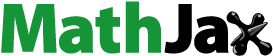
Abstract
The aim of this study was to evaluate the effect of species on added lead (Pb) and cadmium (Cd) content in cow, buffalo, and goat milk and their distribution in fat, casein, and whey fractions. In addition, the oxidative stability of the milk was evaluated. Most of the Pb and Cd were recovered in the skimmed milk (96.74 and 94.21%, respectively). The distribution of Cd and Pb in casein and whey fractions, obtained by enzymatic coagulation, highlighted that they were mainly associated with casein (on average 94.77 and 90.54% of Pb and Cd, respectively). The species significantly affected the distribution of Cd and Pb in the casein and fat fractions (p < 0.01). In particular, Cd and Pb levels in fat fraction were the highest in the buffalo milk, whereas casein fraction was the highest in bovine milk. Furthermore, the presence of metals negatively influenced the oxidative stability of the milk and the species influenced its response. The results showed that in the presence of Cd and Pb, bovine milk increased the content of Malondialdehyde (MDA), advanced oxidation protein products (AOPP) and dithyrosines compared to other species. In addition, there was a significant decrease in the thiol content, highlighting a reduction in the antioxidant capacity of the contaminated milk.
HIGHLIGHTS
Pb and Cd added to milk: species effect.
Greater presence of heavy metals in the casein fraction.
Metals negatively affected the oxidative stability of the milk and the species influenced its response.
Keywords:
Introduction
Heavy metals toxicity is one of the oldest environmental problems and it remains a serious concern for the repercussions on both humans and animals. Cadmium (Cd) and lead (Pb), very toxic heavy metals easily found in the environment (Ali et al. Citation2019) are characterised by a high affinity with biological tissues, from which they are slowly eliminated (Peres et al. Citation1997; EFSA Citation2009). The exposure and ingestion of these metals cause several adverse health effects in humans (Rafiq et al. Citation2016), especially in sensitive individuals such as children and the elderly. Among the factors that influence metals toxicity are the concentration, the exposure routes, the chemical species, but also the genetics, diet and age of the exposed individuals (EFSA Citation2009). These heavy metals are not biodegradable, so they accumulate in food chains through biotransformation, bioaccumulation, and biomagnification (Ali et al. Citation2019). Furthermore, causes an increase in free radicals (ROS, Reacting Oxygen Species) in the cell, both by inducing an increase in mitochondrial production of ROS and by inhibiting the antioxidants production (Ellman Citation1959). Milk contamination could derive directly from the udder of the animal to milk or be favoured by the equipment used for processing and storing the milk (Estévez and Luna Citation2017).
The absorption of these heavy metals is positively favourited by lactose and fat (Nwani et al. Citation2010; Tchounwou et al. Citation2012) without causing particular variations in colour, smell, taste and consolidation, acting as a “silent killer” of toxicity (Manoni et al. Citation2020).
Among these, the species represents an important factor of variation because influences the milk composition (Gantner et al. Citation2015) and the quantitative and qualitative characteristics of proteins, including those of MFGM (Mata et al. Citation1995). However, the distribution of Pb and Cd in milk has been poorly studied; to date, some evidence has been found in human, bovine, and rat milk (Beach and Henning Citation1988; Matović et al. Citation2012; Rubino Citation2015).
Thus, the aim of this study was to evaluate the distribution of added Pb and Cd in cow, buffalo, and goat milk in fat, casein, and whey fractions. In addition, the oxidative stability of the milk compounds was studied to evaluate the damage caused by contamination.
Materials and methods
Samples
Samples of bulk milk from cows (Italian Frisona), buffalo (Italian Buffalo), and mestizo goats were collected in April-May 2022 from farms in the province of Potenza (southern Italy). The bulk milk samples (3 L for each species) were split into three equal batches of 1 L each: in the first was added lead acetate (Pb(CH3COO)2;Merck kGaA, 64271 Dermstadt, Germany) to obtain a final concentration of 1 ppm; in the second was added cadmium chloride (CdCl2; Sigma Aldrich, Milan, Italy) to obtain a final concentration of 1 ppm; the third was milk control (without any addition). The milk samples were stored at a refrigeration temperature (4 °C) for 24 h in glass containers. The physicochemical properties of cow, buffalo and goat milk were determined according to the Official EC method (Ferranti et al. Citation1998). The experiment was repeated three times on different days, obtaining three independent replicates per formulation.
Fractionation of the main components of milk
The fat was recovered from the milk samples of each species by centrifugation at 2,000 g for 20 min at 4 °C, the defatted milk was coagulated with chymosin (Sigma-Aldrich, Milan, Italy), and the casein recovered by centrifugation at 4000 g for 20 min. To remove all serum, the pellet was washed with distilled water and then centrifuged at 4000 g for 20 min. The fat, casein (CN), and whey samples were stored at −20 °C until analysis.
Lead and cadmium determination
Two grams of each samples were put in porcelain crucibles, heated in an oven at 105 °C, and then put in a muffle furnace at 450 °C until burned to ash. Cd and Pb content in fat, casein and whey samples were determined in triplicate by inductively coupled plasma -mass spectrometry (ICP-QMS; Elan DRC II, Perkin-Elmer SCIEX, CT, USA) as reported by Perna et al. Citation2014. The water used for washing the casein was also analysed and the results were added to those of whey. The results were expressed as a percentage distribution of Cd and Pb in the three analysed fractions (fat, casein, and whey).
Determination of lipid oxidation: Tiobarbituric acid reactive substances (TBARS)
Analysis of TBARS was performed as described by Baakdah and Tsopmo (Citation2016) with some modifications. Briefly, 500 µL of milk sample was homogenised with 1 mL of hexane:isopropanol (4:1) by a Polytron (PT-MR 2100, Kinematica AG, Littau, Luzern, Switzerland) at 13,500 x g for 15 s. The homogenates were placed in an ultrasound water bath apparatus (Elma Transsonic 460/H, Singen, Germany) for 10 min at room temperature (RT). An aliquot of each homogenate (145 µL) was transferred into a test tube, and 255 µL of distilled water and 600 µL of 2-thiobarbituric acid (TBA) solution (0.375% thiobarbituric acid, 15% trichloroacetic acid, and 0.25 M HCl) were added. The mixture was kept at 90 °C for 30 min, followed by cooling in a refrigerator (4 °C) for 30 min. Subsequently, the mixture was centrifuged at 3600 × g for 15 min at RT, and the absorbance of the obtained supernatant was measured at 532 nm. TBARS value was calculated from the standard curve draw using 1,1,3,3-tetraethoxypropane (0.3–2 µg mL−1), and the results were expressed as µg malondialdehyde (MDA)/g sample. Each determination was made in triplicate.
Spectrophotometric analysis of advanced oxidation protein products (AOPPs) and dityrosine content
AOPPs and dityrosine in milk samples were determined and measured by spectrophotometric method (UV-VIS Spectrophotometer 1204; Shimadzu, Japan) The AOPPs in milk samples were measured using the method of Witko-Sarsat et al. (Citation1996), using a calibration curve with chloramine-T standard solution (Sigma-Aldrich, Milan, Italy; 0–100 µM). The absorbance was measured at 340 nm and AOPP concentrations were expressed in mM of chloramine-T equivalent. Dityrosines were determined by the spectrophotometric method as described by Witko-Sarsat et al. (Citation1996). The absorbance was measured at 315 nm and dityrosine concentration was determined using the Lambert-Beer formula (ε = 5 mM−1 cm−1 at pH 7.5).
Determination of free thiol groups
Ellman’s (Citation1959) method was used to determine the number of free thiol groups, with some modifications. Two-hundred fifty μL of water-soluble extract of samples were mixed with 2.5 mL of 0.1 M sodium phosphate buffer (containing 1 mM EDTA; pH 8.0, reaction buffer) and 50 μL of DTNB reagent solution (4 mg in 1 ml of sodium phosphate buffer). After the solution was mixed and left to stand at room temperature (25 °C) for 30 min the absorbance was read at 412 nm using the UV-VIS 1204 spectrophotometer (Shimadzu, Kyoto, Japan). Reaction buffer was used instead of the sample as a reagent blank. The molar extinction coefficient of 14,150 M−1 cm−1 was used to calculate the moles of thiol groups. Each determination and measurement was made in triplicate.
Statistical analysis
Statistical analysis was performed by the general linear model (GLM) procedure of SAS (Citation1996) software using:
- a monofactorial model was applied to evaluate the Specie effect on milk quality:
where yi is the experimental observations; μ is the overall mean; αi is the effect of the species (i = cow, buffalo, goat); εi is the residual random error.
Student’s t-test was used for all variable comparisons and differences between means at the 95% (p < 0.05) confidence level were considered statistically significant;
A 2-factor model whit interaction:
where yijk is the dependent variable of the kth observation; μ is the overall mean, ai is the effect of the species (i = cow, buffalo, goat), bj is the effect of the heavy metals (j = Cd, Pb), (a*β)ij is the effect of the interaction between Species and heavy metals; εijk is the residual random error associated with the kth observation.
Before setting the values, expressed in percentage terms, they were subjected to arcsine transformation (Ahrens et al. Citation1990).
Significance of mean differences was determined by Bonferroni at a 5% significance level.
Results and discussion
Characterisation of the chemical-physical composition
In Table the chemical-physical properties of cow, buffalo and goat milk were reported. Statistical analysis showed that the species significantly influenced the quantitative-qualitative characteristics of the milk (p < 0.001). It is known that the chemical composition of milk is species-specific, in particular the percentage of fat, protein, and casein differ for cow, buffalo, and goat milk. The highest fat, protein, and total solids content was recorded in buffalo milk (p < 0.001), in line with what was reported by Niero et al. (Citation2018); whereas, goat milk showed the lowest fat, protein, lactose, and total solids content (p < 0.001). Average fat percentage of goat milk was minor and lactose, protein and casein, ash percentages were almost comparable to the values of Park (Citation2007). The higher content of caseins was observed in buffalo milk (3,26%; p < 0.001) while the lowest was observed in goat milk (2,15%). The pH of each species was between 6.52 and 6.72. In buffalo milk, Bonfatti et al. (Citation2012) found a higher fat content (10.19%) than the present study (6.84%). This difference can be explained by the geographical area and farming conditions which, as is known, represent an important factor in the variation of milk quality. De Vivo et al. (Citation2017) evaluated the influence of the season on the chemical composition of Italian buffalo milk, highlighting a decreasing fat content until April.
Table 1. The pH value and chemical properties (g/100 g) of cow, buffalo and goat milk.
Cadmium and lead content among milk macrocomponents
The Cd and Pb distribution in the milk fractions (fat, casein and whey) is reported in Table . The heavy metals concentration was determined on whole milk and the control milk fractions of each species. The results showed that the content of the metals studied was below the limit of quantification (LOQ < 0.05 for Pb and 0.04 for Cd ng mL−1; Perna et al. Citation2014).
Table 2. Distribution of added Cd and Pb in the milk fractions (fat, casein, and whey), regardless of the analysed species.
Overall, in spiked milk samples, most of Pb and Cd were recovered in the skimmed milk (96.74 and 94.21%, respectively), whereas only 3.26% of Pb and 5.79% of Cd were associated with the lipid fraction. Furthermore, after skimmed milk treatment with rennet the results showed that Pb and Cd were mainly associated with CN (94.77 and 90.54%, respectively, Pb and Cd), whereas only 1.97% of Pb and 3.67% of Cd were associated with whey fraction. These findings were in agreement with Mata et al. (Citation1996) who reported that most of Pb and Cd were associated with CN obtained by coagulation of chymosin (approximately 97 and 89% of Pb and Cd, respectively). CN micelles bind to the metal to form metalloproteins (Srinivas et al. Citation2017; Henry et al. Citation2015) and the interactions between cations and CN are due to the CN type (αs1-, αs2-, β- or κ-CN); the state of CN phosphorylation and their charge; the number of positive charge, the size and hydration of the considered cation; the type of binding; and the physico-chemical environment (Girma et al. Citation2014).
Moreover, αs1-, αs2-, β-, or κ-CN, as a result of the proteolytic action of the rennet, are precursors of peptides containing phosphoseryl and carboxyl sites that bind metals (Ji et al. Citation2019). Therefore, the presence of numerous amino acid residues with ionic and electron-rich side chains, as well as adequate conformations, make the hydrolysed caseins and peptides able to bind divalent metals improving solubility, stability, and bioavailability (Baakdah and Tsopmo Citation2016).
As regards to the association between studied metals and fat fraction (Table ), Cd showed a higher affinity compared to Pb (5.79 and 3.26%, respectively). Pb and Cd content in the fat fraction is due to the high capacity of these metals to bind proteins and lipoproteins of MFGM. In particular, it is known that Cd and Pb bind to the SH groups of proteins affecting their function and, consequently, the activity of antioxidant enzymes that use thiol group as hydrogen donors such as glutathione, glutathione peroxidase, and catalase (Rubino Citation2015). Fransson and Lönnerdal (Citation1983) in human milk observed that significant amounts (15-30%) of Cu, Zn, Ca, and Fe are mainly bound to the outer of MFGM; In particular, these authors suggested that Fe and Zn are associated with xanthine oxidase and alkaline phosphatase, both metal-carrying enzymes. Since Cd is known to interact with Zn, it may be speculated that in the fat fraction the Cd binds to these enzymes; this may explain the higher Cd content compared to Pb detected in this fraction. Although the specific binding of Pb in fat fraction is not known, Henry et al. (Citation2015) highlighted that several MFGM proteins, such as fatty acid synthase, xanthine dehydrogenase/oxidase, butyrophilin, adipophilin, showed a high number of phosphosites; in particular, these authors detected 24 phosphosites foradipophilin, 2 phosphosites forlactadherin, 20 phosphosites forbutyrophilin, 18 phosphosites forxanthine dehydrogenase/oxidase, and 6 for tail-interacting protein of 47 kDa (TIP-47).
The species significantly affected Cd and Pb distribution in the CN and fat fractions (p < 0.001). The Cd and Pb distribution in the milk fractions (fat, casein), distinctly by species, is reported in Figure . In particular, the recovered percentage of Cd and Pb in fat fraction was the highest in the buffalo milk (8,19 and 3,96%, respectively; Table ; p < 0.001), whereas the CN fraction showed the highest Pb percentage (97.65%; p < 0.001) in bovine milk and the highest Cd percentage (92.56%; p < 0.001) in goat milk.
Rafiq et al. (Citation2016), in a comparative study on milk from different species, detected a higher CN content in buffalo milk compared to cow and goat milk which affected the degree of caseins phosphorylation. Sørensen et al. (Citation2003) highlighted that the phosphorylation pattern of CN is highly variable within and among different CN genetic variants of different mammalian species. In fact, the CN phosphorylation occurs by post-translational modification catalysed by kinase enzymes that binded phosphate groups to specific amino acids in the protein sequence that is specific to each species. Ceballos et al. (Citation2009) reported that goat milk contained a higher presence of metal chelating amino acid compared to cow milk. Moreover, it is now known the high affinity between MFGM proteins and heavy metals (Manoni et al. Citation2020). In our study, Cd level in buffalo fat was 1.6 and 2 times higher than in cow and goat fat, respectively. Similarly, Pb level in buffalo fat was 1.5 and 1.3 times higher than in cow and goat fat, respectively. Although major MFGM proteins have been identified in all species, the molecular functions exerted by MFGM proteomes vary due to the variation in protein expression of the species (Jomova and Valko Citation2011; Yang et al. 2020; Manoni et al. Citation2020). Nguyen et al. (Citation2017) showed a significant difference in the type and quantity of proteins in milk fat fractions of bovine and buffalo milk; in particular, these authors detected significant difference in the xanthine oxidoreductase level between the two considered species. Cebo et al. (Citation2010) observed a significant difference between cow and goat species for lactadherin (LDH).
Oxidation induced by added Cd and Pb in milk
The balance between the anti- and pro-oxidative processes in milk and dairy products determines the oxidative stability. Studies showed that one of the main mechanisms of Cd and Pb toxicity is due to the induction of oxidative stress (Matović et al. Citation2012) with the consequent release of oxygen reactive species (ROS), that causes the release of reactive oxygen species (ROS), which negatively affect on the oxidation of both lipids and proteins (Jomova and Valko Citation2011). The oxidative deterioration of milk was made by determining oxidation markers, such as the malondialdehyde (MDA), one of the most known secondary products of lipid peroxidation, the dithyrosines, components of protein oxidation resulted from the interaction of two tyrosine molecules, and the advanced protein products for oxidation (AOPPs) which determine the formation of reactive species with an increase in protein oxidation (Fuentes-Lemus et al. Citation2018). The presence Cd and Pb in milk resulted in a significant increase in TBARS, dithyrosines, and AOPPs value (p < 0.01; Table ). In control milk the TBARS value was 0.198 µg MDA/g milk, whereas Cd- and Pb-milk showed significantly higher values (0.253 and 0.299 µg MDA/g milk, respectively; p < 0.01). Furthermore, the Pb-milk showed MDA levels higher compared to Cd-milk (p < 0.01). In line with our results, Miedico et al. (Citation2016) on animal tissues found significant increase in the MDA level and changes in the FFA composition due to oxidative stress induced by Cd and Pb. As shown in Table , a significant increase (p < 0.01) in both dithyrosines and AOPPs concentration was detected in the contaminated milk compared to the control milk. In particular, compared to the control, the increase percentages in dithyrosines were 27.86% and 32.06% for Cd- and Pb-milk, respectively, whereas the AOPP content was increase of 35.95% and 51.00% in the milk with Cd and Pb, respectively.
Table 3. Oxidative status of control, Cd and Pb-milk.
Table 4. MDA content (ng/g of milk) in control, Cd- and Pb-milk of cow, goat and buffalo.
The protein oxidation is a less studied phenomenon than lipid oxidation, but noteworthy as it is linked to the loss of the nutritional value and organoleptic properties of the food; moreover, it is known that the intake of the resulting catabolites can have deleterious consequences on human health (Estévez and Luna Citation2017; Chirinos-Peinado and Castro-Bedriñana Citation2020). The oxidation of proteins generated by radicals can lead to damage to the protein skeleton or side chains, also the oxidative damage of proteins is irreversible due to a slight unfolding and loss of function (Ceballos et al. Citation2009). The susceptibility of milk to oxidation can also be assessed by measuring the antioxidant capacity. Thiols (biological components) are the most important antioxidants with a sulfhydryl group (-SH) and are characterised by heavy metals chelating properties, well reported in the review of Bjørklund et al. (Citation2019), moreover, they protect cells from any kind of oxidative damage (Ceballos et al. Citation2009; Bjørklund et al. Citation2019). The average content in thiols showed a decrease compared to control milk of 21% and 16% in the Cd- and Pb milk, respectively (Table ). Many metals, including Pb, Cd and Hg have a high affinity for sulfhydryl groups with formation of covalent bonds between these heavy metals and SH gropus that causes the inhibition of function of various biological enzymes such as aminolevulinate dehydratase (ALAD), superoxide dismutase (SOD), catalase (CAT), glutathione peroxidase (GPx) and glucose-6-phosphate dehydrogenase (G6PD) (Jomova and Valko Citation2011; Kasperczyk et al. Citation2012; Nair et al. Citation2013).
Table 5. Content in dithyrosines and advanced protein products for oxidation (AOPPS) of control and contaminated milk, distinctly by species.
Table 6. Content in free thiol groups (µM/L) in control and contaminated milk, distinctly by species.
In this study the statistical analysis showed a significant interaction between contaminant x species (p < 0.001). The MDA content was similar in the control milk of the three considered species (Table ), while the presence of Cd caused an increase of about 37.00% in bovine milk, 25.36% in buffalo milk, and 22.28% in goat’s milk. The similar trend was recorded in presence of Pb where the increase was 65.64% in bovine milk, 47.32% in buffalo milk, and 40.93% in goat’s milk. The differences observed among the milk of different species could be due to the quantity of milk produced daily; in fact, Kapusta et al. (Citation2018) highlighted the negative effect of high milk yield on their milk quality. Rubino (Citation2015) reported that the cows that produce high quantities of milk are more likely to be exposed to free radicals and thus to the free radical peroxidation processes that lead to the production of MDA. Furthermore, the author above cited highlighted higher MDA values in milk with hig-her fat content. In this study bovine milk showed the higher dithyrosines and AOPPs values (p < 0.05), whereas the lower values resulted in goat milk (p < 0.05; Table ). The percentage increase of dithyrosines in Cd-milk was 24.15% in cow’s milk, 22.61% in buffalo milk, and 20.64% in goat’s milk, whereas Pb contamination caused greater damage in all the samples studied, in particular the percentage increase was 30.20% in cow milk, 26.23% in buffalo milk, and 26.04% in goat’s milk. The damage caused by the presence of heavy metals in milk was most evident in the AOPP content where the percentage increase in presence of Cd was 44.77% in cow’s milk, 33.54% in buffalo milk, and 20.64% in goat’s milk, whereas was 54.65%, 56.52%, and 47.20 in Pb milk of cow, buffalo and goat, respectively (Table ). The susceptibility to proteins oxidation with the formation of dithyrosines and AOPPs is influenced by the composition of the milk, i.e. the presence of a higher percentage of fat, as in buffalo milk, could favour the interaction with the protein components and the attack oxidative of proteins. Rafiq et al. (Citation2016) observed a reduction of free amino groups of proteins due to the interaction with lipids. Mestdagh et al. (Citation2011) reported that low-fat dairy products are more sensitive to protein oxidation than those with higher lipid content. Furthermore, a high presence of SH-group, due to the antioxidant activity they perform, guarantees protection to the protein molecules. Cebo et al. (Citation2010) reported that goat milk contains proteins less subject to oxidative modification being included by a higher SH content than cow’s milk. The thiol content was significantly higher in control buffalo milk, followed by goat and cow milk (Table -). Ahmad et al. (Citation2008) reported that buffalo milk has a higher amount of sulfur-containing amino acids, compared to cow’s milk. Thiol groups are particularly vulnerable to attack by ROS and other radicals, undergoing a wide range of oxidative changes. In the present study, Cd and Pb contamination caused a decrease in free thiols in all studied samples, even if the highest damage was highlighted in buffalo milk which recorded the greatest decrease (22 and 21% for Cd and Pb contamination, respectively) compared to bovine and goat milk (20 and 13%% for Cd and Pb contamination, respectively).
Conclusions
In this work, the effect of the species on the distribution and oxidative stability of lead and cadmium-added milk was studied. It is now known that the chemical composition of milk is species-specific, in particular the percentage of fat, protein and casein are different in the milk of cows, buffaloes and goats and, in turn, are also influenced by the geographical area and the breeding conditions. the distribution of Pb and Cd added to bovine, buffalo and goat milk among the milk macrocomponents was evaluated. More than 94% of Pb and Cd added to milk samples was found in skim milk, while the percentage associated with the lipid fraction was very low. Furthermore, in skimmed milk, most Pb and Cd were mainly associated with the casein fraction; this is due to the presence of numerous amino acid residues with ionic and electron-rich side chains, as well as suitable conformations, which make caseins and hydrolysed peptides capable of binding divalent metals. The presence of Pb and Cd in fats is instead due to the high capacity of these metals to bind the SH groups of MFGM proteins and lipoproteins, negatively influencing the antioxidant activity of enzymes localised on MFGM such as xanthine oxidase, catalase, superoxide dismutase. The studied toxic metals negatively affected the oxidative stability of milk, inducing the formation of lipid and protein oxidation compounds. A significant decrease in the thiol content was also found, highlighting a reduction in the antioxidant capacity of the contaminated milk. The species significantly influenced the distribution of Cd and Pb in the fat and casein fractions; this is due both to the different content of caseins and MFGM proteins, and to the level of protein expression and molecular function, which influenced the degree of phosphorylation and the -SH groups of the lactoproteins and therefore the percentage distribution of Cd and Pb. The susceptibility to oxidative stress indirectly due to the presence of Pb and Cd is significantly influenced by the species; this could be due to the variability found in the composition, which translates into a greater or lesser resistance to types of stress.
Ethical approval
The approval of the work by an ethics committee was not required.
Disclosure statement
No potential conflict of interest was reported by the author(s).
Data availability statement
The data that support the findings of this study are available from the corresponding author, upon reasonable request.
References
- Ahmad S, Anjum FM, Huma N, Sameen A, Zahoor T. 2008. Composition and physico-chemical characteristics of buffalo milk with particular emphasis on lipids, proteins, minerals, enzymes and vitamins. J Anim Plant Sci. 23:62–74.
- Ahrens WH, Cox DJ, Budhwar G. 1990. Use of the arcsine and square root transformations for subjectively determined percentage data. Weed Sci. 38(4-5):452–458 doi: 10.1017/S0043174500056824.
- Ali H, Khan E, Ilahi I. 2019. Environmental chemistry and ecotoxicology of hazardous heavy metals: environmental persistence, toxicity, and bioaccumulation. J Chem. 2019:1–14 doi: 10.1155/2019/6730305.
- Baakdah MM, Tsopmo A. 2016. Identification of peptides, metal binding and lipid peroxidation activities of HPLC fractions of hydrolyzed oat bran proteins. J Food Sci Technol. 53(9):3593–3601 doi: 10.1007/s13197-016-2341-6.
- Beach JR, Henning SJ. 1988. The distribution of lead in milk and the fate of milk lead in the gastrointestinal tract of suckling rats. Pediatr Res. 23(1):58–62 doi: 10.1203/00006450-198801000-00013.
- Bjørklund G, Crisponi G, Nurchi VM, Cappai R, Buha Djordjevic A, Aaseth J. 2019. A review on coordination properties of thiol-containing chelating agents towards mercury, cadmium, and lead. Molecules. 24(18):3247. doi: 10.3390/molecules24183247.
- Bonfatti V, Giantin M, Gervaso M, Coletta A, Dacasto M, Carnier P. 2012. Effect of CSN1S1-CSN3 (α(S1)-κ-casein) composite genotype on milk production traits and milk coagulation properties in mediterranean water buffalo. J Dairy Sci. 95(6):3435–3443.
- Ceballos LS, Morales ER, De La Torre Adarve G, Castro JD, Martínez LP, Sampelayo MRS. 2009. Composition of goat and cow milk produced under similar conditions and analysed by identical methodology. J Food Compost Anal. 22(4):322–329 doi: 10.1016/j.jfca.2008.10.020.
- Cebo C, Caillat H, Bouvie F, Martin P. 2010. Major proteins of the goat milk fat globule membrane. J Dairy Sci. 93(3):868–876 doi: 10.3168/jds.2009-2638.
- Chirinos-Peinado DM, Castro-Bedriñana JI. 2020. Lead and cadmium blood levels and transfer to milk in cattle reared in a mining area. Heliyon. 6(3):e03579 doi: 10.1016/j.heliyon.2020.e03579.
- EFSA (European Food Safety Authority), 2009. Technical report of EFSA prepared by the assessment methodology unit on meta-analysis of dose-effect relationship of cadmium for benchmark dose evaluation. In print.
- Ellman GL. 1959. Tissue Sulfhydryl Groups. Arch Biochem Biophys. 82(1):70–77 doi: 10.1016/0003-9861(59)90090-6.
- De Vivo R, Napolano R, Zicarelli L, 2017. Influence of the month on the chemical composition of Mediterranean Italian buffalo milk. J. Buffalo Sci. 6(1):19–27 doi: 10.6000/1927-520X.2017.06.01.3.
- Estévez M, Luna C. 2017. Dietary protein oxidation: a silent threat to human health? Crit Rev Food Sci Nutr. 57(17):3781–3793 doi: 10.1080/10408398.2016.1165182.
- [EC] European Commission 2008. Commission Regulation (EC) No 273/2008 of 5 March 2008 laying down detailed rules for the application of Council Regulation (EC) No 1255/1999 as regards methods for the analysis and quality evaluation of milk and milk products.
- Ferranti P, Scaloni A, Caira S, Chianese L, Malorni A, Addeo F. 1998. The primary structure of water buffalo alpha(s1)- and beta-casein identification of phosphorylation sites and characterization of a novel beta-casein variant. J Protein Chem. 17(8):835–844 doi: 10.1017/s0022029900030983.
- Fransson GB, Lönnerdal B. 1983. Distribution of trace elements and minerals in human and cow’s milk. Pediatr Res. 17(11):912–915 doi: 10.1203/00006450-198311000-00015.
- Fuentes-Lemus E, Silva E, Leinisch F, Dorta E, Lorentzen LG, Davies MJ, López-Alarcón C. 2018. α- and β-casein aggregation induced by riboflavin-sensitized photo-oxidation occurs via di-tyrosine cross-links and is oxygen concentration dependent. Food Chem. 256:119–128 doi: 10.1016/j.foodchem.2018.02.090.
- Gantner V, Mijic P, Baban M, Skrtic Z, Turalija A, 2015. The overall and fat composition of milk of various species. Mljekarstvo. 65(4):223–231 doi: 10.15567/mljekarstvo.2015.0401.
- Girma K, Tilahun Z, Haimanot D. 2014. Review on milk safety with emphasis on its public health. World j Dairy Food Sci. 9(2):166–183 doi: 10.5829/idosi.wjdfs.2014.9.2.85184.
- Henry C, Saadaoui B, Bouvier F, Cebo C. 2015. Phosphoproteomics of the goat milk fat globule membrane: new insights into lipid droplet secretion from the mammary epithelial cell. Proteomics. 15(13):2307–2317 doi: 10.1002/pmic.201400245.
- Ji X, Xu W, Cui J, Ma Y, Zhou S. 2019. Goat and buffalo milk fat globule membranes exhibit better effects at inducing apoptosis and reduction the viability of HT-29 cells. Sci Rep. 9(1):2577 doi: 10.1038/s41598-019-39546-y.
- Jomova K, Valko M. 2011. Advances in metal-induced oxidative stress and human disease. Toxicology. 283(2-3):65–87 doi: 10.1016/j.tox.2011.03.001.
- Kapusta A, Kuczyńska B, Puppel K. 2018. Relationship between parity and oxidative stress in high-performance Polish Holstein-Friesian cows after the peak of lactation. Anim Prod Sci. 57(2):103–110 doi: 10.22630/AAS.2018.57.2.10.
- Kasperczyk A, Prokopowicz A, Dobrakowski M, Pawlas N, Kasperczyk S. 2012. The effect of occupational lead exposure on blood levels of zinc, iron, copper, selenium and related proteins. Biol Trace Elem Res. 150(1-3):49–55 doi: 10.1007/s12011-012-9490-x.
- Manoni M, Di Lorenzo C, Ottoboni M, Tretola M, Pinotti L. 2020. Comparative proteomics of milk fat globule membrane (MFGM) proteome across species and lactation stages and the potentials of MFGM fractions in infant formula preparation. Foods. 9(9):1251doi: 10.3390/foods9091251.
- Mata L, Perez MD, Puyol P, Calvo M. 1995. Distribution of added lead and cadmium in human and bovine milk. J Food Prot. 58(3):305–309. PMID: 31137296doi: 10.4315/0362-028X-58.3.305.
- Mata L, Sanchez L, Puyol P, Calvo M. 1996. Changes in the distribution of cadmium and lead in human and bovine milk induced by heating or freezing. J Food Prot. 59(1):46–50 doi: 10.4315/0362-028x-59.1.46.
- Matović V, Buha A, Bulat Z, Ðukić-Ćosić D, Miljković M, Ivanišević J, Kotur-Stevuljević J. 2012. Route-dependent effects of cadmium/cadmium and magnesium acute treatment on parameters of oxidative stress in rat liver. Food Chem Toxicol. 50(3-4):552–557 doi: 10.1016/j.fct.2011.12.035.
- Mestdagh F, Barbara K, Tatiana C, Meulenaer BD. 2011. Interaction between whey proteins and lipids during light-induced oxidation. Food Chem. 126(3):1190–1197 doi: 10.1016/j.foodchem.2010.11.170.
- Miedico O, Iammarino M, Paglia G, Tarallo M, Mangiacotti M, Chiaravalle AE. 2016. Environmental monitoring of the area surrounding oil wells in Val d’Agri (Italy): element accumulation in bovine and ovine organs. Environ Monit Assess. 188(6):338doi: 10.1007/s10661-016-5317-0.
- Nair AR, Degheselle O, Smeets K, Van Kerkhove E, Cuypers A. 2013. Cadmium-induced pathologies: where is the oxidative balance lost (or not)? Int J Mol Sci. 14(3):6116–6143 doi: 10.3390/ijms14036116.
- Nguyen HTH, Ong L, Hoque A, Kentish SE, Williamson N, Ang CS, Gras SL. 2017. A proteomic characterization shows differences in the milk fat globule membrane of buffalo and bovine milk. Food Biosci. 19:7–16 doi: 10.1016/j.fbio.2017.05.004.
- Niero G, Currò S, Costa A, Penasa M, Cassandro M, Boselli C, Giangolini G, De Marchi M. 2018. Phenotypic characterization of total antioxidant activity of buffalo. goat and sheep milk. J Dairy Sci. 101(6):4864–4868 doi: 10.3168/jds.2017-13792.
- Nordberg GF, Nogawa K, Nordberg M. 2015. Cadmium. Handbook on the Toxicology of Metals vol. II: specific Metals. Elsevier, Amsterdam. NL, p. 667–716 doi: 10.1016/B978-0-12-369413-3.X5052-6.
- Nwani CD, Nwachi DA, Okogwu OI, Ude EF, Odo GE. 2010. Heavy metals in fish species from lotic freshwater ecosystem at Afikpo. Nigeria. J Environ. 31(5):595–601.
- Park YW. 2007. Impact of goat milk and milk products on human nutrition. CAB Reviews: Perspectives in Agriculture, Veterinary Science, Nutrition and Natural Resources. 8(81). CAB Online Journal. ISSN 1749–8848.
- Peres JM, Bouhallab S, Bureau F, Maubois JL, Arhan P, Bougle ÂD. 1997. Absorption digestive du fer lié au caséinophosphopeptide 1-25 de la β-caséine. Lait. 77(4):433–440 doi: 10.1016/j.foodchem.2012.10.113.
- Perna A, Intaglietta I, Simonetti A, Gambacorta E. 2014. Metals in honeys from different areas of Southern Italy. Bull Environ Contam Toxicol. 92(3):253–258 doi: 10.1007/s00128-013-1177-2.
- Qixiao Z, Arjan N, Wei C. 2015. Dietary strategies for the treatment of cadmium and lead toxicity. Nutrient. 7(1):552–571 doi: 10.3390/nu7010552.
- Rafiq S, Huma N, Pasha I, Sameen A, Mukhtar O, Khan MI. 2016. Chemical composition, nitrogen fractions and amino acids profile of milk from different animal species. Asian-Australas J Anim Sci. 29(7):1022–1028 doi: 10.5713/ajas.15.0452.
- Rubino FM. 2015. Toxicity of glutathione-binding metals: a review of targets and mechanisms. Toxics. 3(1):20–62 doi: 10.3390/toxics3010020.
- Sas I. 1996. SAS User’s Guide: statistics (Version 7th ed.) Cary, NC: SAS Institute Inc.
- Sørensen ES, Møller L, Vinther M, Petersen TE, Rasmussen LK. 2003. The phosphorylation pattern of human αs1-casein is markedly different from the ruminant species. Eur J Biochem. 270(17):3651–3655 doi: 10.1046/J.1432-1033.2003.03755.X.
- Srinivas S, Purnima K, Prakash V. 2007. Mechanism of interaction of Pb(II) with milk proteins: a case study of α-casein. J Agric Food Chem. 55(22):9283–9288 doi: 10.1021/jf070911t.s001.
- Tchounwou PB, Yedjou CG, Patlolla AK, Sutton DJ. 2012. Heavy metal toxicity and the environment. Exp Suppl. 101:133–164 doi: 10.1007/978-3-7643-8340-4_6.
- Witko-Sarsat V, Friedlander M, Capeillère-Blandin C, Nguyen-Khoa T, Nguyen AT, Zingraff J, Jungers P, Descamps-Latscha B. 1996. Advanced oxidation protein products as a novel marker of oxidative stress in uremia. Kidney Int. 49(5):1304–1313 doi: 10.1038/ki.1996.186.
- Yang M, Deng W, Cao X, Wang L, Yu N, Zheng Y, Wu J, Wu R, Yue X. 2020. Quantitative phosphoproteomics of milk fat globule membrane in human colostrum and mature milk: new insights into changes inprotein phosphorylation during lactation. J Agric Food Chem. 68(15):4546–4556 doi: 10.1021/acs.jafc.9b06850.
- Yang Y, Zheng N, Zhao XW, Zhang YD, Han RW, Ma L, Zhao S, Li S, Guo T, Wang J. 2015. Proteomic characterization and comparison of mammalian milk fat globule proteomes by iTRAQ analysis. J Proteomics. 116:34–43 doi: 10.1016/j.dib.2014.12.004.