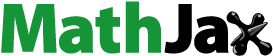
Abstract
In the current study, we tested the effects of dietary cowpea protein hydrolysate (CPH) on broilers’ growth, carcase traits, intestinal histomorphology, The fatty acid profile of breast muscle, blood biochemical parameters, and immune status. Three-day age male Ross-308 broilers (n = 300, average weight 86.72 g ± 0.20) were distributed into five groups and fed on five diets supplied with five levels of CPH at 0, 2, 4, 6, and 8 g kg−1 for 35 days. The results revealed that dietary CPH did not change (P < 0.05) the broilers’ growth performance or carcase traits, except for a quadratic decrease (P < 0.05) in FCR at concentrations 2 and 4 g kg−1 during the finisher period. The villus heights (VH) and goblet cell count (GCC) were increased (P < 0.05) in the duodenum and jejunum; however, the villus width decreased (P < 0.05) in the duodenum without showing changes (P < 0.05) in the jejunum and ileum. Dietary supplementation with 6 g CPH kg−1 increased (P < 0.05) the duodenal crypt depth (CD). Dietary supplementation with 8 g CPH kg −1 increased the VH: CD in the duodenum compared to the control; nevertheless, a quadratic (P < 0.05) change was reported in the jejunum. The VH: CD did not change (P < 0.05) in the ileum; however, dietary supplementation with 6 CPH kg−1 increased (P < 0.05) the ileal VH and GCC. The inclusion of CPH led to a linear (P < 0.05) increase in the total n-3 polyunsaturated fatty acids (PUFA), n-6 PUFA, and n-3/n-6 PUFA in broilers’ breast muscle. An increase in the assessed metabolic and immune status indices was reported, as evidenced by increases (P < 0.05) in thyroid hormones, growth hormone, total protein, albumin, globulin, lysozymes, interferon-gamma, interleukin10, and complement 3 serum levels. However, the serum glucose and leptin levels did not show significant (p > 0.05) changes in response to dietary CPH. Moreover, the dietary CPH levels 4–8 g kg−1 upregulated the immunostaining of IgG in the spleen of these groups. These findings suggested that including CPH in broiler diets could be an effective candidate for modulating the chickens’ metabolic and immune status; however, it had no increased effect on broiler growth.
HIGHLIGHTS
Cowpea protein hydrolysate (CPH) did not positively affect the broiler growth performance or carcase traits, except for a reduction in FCR during the finisher period at levels 2 and 4 g CPH kg−1 diet.
CPH improved the chickens’ metabolic and immune status in a concentration-dependent manner.
Dietary supplementation with CPH would be feasible in the production of broilers.
Introduction
In the past, whole seeds of legumes have been the primary form in which they were used, but in recent years, interest in using legumes in other forms, such as flour, concentrates, and isolate, has increased (Ohanenye et al. Citation2022, Sitohy and Osman Citation2010). The cowpea (Vigna unguiculata) is an essential heat and drought-tolerant grain legume in tropical and subtropical climates (Apata and Ologhobo Citation1997, Mekonnen et al. Citation2022). It is a rich source of many other health-promoting elements, including phenolic compounds, soluble and insoluble dietary fibre, minerals, and other bioactive molecules, including B-group vitamins (Abebe and Alemayehu Citation2022, Liyanage et al. Citation2014, Mudryj et al. Citation2012). It is well-known for contributing to human health by providing various health benefits; however, its impact on animal health, particularly its functional compounds, remains unknown. Cowpea has a crude protein ranging from 22 to 30% dry matter (Abdel-Shafi et al. Citation2019b). The high protein level of cowpeas makes it an ideal material for developing protein hydrolysates produced from cowpea beans using enzymatic hydrolysis (Gómez et al. Citation2021, Segura Campos et al. Citation2010). Hydrolysed storage proteins produce peptides of low molecular weight that generate new plant tissues and organs (Osman et al. Citation2021). These peptides may have unique biological functions and health benefits called bioactive peptides (Abdel-Hamid et al. Citation2016, Abdel-Hamid et al. Citation2017, Osman et al. Citation2016).
Cowpea seed protein hydrolysates (CPH) contain antimicrobial peptides with broad antimicrobial activities and a promising modulatory effect on the host’s immune status (Magana et al. Citation2020, Osman et al. Citation2021). Recently, the development of bacterial drug resistance because of the long-term misuse of conventional antibiotics has posed a severe health problem worldwide. Thus, the World Health Organisation (WHO) designated antibiotic resistance as a priority concern, promoting the discovery of novel antibiotics (Lei et al. Citation2019, Magana et al. Citation2020, Mancuso et al. Citation2021). Because the invention of novel antibiotics is challenging, the latest perspectives have been compelled to find an ingenious way to inhibit antibiotic resistance, such as using natural peptides, natural plant extracts, and probiotics (Abdel-Shafi et al. Citation2020, El-Gazzar and Enan Citation2020, Łojewska and Sakowicz Citation2021, Power et al. Citation2014). Similarly, in the poultry industry, the criticism of using antibiotic growth promoters in poultry diets and the rising demand for organic poultry production have challenged producers and researchers to find natural alternatives that have comparable beneficial effects (Abd El-Hack et al. Citation2022, Anadón Citation2006). In this context, cationic antimicrobial peptides (AMPs) or proteins appear to be a promising possibility. Extracted protein from cowpea beans and other legumes has been shown to have potent antibacterial activity (Abdel-Shafi et al. Citation2019a). AMPs have distinct benefits over traditional antibiotics in that they have been effectively utilised for food preservation without forming multidrug-resistant microbes; they have also demonstrated good regulation of the host immune response (Enan Citation2006, Magana et al. Citation2020, Moretta et al. Citation2021).
Beneficial effects on productive performance, intestinal morphology, and gut microbiota were obtained by dietary supplementation of bioactive peptides derived from sesame meal (50-150 mg/kg diet) in broiler chickens (Salavati et al. Citation2020). The study conducted by Landy et al. (Citation2020) showed positive effects of dietary supplementation of bioactive peptides derived from cottonseed (6 g/kg diet) on growth performance, total antioxidant activity, and immune responses in broiler chicken and can be used as antibiotics alternative.
Previous research has tested the chemical composition of different cowpea cultivars and energy contents in poultry diets and showed that cowpeas are suitable for poultry feed (Tshovhote et al. Citation2003). However, additional research is still required to clarify the mechanism(s) underlying the health and growth-promoting effects of CPH in poultry diets. Protein fragments derived from cowpea beans by enzymatic hydrolysis are gaining interest due to their potential activities in treating metabolic disorders and are known to function favourably within the body (Jayathilake et al. Citation2018, Marques et al. Citation2015, Marques et al. Citation2015). A worthy meat quality characteristic is PUFA content, which is valuable for human health (Korakas et al. Citation2018). Blood biochemistry is a variable biochemical system that can show the organism’s state and the changes that occur to it under the effect of internal and external factors. So far, we have not found any research on the effect of CPH on broiler meat quality, blood biochemistry, and immune status. Thus, the current study aims to evaluate the efficacy of incorporating CPH in broiler diets to emphasise its effects on chickens’ growth performance, metabolic response, immunological status, meat quality, and small intestine histomorphology.
Material and methods
Preparation and description of cowpea bean protein hydrolysates
Cowpea protein isolate (CPI) preparation
Cowpea seeds were ground in a coffee grinder and defatted with hexane (1:10 w/v, seeds to solvent ratio) with constant stirring for 2 h at room temperature. The slurry was defatted twice and then filtered on a glass sinter. After drying, the defatted seeds were used for protein extraction, according to Johnson and Brekke (Johnson and Brekke Citation1983).In brief, dispersions of 5% (w/v) defatted cowpea flour in water were adjusted to pH 10 with 0.2 M NaOH at room temperature, shaken for 1 h, and centrifuged for 10 min at 5000 ×g (Beckman GS-6R centrifuge, Brea, CA). The extraction and centrifugation procedures were repeated on the residue to increase the yield. The extracts were combined, and the pH was adjusted to 4.5 with 1 N HCl to precipitate the protein. The proteins were recovered by centrifugation at 5000 ×g for 10 min, then by decantation, removing the supernatant. Crude protein was washed with distilled water, then dispersed in a limited volume of distilled water at pH 7.5, dialysed overnight, and lyophilised. The protein isolated from cowpea flour was about 9 g protein/100 g flour. The amino acid content of cowpea protein isolate was assessed following the methodology described by Simpson et al. (Citation1976), using an amino acid analyser system (Harvard Bioscience, Inc., Holliston, United States), as outlined in the study conducted by Abdel-Shafi et al. (Citation2016). The amino acid profile of the cowpea protein isolate is shown in .
Table 1. Amino acid composition (g amino acid/100 g protein) of cowpea protein isolate.
Cowpea protein hydrolysates (CPH) production and degree of hydrolysis estimation
Enzymatic hydrolysis was performed using pepsin at the optimal conditions in a 0.1 M glycine-HCl buffer with pH 2. Lyophilised CPI was dissolved at 100 g/L in the buffer, adjusted to the hydrolysis conditions, and the enzyme was added at an Enzyme/substrate (E/S) ratio of 1:200. The reaction mixtures were incubated at 37 °C for 5 h with stirring. Hydrolysates were heated in a boiling water bath for 10 min to inactivate the enzyme and clarified by centrifugation at 4000 × g (Beckman GS-6R centrifuge, Brea, CA) for 30 min at 16 °C to remove insoluble substrate fragments. The final supernatants were lyophilised and kept at −20 °C. The degree of hydrolysis was assessed every 1h throughout 5 h hydrolysis, as specified by Osman, El-Araby, et al. (Citation2016). The degree of hydrolysis was assessed using the trichloroacetic acid (TCA) method. After hydrolysis, a volume of 20 ml of protein hydrolysate was combined with an equal volume of TCA (20%; w/v), such that the final concentration reached 10% TCA. The mixture was left to stand for 30 min, followed by 10-min centrifugation at 8000 x g. Finally, the supernatant was analysed for protein content by the Kjeldahl method, and the obtained data were used to conclude the degree of hydrolysis (DH) following the formula below:
Birds, diets, and management
Hatchling male Ross-308 broilers (n = 300) were obtained from a commercial hatchery (Dakahlia Poultry, Mansora, Egypt) and given a 3-day adaption time before the experiment began (starting body weight was 86.72 g ± 0.20). In a completely randomised design, chicks were randomly allocated to five experimental treatments (6 replicates per treatment and ten chicks for each replicate). Birds were fed isocaloric, isonitrogenous corn-soybean meal basal diets supplemented with five levels of CPH, 0 (control group without CPH), 2, 4, 6, and 8 g kg−1 diet. For the first three days, the lighting system was set to 23:1 h light/dark, then to 20:4 h light/dark until the end of the experiment. The study extended 35 days, with mash feed and freshwater fed ad libitum. The managerial conditions and the experimental diets were done following the Ross-308 broilers manual guide (Aviagen Citation2009) (); the starter diet was fed to the chicks until they were 10 days old, followed by the grower and finisher diets from day 11 to 23 and 24 to 35, respectively. Following the end of the experiment, all remaining birds were released.
Table 2. The proximate chemical composition of the experimental diets as fed basis (%).
Growth performance
The average initial body weight (BW) was determined on the fourth day of life. The BW was then assessed on a replicate basis at 10, 23, and 35 days, and body weight gain (BWG) was calculated as a result (Amer et al. Citation2022). The difference in weight between the given feed and the remaining feed was used to calculate feed intake (FI) per replicate. The feed conversion ratio (FCR) was calculated (Brody Citation1945) as follows;
Carcase traits
To calculate the dressing percentage, nine chicks were chosen from each group, weighed, and euthanized using cervical dislocation, according to Amer et al.(Amer et al. Citation2021). The carcases were plucked, eviscerated, and weighed to determine the hot carcase weight. The percentage of dressing was determined as follows:
While the percentages of the internal organs (liver, gizzard (empty with the entire membrane), and intestine) and immune organs (spleen and bursa of Fabricius) were determined as follows:
Sample collection
For blood collection, tissue collection, and carcase trait measurements, 6 birds per treatment were randomly selected. They were slaughtered according to the institutional committee recommendations after 8 h of fasting on day 35 of the experiment. Blood was collected into 3 mL vacutainer tubes (BD Vacutainer, Becton Dickinson, Franklin Lakes, NJ, USA), and the serum was obtained by centrifuging the samples at 3000 g for 15 min at 4 °C. The serum was then stored at–20 °C for further analysis. After blood collection, breast muscle portions were collected from each bird, vacuum-packed, and stored at −20 °C for fatty acid composition analysis. Following the earlier method for histological analysis, 2 cm tissue samples were cut from several sections of the small intestine (duodenum, jejunum, and ileum) and spleen (Sikandar et al. Citation2017).
Micromorphological examination of the small intestine
Intestinal samples were fixed in 10% neutral buffered formaldehyde (NBF) for 72 h. The samples were then dehydrated and cleaned before being embedded in paraffin wax. Histological examination was performed on 5 μm thick transverse sections using a microtome (Leica RM 2155, England) placed on slides and stained with haematoxylin and eosin (Bancroft and Gamble Citation2008). The villous height (VH) was measured from the tip of the villus (with a lamina propria) to the base (villus-crypt junction), and the crypt depth (CD) was estimated from the villus-crypt junction to the crypt’s distal limit (Amer et al. Citation2020). ImageJ software (National Institute of Health, USA) was used to calculate the number of goblet cells per unit of epithelial area (mm2) and single goblet cell areas (m2) in PAS-stained sections.
Fatty acid composition of breast muscle
For the fatty acid analysis, five breast muscle samples (50 g/sample) were collected from each group. As described by Belitz et al. (Citation2009), a chloroform/methanol (2:1, v/v) solvent method was used to extract fat. The extracted fatty acids were then measured according to AOAC (Citation2000)
Blood biochemical analysis
Triiodothyronine (T3), thyroxine (T4), leptin, and growth hormone (GH) were determined using chicken commercial diagnostic ELISA kits (My Biosource Co. San Diego, CA, USA, with CAT. NO. MBS269454, MBS265796, MBS025331, and MBS266317, respectively). An automated biochemical analyser (Robotnik Prietest ECO Ambernath (W), Thane, India) was used to measure blood glucose levels. The methods of Grant (Grant Citation1987) and Doumas et al. (Doumas et al. Citation1981) were used to assess the serum total protein (TP) and albumin levels and the difference between the TP and albumin then calculated the globulin level.
Immune status indices
The serum levels of interleukin 10 (IL10), complement 3 (C3), and interferon-gamma (INF-γ) were tested using the chicken-specific ELISA kits (My BioSource Co. CAT.NO. MBS701683, Life Span Biosciences, Inc. CAT. NO. LS-F9287, and My BioSource co. CAT.NO. MBS220076, respectively). The lysozyme activity in serum was assessed according to the method of Lie et al. (Lie et al. Citation1986).
Immunohistochemical examination
Spleen tissues were collected for immunoglobulin G (IgG) immunohistochemical examination according to Saber et al. (Citation2019) and placed in NBF. The samples were fixed for four days and were subjected to routine histological methods, including dehydration, clearing, embedding, and microtome cutting, as mentioned in the earlier section. The paraffin-embedded sections were deparaffinized in xylene before being rehydrated in ethyl alcohol. As previously mentioned, immunohistochemical staining was performed (Amer et al. Citation2022). The staining intensity was determined using the ImageJ ecosystem (IJ 1.46r, 2012, National Institutes of Health, WA, USA), and data were reported as a percentage of the positive area (immunostained cells). The labelling indices were calculated by counting the number of positive cells in 1000 cells.
Statistical analysis
The data were analysed using SPSS Version 16 for Windows (SPSS Inc., Chicago, IL, USA). Based on polynomial orthogonal contrasts, one-way ANOVA was applied to calculate linear and quadratic regression equations. Tukey’s honestly significant difference test was used to test mean differences. Pooled SEM was listed for all analyses, and P < 0.05 was considered statistically significant.
Results
Degree of hydrolysis (DH)
The degree of hydrolysis (%) of cowpea protein isolate treated with pepsin (E/S ratio 1:200) for 5 h is presented in . The degree of enzymatic degradation increases gradually with time. The enzymatic reaction time, which impacts peptide bond breakage, is typically the cause of variations in DH.
Growth performance
The effects of dietary CPH on broiler growth performance are shown in . Different levels of CPH supplementation to the broiler diet resulted in no significant (P > 0.05) changes in broiler growth performance during the different rearing stages and the overall performance. However, during the finisher period, the feed conversion ratio (FCR) decreased quadratically (P < 0.05) at concentrations 2 and 4 g kg −1 compared to the control.
Table 3. Effect of dietary Cowpea protein hydrolysate (CPH) on the growth performance of broiler chickens.
Carcase traits
Dietary CPH had no significant (p > 0.05) effect on carcase, intestine, liver, spleen, bursa, and gizzard weights relative to live weight, as shown in .
Table 4. Effect of dietary Cowpea protein hydrolysate (CPH) on the carcase traits (%) relative to live weight.
Histomorphology of the small intestine
The histomorphology of broilers’ small intestines is shown in , . A linear (P < 0.05) increase in duodenal villus height (VH) and goblet cell count (GCC) was shown in response to dietary CPH. Likewise, the dietary CPH resulted in a quadratic change (P < 0.05) in duodenal GCC. Birds fed the 6 g CPH kg−1 had an increase (P < 0.05) in crypt depth (CD) compared to other dietary treatments. Birds fed 8 g CPH kg−1 showed increased VH: CD compared to the control. However, other dietary treatments showed a significant (P < 0.05) decrease in CD. The villi width (VW) decreased (P < 0.05) at the level of 8 g CPH kg−1 compared to the control. In the jejunum, the VH and GCC linearly and quadratically (P < 0.05) increased in response to dietary supplementation of CPH. A quadratic (P < 0.05) change was reported in the jejunal CD and VH: CD in response to dietary CPH supplementation. The jejunal VW did not show a significant (p > 0.05) change between all dietary treatments. Birds fed 6 g CPH kg−1 had an increase (P < 0.05) in ileal VH and GCC compared to the control. However, the 8 g CPH kg−1 resulted in a significant (P < 0.05) decrease in CD compared to the 6 g CPH kg−1 treatment. Ileal VW and VH: CD did not show a significant (p > 0.05) difference between all dietary treatments.
Figure 2. Photomicrograph of the small intestine (duodenum (I), jejunum (II), and ileum (III)) stained with haematoxylin and eosin. A: Cowpea protein hydrolysate (CPH)0, B: CPH2, C: CPH4, D: CPH6, and E: CPH8.

Table 5. Effect of dietary Cowpea protein hydrolysate (CPH) on the morphometric measures (μm) of the small intestine of broiler chickens.
Fatty acid composition of the breast muscle
The CPH-supplied groups showed linear (P < 0.05) increase in linoleic acid (18:2n-6c), ∝-linolenic acid (ALA) (18:3n-3), arachidonic acid (20:4n-6), eicosapentaenoic acid (EPA) (20:5n-3), docosahexaenoic acid (DHA) (22:6n-3), total n-3 PUFA, n-6 PUFA, and the n-3/n-6 PUFA ratio in broilers’ breast muscle ().
Table 6. Effect of dietary Cowpea protein hydrolysate (CPH) on the fatty acid composition of the breast muscle in broiler chickens.
Blood biochemical parameters
shows the impacts of dietary CPH on blood biochemical parameters. Compared to the control, dietary CPH linearly (P < 0.05) increased the triiodothyronine (T3), thyroxin (T4), and growth hormone (GH) levels; additionally, the dietary CPH produced a quadratic (P < 0.05) change in T4 level. However, the serum glucose and leptin levels did not show significant (p > 0.05) changes in response to the inclusion of CPH into broiler diets.
Table 7. Effect of dietary Cowpea protein hydrolysate (CPH) on blood biochemical parameters of broiler chickens.
Immune status
As shown in , CPH supplementation to the broiler diet led to linear and quadratic (P < 0.05) increases in interferon-γ (INF-γ), total protein (TP), albumin, globulin, lysozyme, and interleukin10 (IL10) levels. The complement3 (C3) level showed a linear (P < 0.05) increase in CPH-supplemented treatments compared with the control.
Table 8. Effect of dietary Cowpea protein hydrolysate (CPH) on immune indices of broiler chickens.
Immunohistochemical analysis
The photomicrograph of splenic tissues immunostained with immunoglobulin G (IgG) antibody and the morphometric measures are illustrated in . The dietary CPH levels 4-8 g Kg−1 showed linear and quadratic upregulation of the immunostaining of IgG in the spleen of these groups (p < 0.01) compared to the control group (CPH0). The IgG immunostaining percentage was 31, 37, 56, 61, and 62% for the CPH0, CPH2, CPH4, CPH6, and CPH8 groups, respectively.
Discussion
Using CPH as a dietary supplement has a lot of appeal; nonetheless, its application in poultry feed is still in its infancy. Few applications have been reported in the literature concerning physically treated cowpea protein byproducts in poultry feed or the functional properties of cowpea in human health compared to the inclusion of CPH into chicken feed (Adebiyi et al. Citation2010, Jayathilake et al. Citation2018). The poultry industry’s requests for novel feed supplements to enhance poultry performance and production are boosting the application of CPH thanks to its high functional properties. The current study found that adding CPH to broiler diets did not affect growth performance or carcase traits, except for a quadratically decreased FCR during the finisher period at 2 and 4 g kg −1. This may be explained by the fact that birds’ maximum utilisation of CPH to improve the FCR was achieved at dietary supplementation up to 4 g CPH kg −1, then declined to be as the control group at levels 6 – 8 g CPH kg −1. Similarly, Salavati et al. (Citation2020) reported improved FCR on days 11–24 and 1–32 in broiler chickens fed diets supplemented with bioactive peptides derived from sesame meal (100 mg/kg diet). The results conducted by Abdollahi et al. (Citation2017) indicated that dietary supplementation of soybean bioactive peptides (1-6 g/kg diet) had no significant effects on the daily feed intake of broilers. Landy et al. (Citation2020) reported increased daily feed intake of broilers by dietary supplementation of cottonseed bioactive peptides (5 g/kg) throughout the experimental period.
Dietary CPH had an increased metabolic effect, as exampled by linear and quadratic increases in T3, T4, and GH levels. Indeed, blood biochemistry indices in poultry provide useful content about their general health and the nutritional value of the diets provided (Islam et al. Citation2019). Thyroid hormones are known in this context for their effect on body metabolism and development. They have a biphasic effect on protein and lipid metabolism and promote growth and differentiation during the developmental stages (Darras et al. Citation2000). The increase in T3 and T4 levels in the current experiment appears to indicate that CPH has a positive impact on the metabolic status of the broiler. Meanwhile, the increase in GH level in response to dietary CPH supplementation may support the positive relationship between CPH supplementation and broiler metabolic status. So far, peptides resulting from the hydrolysis of plant proteins are included in the diet for feeding pigs, poultry, fish, and companion animals. The results are positive for improved gut health, growth, and productive performance (McCalla et al. Citation2010). The primary mechanisms may be the absorption rate of small peptides is greater than that of an equal amount of free amino acids; the rate of catabolism of small peptides by small intestinal bacteria is less than the rate of the equivalent amount of free amino acids; providing functional AAs (e.g. glutamate, glutamine, arginine, glycine, proline, and taurine) to improve muscle protein synthesis and antioxidant reactions (Hou et al. Citation2016, Hou et al. Citation2015). Studies involving peptides addition to diets of post-weaning pigs showed improved palatability, feed efficiency, growth, and health [53–58]. Furthermore, peptide products have been added to the diets of poultry (Frikha et al. Citation2014, Opheim et al. Citation2016), fish (Abdel Rahman et al. Citation2023, Ibrahim et al. Citation2023), and companion animals (Nagodawithana et al. Citation2010) to enhance their nutritional status, intestinal function, and infection-fighting abilities.
Our findings revealed that dietary CPH did not change the serum glucose and leptin hormone levels. According to previous research, phenolic extracts of cowpea have hypoglycaemic effects in vitro via inhibition of ∝-amylase and ∝-glucosidase enzymatic activity, which aids in slowing the release of glucose into the circulation and restricting the steep increase in postprandial blood glucose level (Sreerama et al. Citation2012). Because glucose is the most common circulating carbohydrate in birds and its blood level is frequently used as a biometric indicator of general health, blood glucose levels should be measured (Kawasaki et al. Citation2020). Based on the current study, a stable blood glucose level may indicate a stable health status; however, more research is needed to confirm such impact of dietary CPH on poultry.
Dietary inclusion of CPH in broilers improved intestinal VH and GCC in various parts of the small intestine. In broilers, the small intestine, particularly the duodenum and jejunum, is critical in the digestion and absorption of feed nutrients (Gao et al. Citation2008). Such intestinal development is typically evaluated using VH, VW, and CD, with longer and broader villus and lower CD resulting in greater digestive efficiency (Zeitz et al. Citation2015). Duodenal and jejunal VH increased linearly and quadratically, with ileal VH increasing significantly at 6 g CPH kg−1. However, an improvement in VH histomorphology was not accompanied by an improvement in VW. The intestinal villi’s length and width influence the small intestine’s surface area, affecting the ability to digest and absorb feed, increasing broiler chicken productivity (Yamauchi Citation2002). In the same context, the surface area of the small intestine may be related to an increase in its weight and length (Yamauchi Citation2002), which was not demonstrated in our study because no significant change was observed in the intestinal percentage relative to the live weight among the experimental groups.
Furthermore, a shallower CD was not detected at the different inclusion levels of CPH compared to the control. As previously reported, the crypt is the area where stem cells divide to allow villus renewal; a large crypt indicates rapid tissue turnover and an increased mandate for new tissue; thus, shallower crypts indicate the sustained survival of villi without the necessity for renewal, with lower energy expenditure for this process and hence enabled other tissues growth (Biasato et al. Citation2018, Miles et al. Citation2006, Xia et al. Citation2004). In parallel, we reported linear and quadratic increases in the duodenal and jejunal GCC, with a significant increase in ileum at the 6 g CPH kg−1. These results are consistent with earlier research that showed that dietary micronutrients and probiotics in broilers increased the number of small intestinal cells that contain acidic mucin (Shah et al. Citation2019). According to reports, this increase in the GCC was a significant inducer for the secretion of bioactive factors, either directly for the GC or indirectly through host immune cell activation, which improved the birds’ general health.
Our findings on the fatty acid profile in breast broiler muscle showed a linear increase in all detected fatty acids and an increase in the n-3/n-6 PUFA ratio. According to earlier studies, different dietary supplemental protein sources to the diets of broiler chickens caused noticeable changes in the FA composition of the breast and thigh muscles (Ciurescu et al. Citation2017, Laudadio et al. Citation2011). Like our findings, other colleagues found that dietary cowpea protein significantly increased n-3 FAs, particularly for those that may benefit human nutrition, such as ALA and EPA (Ciurescu et al. Citation2022). However, in our study, we used the protein hydrolysate from the cowpea seeds, which relies on protein conformation and structure-function relationships linked to enzymatic hydrolysis to deliver its biological activities primarily due to their bioactive peptides (Ghribi et al. Citation2015). Even such prior work by Ciurescu and colleagues (Ciurescu et al. Citation2022) used untreated cowpea seed in the diet of broilers; we still can speculate that CPH has a favourable impact on the quality of broilers’ meat, which is advantageous for consumer health.
Enzymatic hydrolysis of proteins forms bioactive peptides with immunomodulatory and antibacterial functions (Kotzamanis et al. Citation2007). The immunomodulatory activity of peptides is generated by regulating cytokines, lymphocyte proliferation, antibody synthesis, and natural killer cell activity (Fitzgerald and Murray Citation2006, Hartmann and Meisel Citation2007, Horiguchi et al. Citation2005). Their immune-boosting effect varies depending on the size and level of hydrolysates. In the present study, we reported an increase in the assessed immune indices in response to dietary CPH. Interestingly, an increase in INF-γ and IL10 levels was observed in response to dietary CPH. These cytokines are considered major immune status effectors; however, INF-γ has been identified as a pro-inflammatory cytokine that regulates anti-inflammatory responses by antagonising IL-10 (Herrero et al. Citation2003, Yu et al. Citation2009). Earlier literature assessed the cowpea’s anti-inflammatory activity concerning their bioactive compounds and revealed that it inhibited the production of pro-inflammatory cytokines (Lee et al. Citation2011, Ojwang et al. Citation2015). Thus, to properly test the host biological response to dietary CPH, it is necessary to conduct additional research in poultry in light of the controversial findings made thus far.
Furthermore, an increase in TP, albumin, and globulin levels was evidenced in the current study, indicating that there has been an improvement in birds’ health because of improved nutrient absorption and utilisation with dietary inclusion of CPH. Furthermore, we found an increase in serum lysozymes and C3 levels and upregulation of the immunostaining of IgG in the spleen, indicating that dietary CPH has an immune-modulating effect in broiler chickens, which could be attributed to its phenolic compounds and its other functional components (Liyanage et al. Citation2014).C3 has been reported to work on triggering inflammation as a defense mechanism against foreign invaders; additionally, the lysozymes know to eliminate intestinal pathogens in poultry via different mechanisms, including stimulation of macrophages’ phagocytic functions. Thus, in light of the earlier assessment of the cowpea’s anti-inflammatory activity, further investigation of dietary CPH concerning C3 and lysozymes production levels in poultry is still required (Lee et al. Citation2011, Ricklin et al. Citation2016, Xia et al. Citation2019). Recently, it has been reported that plant protein-hydrolysates have immunostimulant and antimicrobial effects (Abdel Rahman et al. Citation2023, Amer et al. Citation2024), as reported for some endogenous peptides in the small intestine (Bevins and Salzman Citation2011). These antimicrobial peptides exert their actions by destroying the bacterial cell membrane, inhibiting the functions of their intracellular proteins, stimulating the accumulation of cytoplasmic proteins, and altering bacterial metabolism (Lima et al. Citation2015, Wald et al. Citation2016). Improved immune responses have also been reported by dietary supplementation of cottonseed bioactive peptides in broiler chickens (Landy et al. Citation2020). Osho et al. (Citation2019) showed ameliorated coccidia infection in broiler chicken by dietary supplementation of soybean bioactive peptide. They explained the immunomodulatory effects of bioactive peptides by increasing the production of TNF-α, IL-8, IL-10, and IL6 due to Toll-like receptor stimulation.
Conclusions
From the current study, it can be concluded that CPH did not positively affect the broiler growth performance or carcase traits, except for a reduction in FCR during the finisher period at levels 2 and 4 g CPH/kg diet. As shown by increases in T3, T4, GH, TP, albumin, globulin, lysozymes, and C3 serum levels, as well as an upregulation of IgG immunostaining in the spleen, it did, however, improve the chickens’ metabolic and immune status in a concentration-dependent manner. Our results indicate that dietary supplementation with CPH would be feasible in the production of broilers; however, they still call for further study because CPH application in poultry feed is still in its early stages.
Ethical approval
All methods and procedures used in this study were approved by the Committee of Animal Welfare and Research Ethics guidelines at Zagazig University, Egypt (Approval No. ZU-IACUC/2/F/15/2022). All methods in the study were carried out following relevant institutional guidelines, and all animal experiments were performed following the ARRIVE guidelines.
Acknowledgement
This work was supported by Researchers Supporting project number (RSPD2023R700), King Saud University, Riyadh, Saudi Arabia.
Data availability statement
The datasets generated and/or analysed during the current study are not publicly available due to institution roles and permission but are available from the corresponding author upon reasonable request.
.
References
- Abd El-Hack ME, El-Saadony MT, Salem HM, El-Tahan AM, Soliman MM, Youssef GBA, Taha AE, Soliman SM, Ahmed AE, El-Kott AF, et al. 2022. Alternatives to antibiotics for organic poultry production: types, modes of action and impacts on bird’s health and production. Poult Sci. 101(4):101696. doi: 10.1016/j.psj.2022.101696.
- Abdel-Hamid M, Goda HA, De Gobba C, Jenssen H, Osman A. 2016. Antibacterial activity of papain hydrolysed camel whey and its fractions. Int Dairy J. 61:91–98. doi: 10.1016/j.idairyj.2016.04.004.
- Abdel-Hamid M, Otte J, De Gobba C, Osman A, Hamad E. 2017. Angiotensin I-converting enzyme inhibitory activity and antioxidant capacity of bioactive peptides derived from enzymatic hydrolysis of buffalo milk proteins. Int Dairy J. 66:91–98. doi: 10.1016/j.idairyj.2016.11.006.
- Abdel-Shafi S, Al-Mohammadi A-R, Almanaa TN, Moustafa AH, Saad TM, Ghonemey A-R, AnACarso I, Enan G, El-Gazzar N. 2020. Identification and testing of antidermatophytic oxaborole-6-benzene sulphonamide derivative (OXBS) from streptomyces atrovirens KM192347 isolated from soil. Antibiotics. 9(4):176. doi: 10.3390/antibiotics9040176.
- Abdel-Shafi S, Al-Mohammadi A-R, Osman A, Enan G, Abdel-Hameid S, Sitohy M. 2019. Characterization and antibacterial activity of 7S and 11S globulins isolated from cowpea seed protein. Molecules. 24(6):1082. doi: 10.3390/molecules24061082.
- Abdel-Shafi S, Osman A, Enan G, El-Nemer M, Sitohy M. 2016. Antibacterial activity of methylated egg white proteins against pathogenic G + and G − bacteria matching antibiotics. SpringerPlus. 5(1):983. doi: 10.1186/s40064-016-2625-3.
- Abdel Rahman AN, Amer SA, Behairy A, Younis EM, Abdelwarith AA, Osman A, Moustafa AA, Davies SJ, Ibrahim RE. 2023. Using Azadirachta indica protein hydrolysate as a plant protein in Nile tilapia (Oreochromis niloticus) diet: effects on the growth, economic efficiency, antioxidant‐immune response and resistance to Streptococcus agalactiae. J Anim Physiol Anim Nutr. In press. doi: 10.1111/jpn.13857.
- Abdel Rahman AN, Amer SA, Masoud SR, El-Saber MM, Osman A, Younis EM, Abdelwarith AA, Davies SJ, Khamis T, Ibrahim RE. 2023. Neem seed protein hydrolysate as a fishmeal substitute in Nile tilapia: effects on antioxidant/immune pathway, growth, amino acid transporters-related gene expression, and Aeromonas veronii resistance. Aquaculture. 573:739593. doi: 10.1016/j.aquaculture.2023.739593.
- Abdollahi M, Zaefarian F, Gu Y, Xiao W, Jia J, Ravindran V. 2017. Influence of soybean bioactive peptides on growth performance, nutrient utilisation, digestive tract development and intestinal histology in broilers. JAAN. 5:e7. doi: 10.1017/JAN.2017.6.
- Abebe BK, Alemayehu MT. 2022. A review of the nutritional use of cowpea (Vigna unguiculata L. Walp) for human and animal diets. JAgric Food Res. 10:100383. doi: 10.1016/j.jafr.2022.100383.
- Adebiyi O, Ologhobo A, Adu O, Olasehinde T. 2010. Evaluation of the nutritional potentials of physically treated cowpea seed hulls in poultry feed. Emir J Food Agric. 22(3):232–239. doi: 10.9755/ejfa.v22i3.4893.
- Amer SA, A-Nasser A, Al-Khalaifah HS, AlSadek DMM, Abdel Fattah DM, Roushdy EM, Sherief WRIA, Farag MFM, Altohamy DE, Abdel-Wareth AAA, et al. 2020. Effect of dietary medium-chain α-monoglycerides on the growth performance, intestinal histomorphology, amino acid digestibility, and broiler chickens’ blood biochemical parameters. Animals. 11(1):57. doi: 10.3390/ani11010057.
- Amer SA, Al-Khalaifah HS, Gouda A, Osman A, Goda NI, Mohammed HA, Darwish MI, Hassan AM, Mohamed SKA. 2022. Potential effects of anthocyanin-rich roselle (Hibiscus sabdariffa L.) extract on the growth, intestinal histomorphology, blood biochemical parameters, and the immune status of broiler chickens. Antioxidants. 11(3):544. doi: 10.3390/antiox11030544.
- Amer SA, Mohamed WA, Gharib HS, Al-Gabri NA, Gouda A, Elabbasy MT, El-Rahman A, Ghada I, Omar AE. 2021. Changes in the growth, ileal digestibility, intestinal histology, behavior, fatty acid composition of the breast muscles, and blood biochemical parameters of broiler chickens by dietary inclusion of safflower oil and vitamin C. BMC Vet Res. 17(1):68. doi: 10.1186/s12917-021-02773-5.
- Amer SA, Rahman ANA, ElHady M, Osman A, Younis EM, Abdel-Warith A-WA, Moustafa AA, Khamis T, Davies SJ, Ibrahim RE. 2024. Use of moringa protein hydrolysate as a fishmeal replacer in diet of Oreochromis niloticus: effects on growth, digestive enzymes, protein transporters and immune status. Aquaculture. 579:740202. doi: 10.1016/j.aquaculture.2023.740202.
- Anadón A. 2006. WS14 The EU ban of antibiotics as feed additives (2006): alternatives and consumer safety. J Vet Pharm Ther. 29(s1):41–44. doi: 10.1111/j.1365-2885.2006.00775_2.x.
- AOAC. 2000. Official methods of analysis of AOAC International.
- Apata DF, Ologhobo AD. 1997. Trypsin inhibitor and other anti-nutritional factors in tropical legume seeds. Tropical Science (United Kingdom
- Aviagen R. 2009. Ross broiler management manual. Scotland, UK: www aviagen pp.1–114.
- Bancroft JD, Gamble M. 2008. Theory and practice of histological techniques: Elsevier health sciences. Amsterdam, The Netherlands.
- Belitz H-D, Grosch W, Schieberle P. 2009. Meat. Food chemistry. Berlin, Heidelberg: Springer-Verlag; p. 563–616. doi: 10.1007/978-3-540-69934-7_13.
- Bevins CL, Salzman NH. 2011. Paneth cells, antimicrobial peptides and maintenance of intestinal homeostasis. Nat Rev Microbiol. 9(5):356–368. doi: 10.1038/nrmicro2546.
- Biasato I, Ferrocino I, Biasibetti E, Grego E, Dabbou S, Sereno A, Gai F, Gasco L, Schiavone A, Cocolin L, et al. 2018. Modulation of intestinal microbiota, morphology and mucin composition by dietary insect meal inclusion in free-range chickens. BMC Vet Res. 14(1):383. doi: 10.1186/s12917-018-1690-y.
- Brody S. 1945. Bioenergetics and growth; with special reference to the efficiency complex in domestic animals.
- Ciurescu G, Idriceanu L, Gheorghe A, Ropotă M, Drăghici R. 2022. Meat quality in broiler chickens fed on cowpea (Vigna unguiculata [L.] Walp) seeds. Sci Rep. 12(1):9685. doi: 10.1038/s41598-022-13611-5.
- Ciurescu G, Vasilachi A, Ropota M, Palade M, Dragomir C. 2017. Beneficial effects of increasing dietary levels of raw lentil seeds on meat fatty acid and plasma metabolic profile in broiler chickens. Indian J Anim Sci. 87:1385–1390.
- Darras VM, Van der Geyten S, Kühn ER. 2000. Thyroid hormone metabolism in poultry. Biotechnol. Agron. Soc. Environ. 4(1):13–20.
- Doumas BT, Bayse DD, Carter RJ, Peters T, Jr, Schaffer R. 1981. A candidate reference method for determination of total protein in serum. I. Development and validation. Clin Chem. 27(10):1642–1650. doi: 10.1093/clinchem/27.10.1642.
- El-Gazzar NS, Enan G. 2020. Advances in phage inspired nanoscience based therapy. In: nanoBioMedicine. Springer. p. 237–257.
- Enan G. 2006. Behaviour of Listeria monocytogenes LMG 10470 in poultry meat and its control by the bacteriocin Plantaricin UG 1. International J of Poultry Science. 5(4):355–359. doi: 10.3923/ijps.2006.355.359.
- Fitzgerald RJ, Murray BA. 2006. Bioactive peptides and lactic fermentations. Int J of Dairy Tech. 59(2):118–125. doi: 10.1111/j.1471-0307.2006.00250.x.
- Frikha M, Mohiti-Asli M, Chetrit C, Mateos G. 2014. Hydrolyzed porcine mucosa in broiler diets: effects on growth performance, nutrient retention, and histomorphology of the small intestine. Poult Sci. 93(2):400–411. doi: 10.3382/ps.2013-03376.
- Gao J, Zhang H, Yu S, Wu S, Yoon I, Quigley J, Gao Y, Qi G. 2008. Effects of yeast culture in broiler diets on performance and immunomodulatory functions. Poult Sci. 87(7):1377–1384. doi: 10.3382/ps.2007-00418.
- Ghribi AM, Gafsi IM, Sila A, Blecker C, Danthine S, Attia H, Bougatef A, Besbes S. 2015. Effects of enzymatic hydrolysis on conformational and functional properties of chickpea protein isolate. Food Chem. 187:322–330. doi: 10.1016/j.foodchem.2015.04.109.
- Gómez A, Gay C, Tironi V, Avanza MV. 2021. Structural and antioxidant properties of cowpea protein hydrolysates. Food Biosci. 41:101074. doi: 10.1016/j.fbio.2021.101074.
- Grant GH. 1987. Amino acids and proteins. Fundamentals of clinical chemistry.
- Hartmann R, Meisel H. 2007. Food-derived peptides with biological activity: from research to food applications. Curr Opin Biotechnol. 18(2):163–169. doi: 10.1016/j.copbio.2007.01.013.
- Herrero C, Hu X, Li WP, Samuels S, Sharif MN, Kotenko S, Ivashkiv LB. 2003. Reprogramming of IL-10 activity and signaling by IFN-γ. J Immunol. 171(10):5034–5041. doi: 10.4049/jimmunol.171.10.5034.
- Horiguchi N, Horiguchi H, Suzuki Y. 2005. Effect of wheat gluten hydrolysate on the immune system in healthy human subjects. Biosci Biotechnol Biochem. 69(12):2445–2449. doi: 10.1271/bbb.69.2445.
- Hou Y, Yao K, Yin Y, Wu G. 2016. Endogenous synthesis of amino acids limits growth, lactation, and reproduction in animals. Adv Nutr. 7(2):331–342. doi: 10.3945/an.115.010850.
- Hou Y, Yin Y, Wu G. 2015. Dietary essentiality of “nutritionally non-essential amino acids” for animals and humans. Exp Biol Med (Maywood). 240(8):997–1007. doi: 10.1177/1535370215587913.
- Ibrahim RE, Tolba SA, Younis EM, Abdel-Warith A-WA, Shalaby SI, Osman A, Khamis T, Eissa MA, Davies SJ, Amer SA. 2023. Kidney bean protein hydrolysate as a fish meal replacer: effects on growth, digestive enzymes, metabolic functions, immune-antioxidant parameters and their related gene expression, intestinal and muscular gene expression. Aquaculture. 575:739803. doi: 10.1016/j.aquaculture.2023.739803.
- Islam MR, Lepp D, Godfrey DV, Orban S, Ross K, Delaquis P, Diarra MS. 2019. Effects of wild blueberry (Vaccinium angustifolium) pomace feeding on gut microbiota and blood metabolites in free-range pastured broiler chickens. Poult Sci. 98(9):3739–3755. doi: 10.3382/ps/pez062.
- Jayathilake C, Visvanathan R, Deen A, Bangamuwage R, Jayawardana BC, Nammi S, Liyanage R. 2018. Cowpea: an overview on its nutritional facts and health benefits. J Sci Food Agric. 98(13):4793–4806. doi: 10.1002/jsfa.9074.
- Johnson EA, Brekke CJ. 1983. Functional properties of acylated pea protein isolates. J Food Sci. 48(3):722–725. JoFS. doi: 10.1111/j.1365-2621.1983.tb14883.x.
- Kawasaki T, Iwasaki T, Ohya I, Hasegawa Y, Noguchi M, Watanabe T. 2020. Effects of sampling and storage method on chicken blood glucose measurement. J Poult Sci. 57(3):241–245. doi: 10.2141/jpsa.0190106.
- Korakas E, Dimitriadis G, Raptis A, Lambadiari V. 2018. Dietary composition and cardiovascular risk: a mediator or a bystander? Nutrients. 10(12):1912. doi: 10.3390/nu10121912.
- Kotzamanis Y, Gisbert E, Gatesoupe F, Infante JZ, Cahu C. 2007. Effects of different dietary levels of fish protein hydrolysates on growth, digestive enzymes, gut microbiota, and resistance to Vibrio anguillarum in European sea bass (Dicentrarchus labrax) larvae. Comp Biochem Physiol A Mol Integr Physiol. 147(1):205–214. doi: 10.1016/j.cbpa.2006.12.037.
- Landy N, Kheiri F, Faghani M. 2020. Evaluation of cottonseed bioactive peptides on growth performance, carcase traits, immunity, total antioxidant activity of serum and intestinal morphology in broiler chickens. Ital J Anim Sci. 19(1):1375–1386. doi: 10.1080/1828051X.2020.1844085.
- Laudadio V, Ceci E, Tufarelli V. 2011. Productive traits and meat fatty acid profile of broiler chickens fed diets containing micronized fava beans (Vicia faba L. var. minor) as the main protein source. J Appl Poult Res. 20(1):12–20. doi: 10.3382/japr.2010-00173.
- Lee SM, Lee TH, Cui E-J, Baek N-I, Hong SG, Chung I-S, Kim J. 2011. Anti-inflammatory effects of cowpea (Vigna sinensis K.) seed extracts and its bioactive compounds. J Korean Soc Appl Biol Chem. 54(5):710–717. doi: 10.1007/BF03253149.
- Lei J, Sun L, Huang S, Zhu C, Li P, He J, Mackey V, Coy DH, He Q. 2019. The antimicrobial peptides and their potential clinical applications. Am J Transl Res. 11:3919.
- Lie Ø, Syed M, Solbu H. 1986. Improved agar plate assays of bovine lysozyme and haemolytic complement activity. Acta Vet Scand. 27(1):23–32. doi: 10.1186/BF03548556.
- Lima CA, Campos JF, Filho JLL, Converti A, da Cunha MGC, Porto AL. 2015. Antimicrobial and radical scavenging properties of bovine collagen hydrolysates produced by Penicillium aurantiogriseum URM 4622 collagenase. J Food Sci Technol. 52(7):4459–4466. doi: 10.1007/s13197-014-1463-y.
- Liyanage R, Perera O, Weththasinghe P, Jayawardana B, Vidanaarachchi J, Sivakanesan R. 2014. Nutritional properties and antioxidant content of commonly consumed cowpea cultivars in Sri Lanka. J Food Legumes. 27:215–217.
- Łojewska E, Sakowicz T. 2021. An alternative to antibiotics: selected methods to combat zoonotic foodborne bacterial infections. Curr Microbiol. 78(12):4037–4049. doi: 10.1007/s00284-021-02665-9.
- Magana M, Pushpanathan M, Santos AL, Leanse L, Fernandez M, IOAnnidis A, Giulianotti MA, Apidianakis Y, Bradfute S, Ferguson AL, et al. 2020. The value of antimicrobial peptides in the age of resistance. Lancet Infect Dis. 20(9):e216–e230. doi: 10.1016/S1473-3099(20)30327-3.
- Mancuso G, Midiri A, Gerace E, Biondo C. 2021. Bacterial antibiotic resistance: the most critical pathogens. Pathogens. 10(10):1310. doi: 10.3390/pathogens10101310.
- Marques MR, Fontanari GG, Pimenta DC, Soares-Freitas RM, Arêas JAG. 2015. Proteolytic hydrolysis of cowpea proteins is able to release peptides with hypocholesterolemic activity. Food Res Int. 77:43–48. doi: 10.1016/j.foodres.2015.04.020.
- Marques MR, Freitas RAMS, Carlos ACC, Siguemoto ÉS, Fontanari GG, Arêas JAG. 2015. Peptides from cowpea present antioxidant activity, inhibit cholesterol synthesis and its solubilisation into micelles. Food Chem. 168:288–293. doi: 10.1016/j.foodchem.2014.07.049.
- McCalla J, Waugh T, Lohry E. 2010. Protein hydrolysates/peptides in animal nutrition. In: Pasupuleti V, Demain A, editors. Protein Hydrolysates in Biotechnology. Dordrecht (NL): Springer; p. 179–190. © Springer Science+Business Media B.V. doi: 10.1007/978-1-4020-6674-0_10.
- Mekonnen TW, Gerrano AS, Mbuma NW, Labuschagne MT. 2022. Breeding of vegetable cowpea for nutrition and climate resilience in Sub-Saharan Africa: progress, opportunities, and challenges. Plants. 11(12):1583. doi: 10.3390/plants11121583.
- Miles R, Butcher G, Henry P, Littell R. 2006. Effect of antibiotic growth promoters on broiler performance, intestinal growth parameters, and quantitative morphology. Poult Sci. 85(3):476–485. doi: 10.1093/ps/85.3.476.
- Moretta A, Scieuzo C, Petrone AM, Salvia R, Manniello MD, Franco A, Lucchetti D, Vassallo A, Vogel H, Sgambato A, et al. 2021. Antimicrobial peptides: a new hope in biomedical and pharmaceutical fields. Front Cell Infect Microbiol. 11:668632. doi: 10.3389/fcimb.2021.668632.
- Mudryj AN, Yu N, Hartman TJ, Mitchell DC, Lawrence FR, Aukema HM. 2012. Pulse consumption in Canadian adults influences nutrient intakes. Br J Nutr. 108 Suppl 1(S1):S27–S36. doi: 10.1017/S0007114512000724.
- Nagodawithana TW, Nelles L, Trivedi NB. 2010. Protein hydrolysates as hypoallergenic, flavors and palatants for companion animals. In Pasupuleti V, Demain A, editors. Protein Hydrolysates in Biotechnology. Dordrecht (NL): Springer; p. 191–207. © Springer Science+Business Media B.V. doi: 10.1007/978-1-4020-6674-0_11.
- Ohanenye IC, Ekezie F-GC, Sarteshnizi RA, Boachie RT, Emenike CU, Sun X, Nwachukwu ID, Udenigwe CC. 2022. Legume seed protein digestibility as influenced by traditional and emerging physical processing technologies. Foods. 11(15):2299. doi: 10.3390/foods11152299.
- Ojwang LO, Banerjee N, Noratto GD, Angel-Morales G, Hachibamba T, Awika JM, Mertens-Talcott SU. 2015. Polyphenolic extracts from cowpea (Vigna unguiculata) protect colonic myofibroblasts (CCD18Co cells) from lipopolysaccharide (LPS)-induced inflammation–modulation of microRNA 126. Food Funct. 6(1):146–154. doi: 10.1039/c4fo00459k.
- Opheim M, Sterten H, Øverland M, Kjos N. 2016. Atlantic salmon (Salmo salar) protein hydrolysate–Effect on growth performance and intestinal morphometry in broiler chickens. Livestock Science. 187:138–145. doi: 10.1016/j.livsci.2016.03.005.
- Osho S, Xiao W, Adeola O. 2019. Response of broiler chickens to dietary soybean bioactive peptide and coccidia challenge. Poult Sci. 98(11):5669–5678. doi: 10.3382/ps/pez346.
- Osman A, El-Araby GM, Taha H. 2016. Potential use as a bio-preservative from lupin protein hydrolysate generated by alcalase in food system. J Appl Biol Biotechnol. 4:076–081.
- Osman A, Enan G, Al-Mohammadi A-R, Abdel-Shafi S, Abdel-Hameid S, Sitohy MZ, El-Gazzar N. 2021. Antibacterial peptides produced by alcalase from Cowpea seed proteins. Antibiotics. 10(7):870. doi: 10.3390/antibiotics10070870.
- Osman A, Goda HA, Abdel-Hamid M, Badran SM, Otte J-F, . 2016. Antibacterial peptides generated by Alcalase hydrolysis of goat whey. LWT - Food Sci Technolo. 65:480–486. doi: 10.1016/j.lwt.2015.08.043.
- Power O, Nongonierma AB, Jakeman P, FitzGerald RJ. 2014. Food protein hydrolysates as a source of dipeptidyl peptidase IV inhibitory peptides for the management of type 2 diabetes. Proc Nutr Soc. 73(1):34–46. doi: 10.1017/S0029665113003601.
- Ricklin D, Reis ES, Mastellos DC, Gros P, Lambris JD. 2016. Complement component C3–The “Swiss Army Knife” of innate immunity and host defense. Immunol Rev. 274(1):33–58. doi: 10.1111/imr.12500.
- Saber S, Khalil RM, Abdo WS, Nassif D, El-Ahwany E. 2019. Olmesartan ameliorates chemically-induced ulcerative colitis in rats via modulating NFκB and Nrf-2/HO-1 signaling crosstalk. Toxicol Appl Pharmacol. 364:120–132. doi: 10.1016/j.taap.2018.12.020.
- Salavati ME, Rezaeipour V, Abdullahpour R, Mousavi N. 2020. Effects of graded inclusion of bioactive peptides derived from sesame meal on the growth performance, internal organs, gut microbiota and intestinal morphology of broiler chickens. Int J Pept Res Ther. 26(3):1541–1548. doi: 10.1007/s10989-019-09947-8.
- Segura Campos MR, Chel Guerrero LA, Betancur Ancona DA. 2010. Angiotensin‐I converting enzyme inhibitory and antioxidant activities of peptide fractions extracted by ultrafiltration of cowpea Vigna unguiculata hydrolysates. J Sci Food Agric. 90(14):2512–2518. doi: 10.1002/jsfa.4114.
- Shah M, Zaneb H, Masood S, Khan RU, Ashraf S, Sikandar A, Rehman HFU, Rehman HU. 2019. Effect of dietary supplementation of zinc and multi-microbe probiotic on growth traits and alteration of intestinal architecture in broiler. Probiotics Antimicrob Proteins. 11(3):931–937. doi: 10.1007/s12602-018-9424-9.
- Sikandar A, Zaneb H, Younus M, Masood S, Aslam A, Khattak F, Ashraf S, Yousaf MS, Rehman H. 2017. Effect of sodium butyrate on performance, immune status, microarchitecture of small intestinal mucosa and lymphoid organs in broiler chickens. Asian-Australas J Anim Sci. 30(5):690–699. doi: 10.5713/ajas.16.0824.
- Simpson RJ, Neuberger MR, Liu T-Y. 1976. Complete amino acid analysis of proteins from a single hydrolysate. J Biol Chem. 251(7):1936–1940. doi: 10.1016/S0021-9258(17)33637-2.
- Sitohy M, Osman AJFC. 2010. Antimicrobial activity of native and esterified legume proteins against Gram-negative and Gram-positive bacteria. Food Chem. 120(1):66–73. doi: 10.1016/j.foodchem.2009.09.071.
- Sreerama YN, Sashikala VB, Pratape VM. 2012. Phenolic compounds in cowpea and horse gram flours in comparison to chickpea flour: evaluation of their antioxidant and enzyme inhibitory properties associated with hyperglycemia and hypertension. Food Chem. 133(1):156–162. doi: 10.1016/j.foodchem.2012.01.011.
- Tshovhote N, Nesamvuni A, Raphulu T, Gous R. 2003. The chemical composition, energy and amino acid digestibility of cowpeas used in poultry nutrition. SA J an Sci. 33(1):65–69. doi: 10.4314/sajas.v33i1.3739.
- Wald M, Schwarz K, Rehbein H, Bußmann B, Beermann C. 2016. Detection of antibacterial activity of an enzymatic hydrolysate generated by processing rainbow trout by-products with trout pepsin. Food Chem. 205:221–228. doi: 10.1016/j.foodchem.2016.03.002.
- Xia M, Hu C, Xu Z. 2004. Effects of copper-bearing montmorillonite on growth performance, digestive enzyme activities, and intestinal microflora and morphology of male broilers. Poult Sci. 83(11):1868–1875. doi: 10.1093/ps/83.11.1868.
- Xia Y, Kong J, Zhang G, Zhang X, Seviour R, Kong Y. 2019. Effects of dietary supplementation with lysozyme on the structure and function of the cecal microbiota in broiler chickens. PLoS One. 14(6):e0216748. doi: 10.1371/journal.pone.0216748.
- Yamauchi K-e. 2002. Review on chicken intestinal villus histological alterations related with intestinal function. J Poult Sci. 39(4):229–242. doi: 10.2141/jpsa.39.229.
- Yu D, Rao S, Tsai LM, Lee SK, He Y, Sutcliffe EL, Srivastava M, Linterman M, Zheng L, Simpson N, et al. 2009. The transcriptional repressor Bcl-6 directs T follicular helper cell lineage commitment. Immunity. 31(3):457–468. doi: 10.1016/j.immuni.2009.07.002.
- Zeitz J, Fennhoff J, Kluge H, Stangl G, Eder K. 2015. Effects of dietary fats rich in lauric and myristic acid on performance, intestinal morphology, gut microbes, and meat quality in broilers. Poult Sci. 94(10):2404–2413. doi: 10.3382/ps/pev191.