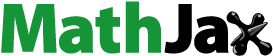
Abstract
Organic wastes from vegetable mix (V) and butchers (B) were used as substrates (V100%, V75%+B 25%, and V50%+B50%) for Hermetia illucens to assess the mineral profile of the larvae and frass, and the potential bioaccumulation of toxic elements (As, Cd, Pb, Hg). The V100 larvae had the highest concentrations of all minerals, except for Se and P, higher in the control group (poultry diet as substrate) (p < .01). The V50B50 and V75B25 larvae showed lower (p < .01) mineral concentrations than the other groups. The V50B50 group had the highest (p < .01) bioaccumulation factor (BAF) for Cd (8.52%), the V100 for Pb (p < .01). The equations to estimate the mineral content of larvae from the substrate were significant only for Ca and Mg (p < .05). The V100 frass had the highest (p < .01) levels of As, Cd, Pb, Fe, K and Mg, while the control group showed the highest (p < .01) levels of Se, Zn, Ca, P and K. Toxic elements can accumulate in black soldier fly larvae and frass, with the exception of Hg. However, organic wastes from V and B had a very low content of toxic elements and that strongly reduced the risk of excessive bioaccumulation in larvae. Indeed, the content of toxic elements in larvae is lower than the maximum values established for feed materials by EU regulations. In conclusion, organic substrates from vegetables or butchery wastes can be used for BSF larvae growth without the risk of accumulation of toxic elements.
HIGHLIGHTS
Insect production for food and feed are increasing worldwide
A well assessment of insect product safety is required by European Union
Toxic elements can give bioaccumulation problems in black soldier fly larvae and frass but the risk depends on their level in the growing substrate
Introduction
Over the last decade, interest in insect production as a way to address the shortage of protein resources for humans and animals has increased, due to the expected future increase in the world’s population (FAO Citation2021; Secci et al. Citation2022). In addition, recent global crisis (COVID-19 pandemic, Russian-Ukrainian war) have drawn attention to the potential difficulties obtaining raw materials and energy, and increased the overall cost of human food and animal feed (Attia et al. Citation2022). Particular attention has been directed towards some bio-converting insects, in particular the black soldier fly (BSF, Hermetia illucens, L.), which is able to rapidly convert a wide range of organic wastes into a biomass (larvae) rich in both proteins and lipids (Scieuzo et al. Citation2023), as well as in bioactive compounds such as chitin, lauric acid and antibacterial peptides which might positively influence the intestinal morphometry and health of animals (Saviane et al. Citation2021; Candian et al. Citation2023). After harvesting of these larvae, the residual frass (containing insect exuviae and excrements, as well as residual substrate) could be used as a fertiliser, or to produce biogas or biofuel (Carroll et al. Citation2023; Wedwitschka et al. Citation2023; Mohan et al. Citation2023).
In the literature, growth performance (Marono et al. Citation2017), chemical-nutritional characteristics (Marono et al. Citation2015), the potential use of insect products as a source of bioactive compounds (Borrelli et al. Citation2017, Citation2021), and the possible applications of insect frass (Carroll et al. Citation2023; Wedwitschka et al. Citation2023) have been investigated. However, there are very few research investigating the mineral content of insect larvae and frass in relation to the abundance of the corresponding minerals in the growth substrate (Proc et al. Citation2020) or in relation to the type of substrate. Today, when the introduction of insects into the food chain is being considered, it is vital to determine all the possible risks associated with their consumption, in particular when different growth substrates were used. In fact, in order to support the impetus to develop a circular economy, several researchers have focused their attention on the use of organic wastes for H. illucens larvae growth. The Regulation (EC) No 767/2009 prohibits the use of ‘unsafe’ rearing substrates such as manure, separate digestive tract contents, catering wastes and processed animal proteins (very often the substrates preferred by BSF adults for oviposition, according to Boafo et al. Citation2023) to reduce microbiological hazards, including prions. However, additional possible hazards have thus far been underestimated. Organic wastes often contain persistent pollutants such as heavy metals, which could accumulate in the larvae and frass entering the food chain and the environment. Heavy metals enter the waste stream in a variety of ways, whether through atmospheric emissions or improper disposal of waste containing heavy metal. The result is that terrestrial organisms may then ingest these contaminants resulting in greater environmental hazard (Diener et al. Citation2015).
Starting from the hypothesis that, in general, minerals can accumulate in BSF larvae in function of the correspondent amount in the substrate, the first aim of this study was to explore the possible relationship among mineral concentrations in larvae and frass and the associated substrate, none of which have been thoroughly examined previously. A further aim was to calculate the potential bio-accumulation of toxic elements in the larvae to identify and assess possible risks to animal and human health. For this purpose we tested substrates including wastes from vegetable and butchery markets, which are readily available throughout the year.
Material and methods
Substrates
Four substrates were used: broiler feed, as a control diet; a vegetable diet (V100); a diet comprising of 75% of vegetable diet + 25% of butchery wastes (V75B25); and a diet consisting of 50% of vegetable diet + 50% of butchery wastes (V50B50). The vegetable diet consisted of a mix of vegetable wastes containing 75% vegetables (broccoli 40%, celery 35%, cabbages 25%) and 25% fruits (50% oranges and 50% apples). The butchery wastes were obtained from butchers in the province of Napoli and mainly consisted of fat and meat resulting from the trimming of bovine carcases and cuts of meat. The collected vegetable wastes were stored for two days at room temperature to reduce the water content, while the butchery wastes were enclosed in dark bags and stored at ∼2 °C. Prior to use, the butchery waste was left at room temperature for approximately 2 h. The vegetables and butchery wastes were also cut into small pieces and mixed to prepare the larvae substrate as described above. A batch feeding strategy was applied, meaning the substrates were placed in plastic containers (60 cm × 40 cm × 15 cm) one day prior to placing the larvae. This allowed the substrates to heat up before the start of the experiment. The scheduled feeding rate (amount of substrate/animal/day) was 0.075 g of DM/larva/day.
Larvae and experimental groups
Five-day-old black soldier fly larvae (Hermetia illucens) were purchased from the commercial insect rearing company Smart Bug’s (Treviso, Italy) in February 2020 and used in a growing trial. Up to 5 days of age the larvae were fed a standard Gainseville diet. Each group (Control, V100, V50B50, and V75B25) consisted of 9 replicates, each placed in a plastic tray, resulting in a total of 36 trays. In each replicate, 10 kg of substrate was placed. On the top of each substrate, 6000 larvae were transferred after weighing. The trays were covered with a perforated cap with a black nylon grid and then placed in a ventilated chamber (air flow around 2 m/s) under controlled environmental conditions (T: 27 ± 0.5 °C; RH: 70 ± 5%; L:D photoperiod: 16:8). More details concerning the experimental design are reported by Addeo et al. (Citation2021). All the groups reached the end of the experiment (25% of prepupae) after 17 d, when the larvae were 22 d old.
At the end of the growing period, the larvae were separated from the frass and fasted for 24 h. Samples of larvae and frass were then collected from each replicate, freeze-dried and stored at room temperature until analysis.
Chemical analysis
The samples of the substrates (n = 4), larvae (n = 9 per group) and frass (n = 9 per group), were analysed to determine their chemical composition in triplicate, according to the AOAC (Citation2005) standard methods. For the larvae only, the calculation of the protein content was performed using with anitrogen-to-protein conversion ratio of 4.76, as indicated by Janssen et al. (Citation2017). The samples of butchery waste were also analysed to determine water, protein and lipid contents.
Mineral profiles
For the mineral profile, before ICP-OES analysis, the sample (0.5 g ± 0.02) was placed in a Teflon vessel with 7.0 ml of 65% HNO3 and 3.0 mL of 30% H2O2 (Romil UpA). The vessel was sealed and placed in a microwave assisted digestion system (Milestone, Bergamo, Italy). Microwave assisted digestion was performed using a mineralisation program for 50 min at 200 °C. The vessel was then cooled to 32 °C, and the digestion mixture was transferred to a 50.0 mL flask and the final volume was obtained by adding Milli-Q water (Ariano et al. Citation2019). Subsequently, the mineralised samples were subjected to a filtration process using 45 µm SY25TF (PTFE) filters.
CETAC U5000AT (ThermoFisher Scientific, Waltham, MA, USA) and Perkin Elmer Optima 2100 DV (Perkin Elmer, Inc., Wellesley, MA, USA) instruments were used to detect the concentrations of trace elements (ICP-OES technique), while the purity of the chemicals was assessed by running two blanks and the calibration curve during each set of analyses. For quality control purposes, reference materials (BCR-422 cod muscle, IRMM Institute for Reference Materials and Measurements, DORM-2 fish protein, National Research Council, Canada), with values resulted all within certified limits, were included.
The Perkin Elmer ICP application study number 57's protocol (Barnard et al. Citation1993) was followed to assess instrumental detection limits, which were then expressed in wet weight.
Participation in interlaboratory studies, as organised by the Food Analysis Performance Assessment Scheme (Sand Hutton, UK) allowed the method’s performance assessment.
Minerals bioaccumulation and rate of absorption
It is widely acknowledged that ‘bioaccumulation’ describes the accumulation and enrichment of contaminants in organisms, relative to those in the environment. Then, the bioaccumulation factor (BAF) for the toxic elements (As, Pb, Cd and Hg) in the studied larvae was calculated according to a dry mass basis using element concentrations determined by ICP analysis and defined according to the equation proposed by Walker (Citation1990) as follows:
Statistical analysis
Data were subjected to a one-way analysis of variance, using the procedure GLM of SAS (Citation2002), according to the following model: Yij = m + Si + eij, where Y is a single observation, m is the general mean and e is the error. The experimental unit was the replicate. To assess the differences among the means, Tukey’s test was used (SAS Citation2002). The relationship among the mineral content of the larvae and frass and the substrate was studied using the PROC REG of SAS (Citation2002), by comparing the average value of the 9 replicates for larvae and frass with the corresponding content in the substrate (n = 4). p values lower than .05 are considered significant, while p values ranging between .05 and 1 are considered as a tendency.
Results
The water contents of the vegetable and butchery wastes were 85.37 ± 1.12% and 38.92 ± 2.85%, respectively. The protein and lipid contents of the butchery waste on a dry matter basis were 7.12 ± 0.65 and 42.23 ± 1.33, respectively.
The V100 substrate (Table ) had the highest concentrations of heavy metals (As, Cd, Pb), and it was the only substrate containing traces of Hg. The V100 substrate was also the richest in Fe and P. The substrates including butchery wastes showed the lowest Mg values.
Table 1. Chemical characteristics and mineral profile of the 4 substrates used for black soldier fly larvae growing.
The mineral profile of the BSF larvae (Table ) showed that animals on the V100 had the highest concentrations of all the minerals studied, except for Se and P, which had the highest levels in the larvae from the control group (p < .01). In general, groups V50B50 and V75B25 showed lower (p < .01) mineral concentrations than the other groups.
Table 2. Toxic, micro and macro elements of black soldier fly larvae raised on the 4 different substrates.
The bio-accumulation factors for the toxic elements in BSF larvae as affected by the 4 tested substrates are reported in Table . Excluding Hg, the highest BAF was obtained for Cd in the V50B50 group (8.52), and the lowest (p < .01) for As in the V75B25 group (0.92). The V100 group had the highest BAF for Pb, followed by both the control group and the V50B50 and V75B25 groups combined (p < .01).
Table 3. Bioaccumulation factor for toxic elements in black soldier fly larvae raised on the 4 tested substrates.
Of the heavy metals, As, Cd, and Pb showed a positive correlation with the respective content in the substrate, as did Fe, Ca, P and Mg while for Se, Zn and K the relationship with the substrate was negative (Table ). However, the equation regression was significant only for Ca and Mg (p < .05) and the amount of the minerals in the substrate can explain the 97.83% and the 93.71% of the mineral content in the larvae, respectively.
Table 4. Equations to estimate mineral concentration in black soldier fly larvae (Y) from that of substrates (X).
In general, the frass obtained from V100 had the highest (p < .01) levels of the heavy metal elements (As, Cd, Pb) and of Fe, K and Mg, while the control group showed the highest (p < .01) levels of Se, Zn, Ca, P and K (Table ).
Table 5. Toxic, micro and macro elements measured in residual frass obtained at the end of growing period of black soldier fly larvae on the 4 tested substrates.
Table shows the equations to estimate the content of a specific mineral in the frass, from the corresponding levels in the larvae and substrate. The estimations reached the significance (p < .05) only for Cd and Pb. However, the r2 values were higher than 98% for almost all the heavy metals studied, with the exception of the Zn.
Table 6. Equations to estimate mineral content of residual frass (Y) fom that of substrate (X) and black soldier fly larvae (Z).
Table shows the maximum levels set by the European Commission (Citation2002) for As, Cd, Pb and Hg in feed, and compared them to the medium and maximum levels detected in our larvae samples. Compared to the maximum levels for heavy metals set by the EU Commission for feed materials, our samples were always resulted lower than the maximum values.
Table 7. Comparison of the heavy metal levels of our samples with the maximum limits imposed by the European Commission in feed.
Discussion
Black soldier fly larvae and frass are of considerable interest to the industry as the larvae can be used as either animal feed or food, and the frass to amendment for soil. In both contexts, the study of minerals accumulation from the growing substrate is a pivotal point. Considering macro and micro-minerals, it is important to determine if the insect products used for humans, animals or plants can be able to satisfy the mineral requirements. In addition, heavy metals are among the main contaminants of our food supply, and represent a serious issue for the ecosystem (Korish and Attia Citation2020; Mehar et al. Citation2023). Chronic exposure to toxic elements in human and animals can cause nephron, neuro, hepato, skin, and cardiovascular toxicity (Mitra et al. Citation2022). In addition, high levels of toxic elements in residual frass, used as plant amendment, can contribute to introduce these elements into the food chain, increasing the risk of chronic exposure for humans, and also having a direct effect on plants. In fact, heavy metal toxicity is one of the principal abiotic stressors on plants, affecting their growth and metabolism (Saxena and Shekhawat Citation2013).
The present research provides a unique contribution to the field, assessing the relationship among mineral concentrations in BSF larvae and frass and the associated substrate, which has not been thoroughly examined previously, to the best of our knowledge. Our research confirmed that the mineral profile of black soldier fly larvae is affected by the growing substrate, despite other factors also being involved in determining the bio-accumulation factor of the toxic elements.
Of the minerals most studied in insects, the toxic elements (Cd, As, Pb, Hg) play a pivotal role. In our trial, Cd showed the highest BAF, with an average value of 6.06, followed by Pb (2.51) and As (1.93). The Hg was not detected in any of the substrates used in the present trial and, when measured (as in V100 substrate), the BAF value in the corresponding larvae was less than 1, indicating no hazards were associated with possible accumulation, probably due to the very low levels contained in the V100 substrate. These results are in partial agreement with Proc et al. (Citation2020) who found higher BAF values in BSF larvae for Cd than for Hg, despite the same authors founding no bio-accumulation for Pb and As. It has been reported that Cd has high toxicity for humans and animals, with long-term oral exposure having negative effects on several organs (kidney, liver, lung, bone, immune system, blood, and nervous system) and inducing the development of cancerous growths (Huff et al. Citation2007). Interestingly, the Cd levels found in our trial are lower than those detected in other studies examining edible insects (from 110 to 190 μg/kg in the trials by Truzzi et al. Citation2019; Bessa et al. Citation2021): in the present trial the Cd concentration in the larvae ranged from 2.98 to 7.20 μg/kg. This is probably associated with the very low level of this toxic element in the growing substrates. However, the BAFs calculated for Cd (ranging from 2.03 to 8.52) did align with those noted in other studies (Truzzi et al. Citation2019; Van der Fels-Klerx et al. Citation2020), confirming that Cd has a high level of bio-accumulation in BSF larvae and, in general, its accumulation showed a positive relationship with the level of Cd in the growing substrate (X coefficient +0.5422, r2 = 0.6349). In the case of some insects, like H. illucens, Cd can be absorbed by cells through Ca2+ channels due to its similarity to Ca2+ (Braeckman et al. Citation1999). In addition, the HSP70 protein family can be synthesised in dipterous cells as a consequence of the elevated Cd content. This protects other proteins from Cd interaction, and these mechanisms may explain the elevated Cd concentration in BSF larvae (Hu et al. Citation2023).
In disagreement with Proc et al. (Citation2020), the bio-accumulation of lead occurred in all the tested groups, without a significant relationship between lead levels in the substrate and the larvae. This result can be attributed to the physiological metabolism of this element in some insects: in fact, Pb accumulates in the exoskeleton of the BSF larvae (Wang et al. Citation2018); so that the moulting could be considered a strategy to reduce the Pb concentration (Hu et al. Citation2023) in the insect body, as, unlike Cd, there are no active channels for Pb absorption. In our trial, the levels of lead detected in the BSF larvae were positively related to its content in the substrate with a p value tending towards significance (r2 = 0.8906, p = 0.0515). In addition, the levels of lead in the residual frass were positively related to lead content in both the substrate and the larvae with a high determination coefficient (r2 = 0.9937, p = 0.0490). Very interesting, even if V50B50 and V75B25 groups had different concentrations of lead in their growing substrates, the BAF coefficient was not different between the two groups. Looking at the growth performance of the larvae, as reported in previous research by Addeo et al. (Citation2021), at the end of the trial, the larvae from the V75B25 group had a higher live weight than the V50B50 group (0.2163 vs 0.2047 g, p < .01). Thus, our hypothesis is that the high growing rate of V75B25 group was responsible for the more effective elimination of the lead from the insect body. This hypothesis is further confirmed by the Pb levels in frass which are higher in the V75B25 than in V50B50 group.
The bio-accumulation of As occurred in almost all the groups with the exception of V75B25, the concentration of which in the larvae reflected that in the substrate (r2 = 0.8626, p = .0711), according to Biancarosa et al. (Citation2018). However, the bio-accumulation of the arsenic in the BSF larvae was lower than that of other toxic elements. Lievens et al. (Citation2021) reported that arsenic concentrations in BSF larvae decreased by starving, indicating that the measured As level could be linked to its presence in the gut contents and had not accumulated in the organism. This assertion suggests that probably As is unlikely to constitute a chemical hazard if the larvae are starved (Van Der Fels-Klerx et al. Citation2016). However, our results conflict with this finding as the larvae were starved at the end of the trial and a bio-accumulation of As occurred. This indicates that further study of As accumulation in BSF larvae is required.
In insects, Iron can be considered both an essential nutrient and a strong toxin; it can be stored in the insect body linked to the ferritin protein and is responsible for the larvae’s colour (Janssen et al. Citation2019). Nairuti et al. (Citation2022) observed that BSF larvae can accumulate high levels of Iron, relative to the level of this element in the growing substrate. However, considering our results suggest it is not only the levels of Fe in the substrate that regulate the concentration of Fe in BSF larvae, rather it is the form of Iron in the substrate that is crucial, as BSF larvae can only utilised some forms of Iron. In vegetables, Iron is in a non-haem form, and in general ferric ions that must be reduced by some mechanism in the mid-gut lumen of insects prior to uptake; in contrast, in substrates of animal origin Fe is in a haem form (Gorman Citation2023). In our trial, V100 larvae had the highest concentrations of Fe, however, the levels of iron in the frass obtained from V100 groups were more than double compared to that of other. The V75B25 group showed the second highest content of Iron, with its accumulation in the frass being 3.64 times greater than that in the larvae, suggesting different sources of the substrate can affect the utilisation of this element, even if, as indicated by Bonelli et al. (Citation2020) a diet-dependent adaptation processes in the BSF mid-gut can ensure the exploitation of the substrates, in particular when there is a deficiency of a particular nutrient. In our trial, no significant relationship was observed between the growing substrate and larvae or among frass, larvae and substrates for iron.
Few studies are available in the literature that consider the concentration of Se in BSF larvae and frass. Selenium is an essential element, for both animals and humans (Attia et al. Citation2010; Adadi et al. Citation2019), as it is involved in the functioning of several enzyme systems (Constantinescu-Aruxandei et al. Citation2018). Ferrari et al. (Citation2022), evaluated the possibility of producing BSF larvae fortified with Se, and observed that its accumulation in larvae was linked to the form of Se (organic or inorganic) in the substrate. They concluded that a diet enriched with inorganic selenite caused an increased Se levels in the BSF prepupae. Further investigations are mandatory to establish whether our results may be affected by the different form of Se in the different substrates.
Zn showed high concentrations in the BSF larvae. However, its levels in the substrates showed a trend (V75B25 > control > V100 > V50B50) that was completely different from that observed in larvae (V100 > control > V50B50 > V75B25) and frass (control > V100 > V75B25 > V50B50). This result agrees with the finding of Diener et al. (Citation2015) which suggested active regulation of Zn absorption in the insect body. This element (Zn) is a mineral essential for insect metabolism, so when concentrations of this heavy metal in the substrate are low, the BSF larvae, similar to other insects, are able to increase their uptake from the substrate (Bohm et al. Citation2023). Looking at the estimation equation for Zn from substrate to larvae, even if the significance for the R2 value is not reached, the estimated coefficient for the larvae value is negative, reinforcing the negative relationship between substrate Zn and larvae Zn.
According to Proc et al. (Citation2020) the concentration of all the minerals in the residual frass is higher than in the corresponding substrate and, in some cases, higher than that recorded in the larvae at the end of the growing period. The frass, which is the residue at the end of the larval growth period consists of residues of unused substrate, larvae faeces and exuviae. Therefore, we can observe three active mechanisms that regulate the amount of a single mineral in the frass: (1) the ‘concentration’ of a specific mineral due to the reduction of water and other nutrients; (2) the reduction of the mineral due to the accumulation in the insect body; (3) the increase of a specific mineral due to its elimination through insect excrements and exuviae. The balance among these three factors can explain the different behaviours of a specific mineral considering its concentration in feed, larvae and frass. With regard to the BSF larvae, there are contradictory results in the literature. For example, Romano et al. (Citation2022) observed that when feeding BSF larvae on orange, banana and combinations of these, Ca was higher in the frass than in the BSF larvae due to the presence of the accumulated exuviae in the residual material. Conversely, Proc et al. (Citation2020) observed higher contents of Ca in larvae than in frass when grown on fish feed. In our trial, Ca levels in the BFL larvae were positively and significantly (r2 = 0.9783, p = .0110) related to the growing substrate, while no significant relationship was noted among Ca levels in frass, larvae and substrate; even if in the estimation equation, the coefficient for the substrate was negative. Similar to Pb, the Ca is accumulated in the insect exoskeleton but the role of Ca differed completely from the toxic element. Paul et al. (Citation2017) observed that an increase in the Ca concentration in the substrate was directly linked to increased Ca accumulation in the BSF larvae due to its future role in pupation.
In the case of Se and P, very few studies have focused on concentrations of these elements in BSF larvae. In our trial, the V100 substrate showed the highest level of P, while in both the larvae and the frass the highest concentration of this element was detected in the control group. This result can be explained by the different form of P in the substrates. In fact, in vegetables, the phosphorous has an inorganic form, which is in general linked to phytic acid and has low bioavailability for animals. The control group was also grown on a vegetable substrate (poultry feed), but it is normally supplemented with minerals, and in particular P to guarantee a high bioavailability for animals. In general, the amount of P in the frass is lower than that in the larvae and this is very interesting considering the possible applications of frass as a soil amendment. The relationship between P in the growing substrate and larvae is positive and tends to the significant (r2 = 08164, p = .0901).
According to the findings of other authors (Romano et al. Citation2022), the BSF larvae showed a very poor concentrations of K, which is confirmed as one of the few minerals whereby the concentration in frass is generally lower than in larvae.
The concentration of Mg in the BSF larvae showed a positive relationship with its content in the substrate (r2 = 0.9371, p = .032), suggesting it is possible to modulate the Mg content in BSF larvae and explaining the differences in Mg levels in different trials on BSF larvae.
Conclusions
The current study showed that toxic elements can be accumulated in black soldier fly larvae and frass, even if their concentrations are present in very small amount in the substrates, with the exception of Hg. The relationship between element contents in the larvae and the substrate was significant for only Ca and Mg and tended to a significance for As, Pb and P; this point needs further deep investigation by increasing the number of tested substrates. The same consideration can be applied to the relationship among frass, larvae and substrate which was significant only for Cd and Pb. However, the hazards in the use of organic wastes obtained from products intended for human consumption is very low due to the very low contents of toxic elements in these substrates, in compliance with EU regulations. Although further studies on a greater number of samples are needed for the assessment of heavy metals and other pollutants on the environment bio-accumulation. Concerning Pb, Cd, Hg and As concentrations, these preliminary results support the possibility to use these analysed samples for animal feed with no additional hazards.
Ethical statement
Ethical approval is not required by national laws.
Acknowledgments
This study was carried out within the Agritech National Research Center and received funding from the European Union Next-Generation EU (PIANO NAZIONALE DI RIPRESA E RESILIENZA (PNRR) – MISSIONE 4 COMPONENTE 2, INVESTIMENTO 1.4 – D.D. 1032 17/06/2022, CN00000022). This manuscript reflects only the authors’ views and opinions, neither the European Union nor the European Commission can be considered responsible for them.
Disclosure statement
No potential conflict of interest was reported by the author(s).
Data availability statement
The data that support the findings of this study are made available by the authors upon request.
References
- Adadi P, Barakova NV, Muravyov KY, Krivoshapkina EF. 2019. Designing selenium functional foods and beverages: a review. Food Res Int. 120:708–725. doi: 10.1016/j.foodres.2018.11.029.
- Addeo NF, Vozzo S, Secci G, Mastellone V, Piccolo G, Lombardi P, Parisi G, Asiry KA, Attia YA, Bovera F. 2021. Different combinations of butchery and vegetable wastes on growth performance, chemical-nutritional characteristics and oxidative status of black soldier fly growing larvae. Animals. 11(12):3515. doi: 10.3390/ani11123515.
- Ariano A, Marrone R, Andreini R, Smaldone G, Velotto S, Montagnaro S, Anastasio A, Severino L. 2019. Metal concentration in muscle and digestive gland of common octopus (Octopus vulgaris) from two coastal site in Southern Tyrrhenian sea (Italy). Molecules. 24(13):2401. doi: 10.3390/molecules24132401.
- Attia YA, Rahman MT, Hossain MJ, Basiouni S, Khafaga AF, Shehata AA, Hafez HM. 2022. Poultry production and sustainability in developing countries under the COVID-19 crisis: lessons learned. Animals. 12(5):644. doi: 10.3390/ani12050644.
- Attia YA, Abdalah AA, Zeweil HS, Bovera F, Tag El-Din AA, Araft MA. 2010. Effect of inorganic or organic selenium supplementation on productive performance, egg quality and some physiological traits of dual purpose breeding hens. Czech J Anim Sci. 55(1):505–519. doi: 10.17221/1702-CJAS.
- AOAC. 2005. Official methods of analysis. 18th ed. Arlington (VA): Association of Official Analytical Chemists.
- Barnard TW, Crockett MI, Ivaldi JC, Lundberg PL. 1993. Design and evaluation of an echelle grating optical system for ICP-OES. Anal Chem. 65(9):1225–1230. doi: 10.1021/ac00057a020.
- Bessa LW, Pieterse E, Marais J, Dhanani K, Hoffman LC. 2021. Food safety of consuming black soldier fly (Hermetia illucens) larvae: microbial, heavy metal and cross-reactive allergen risks. Foods. 10(8):1934. doi: 10.3390/foods10081934.
- Biancarosa I, Liland NS, Biemans D, Araujo P, Bruckner CG, Waagbø R, Torstensen BE, Lock EJ, Amlund H. 2018. Uptake of heavy metals and arsenic in black soldier fly (Hermetia illucens) larvae grown on seaweed-enriched media. J Sci Food Agric. 98(6):2176–2183. doi: 10.1002/jsfa.8702.
- Boafo HA, Gbemavo DSJC, Timpong-Jones EC, Eziah V, Billah M, Chia SY, Aidoo OF, Clottey VA, Kenis M. 2023. Substrates most preferred for black soldier fly Hermetia illucens (L.) oviposition are not the most suitable for their larval development. JIFF. 9(2):183–192. doi: 10.3920/JIFF2022.0034.
- Bohm M, Anker S, Mahfoud F, Lauder L, Filippatos G, Ferreira JP, Pocock SJ, Brueckmann M, Saloustros I, Schler E, et al. 2023. Empagliflozin, irrespective of blood pressure, improves outcomes in heart failure with preserved ejection fraction: the EMPEROR-preserved trial. Eur Heart J. 44(5):396–407. doi: 10.1093/eurheartj/ehac693.
- Bonelli M, Bruno D, Brilli M, Gianfranceschi N, Tian L, Tettamanti G, Caccia S, Casartelli M. 2020. Black soldier fly larvae adapt to different food substrates through morphological and functional responses of the midgut. Int J Mol Sci. 21(14):4955. doi: 10.3390/ijms21144955.
- Borrelli L, Coretti L, Dipineto L, Bovera F, Menna F, Chiariotti L, Nizza A, Lembo F, Fioretti A. 2017. Insect-based diet, a promising nutritional source, modulates gut microbiota composition and SCFAs production in laying hens. Sci Rep. 7(1):16269. doi: 10.1038/s41598-017-16560-6.
- Borrelli L, Varriale L, Dipineto L, Pace A, Menna LF, Fioretti A. 2021. Insect derived lauric acid as promising alternative strategy to antibiotics in the antimicrobial resistance scenario. Front Microbiol. 12(12):620798. doi: 10.3389/fmicb.2021.620798.
- Braeckman B, Smagghe G, Brutsaert N, Cornelis R, Raes H. 1999. Cadmium uptake and defense mechanism in insect cells. Environ Res. 80(3):231–243. doi: 10.1006/enrs.1998.3897.
- Candian V, Meneguz M, Tedeschi R. 2023. Immune responses of the black soldier fly Hermetia illucens (L.) (Diptera: stratiomyidae) reared on catering waste. Life. 13(1):213. doi: 10.3390/life13010213.
- Carroll A, Fitzpatrick M, Hodge S. 2023. The effects of two organic soil amendments, biochar and insect frass fertilizer, on shoot growth of cereal seedlings. Plants. 12(5):1071. doi: 10.3390/plants12051071.
- Constantinescu-Aruxandei D, Frîncu RM, Capră L, Oancea F. 2018. Selenium analysis and speciation in dietary supplements based on next-generation selenium ingredients. Nutrients. 10(10):1466. doi: 10.3390/nu10101466.
- Diener S, Zurbrügg C, Tockner K. 2015. Bioaccumulation of heavy metals in the black soldier fly, Hermetia illucens and effects on its life cycle. JIFF. 1(4):261–270. doi: 10.3920/JIFF2015.0030.
- European Commission. 2002. Directive No 2002/32 of the European Parliament and of the Council of 7 May 2002 on undesirable substances in animal feed. https://eur-lex.europa.eu/legal-content/EN/TXT/?uri=CELEX%3A32002L0032.
- EC. 2004. Regulation No 767/2009 of the European Parliament and of the Council of 13 July 2009 on the placing on the market and use of feed, amending European Parliament and Council Regulation (EC) No 1831/2003 and repealing Council Directive 79/373/EEC, Commission Directive 80/511/EEC, Council Directives 82/471/EEC, 83/228/EEC, 93/74/EEC, 93/113/EC and 96/25/EC and Commission Decision.
- FAO. 2021. Meat market review: overview of global meat market developments in 2020. FAO. [accessed 2023 February 3]. https://www.fao.org/3/cb3700en/cb3700en.pdf.
- Ferrari L, Sele V, Silva M, Bonilauri P, De Filippo F, Selmin F, Ørnsrud R, Pinott L, Ottoboni M. 2022. Biofortification of selenium in black soldier fly (Hermetia illucens) prepupae reared on seaweed or selenium enriched substrates. JIFF. 8(8):887–899. doi: 10.3920/JIFF2021.0153.
- Gorman MJ. 2023. Iron homeostasis in insects. Annu Rev Entomol. 68(1):51–67. doi: 10.1146/annurev-ento-040622-092836.
- Hu C, Yang L, Wang H, Xiao X, Wang Z, Gong X, Liu X, Li W. 2023. Analysis of heavy metals in the conversion of lake sediment and restaurant waste by black soldier fly (Hermetia illucens). Front Bioeng Biotechnol. 11:1163057. doi: 10.3389/fbioe.2023.1163057.
- Huff J, Lunn RM, Waalkes MP, Tomatis L, Infante PF. 2007. Cadmium-induced cancers in animals and in humans. Int J Occup Environ Health. 13(2):202–212. doi: 10.1179/oeh.2007.13.2.202.
- Janssen RH, Canelli G, Sanders MG, Bakx EJ, Lakemond CMM, Fogliano V, Vincken JP. 2019. Iron-polyphenol complexes cause blackening upon grinding Hermetia illucens (black soldier fly) larvae. Sci Rep. 9(1):2967. doi: 10.1038/s41598-019-38923-x.
- Janssen RH, Vincken JP, Van den Broek LA, Fogliano V, Lakemond CM. 2017. Nitrogen-to-protein conversion factors for three edible insects: Tenebrio molitor, Alphitobius diaperinus, and Hermetia illucens. J Agric Food Chem. 65(11):2275–2278. doi: 10.1021/acs.jafc.7b00471.
- Korish MM, Attia YA. 2020. Evaluation of heavy metal content in feed, litter, meat, meat products, liver, and table eggs of chickens. Animals. 10(4):727. doi: 10.3390/ani10040727.
- Lievens S, Poma G, De Smet J, Van Campenhout L, Covaci L, Van Der Borght M. 2021. Chemical safety of black soldier fly larvae (Hermetia illucens), knowledge gaps and recommendations for future research: a critical review. JIFF. 7(4):383–396. doi: 10.3920/JIFF2020.0081.
- Marono S, Loponte R, Lombardi P, Vassalotti G, Pero ME, Russo F, Gasco L, Parisi G, Piccolo G, Nizza S, et al. 2017. Productive performance and blood profiles of laying hens fed Hermetia illucens larvae meal as total replacement of soybean meal from 24 to 45 weeks of age. Poult Sci. 96(6):1783–1790. doi: 10.3382/ps/pew461.
- Marono S, Piccolo G, Loponte R, Di Meo C, Attia YA, Nizza A, Bovera F. 2015. In vitro crude protein digestibility of Tenebrio molitor and Hermetia Illucens insect meals and its correlation with chemical composition traits. Ital J Anim Sci. 14:3.
- Mehar S, Anam I, Masood Z, Alvi S, Khan W, Kabir M, Shahbaz M, Khan T. 2023. Bioaccumulation of heavy metals in the different tissues of Mackerel scad, Decapterus macarellus (Cuvier, 1833) collected from Karachi and Gwadar coasts of Pakistan. Saudi J Biol Sci. 30(2):103540. doi: 10.1016/j.sjbs.2022.103540.
- Mitra S, Chakraborty AJ, Tareq AM, Emran TB, Nainu F, Khusro A, Idris AM, Khandaker MU, Osman H, Alhumaydhi FA, et al. 2022. Impact of heavy metals on the environment and human health: novel therapeutic insights to counter the toxicity. J King Saud Univ Sci. 34(3):101865. doi: 10.1016/j.jksus.2022.101865.
- Mohan K, Sathishkumar P, Rajan DK, Rajarajeswaran J, Ganesan AR. 2023. Black soldier fly (Hermetia illucens) larvae as potential feedstock for the biodiesel production: recent advances and challenges. Sci Total Environ. 859(Pt 1):160235. doi: 10.1016/j.scitotenv.2022.160235.
- Nairuti RN, Musyoka SN, Yegon MJ, Opiyo MA. 2022. Utilization of black soldier fly (Hermetia illucens Linnaeus) larvae as a protein source for fish feed – a review. Aquacult Stud. 22(2):AQUAST697.
- Paul A, Frederich M, Megido RC, Alabi T, Malik P, Uyttenbroeck R, Francis F, Blecker C, Haubruge E, Lognay G, et al. 2017. Insect fatty acids: A comparison of lipids from three Orthopterans and Tenebrio molitor L. larvae. J Asia-Pacific Entomol. 20(2):337–340. doi: 10.1016/j.aspen.2017.02.001.
- Proc K, Bulak P, Wiącek D, Bieganowski A. 2020. Hermetia illucens exhibits bioaccumulative potential for 15 different elements – Implications for feed and food production. Sci Total Environ. 723:138125. doi: 10.1016/j.scitotenv.2020.138125.
- Romano N, Powell A, Islam S, Fischer H, Renukdas N, Sinha AK, Francis S. 2022. Supplementing aquaponics with black soldier fly (Hermetia illucens) larvae frass tea: effects on the production and composition of sweetpotato slips and sweet banana peppers. Aquaculture. 555:738160. doi: 10.1016/j.aquaculture.2022.738160.
- SAS. 2002. Statistical analysis system: version 9.0. Cary: SAS Institute Inc.
- Saviane A, Tassoni L, Naviglio D, Lupi D, Savoldelli S, Bianchi G, Cortellino G, Bondioli P, Folegatti L, Casartelli M, et al. 2021. Mechanical processing of Hermetia illucens Larvae and Bombyx mori pupae produces oils with antimicrobial activity. Animals. 11(3):783. doi: 10.3390/ani11030783.
- Saxena I, Shekhawat GS. 2013. Nitric oxide (NO) in alleviation of heavy metal induced phytotoxicity and its role in protein nitration. Nitric Oxide. 32:13–20. doi: 10.1016/j.niox.2013.03.004.
- Scieuzo C, Franco A, Salvia R, Triunfo M, Addeo NF, Vozzo S, Piccolo G, Bovera F, Ritieni A, Francia AD, et al. 2023. Enhancement of fruit byproducts through bioconversion by Hermetia illucens (Diptera: stratiomyidae). Insect Sci. 30(4):991–1010. doi: 10.1111/1744-7917.13155.
- Secci G, Dabbou S, Lira de Medeiros AC, Addeo NF, Atallah E, Parisi G, Moniello G, Bovera F. 2022. Low dietary inclusion levels of Tenebrio molitor larva meal slightly modify growth performance, carcass and meat traits of Japanese quail (Coturnix japonica). J Sci Food Agric. 102(14):6578–6585. doi: 10.1002/jsfa.12023.
- Truzzi C, Illuminati S, Girolametti F, Antonucci M, Scarponi G, Ruschioni S, Riolo P, Annibaldi A. 2019. Influence of feeding substrates on the presence of toxic metals (Cd, Pb, Ni, As, Hg) in larvae of Tenebrio molitor: risk assessment for human consumption. Int J Environ Res Public Health. 16(23):4815. doi: 10.3390/ijerph16234815.
- van der Fels-Klerx HJ, Camenzuli L, van der Lee MK, Oonincx DGAB. 2016. Uptake of cadmium, lead and arsenic by Tenebrio molitor and Hermetia illucens from contaminated substrates. PLOS One. 11(11):e0166186. doi: 10.1371/journal.pone.0166186.
- van der Fels-Klerx HJ, Meijer N, Nijkamp MM, Schmitt E, van Loon JJA. 2020. Chemical food safety of using former foodstuffs for rearing black soldier fly larvae (Hermetia illucens) for feed and food use. JIFF. 6(5):475–488. doi: 10.3920/JIFF2020.0024.
- Walker CH. 1990. Kinetic models to predict bioaccumulation of pollutants source: functional ecology. New Horiz Ecotoxicol. 4(3):295–301.
- Wang X, Huang Q, Hao Q, Ran S, Wu Y, Cui P, Yang J, Jiang C, Yang Q. 2018. Insecticide resistance and enhanced cytochrome P450 monooxygenase activity in field populations of Spodoptera litura from Sichuan. China Crop Protect. 106:110–116. doi: 10.1016/j.cropro.2017.12.020.
- Wedwitschka H, Gallegos Ibanez D, Jáquez DR. 2023. Biogas production from residues of industrial insect protein production from black soldier fly larvae Hermetia illucens (L.): an evaluation of different insect frass samples. Processes. 11(2):362. doi: 10.3390/pr11020362.