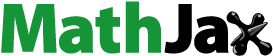
Abstract
Essential oils (EOs) and nature identical compounds (NICs) express different antimicrobial activity (AAc) which can be affected by variability in composition, stability over time, and the carrier employed for their inclusion in feedstuff and products for animal treatments. The aim of the present study was to evaluate the stability over time of the AAc of 8 EOs and 5 NICs, alone and in combination with Tween 20, on four major bacterial livestock pathogens (E. coli, S. Typhimurium, S. aureus and MRSA). For this purpose, minimal inhibitory concentration (MIC) and checkerboard assay (CkA) tests were performed on EOs and NICs at batch first use (T0 – only MIC), and after 12 (T1) and 24 (T2) months. Several EOs showed initial MICs lower than 2% (v/v) and, except for clove bud and cinnamon EOs, reduced their efficacy over time. The best NICs’ AAc was showed at T1 by cinnamic aldehyde (against MRSA), carvacrol (against E. coli), and thymol (except against MRSA), while at T2 the MIC values decreased for most of the NICs. The CkA between EOs and Tween 20 showed different interactions (Fractional Inhibitory Concentration -FIC- index from 0.06 to 32.04) based on bacteria and time. No interactions were found between NICs and Tween 20 (FIC index from 1.0 to 3.0). The AAc of EOs and NICs changes over time. Therefore, the preservation interval of these additives is critical for the AAc of feedstuff and products intended for livestock animals. Moreover, the NICs seem more suitable for the association with Tween 20.
HIGHLIGHTS
EOs vary their antimicrobial activity over time
NICs are more stable than EOs in terms of antimicrobial activity
Tween 20 does not interfere with the antimicrobial activity of NICs, and the interaction is consistent over time.
Introduction
Antibiotics have been widely used at sub-therapeutic doses in animal husbandry to improve growth performances and to prevent infectious diseases (Papatsiros et al. Citation2013). However, antibiotics exert a selective pressure on bacteria allowing the spread of resistances, both in commensal and pathogenic species, thus posing a risk to human and animal health (Low et al. Citation2021). Therefore, in the last decades, under the pressure of the international authorities, the feed industry, and the livestock sector in general, have made strong efforts to reduce the use of antibiotics and promote antibiotic-free productions (Rossi et al. Citation2020). The European Union banned in fact the use of antibiotics as feed additives and encouraged research of alternative molecules, especially of natural origin (Regulation (EC) No. 1831/Citation2003). In this scenario, plants and their by-products can play an important role as additives in animal diets, thanks to their antimicrobial and prebiotic or performance-enhancing effects (Guil-Guerrero et al. Citation2016; Pitino et al. Citation2021). Among these compounds, particular attention was paid to the essential oils and their active pure principles.
Essential oils (EOs) are secondary metabolites of aromatic plants known in traditional medicine as remedies for several diseases (Savoia Citation2012), and they comprehend a complex mixture of various active compounds, able to cross the bacterial cell wall (Bakkali et al. Citation2008; Andrade-Ochoa et al. Citation2018). The EOs express many properties including antimicrobial, antiviral, antiparasitic, and antifungal, as well as antioxidant, anti-inflammatory and analgesic activities (Hammer et al. Citation1999; Chouhan et al. Citation2017; Tsiplakou et al. Citation2021). The composition of the EOs depends on several factors such as extraction procedures, botanical characteristics (e.g. plant species and plant organ; Verma et al. Citation2010), plant harvesting (harvest time and post-harvest storage and processing) (Hammer et al. Citation1999; Aligiannis et al. Citation2001; Rocha et al. Citation2014) and climate/soil conditions (Sivropoulou et al. Citation1995; Sangwan et al. Citation2001; Janssen et al. Citation2007) where the plant matrix is grown. Therefore, different batches from the same EOs may differ in composition. Moreover, due to their hydrophobic nature and high volatility, they tend to be unstable, possibly changing their activity over time (Turek and Stintzing Citation2013). This creates serious technological issues when feedstuff and products containing EOs for livestock use have to be preserved for variable time intervals before their consumption.
Nature Identical Compounds (NICs) are the chemically synthesised pure active principles of EOs and belong to a variety of chemical classes. NICs are considered promising compounds due to their antibacterial activity both alone and in combination with conventional antibiotics, as well as due to their antioxidant activities (Hammer et al. Citation1999; Zyl et al. Citation2006; Giovagnoni et al. Citation2019). In addition, they can be very advantageous, compared to essential oils, for a possible commercial application thanks to their lower costs as well as their ease of use in experimental studies (García Beltrán and Esteban Citation2022).
Due to their chemical characteristics, to be included in commercial feeds, EOs and NICs must be adsorbed or emulsified using carriers. Non-ionic surfactants such as polysorbates represent valid alternatives to this scope (Simoni et al. Citation2020). However, the interaction of both EOs and NICs with carriers may alter their antimicrobial activity (AAc), as reported by several authors (Remmal et al. Citation1993; Pavoni et al. Citation2020). The European Food Safety Authority (EFSA) reported that Tween 20 is an effective feed emulsifier that promotes and maintains the homogeneous distribution of feed materials and/or additives in feedstuffs (European Food Safety Authority Citation2005). The safety of Tween 20 for oral administration was confirmed at a maximum daily exposure of 0-25 mg/kg of body weight/day (Hazardous Substances Data Bank (HSDB), Citation2023). Consequently, its use in feed seems a possible option.
Based on the above, it appears that the profitable use of EOs and NICs can be influenced by several factors, including variability in composition, stability over time, and the carrier employed for their inclusion in animal feeds and products for animal treatments.
The aim of the present study was to evaluate the stability over time of the AAc of eight EOs of known composition and five NICs, alone and in combination with Tween 20, on four major bacterial livestock pathogens, namely E. coli, S. Typhimurium, S. aureus and MRSA, providing information for their proper use in feedstuff or products for livestock animals.
Materials and methods
Products tested
The Tween 20 emulsifier was provided by Sigma Aldrich (batch n. S7922284015).
Essential oils
The tested EOs and NICs were provided by Biotrade of Malavasi Claudio S.N.C. (Mirandola, MO, Italy). Eight EOs were tested in this study: Melaleuca alternifolia leaf oil (tea tree EO, trade code OE5370), Menthae piperitae aetheroleum herb oil (peppermint EO, trade code - t.c. - OE0228), Origanum vulgare leaf oil (oregano Spain EO, t.c. OE0375), Cinnamomum zeylanicum leaf oil (cinnamon Ceylon leaf EO, t.c. OE0576), Eugenia caryophyllus bud oil (clove bud EO, t.c. OE0898), Thymus vulgaris leaf oil (thyme EO, t.c. OE0969), Lavandula angustifolia herb oil (lavender altitude EO, t.c. OE0985) and Rosmarinus officinalis leaf oil (rosemary EO, t.c. OE1318).
Nature identical compounds
The NICs tested were 5 and included Carvacrol – code S0404041; Cinnamic aldehyde – code S0400710; Terpineol – code S0400648; Thymol – code S0400715; Menthol – code S0401789.
Gas chromatographic analysis of EOs
For EOs characterisation, after a preliminary steam distillation extraction lasting four hours and performed using a Clevenger apparatus, the procedure reported by Mongelli et al. (Citation2016) was applied. Briefly, samples were analysed through a Thermo Scientific (SanJose, CA, USA) TRACE 1300 gas-chromatograph coupled to a Thermo Scientific ISQTM Single Quadrupole mass spectrometer. The gas-chromatograph was equipped with Supelcowax 10 (30 m × 0.25 mm, f.t. 0.25 μm) (Supelco, Bellefonte, PA, USA) capillary columns; helium was used as carrier gas (1 ml min − 1). The temperature gradient started from 50 °C, maintained for 3 min, followed by a rise to the final temperature of 200 ◦C at the rate of 5 °C/min, maintained for 18 min. The injector operated in split modality (ratio 1:20) at 230 °C. The mass spectrometer, equipped with an electron impact source (EI, 70 eV) was set in full scan (from 40 m/z to 500 m/z) acquisition mode, applying a solvent delay time of 4 min. The main volatile compounds were by comparison with reference mass spectra libraries (WILEY275, NBS75K, Adams 2001) and by their calculated Retention Indexes through the application of the Kovats’ formula (KI). A mixture of alkanes (C8–C20) was injected in the GC-MS equipment and analysed under the same conditions described above. In order to determine the linear retention index (LRI) of the components, a manual integration of the gas-chromatographic signals was performed, and the peak areas were compared with the total sum of the areas and expressed in percentage (mean ± sd). The amount of EOs’ active principles was determined through calibration curves built using synthetic standards and expressed as mg/100 mg.
Tested bacteria and bacterial inoculum preparation
The tests were performed on four reference bacterial strains of veterinary interest: two Gram-positive (Staphylococcus aureus ATCC 25923– SA and Methicillin-Resistant Staphylococcus aureus ATCC 43300– MRSA) and two Gram-negative (E. coli ATCC 25922– EC and Salmonella enterica, subsp. enterica, serovar Typhimurium ATCC 14028– ST), all purchased from ATCC® (USA). The bacterial inoculums were prepared following the CLSI guidelines. All microbiological assays were performed within 30 min after inoculums standardisation (Clinical and Laboratory Standards Institute (CLSI), Citation2015). Briefly, five bacterial colonies from solid fresh cultures of each tested strain were inoculated in sterile tubes with MHB and incubated at 37 °C in aerobiosis for 24 h. After incubation, the bacterial suspension was centrifuged at 2000 x g at 4 °C for 20 min to separate the bacterial pellet from the supernatant. Then, the pellet was resuspended in phosphate buffer (PB) 10 mM, pH 7. The bacterial suspension was adjusted in PB to obtain an optical density (OD) value in the range 0.08–0.13 at 600 nm in a 1 cm light path cuvette, approximately equivalent to a 108 CFU/mL suspension. This suspension was further diluted 1:100 in sterile MHB. Fifty microliters of the bacterial suspension containing 106 CFU/mL were inoculated into each well, to obtain a final concentration of 5∙105 CFU/mL. Bacterial suspensions were assessed through a Biophotometer plus (Eppendorf, Hamburg, Germany) spectrophotometer (λ = 600 nm).
Minimal inhibitory concentration assay of EOs and NICs
The Minimal Inhibitory Concentration (MIC) assay of EOs and NICs was performed following the methods reported by Spaggiari et al. (Citation2023) with minor modifications. The EOs concentrations were expressed as percentages (% v/v) (Verma R.S. et al. 2010). Briefly, each EO was diluted to a concentration of 6.25% in MHB to obtain the stock solution. Twofold dilutions of each oil ranging from 3.125% to 0.0061% were performed in a 96-well microtiter plate (Greiner, Milan, Italy). Due to the intrinsic turbidity of EOs in broth solution, a resazurin assay was performed to evaluate the effective inhibition or growth: ten microliters of resazurin at 0.02% in Phosphate Buffer (PB) pH 7 were added in each well and incubated for two hours at 37 °C in the dark before reading (Sarker et al. Citation2007). NICs were diluted to a stock solution in DMSO at different concentrations based on preliminary tests: at 102.4 mg/mL for carvacrol, terpineol, and cinnamic aldehyde and 204.8 mg/mL for thymol and menthol. Subsequently, each compound was further diluted to a concentration of 4096 µg/mL in MHB.
The antibacterial activity of Tween 20 alone was evaluated by testing a range of concentrations from 25% to 0.05%.
Antimicrobial activity of EOs and NICs over time
The MIC values of EOs (% v/v) and NICs (µg/mL) were preliminary evaluated at T0 in February 2020 and subsequently at two different time points with intervals of approximately 1 year: February 2021 (T1); and February 2022 (T2). During this period, EOs were stored in their original packaging, protected from light, at +4 °C. The NICs were stocked in DMSO solution at the concentrations mentioned above and under the same conditions previously described.
Checkerboard assays
The associations EOs-Tween 20 and NICs-Tween 20 were investigated by checkerboard assay (CkA) performed as described by Meletiadis et al. (Citation2010). Ten serial dilutions of each EO at sub-MIC concentrations were tested in combination with Tween 20 in MHB. Regarding the association NICs-Tween 20, carvacrol, thymol, and cinnamic aldehyde were tested at concentrations ranging from 256.00 to 1.00 µg/mL, while menthol and terpineol were tested at concentrations ranging from 2048.00 to 8.00 µg/mL.
In all CkA, the Tween 20 concentration ranged from 25.00 to 0.40%, v/v.
The Fractional Inhibitory Concentration Index (FIC index) was calculated to evaluate the antimicrobial effect of each association. The MICs of each compound tested individually and in combination with the carrier were evaluated through the following formula:
where MICA in combination and MICB in combination represent the MIC values obtained for each compound (EO or NIC and emulsifier) in combination, and MICA and MICB represent the MIC values obtained for each compound alone (EUCAST Citation2000). Based on the FIC index value, the AAc of the associations was interpreted as ‘synergy’ if the FIC was ≤ 0.5; ‘no interaction’ when 0.5 < FIC ≤ 4.0; or ‘antagonism’, if the FIC was > 4.0 (Odds Citation2003). The CkA with EOs and NICs in combination with Tween 20 were performed at T1 and T2. At T0 CkA was not performed due to restricted access to laboratories during the COVID-19 pandemic.
Results
Chemical composition of EOs
The chemical composition and the active principles of the eight tested EOs, evaluated at batch first use (T0), is reported in Tables and . Lavender EO was composed for more than 70% by β-Linalool and Linalyl acetate; clove bud and cinnamol oils contained eugenol for more than 80 and around 65% respectively; mint EO was represented by menthol and p-menthan-3-one for more than 75%, while in oregano EO, carvacrol and cymene together were around 80%. Eucaliptol and camphol represented about 65% of the rosemary and a similar percentage of cymene and thymol composed the thyme EO; finally, tea tree was comprised mainly of terpinen-4-ol (around 40%) and secondarily by cymene and α-terpineol, which together amounted to almost 30% of the EO. Concerning the antimicrobial activity, thymol was the main active compound of lavender (together with carvacrol), rosemary and thyme (along with carvacrol) EOs; carvacrol was the main active compound of clove bud, cinnamon (together with cinnamic aldehyde and menthol) and oregano (in association to thymol and menthol) EOs. Menthol and terpineol were the main active molecules of mint while terpineol, carvacrol and thymol were the main tea tree EO active molecules.
Table 1. Phytochemical composition of the essential oils tested (mean relative percentage ± standard deviation).
Table 2. Amount of active principles (mg/100 mg) in the essential oils, calculated through calibration curves built with synthetic standards.
EOs
Preliminary MIC evaluations of EOs and Tween 20 batches at T0
The AAc of Tween 20 alone showed a MIC value of 25.00% against all the bacteria tested, except for EC (27.80 ± 8.30%).
For all the tested EOs, the average MIC value was lower than 5.00% and for several of them was lower than 2.00%. The overall average MIC values for each bacterium were: 1% for EC, 1.20% for MRSA, and 1.50% for ST and SA. The lowest MIC values were found for oregano oil (0.03% ± 0.04%), followed by thyme (0.08% ± 0.02%), tea tree (0.36% ± 0.20%) and rosemary oils (1.21% ± 0.62%). The highest MIC values were found for lavender (2.93% ± 0.14%) and mint oils (2.12% ± 0.93%), followed by clove bud and cinnamon oils (1.93% ± 0.41% and 1.24% ± 0.26%, respectively). Considering all tested bacteria, the lowest mean MIC value was obtained against EC (1.02% ± 1.00%), followed by SA (1.19% ± 1.03%), MRSA (1.26% ± 1.05%) and ST (1.48% ± 1.32%).
EOs MIC values at T1
At T1, the MIC values against all the tested bacteria increased for tea tree, rosemary, thyme, and lavender oils. The mint action against EC halved, while oregano and mint oils preserved their activity. Conversely, cinnamon and clove bud oils’ MIC values decreased against all the tested bacteria after one year. Tea tree oil MIC was 15.5, 10 and 8 times higher against the tested Gram-negative bacteria, SA, and MRSA, respectively. At T1, rosemary oil MIC values were 9 times higher for EC, and 6, 7 and 5 times higher for ST, SA, and MRSA, respectively. Thyme oil MIC values were higher by 32 times for EC, 8 for ST, 4 for SA and 4 for MRSA at T1. Lavender oil MIC values doubled at T1 against EC, ST, and MRSA while they were similar for SA. Cinnamon oil reported a 5.5-, 7-, 4.5- and 7.5-fold decrease of MIC values at T1 on EC, ST, SA, and MRSA respectively. For clove bud oil, a 16-fold decrease MIC values at T1 for EC and ST, 24 for SA and 10-fold for MRSA were found.
EOs MIC values at T2
MIC values generally increased at T2 in comparison to T0, except for clove bud oil. The greatest increase in MIC value was observed for thyme oil against ST and EC, with a 31.2 and 26-fold increment respectively, while the MIC increased only by two times against MRSA. The tea tree and rosemary oils showed higher MIC values, less evident in Gram-positive than in the Gram-negative tested bacteria. Cinnamon oil mostly improved its activity showing a 1.5-fold MIC reduction against ST and SA and a decrease by 2.7 times against MRSA.
The activity against MRSA appeared to be the most improved with an 8.4-fold increment for mint oil, followed by clove bud (4.2-fold) and oregano (3.3-fold) oils. At T2, oregano and clove bud oils showed lowest the MIC values overall.
The MIC values of the EOs tested at the three timepoints are reported in Table .
Table 3. MIC (% v/v) of essential oils (EOs) against tested bacteria at the batch initial evaluation, after one year (T1), and after 2 years (T2).
NICs
Preliminary MIC evaluation of NICs batches at T0
The lowest MIC value was found for cinnamic aldehyde against SA (128 µg/mL), while a MIC value of 256 µg/mL was found against the other tested strains. For carvacrol and thymol the MIC value was 256 µg/mL against all tested strains. The highest MIC values were recorded by both terpineol and menthol: the former presented the same MIC value (2048 µg/mL) against all strains tested while menthol showed MIC values between 4096 and 1251 µg/mL against the different bacteria tested.
NICs’ MIC evaluation at T1
At T1, the tested NICs generally showed the same MIC values of the initial evaluation. Exceptions were cinnamic aldehyde and carvacrol, whose MIC value halved at T1 against EC and MRSA, as well as terpineol, whose MIC value halved towards EC. Thymol showed halved MIC values against EC, ST, and SA, while menthol oil’s MIC decreased by 1.1, 1.2 and 1.8 times against EC, SA, and MRSA respectively.
NICs’ MIC evaluation at T2
At T2, the MIC values showed a decrease compared to T0, while they slightly increased compared to T1. The most important reduction was found for Terpineol against ST (2.12 times) and carvacrol towards ST and SA, whose MIC values halved at T2 compared to T1. A slight increase of the MIC value at T2 compared to T1 was found for carvacrol against EC and MRSA, cinnamic aldehyde against MRSA, and thymol against SA and MRSA. The MIC values of terpineol and menthol remained the highest at T2, without variation of the MIC values of terpineol on Gram-positive strains. The MIC values of terpineol decreased by 2.12 times against Gram-negative strains compared to the initial MIC value, while for menthol, the MIC value halved against SA in the whole interval considered.
The MIC values of the NICs tested at the three timepoints are reported in Table .
Table 4. MIC (µg/mL) values of nature identical compounds (NICs) at the batch initial evaluation, after one year (T1), and after 2 years (T2) against reference bacteria strains of zootechnical interest.
Checkerboard assays of EOs over time
Due to the Covid-19 outbreak, it was not possible to perform the initial CkA of EOs. The FIC indexes evaluations generated by the CkA are reported in Table . Generally, the combinations of EOs and Tween 20 showed a prevalence of no interaction (53.13%) compared to synergy (37.50%) and antagonism (9.37%). Furthermore, the worst activity was observed for the association Tween 20 – cinnamon oil, which showed antagonism of action against most of the bacteria. For many associations, the AAc worsened, and the FIC index increased over time. In general, the interaction between EOs and Tween 20 worsened over time also at T2, but less frequently against SA. Tween 20 combined with rosemary, thyme, mint, and lavender oils showed synergy of action against EC, as observed on ST with mint and lavender oils. Concerning the action towards Gram-positive bacteria, tea tree, rosemary and mint oils showed synergy with Tween 20 against SA, while only thyme, mint and lavender were synergic with the carrier against MRSA.
Table 5. FIC indexes of essential oils (EOs) with Tween 20 after one year (T1), and after 2 years (T2) from the batch initial evaluation, against reference bacteria strains of zootechnical interest.
At T2, the absence of interaction was observed in 62.50% of all the EOs-Tween 20 combinations, followed by antagonism (28.13%) and synergy (9.38%). At this time, a synergy of action was seen only for the combinations of Tween 20 with rosemary oil against SA and MRSA and thyme against SA.
Checkerboard NICs-Tween 20 assays over time
Due to the Covid-19 outbreak, it was not possible to perform the initial CkA of NICs. CkA performed at T1 showed no interactions of the tested NICs with the carrier against all the considered bacteria (Table ). Overall, Tween 20 did not influence the AAc of NICs. The evaluation of NICs associated with Tween 20 at T2 did not show any changes in the interaction in comparison to T1, despite the variations in FIC indexes and MIC values of NICs.
Table 6. FIC indexes of nature identical compounds (NICs) with Tween 20 after one year (T1), and after 2 years (T2) from the batch initial evaluation, against reference bacteria strains of zootechnical interest.
Discussion
The results obtained show that EOs and NICs express antimicrobial proprieties against reference bacterial strains of zootechnical interest both alone and in combination with Tween 20. The average MIC values of EOs obtained at T0 on Gram positive were lower than those found against Gram negative. It is well known that the AAc of EOs is stronger on Gram-positive than Gram-negative bacteria. This appears to be due to the presence of lipopolysaccharide (LPS) in Gram-negative bacteria, which prevents the oil from accumulating on the cell membrane (Smith-Palmer et al. Citation1998).
Overall, the EOs showing the highest AAc on all the tested strains were oregano, thyme, and tea tree. This is probably due to their chemical composition, being oregano and thyme oils rich in specific phenolic compounds, namely carvacrol and thymol, that strengthen their antimicrobial properties (Chouhan et al. Citation2017). Data on the chemical composition of EOs show in fact that thymol is abundantly present in the thyme oil while carvacrol is well represented in the oregano oil. Tea tree oil is rich in 4-terpineol, having also a considerable AAc (Brun et al. Citation2019). As reported by Hammer et al. (Citation1999), the comparison of the AAc of EOs between studies could be problematic and misleading since the antimicrobial effect of EOs depends both on the method adopted for the evaluation and on factors that could influence their chemical composition, including the plant organ of extraction (e.g. leaves or flowers) or the plant stage of maturity at harvesting (Turek and Stintzing Citation2013). Furthermore, each active fraction of EOs can interact with the others, leading to a possible synergistic effect influencing the AAc of each EO (Tardugno et al. Citation2018). Taking this into account, our initial data showed higher MIC values in some cases (e.g. lavender on SA and EC) and lower MIC values in others (e.g. thyme on ST) when compared to findings from Hammer et al. (Citation1999). However, the MIC values of tea tree, rosemary and oregano were comparable to those reported by the cited author.
The evaluation of AAc of EOs at different time intervals (T1 and T2) showed marked differences when compared to MIC values obtained at T0. Despite some exceptions (cinnamon and clove bud oils), the overall MIC values increased over time, suggesting a possible variation of the intrinsic composition of each EO related to individual molecule degradation or modifications. These variations were not investigated in the present study and need to be considered in future studies. The stability of essential oils can depend on various factors including the preservation temperature and exposure to light, which in turn affect the occurrence of autoxidation reactions. The latter can generally be prevented by EOs compounds expressing strong antioxidant action (e.g. phenolic compounds such as carvacrol, thymol and eugenol) whose specific content affects oxidation over time (Turek and Stintzing Citation2013; Marchese et al. Citation2017). This could therefore justify the greater stability over time of oregano and thyme, both rich in carvacrol and thymol, and the improvement over time of the activity of clove bud and cinnamon, both rich in eugenol, very effective in preventing auto-oxidation (Turek and Stintzing Citation2013). Analysis of the composition of the EOs at different preservation intervals coupled with MIC evaluation should be carried out to verify how these changes occur and affect their AAc.
The MIC values of the NICs at T0 showed that cinnamic aldehyde, thymol and carvacrol were the compounds with the greatest AAc. These results are consistent with those reported by Gallucci et al. (Citation2009) and Ye et al. (Citation2013). The MIC of cinnamic aldehyde on the Gram-negative strains was significantly lower if compared to those obtained by Pereira et al. (Citation2021) who found values of 780.00-3120.00 µg/mL on different pathogenic and not-pathogenic EC strains. The initial MIC values of carvacrol and thymol were found identical for all the tested strains and were comparable to data obtained in another study where the same compounds were tested against SA (511.84 ± 299.76 μg/mL and 384.21 ± 78.40 μg/mL, respectively; Rúa et al. Citation2019). The MIC values decreased, or in some cases remained unchanged during the storage, possibly suggesting a higher stability over time of NICs compared to EOs.
Important outcomes of the present work are the results obtained relative to the AAc of the associations of EOs and NICs with Tween 20. For the interpretation of FIC index, the criteria proposed by Odds (Citation2003) were followed since are more selective for synergies, differently from the approaches by EUCAST (Citation2000) and Fratini et al. (Citation2017) which, additionally, deal with antibiotics and combinations between EOs respectively. In the present study, the associations of both EOs and NICs with an emulsifier were evaluated over time, thus the Odds’s approach, was adopted homogeneously for EOs and NICs. As reported by Pavoni et al. (Citation2020), the associations with Tween 20 can have various effects based on the mechanisms of interaction: for instance, the higher solubility of some EOs in Tween 20 may reduce the microorganism-molecule interaction. Our results confirm how different EOs may have a different interaction with the Tween 20. Most of them showed indifference or synergy in the various combinations on the different bacteria already at T1. However, at the same interval, the combination of cinnamon oil and Tween 20 showed antagonism on EC, ST, and MRSA. It should be noted here that the checkerboard assay was first performed one year after the batch first use (this evaluation was impossible at T0 due to the COVID-19 pandemic outbreak) and the cinnamon oil could have degraded over time. At T2, on the other hand, a worsening of the results was observed for many combinations, which could be related to the decline in AAc observed also in the individual MIC data at different times. Thus, the instability of AAc over time could also lead to a worsening of the activity expressed in combinations with Tween 20 as well as to a modification of the interaction between the EO and the emulsifier.
The association of NICs with Tween 20 in the checkerboard assay showed only the absence of interaction at both T1 and T2, suggesting that this emulsifier does not negatively affect the AAc of the tested NICs. Furthermore, the associations of NICs with Tween 20 were more stable over time than the ones with EOs. The advantage offered by NICs compared to EOs is the standardisation of the activity of the active principle. This is crucial when NICs are employed as feed additives or in other products for animal treatments, to improve the stability and obtain the reproducibility of the antimicrobial effect of NICs associations with other compounds, like emulsifiers or excipients (Rossi et al. Citation2020).
Conclusions
Except for cinnamon and clove bud, the tested EOs show a decrease in AAc, particularly after one year from the batch’s first use, while the NICs seem in general to increase or maintain their AAc over time. The tested EOs interact with Tween 20 in different ways based on bacteria and time while NICs do not interact with the emulsifier. The preservation interval of EOs and NICs used as additives can be critical in determining the AAc of feedstuff and products intended for livestock animals. Moreover, the NICs seems more suitable for the association with the emulsifier Tween 20.
Disclosure statement
The authors report there are no competing interests to declare.
Data availability statement
Data will be shared on request.
Additional information
Funding
References
- Aligiannis N, Kalpoutzakis E, Mitaku S, Chinou IB. 2001. Composition and antimicrobial activity of the essential oils of two Origanum species. J Agric Food Chem. 49(9):4168–4170. doi: 10.1021/jf001494m.
- Andrade-Ochoa S, Sánchez-Aldana D, Chacón-Vargas KF, Rivera-Chavira BE, Sánchez-Torres LE, Camacho AD, Nogueda-Torres B, Nevárez-Moorillón GV. 2018. Oviposition deterrent and larvicidal and pupaecidal activity of seven essential oils and their major components against Culex quinquefasciatus say (Diptera: culicidae): Synergism–antagonism effects. Insects. 9(1):25. doi: 10.3390/insects9010025.
- Bakkali F, Averbeck S, Averbeck D, Idaomar M. 2008. Biological effects of essential oils - A review. Food Chem Toxicol. 46(2):446–475. doi: 10.1016/j.fct.2007.09.106.
- Bianchi F, Careri M, Mangia A, Musci M. 2007. Retention indices in the analysis of food aroma volatile compounds in temperature-programmed gas chromatography: database creation and evaluation of precision and robustness. J Sep Sci. 30:563–572.
- Brun P, Bernabè G, Filippini R, Piovan A. 2019. In vitro antimicrobial activities of commercially available tea tree (Melaleuca alternifolia) essential oils. Curr Microbiol. 76(1):108–116. doi: 10.1007/s00284-018-1594-x.
- Chouhan S, Sharma K, Guleria S. 2017. Antimicrobial activity of some essential oils—Present status and future perspectives. Medicines. 4(3):58. doi: 10.3390/medicines4030058.
- Clinical and Laboratory Standards Institute (CLSI). 2015. Methods for dilution antimicrobial susceptibility tests for bacteria that grow aerobically; approved standards. In. CLSI document (9th ed., p. M07–A9). Wayne, PA: CLSI.
- EUCAST. 2000. Terminology relating to methods for the determination of susceptibility of bacteria to antimicrobial agents. Clin Microbiol Infect. 6:503–508.
- European Food Safety Authority. 2005. Opinion of the Scientific Panel on additives and products or substances used in animal feed (FEEDAP) on the updating of the criteria used in the assessment of bacteria for resistance to antibiotics of human or veterinary importance. Efsa J. 3:223.
- Fratini F, Mancini S, Turchi B, Friscia E, Pistelli L, Giusti G, Cerri D. 2017. A novel interpretation of the Fractional Inhibitory Concentration Index: the case Origanum vulgare L. and Leptospermum scoparium J. R. et G. Forst essential oils against Staphylococcus aureus strains. Microbiol Res. 195:11–17. doi: 10.1016/j.micres.2016.11.005.
- Gallucci MN, Oliva M, Casero C, Dambolena J, Luna A, Zygadlo J, Demo M. 2009. Antimicrobial combined action of terpenes against the food-borne microorganisms Escherichia coli, Staphylococcus aureus and Bacillus cereus. Flavour & Fragrance J. 24(6):348–354. doi: 10.1002/ffj.1948.
- García Beltrán JM, Esteban MÁ. 2022. Nature-identical compounds as feed additives in aquaculture. Fish Shellfish Immunol. 123:409–416. doi: 10.1016/j.fsi.2022.03.010.
- Giovagnoni G, Tugnoli B, Piva A, Grilli E. 2019. Organic acids and nature identical compounds can increase the activity of conventional antibiotics against clostridium perfringens and enterococcus cecorum in vitro. J Appl Poult Res. 28(4):1398–1407. doi: 10.3382/japr/pfz101.
- Guil-Guerrero JL, Ramos L, Moreno C, Zúñiga-Paredes JC, Carlosama-Yepez M, Ruales P. 2016. Antimicrobial activity of plant-food by-products: a review focusing on the tropics. Livest Sci. 189:32–49. doi: 10.1016/j.livsci.2016.04.021.
- Hammer KA, Carson CF, Riley TV. 1999. Antimicrobial activity of essential oils and other plant extracts. J Appl Microbiol. 86(6):985–990. doi: 10.1046/j.1365-2672.1999.00780.x.
- Hazardous Substances Data Bank (HSDB). 2023. [accessed May 2023]. 1928 - PubChem. https://pubchem.ncbi.nlm.nih.gov/source/hsdb/1928.
- Janssen AM, Scheffer JJC, Svendsen AB, Scheffer C, Baerheim Svendsen A. 2007. Antimicrobial activity of essential oils: a 1976-1986 literature review. Aspects Test Methods. Planta Med. 53:395–398.
- Low CX, Tan LTH, Mutalib NSA, Pusparajah P, Goh BH, Chan KG, Letchumanan V, Lee LH. 2021. Unveiling the impact of antibiotics and alternative methods for animal husbandry: a review. Antibiotics. 10(5):578. doi: 10.3390/antibiotics10050578.
- Marchese A, Barbieri R, Coppo E, Orhan IE, Daglia M, Nabavi SF, Izadi M, Abdollahi M, Nabavi SM, Ajami M. 2017. Antimicrobial activity of eugenol and essential oils containing eugenol: a mechanistic viewpoint. Crit Rev Microbiol. 43(6):668–689. doi: 10.1080/1040841X.2017.1295225.
- Meletiadis J, Pournaras S, Roilides E, Walsh TJ. 2010. Defining fractional inhibitory concentration index cutoffs for additive interactions based on self-drug additive combinations, Monte Carlo simulation analysis, and in vitro-in vivo correlation data for antifungal drug combinations against Aspergillus fumi. Antimicrob Agents Chemother. 54(2):602–609. doi: 10.1128/AAC.00999-09.
- Mongelli A, Rodolfi M, Ganino T, Marieschi M, Caligiani A, Dall’Asta C, Bruni R. 2016. Are Humulus lupulus L. ecotypes and cultivars suitable for the cultivation of aromatic hop in Italy? A phytochemical approach. Ind Crops Prod. 83:693–700. doi: 10.1016/j.indcrop.2015.12.046.
- Odds FC. 2003. Editorial synergy, antagonism, and what the chequerboard puts between them. J Antimicrob Chemother. 52(1):1–1. doi: 10.1093/jac/dkg301.
- Papatsiros VG, Katsoulos PD, Koutoulis KC, Karatzia M, Dedousi A, Christodoulopoulos G. 2013. Alternatives to antibiotics for farm animals. CAB Reviews: perspectives in Agriculture, Veterinary Science, Nutrition and Natural Resources. 8:1–15. doi: 10.1079/PAVSNNR20138032.
- Pavoni L, Perinelli DR, Bonacucina G, Cespi M, Palmieri GF. 2020. An overview of micro- and nanoemulsions as vehicles for essential oils: formulation, preparation and stability. Nanomaterials. 10(1):135. doi: 10.3390/nano10010135.
- Pereira WA, Pereira CDS, Assunção RG, Silva I d, Rego FS, Alves LSR, Santos JS, Nogueira FJR, Zagmignan A, Thomsen TT, et al. 2021. New insights into the antimicrobial action of cinnamaldehyde towards escherichia coli and its effects on intestinal colonization of mice. Biomolecules. 11(2):302. doi: 10.3390/biom11020302.
- Pitino R, Marchi MD, Manuelian CL, Johnson M, Simoni M, Righi F, Tsiplakou E. 2021. Plant feed additives as natural alternatives to the use of synthetic antioxidant vitamins on yield, quality, and oxidative status of poultry products: a review of the literature of the last 20 years. Antioxidants. 10(5):757. doi: 10.3390/antiox10050757.
- Regulation (EC) No. 1831/ 2003. The European Parliament and of the Council of 22 September 2003 on additives for use in animal nutrition.
- Remmal A, Bouchikhi T, Rhayour K, Ettayebi M, Tantaoui-Elaraki A. 1993. Improved method for the determination of antimicrobial activity of essential oils in agar medium. J Essent Oil Res. 5(2):179–184. doi: 10.1080/10412905.1993.9698197.
- Rocha RP, Melo E de C, Barbosa LCA, Santos R d, Cecon PR, Dallacort R, Santi A. 2014. Influence of plant age on the content and composition of essential oil of Cymbopogon citratus (DC.) Stapf. J Med Plants Res. 8:1121–1126.
- Rossi B, Toschi A, Piva A, Grilli E. 2020. Single components of botanicals and nature-identical compounds as a non-antibiotic strategy to ameliorate health status and improve performance in poultry and pigs. Nutr Res Rev. 33(2):218–234. doi: 10.1017/S0954422420000013.
- Rúa J, Valle PD, Arriaga DD, Fernández-Álvarez L, García-Armesto MR. 2019. Combination of Carvacrol and Thymol: antimicrobial activity against Staphylococcus aureus and antioxidant activity. Foodborne Pathog Dis. 16(9):622–629. doi: 10.1089/fpd.2018.2594.
- Sangwan NS, Farooqi AHA, Shabih F, Sangwan RS. 2001. Regulation of essential oil production in plants. Plant Growth Regul. 34(1):3–21. doi: 10.1023/A:1013386921596.
- Sarker SD, Nahar L, Kumarasamy Y. 2007. Microtitre plate-based antibacterial assay incorporating resazurin as an indicator of cell growth, and its application in the in vitro antibacterial screening of phytochemicals. Methods. 42(4):321–324. doi: 10.1016/j.ymeth.2007.01.006.
- Savoia D. 2012. Plant-derived antimicrobial compounds: alternatives to antibiotics. Future Microbiol. 7(8):979–990. doi: 10.2217/fmb.12.68.
- Simoni M, Temmar R, Bignamini DA, Foskolos A, Sabbioni A, Ablondi M, Quarantelli A, Righi F. 2020. Effects of the combination between selected phytochemicals and the carriers silica and Tween 80 on dry matter and neutral detergent fibre digestibility of common feeds. Ital J Anim Sci. 19(1):723–738. doi: 10.1080/1828051X.2020.1787882.
- Sivropoulou A, Kokkini S, Lanaras T, Arsenakis M. 1995. Antimicrobial activity of mint essential oils. J Agric Food Chem. 43(9):2384–2388. doi: 10.1021/jf00057a013.
- Smith-Palmer A, Stewart J, Fyfe L. 1998. Antimicrobial properties of plant essential oils and essences against five important food-borne pathogens. Lett Appl Microbiol. 26(2):118–122. doi: 10.1046/j.1472-765x.1998.00303.x.
- Spaggiari C, Righetti L, Spadini C, Annunziato G, Nsanzurwimo A, Cabassi CS, Bruni R, Costantino G. 2023. Metabolite profiling and bioactivities of leaves, stems, and flowers of Rumex usambarensis (Dammer) dammer, a traditional African medicinal plant. Plants. 12(3):482. doi: 10.3390/plants12030482.
- Tardugno R, Spagnoletti A, Grandini A, Maresca I, Sacchetti G, Pellati F, Benvenuti S. 2018. Chemical profile and biological activities of Cedrelopsis grevei H. Baillon bark essential oil. Plant Biosyst. 152(1):120–129. doi: 10.1080/11263504.2016.1255271.
- Tsiplakou E, Pitino R, Manuelian CL, Simoni M, Mitsiopoulou C, Marchi MD, Righi F. 2021. Plant feed additives as natural alternatives to the use of synthetic antioxidant vitamins in livestock animal products yield, quality, and oxidative status: a review. Antioxidants. 10(5):780. doi: 10.3390/antiox10050780.
- Turek C, Stintzing FC. 2013. Stability of essential oils: a review. Comp Rev Food Sci Food Safe. 12(1):40–53. doi: 10.1111/1541-4337.12006.
- Verma RS, Rahman L, Verma RK, Chauhan A, Yadav AK, Singh A. 2010. Essential oil composition of menthol mint (Mentha arvensis L.) and peppermint (Mentha piperita L.) cultivars at different stages of plant growth from Kumaon region of Western Himal. J Med Arom Plants. 1:13–18.
- Ye H, Shen S, Xu J, Lin S, Yuan Y, Jones GS. 2013. Synergistic interactions of cinnamaldehyde in combination with carvacrol against food-borne bacteria. Food Control. 34(2):619–623. doi: 10.1016/j.foodcont.2013.05.032.
- Zyl RV, Seatlholo ST, Vuuren SV, Viljoen AM. 2006. The biological activities of 20 nature identical essential oil constituents. J Essent Oil Res. 18(sup1):129–133. doi: 10.1080/10412905.2006.12067134.