents that may improve the fatty acid content and oxidative stability of meat; however, further studies are needed to investigate these indicators from a molecular perspective.
Supplemental Material
Download MS Word (19.1 KB)Acknowledgments
The authors extend their appreciation to the Deputyship for Research and Innovation, ‘Ministry of Education’ in Saudi Arabia for funding this research (IFKSUOR3-449-1).
Disclosure statement
No potential conflict of interest was reported by the author(s).
Data availability statement
The data presented in this study are available on request from the corresponding author upon reasonable request.
Additional information
Funding
References
- Aati H, El-Gamal A, Shaheen H, Kayser O. 2019. Traditional use of ethnomedicinal native plants in the Kingdom of Saudi Arabia. J Ethnobiol Ethnomed. 15(1):2. doi: 10.1186/s13002-018-0263-2.
- Agnew MP, Craigie CR, Weralupitiya G, Reis MM, Johnson PL, Reis MG. 2019. Comprehensive evaluation of parameters affecting one-step method for quantitative analysis of fatty acids in meat. Metabolites. 9(9):189. doi: 10.3390/metabo9090189.
- Ahmed EGM, Twafik w, Basher AW, Hashim MA, Seddek A-LS, Morad SA, Abdelmageed N. 2023. The evaluation of the safety and toxicological characteristics of Acacia nilotica in broiler. SVU Int J Vet Sci. 6(2):124–135. doi: 10.21608/svu.2023.205976.1268.
- Al-Garadi MA, Qaid MM, Alqhtani AH, Pokoo-Aikins A, Al-Mufarrej SI. 2022. In vitro phytochemical analysis and antibacterial and antifungal efficacy assessment of ethanolic and aqueous extracts of Rumex nervosus leaves against selected bacteria and fungi. Vet World. 15(11):2725–2737. doi: 10.14202/vetworld.2022.2725-2737.
- Alagbe J. 2021. Daniellia oliveri leaf extracts as an alternative to antibiotic feed additives in broiler chicken diets: meat quality and fatty acid composition. IJIAS. 1(3):177–186. doi: 10.47540/ijias.v1i3.174.
- Alagbe OJ. 2023. Sensory evaluation and fatty acid composition of broiler chickens fed diets containing with Prosopis africana essential oil. JHBS. 1(2):35–45. doi: 10.31098/jhbs.v1i2.1721.
- Al-Khalaifa H, Al-Nasser A, Al-Surayee T, Al-Kandari S, Al-Enzi N, Al-Sharrah T, Ragheb G, Al-Qalaf S, Mohammed A. 2019. Effect of dietary probiotics and prebiotics on the performance of broiler chickens. Poultry science. 98(10): 4465–4479.
- Asante-Addo C. 2020. Analysis of consumer attitudes, preferences, and demand for poultry meat in Ghana [dissertation]. Göttingen: Georg-August Universität.
- Attia Y. 2003. Nutritional values of soaked linseed cake and its iinclusion in finishing diets for male broiler chicks a source of protein and n-3 fatty acids. Egyptian Poul Sci J. 23:739–759.
- Attia YA, Al-Harthi MA, Al-Sagan AA, Hussein EO, Alhotan RA, Suliman GM, Abdulsalam NM, Olal MJ. 2022. Responses of egg quality sustainability, sensory attributes and lipid profile of eggs and blood to different dietary oil supplementations and storage conditions. Ital J Anim Sci. 21(1):1160–1169. doi: 10.1080/1828051X.2022.2101390.
- Attia YA, Al-Harthi MA, Korish MA, Shiboob MM. 2017. Fatty acid and cholesterol profiles, hypocholesterolemic, atherogenic, and thrombogenic indices of broiler meat in the retail market. Lipids Health Dis. 16(1):40. doi: 10.1186/s12944-017-0423-8.
- Blasbalg TL, Hibbeln JR, Ramsden CE, Majchrzak SF, Rawlings RR. 2011. Changes in consumption of omega-3 and omega-6 fatty acids in the United States during the 20th century. Am J Clin Nutr. 93(5):950–962. doi: 10.3945/ajcn.110.006643.
- Brus M, Frangež R, Gorenjak M, Kotnik P, Knez Ž, Škorjanc D. 2021. Effect of hydrolyzable tannins on glucose-transporter expression and their bioavailability in pig small-intestinal 3D cell model. Molecules. 26(2):345. doi: 10.3390/molecules26020345.
- Butler LG. 1992. Basic Life Sciences book series (BLSC, volume 59). Plant polyphenols: synthesis, properties, significance. In: Richard WH, Peter EL, editors. Antinutritional effects of condensed and hydrolyzable tannins. SpringerLink..
- Calder PC. 2006. Polyunsaturated fatty acids and inflammation. Prostaglandins Leukot Essent Fatty Acids. 75(3):197–202. doi: 10.1016/j.plefa.2006.05.012.
- Cartoni Mancinelli A, Mattioli S, Twining C, Dal Bosco A, Donoghue AM, Arsi K, Angelucci E, Chiattelli D, Castellini C. 2022. Poultry meat and eggs as an alternative source of n-3 long-chain polyunsaturated fatty acids for human nutrition. Nutrients. 14(9):1969. doi: 10.3390/nu14091969.
- Chen S, Bobe G, Zimmerman S, Hammond EG, Luhman CM, Boylston TD, Freeman AE, Beitz DC. 2004. Physical and sensory properties of dairy products from cows with various milk fatty acid compositions. J Agric Food Chem. 52(11):3422–3428. doi: 10.1021/jf035193z.
- Chen Y, Qiao Y, Xiao Y, Chen H, Zhao L, Huang M, Zhou G. 2016. Differences in physicochemical and nutritional properties of breast and thigh meat from crossbred chickens, commercial broilers, and spent hens. Asian-Australas J Anim Sci. 29(6):855–864. doi: 10.5713/ajas.15.0840.
- Ciftci M, Simsek UG, Yuce A, Yilmaz O, Dalkilic B. 2010. Effects of dietary antibiotic and cinnamon oil supplementation on antioxidant enzyme activities, cholesterol levels and fatty acid compositions of serum and meat in broiler chickens. Acta Vet Brno. 79(1):33–40. doi: 10.2754/avb201079010033.
- Cimrin T, Tunca RI, Avsaroglu MD, Ayasan T, Küçükersan S. 2020. Effects of an antibiotic and two phytogenic substances (cinnamaldehyde and 1, 8-cineole) on yolk fatty acid profile and storage period-associated egg lipid peroxidation level. R Bras Zootec. 49:1–10.
- Crespo N, Esteve-Garcia E. 2001. Dietary fatty acid profile modifies abdominal fat deposition in broiler chickens. Poult Sci. 80(1):71–78. doi: 10.1093/ps/80.1.71.
- Crupi R, Cuzzocrea S. 2022. Role of EPA in inflammation: mechanisms, effects, and clinical relevance. Biomolecules. 12(2):242. doi: 10.3390/biom12020242.
- Dal Bosco A, Cartoni Mancinelli A, Vaudo G, Cavallo M, Castellini C, Mattioli S. 2022. Indexing of fatty acids in poultry meat for its characterization in healthy human nutrition: a comprehensive application of the scientific literature and new proposals. Nutrients. 14(15):3110. doi: 10.3390/nu14153110.
- Dal Bosco A, Mugnai C, Mattioli S, Rosati A, Ruggeri S, Ranucci D, Castellini C. 2016. Transfer of bioactive compounds from pasture to meat in organic free-range chickens. Poult Sci. 95(10):2464–2471. doi: 10.3382/ps/pev383.
- Dal Bosco A, Mugnai C, Ruggeri S, Mattioli S, Castellini C. 2012. Fatty acid composition of meat and estimated indices of lipid metabolism in different poultry genotypes reared under organic system. Poult Sci. 91(8):2039–2045. doi: 10.3382/ps.2012-02228.
- Das UN. 2006. Essential fatty acids: biochemistry, physiology and pathology. Biotechnol J. 1(4):420–439. doi: 10.1002/biot.200600012.
- Draycott SA, Liu G, Daniel ZC, Elmes MJ, Muhlhausler BS, Langley-Evans SC. 2019. Maternal dietary ratio of linoleic acid to alpha-linolenic acid during pregnancy has sex-specific effects on placental and fetal weights in the rat. Nutr Metab. 16(1):1–12. doi: 10.1186/s12986-018-0330-7.
- Elbadawy M, Aboubakr M. 2017. Efficacy of colimoxŪ (a new combination of amoxicillin and colistin) in the control of experimentally induced necrotic enteritis in broiler chickens. IJPT. 5(1):51–56. doi: 10.14419/ijpt.v5i1.7193.
- Failla S, Buttazzoni L, Zilio DM, Contò M, Renzi G, Castellini C, Amato MG. 2021. An index to measure the activity attitude of broilers in extensive system. Poult Sci. 100(8):101279. doi: 10.1016/j.psj.2021.101279.
- Gemechu W, Woldekidan S, Teka F, Mohammed J, Ashebir R, Sisay B, Abebe A, Meresa A. 2021. Ethnomedicinal uses, phytochemisty and pharmacological activities of Rumex nervosus. JAPLR. 10(2):65–69. doi: 10.15406/japlr.2021.10.00367.
- Gholami‐Ahangaran M, Ahmadi‐Dastgerdi A, Azizi S, Basiratpour A, Zokaei M, Derakhshan M. 2022. Thymol and carvacrol supplementation in poultry health and performance. Vet Med Sci. 8(1):267–288. doi: 10.1002/vms3.663.
- Giannenas I, Bonos E, Christaki E, Florou-Paneri P. 2013. Essential oils and their applications in animal nutrition. Med Aromat Plants. 2(140):2167–0412.
- Gowe C. 2015. Review on potential use of fruit and vegetables by-products as a valuable source of natural food additives. Food Sci Qual Manag. 45(5):47–61.
- Haque MI, Ahmad N, Miah, MA. 2017. Comparative analysis of body weight and serum biochemistry in broilers supplemented with some selected probiotics and antibiotic growth promoters. J Adv Vet Anim Res. 4(3):288–294. doi: 10.5455/javar.2017.d226.
- Houten SM, Wanders RJ. 2010. A general introduction to the biochemistry of mitochondrial fatty acid β-oxidation. J Inherit Metab Dis. 33(5):469–477. doi: 10.1007/s10545-010-9061-2.
- Islam MA, Nishibori M. 2023. Use of cinnamon and Bacillus subtilis probiotics in the diet of broiler chickens. Canadian J Anim Sci. 1–23.
- Jachimowicz K, Winiarska-Mieczan A, Tomaszewska E. 2022. The impact of herbal additives for poultry feed on the fatty acid profile of meat. Animals. 12(9):1054. doi: 10.3390/ani12091054.
- Jahan M, Asaduzzaman M, Sarkar A. 2006. Performance of broiler fed on mash, pellet and crumble. Int J Poult Sci. 5(3):265–270.
- Krishan G, Narang A. 2014. Use of essential oils in poultry nutrition: a new approach. J Adv Vet Anim Res. 1(4):156–162. doi: 10.5455/javar.2014.a36.
- Kwiecień M, Winiarska-Mieczan A, Danek-Majewska A, Kwiatkowska K, Krusiński R. 2021. Effects of dietary alfalfa protein concentrate on lipid metabolism and antioxidative status of serum and composition and fatty acid profile and antioxidative status and dietetic value of muscles in broilers. Poultr Sci. 100(4):100974. doi: 10.1016/j.psj.2020.12.071.
- Li Y, Mei H, Liu Y, Li Z, Qamar H, Yu M, Ma X. 2023. Dietary supplementation with Rutin alters meat quality, fatty acid profile, antioxidant capacity, and expression levels of genes associated with lipid metabolism in breast muscle of Qingyuan partridge chickens. Foods. 12(12):2302. doi: 10.3390/foods12122302.
- Mogotlane PM, Ng’ambi JW, Nyazema NZ, Chitura T. 2023. Effect of feed supplementation with probiotics and antimicrobial agents on meat quality of broiler chickens. Agri Sci Digest. doi: 10.18805/ag.DF-492:1-6.
- Nuerjiang M, Li Y, Yue X, Kong B, Liu H, Wu K, Xia X. 2023. Analysis of inhibition of guava (Psidium guajava l.) leaf polyphenol on the protein oxidative aggregation of frozen chicken meatballs based on structural changes. Food Res Int. 164:112433. doi: 10.1016/j.foodres.2022.112433.
- Ochi E, Tsuchiya Y. 2018. Eicosapentaenoic acid (EPA) and docosahexaenoic acid (DHA) in muscle damage and function. Nutrients. 10(5):552. doi: 10.3390/nu10050552.
- Omri B, Chalghoumi R, Izzo L, Ritieni A, Lucarini M, Durazzo A, Abdouli H, Santini A. 2019. Effect of dietary incorporation of linseed alone or together with tomato-red pepper mix on laying hens’ egg yolk fatty acids profile and health lipid indexes. Nutrients. 11(4):813. doi: 10.3390/nu11040813.
- Oppedisano F, Macrì R, Gliozzi M, Musolino V, Carresi C, Maiuolo J, Bosco F, Nucera S, Caterina Zito M, Guarnieri L, et al. 2020. The anti-inflammatory and antioxidant properties of n-3 PUFAs: their role in cardiovascular protection. Biomedicines. 8(9):306. doi: 10.3390/biomedicines8090306.
- Qaid MM, Al-Mufarrej SI, Al-Garadi MA, Al-Haidary AA. 2023. Effects of Rumex nervosus leaf powder supplementation on carcasses compositions, small intestine dimensions, breasts color quality, economic feasibility in broiler chickens. Poult Sci. 102(10):102943. doi: 10.1016/j.psj.2023.102943.
- Qaid MM, Al-Mufarrej SI, Azzam MM, Al-Garadi MA, Albaadani HH, Alhidary IA, Aljumaah RS. 2021. Growth performance, serum biochemical indices, duodenal histomorphology, and cecal microbiota of broiler chickens fed on diets supplemented with cinnamon bark powder at prestarter and starter phases. Animals. 11(1):94. doi: 10.3390/ani11010094.
- Qasem MA, Dkhil MA, Al-Shaebi EM, Murshed M, Mares M, Al-Quraishy S. 2020. Rumex nervosus leaf extracts enhance the regulation of goblet cells and the inflammatory response during infection of chickens with Eimeria tenella. J King Saud Univ Sci. 32(3):1818–1823. doi: 10.1016/j.jksus.2020.01.024.
- Rahman MRT, Fliss I, Biron EJA. 2022. Insights in the development and uses of alternatives to antibiotic growth promoters in poultry and swine production. Antibiotics. 11(6):766. doi: 10.3390/antibiotics11060766.
- Reda F, Alagawany M, Mahmoud H, Mahgoub S, Elnesr S. 2020. Use of red pepper oil in quail diets and its effect on performance, carcass measurements, intestinal microbiota, antioxidant indices, immunity and blood constituents. Animal. 14(5):1025–1033. doi: 10.1017/S1751731119002891.
- Ryman V, Packiriswamy N, Norby B, Schmidt S, Lock A, Sordillo L. 2017. Supplementation of linoleic acid (C18: 2n-6) or α-linolenic acid (C18: 3n-3) changes microbial agonist-induced oxylipid biosynthesis. J Dairy Sci. 100(3):1870–1887. doi: 10.3168/jds.2016-11599.
- Samuel K, Wang J, Yue H, Wu S, Zhang H, Duan Z, Qi G. 2017. Effects of dietary gallic acid supplementation on performance, antioxidant status, and jejunum intestinal morphology in broiler chicks. Poult Sci. 96(8):2768–2775. doi: 10.3382/ps/pex091.
- SAS. 2012. SAS Institute/OR 9.3 user’s guide: mathematical programming examples. Cary, NC: SAS Institute. Inc.
- Simopoulos AP. 2008. The importance of the omega-6/omega-3 fatty acid ratio in cardiovascular disease and other chronic diseases. Exp Biol Med. 233(6):674–688. doi: 10.3181/0711-MR-311.
- Simopoulos AP. 2016. An increase in the omega-6/omega-3 fatty acid ratio increases the risk for obesity. Nutrients. 8(3):128. doi: 10.3390/nu8030128.
- Sirri F, Castellini C, Bianchi M, Petracci M, Meluzzi A, Franchini A. 2011. Effect of fast-, medium-and slow-growing strains on meat quality of chickens reared under the organic farming method. Animal. 5(2):312–319. doi: 10.1017/S175173111000176X.
- Smith J. 2019. Broiler production without antibiotics: United States field perspectives. Technology. 250:93–98. doi: 10.1016/j.anifeedsci.2018.04.027.
- Song B, Fu M, He F, Zhao H, Wang Y, Nie Q, Wu B. 2021. Methionine deficiency affects liver and kidney health, oxidative stress, and ileum mucosal immunity in broilers. Front Vet Sci. 8:722567. doi: 10.3389/fvets.2021.722567.
- Song H, Zhang L, Wu L, Huang W, Wang M, Zhang L, Shao Y, Wang M, Zhang F, Zhao Z, et al. 2020. Phenolic acid profiles of common food and estimated natural intake with different structures and forms in five regions of China. Food Chem. 321:126675. doi: 10.1016/j.foodchem.2020.126675.
- Suliman GM, Alowaimer AN, Al-Mufarrej SI, Hussein EO, Fazea EH, Naiel MA, Alhotan RA, Swelum AA. 2021. The effects of clove seed (Syzygium aromaticum) dietary administration on carcass characteristics, meat quality, and sensory attributes of broiler chickens. Poult Sci. 100(3):100904. doi: 10.1016/j.psj.2020.12.009.
- Timmis A, Vardas P, Townsend N, Torbica A, Katus H, De Smedt D, Gale CP, Maggioni AP, Petersen SE, Huculeci R, Atlas Writing Group, European Society of Cardiology, et al. 2022. European Society of Cardiology: cardiovascular disease statistics 2021. Eur Heart J. 43(8):716–799. doi: 10.1093/eurheartj/ehab892.
- Toghyani M, Toghyani M, Gheisari A, Ghalamkari G, Eghbalsaied S. 2011. Evaluation of cinnamon and garlic as antibiotic growth promoter substitutions on performance, immune responses, serum biochemical and haematological parameters in broiler chicks. Livestock Science. 138(1–3):167–173. doi: 10.1016/j.livsci.2010.12.018.
- Untea AE, Varzaru I, Saracila M, Panaite TD, Oancea AG, Vlaicu PA, Grosu IA. 2023. Antioxidant properties of cranberry leaves and walnut meal and their effect on nutritional quality and oxidative stability of broiler breast meat. Antioxidants. 12(5):1084. doi: 10.3390/antiox12051084.
- Vessby B, Gustafsson IB, Tengblad S, Boberg M, Andersson A. 2002. Desaturation and elongation of fatty acids and insulin action. Ann N Y Acad Sci. 967(1):183–195. doi: 10.1111/j.1749-6632.2002.tb04275.x.
- Waheed S, Hasnain A, Ahmad A, Tarar O, Yaqeen Z, Ali T. 2018. Effect of botanical extracts on amino acid and fatty acid profile of broiler meat. Braz J Poult Sci. 20(3):507–516. doi: 10.1590/1806-9061-2017-0651.
- Wu Q, Zhou J. 2021. The application of polyphenols in food preservation. Adv Food Nutr Res. 98:35–99.
- Yang L, Liu G, Zhu X, Luo Y, Shang Y, Gu X-L. 2019. The anti-inflammatory and antioxidant effects of leonurine hydrochloride after lipopolysaccharide challenge in broiler chicks. Poult Sci. 98(4):1648–1657. doi: 10.3382/ps/pey532.
- Yu C, Zhang J, Li Q, Xiang X, Yang Z, Wang T. 2021. Effects of trans-anethole supplementation on serum lipid metabolism parameters, carcass characteristics, meat quality, fatty acid, and amino acid profiles of breast muscle in broiler chickens. Poult Sci. 100(12):101484. doi: 10.1016/j.psj.2021.101484.
- Zhang S, Knight TJ, Stalder KJ, Goodwin RN, Lonergan SM, Beitz D. 2007. Effects of breed, sex, and halothane genotype on fatty acid composition of pork longissimus muscle. J Anim Sci. 85(3):583–591. doi: 10.2527/jas.2006-239.