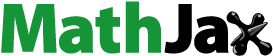
Abstract
To maximise the black soldier fly (BSF) bioconversion capacity, a waste that is not suitable for use as it is, can have a positive effect if combined with others in a formulation. In this perspective, a total of 3600 of 6-day-old larvae were inoculated on 6 iso-proteic, iso-lipidic and iso-energetic diets (named from D1 to D6; 100 larvae/replicate; 6 replicates/treatment) formulated with 21 by-products. To evaluate growth performance, 30 larvae per replicate were randomly sampled every 4 days until the 40% reached the prepupae phase, in which survival rate (SR), larva-prepupae time duration and bioconversion indexes were calculated. When a pupa was identified, it was moved in the emergence box (23 pupae/treatment) to evaluate the adult parameters: pupa-fly duration time, fly live weight, weight reduction, fly lifespan, puparium weight and emergence rate (ER). Considering SR (>92%) and ER (>94.6%), all diets were suitable for the BSF breeding. The D1 and D5 (composed by brewers spent grain, vegetable and fruit waste) groups overall performed the best larvae growth and development time, while D2 (composed by glaze waste and buffalo milk whey) displayed the worst (p < 0.05). The D5 maintained good weight in prepupae, pupae and adult stages and also D2 reached the same outcome. The bioconversion indexes followed the trend of the larvae performance. In conclusion, the results herein obtained allowed to discriminate the protein, fat and energy variables on the BSF life history traits and observe other factors of influence, such as the ash content, which will need to be evaluated with future research.
HIGHLIGHTS
Iso-nutrients diets allow to eliminate the lipid/protein and energy effects on the BSF life history traits.
The use of multiple waste in a diet allows to reduce the inclusion of those that generate better results on growth, which will be most in demand on the market.
Introduction
In nature, black soldier fly (BSF, Hermetia illucens) usually grows on decaying organic materials, and for this reason it is able to bio-convert organic agro-industrial waste into larval biomass (Siddiqui et al. Citation2022). Since in the last decades the environmental sustainability is a clue social issue, the application of natural systems for human waste enhancement arouses great interest and the research is focusing on it. To comply with a circular economy model, waste derived from food and feed production that have an environmental vocation are used in the main production surrounding areas (Ingrao et al. Citation2018). Worldwide, the variety of the waste generates a diversity in the substrate nutritional composition, which leads to a difficulty or impossibility of comparing the research results on larvae growth (Gold et al. Citation2020). Moreover, some waste has already been repeatedly tested – such as brewers’ grain, exhausted yeast, rice straw vegetable and fruits waste (Manurung et al. Citation2016; Chia et al. Citation2018; Meneguz et al. Citation2018) – while others not (coffee silvery film, vinegar and glaze waste, vinasse and broken eggs). Among the waste evaluated, the brewers grain seems to be the only that guaranteed good BSF growth performance also when is not mixed with others ingredients (Chia et al. Citation2018). On the other side, the use of rice straw, fruit and vegetable as unique substrate reduced the growth and the bioconversion capacity of BSF due to their high content of cellulose and poor nutritional composition, respectively (Kim et al. Citation2021; Liu et al. Citation2021). Considering that the nutritional aspects influence the physiology, behaviour and growth of livestock, the research is being applied on the macronutrient requirements determination (Lihoreau et al. Citation2015). In particular, studies on protein, lipid and energy requirements and on the protein and carbohydrate ratio were carried out (Barragan-Fonseca et al. Citation2020; Georgescu et al. Citation2021; Bellezza Oddon et al. Citation2022a; Citation2022b). The protein requirement appeared to vary according to the larva age, in particular, early stages performed better with more protein than later ones (Bellezza Oddon et al. Citation2022a, Citation2022b). Considering the whole larval stage, the optimal protein percentage between 10, 14, 16 and 19 percentages on dry matter (DM) seemed to be the 16 (Bellezza Oddon et al. Citation2022a, Citation2022b). Protein percentage upper than 32% determined reduced growth performance and a survival rate lower than 22% (Tschirner & Simon Citation2015). On the contrary, high carbohydrates concentration (40–60% on DM) did not generate deleterious consequences on larval development (Barragan-Fonseca et al. Citation2020). Despite BSF larvae being able to manage the carbohydrates excess by physiological systems and convert them into fat reserve, formula lipid levels lower than or equal to 1% of EE on the substrate DM negatively affected the larvae weight and the development time (Bellezza Oddon et al. Citation2022a, Citation2022b, Cohn et al. Citation2022). The energy content of the substrate was influenced, logically, by the macronutrient levels, but the larvae weight tended to increase with the increasing of the energy value of the feed (Georgescu et al. Citation2021). On the basis of the requirements determined until now, the waste nutritional characterisation is necessary for a balanced formulation of the diet. In this perspective, a waste that may not be suitable if used as it is, can have a positive effect if combined with others. The study of Gold et al. (Citation2020), in which waste (mill by-products, canteen waste, human faeces, poultry slaughterhouse waste, cow manure and vegetable canteen waste) were tested individually or in a formulation, confirmed this assumption. The authors observed that the use of a waste-based formulation increases the performance when compared to a single waste (Gold et al. Citation2020). In addition to improve growth performance, the use of a formulation allows to reach the nutritional requirement ranges determined to date. In this way, uneven waste-based breeding substrates may become comparable from a nutritional point of view.
Based on the above reported background, the present study aims to evaluate the effect of 6 isoproteic, isolipidic and isoenergetic, waste balanced-formulation on larvae growth performance, pupae size and adult parameters.
Materials and methods
Colony status
For the trial larvae production, the BSF colony present at the Experimental Centre of the Department of Agriculture, Forest and Food Science (University of Turin), usually bred on Gainesville diet (Hogsette Citation1992) was used. The eggs hatching (oviposition period: 24h) was performed without substrate and larvae were collected every 24 h. Larvae, from one to six days old, were fed the GA (1 gram of 1-day-old larvae/1 kg of wet feed) and placed in plastic boxes (19 cm x 13 cm x 6 cm) covered by a lid with a nylon mosquito net.
Waste ingredients
The search for agri-food companies was carried out through the Italian Kompass database. A total of 21 waste were considered suitable from a compositional-nutritional point of view (Table ): (1) buffalo milk whey derived from the mozzarella production, (2) broken eggs derived from the egg’s selection, (3) liquid distillery stillage, (4) dry distillery stillage and (5) filtering waste derived from wheat starch processing, (6) brewer’s spent grains and (7) brewer’s spent yeast derived from brewing process, (8) sunflower oil and (9) chocolate derived from the cleaning of the chocolate machinery, (10) coffee silvery film and (11) cocoa husk obtained after roasting, (12) fruit juice and (13) pulp production waste (both the waste were used also in a mix form (14)), the sedimentation residuals of (15) vinegar and (16) glaze, (17) vegetable cleaning scraps, (18) oversized fruit (strawberry), (19) rice husk, (20) rice hulls and (21) green rice grain derived from the rice production. After the withdrawal from production sites, some wastes were furtherly processed in order to make them physically available for the larvae. In particular, the shells were removed from the eggs through a 4 cm mallet grid; vegetable, oversized fruit, chocolate, fruit juice and pulp were grinded by cutting mill (Fimar, CUCL823050M; Italy); and green rice grain and rice husk were grinded by hammer mill (Ceccato Olindo, M4; Italy). All the waste were analysed following the methods reported in the “Chemical analyses” section.
Table 1. Waste chemical composition (% on DM) and gross energy (MJ/kg DM).
Waste-based substrates
A total of 6 isoenergetic, isolipidic and isoproteic waste-based substrates were formulated (named from D1 to D6; Table ) using the chemical composition values of waste on DM. To facilitate the formulation of iso-nutrient diets, the ingredient inclusion and combination were chosen in relation to their chemical composition. Four of the tested diets (D1, D2, D3 and D5) were composed by two ingredients whose sum made up more than the 50% of the formulation (core diet), while the other (D4 and D6) were formulated using ingredients with a lower inclusion percentage (<23%). In particular, the core diets consist of (D1) 58.5% of brewer’s spent grains and vegetable waste, (D2) 63.3% of buffalo milk whey and glaze, (D3) 62.5% of vegetable and water and (D5) 51% of oversized fruits and mix of fruit juice and pulp waste. A sample of each diets was stored at −20 °C and used for the chemical analyses (Table ).
Table 2. Ingredients of the six (D1 – D6) waste-based diets.
Table 3. Chemical composition (% on DM) and gross energy (MJ/kg DM) of waste-based diets (D1-D6).
Chemical analysis
Before performing the chemical analysis, all the samples (waste-ingredients, diets and larvae) were stored at −20 °C and freeze-dried, with the exception of the waste with a DM over than 60% that were analysed as it. The larvae samples were ground (Retsch, GM 200) as frozen and freeze-dried. The DM (AOAC #934.01), the crude protein (CP; AOAC #984.13; conversion factor for ingredients and diets N x 6.25, for larvae N x 4.67) and the ash (AOAC #942.05) were determined by the International AOAC (Citation2000), and the ether extract (EE; AOAC #2003.05) by the International AOAC (Citation2003). The aNDFom-NDF assayed with a heat stable amylase and expressed exclusive of residual ash was determined by Mertens (Citation2002). The gross energy (GE) was determined using adiabatic calorimetric bomb (C7000; IKA, Staufen, Germany).
Larval and pupal stages
A climatic chamber under controlled condition (T°: 28 ± 0.5 °C; RH: 60 ± 5%) was used for the entire trial. To evaluate growth performance, only 6-day-old larvae that passed through 0.8 mm sieve were selected for the trial setup. The rearing boxes (14 × 14 x 7 cm) were filled with the substrates (one batch feeding, 1.8 g/larva) and placed in the climatic chamber for the temperature acclimatisation phase (1 h). After that, the homogeneous amount of obtained larvae was used to prepare 36 boxes of 100 larvae (6 replicates/treatment), which were manually counted in groups of 5 and weighed (standard deviation between measurements under 0.02) with a precision scale (Kern & Sohn GmbH; Balingen, Germany; d = 0.001). The larvae were inoculated in the substrates after the statistical analyses result, which confirmed absence of significant difference in terms of weight among the replicates. To avoid contamination and/or larvae escape, boxes were covered by a lid with nylon mosquito net. The climatic chamber environment, maintained in total dark, and the boxes were checked daily. Larvae average weight and substrate pH were determined every four days until the end of the stage, considered when 40% of the larvae reached the prepupae phase. In particular, 30 larvae per box were randomly selected, washed with warm tap water, dried with paper tissue and individually weighed. The pH was measured in duplicate angled away (HI 99161, Hanna Instruments volume precision 0.01). Considering that all the measures were not destructive, larvae were put back in the box. At the end of larval stage, according to Bosch et al. (Citation2020), the live biomass (as is) and the digested substrate (as is) were weighed to calculate the substrate reduction rate (RR, Formula 1), the waste reduction index (WRI, Formula 2) and the bioconversion efficiency corrected for residue (BER, Formula 3). The total number of live individuals per replicate was counted to assess the survival rate. The method used to sample larvae was also used to record the prepupae weight, which were placed back in their box after sampling in order to carry on their development. Moreover, the development time from 6-day-old larvae to prepupae (L-Pp, days) was recorded. Starting from the pupal stage, the photoperiod was changed to 12:12 LD. Boxes were checked once a day at 3 pm to identify the pupae presence, and, subsequently, the first 23 pupae per replicate were weighed and placed individually in a transparent plastic emergence box (circular, 8 cm of diameter, 4 cm height; EB) with a perforated plastic lid. The weight of the empty EB was noted to calculate the adult parameter. The emergence rate (ER) was determined by left untouched in their breeding boxes the remained prepupae/pupae to continue the life cycle until the fly death.
Formula 1
Formula 2
Formula 3
Descriptive chemical analyses
To obtain larvae samples for the descriptive chemical analysis, a second simultaneal trial was conducted in the same climatic chamber of the growth trial. A total of 3000 larvae per treatment were estimated (3 samples, variation coefficient under 10%) and bred in 23 × 30 × 9 cm boxes (2 replicate/treatment and 1500 larvae/replicate) covered by a lid with nylon mosquito net.
The feed ratio was maintained equal to the growth trial. The larval harvesting was done when the first prepupa appeared. Specifically, 100 g of larvae were washed with warm tap water, dried with paper tissue, collected and inactivated at −80 °C.
Adult stage
At the fly emergence, the EB containing the puparium and the fly (EBPF) was weighed to calculated the fly live weight (FLW; Formula 4), and the date was recorded to determine the time necessary for the adult development (pupa-fly; P-F). Once fly died, the date was recorded to calculate fly lifespan (FLS), the puparium (PW, g) and the dead fly (DFW, g) were weighed. The fly weight reduction (WR, %) during lifespan was determined using Formula 5. The overall fly ER (Formula 6) was calculated on the individuals remained in the breeding boxes.
Formula 4
Formula 4
Formula 5
Formula 5
Formula 6
Formula 6
Statistical analysis
Data was analysed using the IBM SPSS Statistics software (V20.0.0.). For the parameters recorded during the larval, prepupal and adult stages the statistical unit was the individual, with the exception of pH, WRI, BER, RR, survival rate and ER, in which was the replicate box. The assumptions of residuals normality and equal variances were determined using Shapiro-Wilk and Levene’s homogeneity of variance tests, respectively. The larvae weight data analysis was performed by generalised mixed model (GLMM) with a gamma probability distribution (nonlinear link function [log]), considering two fixed factors (diet/time, and their interaction) and replicate as random effect. The interactions between the levels of the fixed factors were evaluated by means of pairwise contrasts. Since in prepupal, pupal and adult stages the replicate was considered as random effect, an analogous GLMM was applied. The WRI, BER and ER were, instead, analysed by one-way ANOVA test (post-hoc test: Tukey). The correlation between substrate pH and larvae growth/L-Pp duration time was determined by Pearson Correlation Coefficient (PCC). The results were expressed as least square mean (larval, prepupal, pupal and adult parameters) or mean (bioconversion indices) and pooled standard error of the mean (SEM) and the level of significance considered was ≤ 0.05.
Results
Larval and pupal stage
As regard the overall larvae growth (Table ), the D1 and D5 showed the best results (p < 0.001), while D2 treatment performed worst (p < 0.001). The D3, D4 and D6 dietary treatments were statistically different from all the groups (p < 0.001). Considering the time effect on larvae growth, naturally, the weight increased over time (T1 and T2; p < 0.001). The interaction between diet and time on larvae performance at 10 day-old (T1) and 14 day-old (T2) is shown in Figure . At T1, D1 and D2 displayed the best and the worst weight (0.118 and 0.037 g; p < 0.001). No differences were observed between D3 and D5 (0.097 and 0.096 g; p > 0.05). The D4 and D6 diets were statistically different among the other groups (0.062 and 0.067 g; p < 0.001) At T2, all treatments were different from each other (p < 0.05). In particular, the larvae weights range was from 0.100 to 0.222 g, and diets can be ranked from the lowest to the highest weight as follows: D2, D6, D4, D3, D1 and D5 (0.100, 0.135, 0.162, 0.170, 0.176, 0.222 g, respectively; p < 0.001). At 18 day-old, D4 and D2 treatments had not ended the larval stage and the larva average weight was 0.161 g and 0.188 g, respectively.
Figure 1. Effect of time/diet interaction on larvae growth (T1, 10 day old; T2, 14 day old).

Table 4. Effect of the dietary treatment on overall larvae growth (grams; T1, 10 day old; T2, 14 day old).
Moreover, the larvae development was strongly, positively correlated to the substrate pH in both T1 (PCC = 0.871; p = 0.000) and T2 (PCC = 0.757; p = 0.000). The descriptive variation of the substrate pH during the sampling time is illustrated in Figure . At the beginning of the trial, all the pH values ranged between 3.5 and 4.5. At the end of larvae stage, the D1, D3, D5 and D6 had a basic or neutral transformation, while D2 and D4 remained acid.
In Table are illustrated the larva-prepupa (L-Pp) development time, the prepupae and pupae weight and the survival rate. The longest development time was recorded for D2 and D4, while D1, D3 and D5 reached fastest the prepupa stage (p < 0.001). The D6 treatment showed an intermediate result among the other diets (p > 0.05). The L-Pp duration time was negatively correlated to the substrate pH (PCC = −0.668; p = 0.000). The heaviest prepupae were found in D2 and D5 dietary treatments (p < 0.001), followed by D1 and D4 (p < 0.001) – which were equal to each other (p > 0.05). Lowest weight values were recorded in D3 and D6, with D3 performing better than D6 (p < 0.001). Regarding the survival rate, the only difference was observed between D5 and D2 percentages, with the first treatment being higher than the second (p < 0.05). Considering the pupae size, D2 and D5 led to the greatest weight (p < 0.001), whereas D6 group displayed the worst result (p < 0.001). No differences were observed among D1, D3 and D4 (p > 0.05).
Table 5. Larval stage end parameters and pupae weight.
Among the waste reduction and bioconversion indexes (Table ), only the WRI was not affected by the treatments (p > 0.05). The D2 diet showed the highest SR percentage (p < 0.001), while no differences were observed among the other groups (p > 0.05). As regards BER, D1 and D5 performed greater than D6 and D2 (p < 0.001), which were equal to each other (p > 0.05). The D3 and D4 dietary treatments displayed intermediate results (p > 0.05).
Table 6. Bio-conversion efficiency and waste reduction.
Descriptive larvae chemical composition
No statistical analysis was performed for larvae chemical composition. Therefore, reported results are only descriptive. As shown in Table , the descriptive larvae chemical composition (% on DM) varies among the diets. Considering the DM content, all the dietary treatments displayed numerically lower values than the larvae composition at the beginning of the trial (T0), with the exception of D2. In D1, D5 and D6, the ash percentage was numerically similar to T0, while in D2 and D3 was minor. The CP amount increased overtime, except for D2. Finally, the EE content was numerically higher in all the treatments at the end of the trial than T0, and it showed a great variability (from 9.75 to 37.37% of EE on DM).
Table 7. Descriptive larvae chemical composition (expressed as percentage on DM) at the beginning (T0) and at the end of the trial.
Adult stage
The adult stage parameters are illustrated in Table . Every variable was affected by the dietary treatment, with the exception of ER (p > 0.05). The FLW was highest in D2 and D5, and all the other treatments were statistically different from each other (p < 0.001), apart from D3 that was equal to D1 and D4 (p > 0.05). Lower percentage of WR were recorded for D2, D3 and D4 when compared to D1, D5 and D6 (p < 0.001). As regard the FLS, the only difference was observed between D2 and the other diets (p < 0.001). The longest P-F development time was recorded for D2, while the shortest for D1, D3 and D6 (p < 0.001). The D4 and D5 P-F time was equal (p > 0.05). Finally, the PW range was from 0.010 to 0.018 g, diets, which were all different to each other (p < 0.001), are ranked from lowest to highest weight as follows: D6, D4, D3, D1, D2 and D5.
Table 8. Adult stage parameters.
Discussion
Larval stage
Despite the comparability among the diets in terms of nutrients content, the results obtained on larvae growth and development time were variable. Since the survival rate was greater than or equal to 92%, it is possible to state that all the dietary treatments were suitable for the BSF larvae breeding. Regarding the overall larvae growth, D1 and D5 led to the greatest values. Their core ingredients (brewer’s spent grains and vegetable waste, oversized fruits and mix of fruit juice and pulp waste, respectively) are known as good substrates for the BSF breeding (Fischer and Romano Citation2021).
The other diets were characterised by lower results than D1 and D5, and were all statistically different. From a nutritional point of view, the only variable among the diets was the ash percentage, which was higher in D2 (+23.5%), D3 (+32.6%), D4 (+63.1%) and D6 (+67.4%) when reported to D1 and D5. This ash content variation may be due to the filtering waste, made up of 30% ash on DM. With the exception of D2, which showed worse results likely caused by factors that will be discussed later, the growth performance declined as the ash in the substrate increased. Since higher levels of minerals than the one of the present trial did not adversely affect growth in other studies, the cause may be due to the qualitative composition (Kraus et al. Citation2019; Purkayastha and Sarkar Citation2021). For example, the substrate palatability is influenced by the mineral salts and, usually, animals reject high concentrations (de Sousa et al. Citation2022). The Drosophila larvae regulate the feed ingestion in relation to the Na and Ca presence (Zhang et al. Citation2013; Lee et al. Citation2018). Although the macro-mineral composition of the larvae is in some cases known, to the authors’ knowledge there are no studies on the evaluation of the mineral requirement and preference in BSF larvae (Oonincx and Finke Citation2021). The D2 showed a completely different development than the other groups, and this outcome may be explained by two factors and their interaction. Despite the DM content was similar in all the diets, the D2 physical texture was unsuitable due to the supernatant presence. Since the dry ingredients are almost the same of the other formulation, it is improbable their reduced water holding capacity. Consequently, the two main influences can be related to the waste interaction and/or the pH of the substrate. The waste interaction may have determined the supernatant. Since the D2 core ingredients were the buffalo milk whey and the glaze waste, the by-products derived from the main actors of acid coagulation for the cheese production, they probably create a solid-liquid separation. The acid coagulation process occurs at low pH, caused by glaze waste, in the presence of milk proteins, derived from buffalo milk whey, and is dependent on temperature (25 °C or more), normal condition of the breeding substrate of the larvae BSF (Troch et al. Citation2017). The presence of free water results in a decrease in the time spent by the larva in the substrate and, therefore, in a lower feed intake and in an increased use of energy because of the search of a drier place. The pH of the substrate, on the other hand, was the only one that did not increase over time and remained at 3.6. Regarding the effect of the pH on BSF larvae growth, Meneguz et al. (Citation2018) observed negative influence of pH 4 when compared with highest values until 9 days of larvae age.
Going into details in the two-sampling time, D1 and D5 performed the greatest results at 10 and 14 days old, respectively. At 10 days old, the trend of diets 2, 3, 4 and 6 was comparable to the overall larvae growth, with the exception of D3 that displayed an average weight statistically equal to D5. At 14 days old, the growth of the D3 and D5 larvae had differentiated, with the D3 showing a lower weight when compared to D5. This result can be attributed to the particle size of the substrates. The D5 was composed by silvery film, which is a very thin waste, and in presence of the diet humidity and larvae activity was disintegrated over the time, thus may becoming, as a consequence, a secondary nutrient source for the larvae.
The D1 larvae weight at 14 days old was higher (0.176 g) when compared to the one bred by Fischer and Romano (Citation2021) (0.120–0.130 g; two weeks of trial) on vegetable waste, while it was lower than the larvae (0.260 g; 13 days old) fed brewer’s spent grains observed by Beesigamukama et al. (Citation2020). The chemical composition of vegetable waste and brewer’s spent grains is dissimilar, and, generally, vegetables are the poorest in nutrients. This nutritional diversity is, logically, reflected on the larvae performance. The vegetable waste yield in larval biomass can, therefore, be improved through an appropriate formulation of the substrate. As suggested by Gold et al. (Citation2020), indeed, a reasoned mixture of waste increases the growth and reduces the variability of BSF larvae. For this reason, the intermediate outcome obtained in the present study can be explained by the mixture of poor and rich waste, as vegetable and spent brewer’s spent grains.
The prepupae size of D1, D3, D5 and D6 groups followed the tendency of the T2 larvae sampling, while D2 and D4 groups improved, considering also their weight at 18 days old, their results. In particular, the weight of D2 were equal to D5 and D4 to D1. However, both the D2 and the D4 prepupae spent more time as larvae in the substrate and, for that reason, accumulated slowly the nutrient reserve.
As regards the L-Pp duration time, the pH influenced the development time. In particular, larvae grown on low pH took longer to reach the last instar of development (D2 and D4). The same outcome was observed by Ma et al. (Citation2018) with an initial pH lower than 4. Since from the prepupae instar start the non-feeding phase and the weight trend to decrease with the lipid consumption (Liu et al. Citation2017), the size of the pupae is reduced when compared to the prepupae. In according with Georgescu et al. (Citation2021), the statistical differences of the pupae weight were almost the same of the prepupae, with the exception of D3. In particular, D3 became comparable to D1 and D4 that displayed the highest results in terms of prepupae size. This interesting condition means that, for unknown reasons, D3 individuals lost less weight during the non-feeding phase.
The bioconversion indexes reflected, logically, the larvae growth performance. Since the D2 took longest time to reach the prepupae phase and, as reported above, the substrate contained a visible supernatant, it is possible to hypothesise a greater water evaporation and, consequently, a higher SR compared to the other diets. Moreover, thanks to the good SR of D2, no differences were found on the WRI. The WRI of the diets tested in the present study was lower when compared to the results obtained by Addeo et al. (Citation2021) with BSF larvae developed on vegetable and butchery waste. Generally, high WRI values represented the greatest reduction efficiency (Diener et al. Citation2009). The difference of WRI between the two studies may be caused by the reduced feeding rate used in Addeo et al. (Citation2021) trial (1.6 g/larva) when compared to the present study (1.8 g/larva). According to Yuan and Hasan (Citation2022), the optimal feeding rate in term of WRI and growth performance changes in relation to the substrate used, but usually the WRI tends to decrease with the increasing of the feeding rate. Since the nominator of the BER index formula is the larvae weight gain, D1 and D5 displayed the greatest results, while D2 and D6 the worst. The D1, D3, D4 and D5 are within the range of bioconversion efficiency rate of food, canteen, vegetable, abattoir waste, while D2 and D6 values are slightly higher than manure substrate (Yuan and Hasan Citation2022). Considering that the waste conversion efficiency is influenced by several factors such as larval density, feeding rate, BSF strain and nutritional value of the substrate, the comparation among studies is complex and, in some cases, unreliable (Bosch et al. Citation2020; Gold et al. Citation2020; Surendra et al. Citation2020). Furthermore, despite the nutritional uniformity of diets, other factors affected the larvae performance. As a result, it is possible to hypothesise that, in addition to the variables already analysed, also the digestible nutrient component may interfere with the outcome.
Descriptive larvae chemical composition
The larvae chemical composition at the beginning of the trial was different from those analysed by Liu et al. (Citation2017) at the same age (6 day old), in which the protein and lipid percentages were higher (52.2% and 9.6%, respectively). Since the larvae composition is extremely linked to the nutrient amount in the substrate (Hopkins et al. Citation2021), it is possible to ascribe the outcome to the use of GA (present study) and chicken feed (Liu et al. Citation2017) as growing substrate. At the end of the trial, the larvae composition varies among the treatments. As regards the CP, all the dietary treatments are in line with the range that usually is observed (30–51% on DM) (Surendra et al. Citation2020). As reported by Liu et al. (Citation2017), in almost all the treatments the protein content increased overtime (from T0 to the larval stage end), with the exception of the D2. On the other side, the EE percentage was lower in D1, D3, D5 and D6 when compared to the conventional range (21-40% on DM) (Surendra et al. Citation2020) and, also in this case, increased with the larvae growth as reported by Liu et al. (Citation2017). Considering the ash content, it is possible to exclude an accumulation process because all dietary treatments with the lowest percentages generated larvae with the highest amount. Considering the nutritional comparability of the diets, larvae ash composition may be attributed to the micronutrient quality and their absorption. Despite the diet were comparable from a macronutrient point of view, it is possible to speculate that the differences in terms of ingredients and, consequently nutrient quality, affected the chemical composition of the larvae. In particular, the diet in which larvae fed on influences the nutrient metabolisation and digestibility because the enzymes are excreted in relation to the macronutrient present in the gut (Espinoza-Fuentes and Terra Citation1987). Moreover, the microbes that are ingested changed in relation to the substrate and they have, when occur in the intestine, multiple function that impact on the larvae development (Hövemeyer Citation2012; Gold et al. Citation2018). The breakdown of the ingested substrate into nutritional units is, therefore, a complex mechanism that is partially known and determines the larvae final composition.
Adult stage
All the dietary treatments allowed to reach the adult stage with a high ER, but the diets affected the other flies’ parameters. As consequence of pupal phase, D2 and D5 performed the greatest FLW and D6 the worst. On the contrary, D1 and D4, which were comparable during the pupal stage, generated flies with different dimensions, while D3 was intermediate. Considering that studies on Muscidae demonstrated that small adults derived from a reduced quality of the substrate ingested by the larvae, it is possible to hypothesise a different availability of nutrients in those diets (Black and Krafsur Citation1987).
The WR of the flies differentiated between the best diets (D2 and D5). In particular, D2 displayed lower weight losses than D5, which was equal to the worst treatment (D6). Georgescu et al. (Citation2021) reported that the energy invested during the non-feeding phase and reproduction can change. The D2 losses between the prepupae and pupae stage were equal to 11.77%, while D5 and D6 were 9.20% and 8.74%, respectively. Therefore, it is possible to observe a different energy reserve use in relation to the growth substrate that probably may be linked to the qualitative aspect of the stored nutrient. As regards the FLS, D6 survived less than the others group given its low weight and its high WR percentage. Considering the P-F duration time, the dietary treatment that performed different FLW emerged at the same time. For this reason, it is possible to exclude an effect of the weight on the time spent for the adult development. It is known that the diet type consumed by the larvae affect the pupae development (Gobbi et al. Citation2013; Flores et al. Citation2014). Since Flores et al. (Citation2014) noted that the Chrysomya rufifacies (Diptera: Calliphoridae) P-F duration period was dependent on the type of animal muscle used as substrate, it is possible to suppose that the diet effect on the life history traits derived from the nutritional quality more than the quantity. In the case of D2 individuals, the puparium weight was not related to the weight of the flies as mentioned by Bellezza Oddon et al. (Citation2022a,Citation2022b): specifically, despite the FLW being equal to D5, the PW was lower. Therefore, the yield of the fly on the total weight was greater than D5. This outcome may derive from a longer feeding phase in the larval stage and to a bigger storage of the fat sources. Since diets have also influenced aspects of the adult stage, future research is needed to evaluate the effects of larval feeding on the later stages of the life of BSF in order to improve the reproduction sector.
Conclusions
To the authors knowledge, the current study is the first that aims to compare waste-based diets with equal nutritional levels. The results herein obtained allowed to discriminate the nutritional variable and observe other influences on the life history traits of the BSF. Given the formulations complexity and the lack of explanation of BSF physiology, metabolism and digestibility, it is difficult, in some cases, to give objective explanations of results. For this reason, further studies on quantitative and qualitative nutritional requirements, substrate texture and digestibility will be necessary to create a clearer scientific framework and facilitate the basic-choice of a farm: the breeding substrate.
Ethical approval and consent to participate
Not applicable.
Authors’ contributions
SBO, IB and LG conceived and designed the experiment. SBO, IB, AR and LG performed the trial and collected the experimental data. SBO and IB analysed the data. SBO carried out the chemical analyses. SBO wrote the first draft of the manuscript. All the authors critically reviewed the manuscript for its intellectual content and gave their approval for the final version to be published.
Disclosure statement
The authors declare that they have no competing interests.
Data availability statement
The datasets used and/or analysed during the current study are available from the corresponding author on reasonable request.
Additional information
Funding
References
- Addeo NF, Vozzo S, Secci G, Mastellone V, Piccolo G, Lombardi P, Parisi G, Khalid AA, Youssef AA, Bovera F. 2021. Different combinations of butchery and vegetable wastes on growth performance, chemical-nutritional characteristics and oxidative status of black soldier fly growing larvae. Animals (Basel). 11(12):3515. doi: 10.3390/ani11123515.
- AOAC International. 2000. Official methods of analysis of AOAC International. 17th ed. Gaithersburg: Association of Official Analytical Chemists.
- AOAC International. 2003. Official methods of analysis of AOAC International. 17th ed.; 2nd revision. Gaithersburg: Association of Official Analytical Chemists.
- Barragan-Fonseca KB, Gort G, Dicke M, van Loon JJA. 2020. Nutritional plasticity of the black soldier fly (Hermetia illucens) in response to artificial diets varying in protein and carbohydrate concentrations. J Insects Food Feed. (7):5–6. doi: 10.3920/JIFF2020.0034.
- Beesigamukama D, Mochoge B, Korir NK, K M Fiaboe K, Nakimbugwe D, Khamis FM, Subramanian S, Wangu MM, Dubois T, Ekesi S, et al. 2020. Low-cost technology for recycling agro-industrial waste into nutrient-rich organic fertilizer using black soldier fly. Waste Manag. 119:183–194. (): doi: 10.1016/j.wasman.2020.09.043.
- Bellezza Oddon S, Biasato I, Gasco L. 2022a. Isoenergetic-practical and semi-purified diets for protein requirement determination in Hermetia illucens larvae: consequences on life history traits. J Anim Sci Biotechnol. 13(1):17. doi: 10.1186/s40104-021-00659-y.
- Bellezza Oddon S, Biasato I, Resconi A, Gasco L. 2022b. Determination of lipid requirements in black soldier fly through semi-purified diets. Sci Rep. 12(1):10922. doi: 10.1038/s41598-022-14290-y.
- Black IVWB, Krafsur ES. 1987. Fecundity and size in the housefly: investigations of some environmental sources and genetic correlates of variation. Med Vet Entomol. 1(4):369–382.): doi: 10.1111/j.1365-2915.1987.tb00368.x.
- Bosch G, Oonincx DGAB, Jordan HR, Zhang J, van Loon JJA, van Huis A, Tomberlin JK. 2020. Standardisation of quantitative resource conversion studies with black soldier fly larvae. JIFF. 6(2):95–109.): doi: 10.3920/JIFF2019.0004.
- Chia SY, Tanga CM, Osuga IM, Mohamed SA, Khamis FM, Salifu D, Sevgan S, Fiaboe KKM, Niassy A, van Loon JJA, et al. 2018. Effects of waste stream combinations from brewing industry on performance of black soldier fly, Hermetia illucens (Diptera: Stratiomyidae). PeerJ. 6:e5885. () doi: 10.7717/peerj.5885.
- Cohn Z, Latty T, Abbas A. 2022. Understanding dietary carbohydrates in black soldier fly larvae treatment of organic waste in the circular economy. Waste Manag. (137)::9–19. doi: 10.1016/j.wasman.2021.10.013.
- de Sousa RT, Darnell R, Wright GA. 2022. Behavioural regulation of mineral salt intake in honeybees: a self-selection approach. Philos Trans R Soc Lond B Biol Sci. 377(1853):20210169.): doi: 10.1098/rstb.2021.0169.
- Diener S, Zurbrügg C, Tockner K. 2009. Conversion of organic material by black soldier fly larvae: establishing optimal feeding rates. Waste Manag Res. 27(6):603–610.): doi: 10.1177/0734242X09103838.
- Espinoza-Fuentes FP, Terra WR. 1987. Physiological adaptations for digesting bacteria. Water fluxes and distribution of digestive enzymes in Musca domestica larval midgut. Insect Biochem. 17(6):809–817.): doi: 10.1016/0020-1790(87)90015-1.
- Fischer H, Romano N. 2021. Fruit, vegetable, and starch mixtures on the nutritional quality of black soldier fly (Hermetia illucens) larvae and resulting frass. J Insects Food Feed. (7):319–327. doi: 10.3390/insects12040332.
- Flores M, Longnecker M, Tomberlin JK. 2014. Effects of temperature and tissue type on Chrysomya rufifacies (Diptera: Calliphoridae) (Macquart) development. Forensic Sci Int. (245)::24–29. doi: 10.1016/j.forsciint.2014.09.023.
- Georgescu B, Struți D, Păpuc T, Cighi V, Boaru A. 2021. Effect of the energy content of diets on the development and quality of the fat reserves of larvae and reproduction of adults of the black soldier fly, Hermetia illucens (Diptera: Stratiomyidae). Eur J Entomol. (118)::297–306. doi: 10.14411/eje.2021.030.
- Gobbi P, Martínez-Sánchez A, Rojo S. 2013. The effects of larval diet on adult life-history traits of the black soldier fly, Hermetia illucens (Diptera: Stratiomyidae). Eur J Entomol. 110(3):461–468.). doi: 10.14411/eje.2013.061.
- Gold M, Cassar CM, Zurbrügg C, Kreuzer M, Boulos S, Diener S, Mathys A. 2020. Biowaste treatment with black soldier fly larvae: increasing performance through the formulation of biowastes based on protein and carbohydrates. Waste Manag. (102):319–329. doi: 10.1016/j.wasman.2019.10.036.
- Gold M, Tomberlin JK, Diener S, Zurbrügg C, Mathys A. 2018. Decomposition of biowaste macronutrients, microbes, and chemicals in black soldier fly larval treatment: a review. Waste Manag. (82):302–318. doi: 10.1016/j.wasman.2018.10.022.
- Hogsette JA. 1992. New diets for production of house flies and stable flies (Diptera: Muscidae) in the laboratory. J Econ Entomol. 85(6):2291–2294. doi: 10.1093/jee/85.6.2291.
- Hopkins I, Newman LP, Gill H, Danaher J. 2021. The influence of food waste rearing substrates on black soldier fly larvae protein composition: a systematic review. Insects. 12(7):608.). doi: 10.3390/insects12070608.
- Hövemeyer K. 2012. The Symbiotic Habit. Princeton: Princeton University Press.
- Ingrao C, Faccilongo N, Di Gioia L, Messineo A. 2018. Food waste recovery into energy in a circular economy perspective: a comprehensive review of aspects related to plant operation and environmental assessment. J. Clean. Prod. (184)::869–892. doi: 10.1016/j.jclepro.2018.02.267.
- Kim CH, Ryu J, Lee J, Ko K, Lee JY, Park KY, Chung H. 2021. Use of black soldier fly larvae for food waste treatment and energy production in Asian countries: a review. Processes. 9(1):161. doi: 10.3390/pr9010161.
- Kraus S, Monchanin C, Gomez-Moracho T, Lihoreau M. 2019. Insect diet. In Encyclopedia of animal cognition and behavior. Swiss: Springer International Publishing.
- Lee Y, Poudel S, Kim Y, Thakur D, Montell C. 2018. Calcium taste avoidance in Drosophila. Neuron. 97(1):67–74.e4.): doi: 10.1016/j.neuron.2017.11.038.
- Lihoreau M, Buhl J, Charleston MA, Sword GA, Raubenheimer D, Simpson SJ. 2015. Nutritional ecology beyond the individual: a conceptual framework for integrating nutrition and social interactions. Ecol Lett. 18(3):273–286. doi: 10.1111/ele.12406.
- Liu X, Chen X, Wang H, Yang Q, Ur Rehman K, Li W, Minmin C, Li Q, Mazza L, Zhang J, et al. 2017. Dynamic changes of nutrient composition throughout the entire life cycle of black soldier fly. PLOS One. 12(8):e0182601. doi: 10.1371/journal.pone.0182601.
- Liu C, Wang C, Yao H, Chapman SJ. 2021. Pretreatment is an important method for increasing the conversion efficiency of rice straw by black soldier fly larvae based on the function of gut microorganisms. Sci Total Environ. (762):144118. doi: 10.1016/j.scitotenv.2020.144118.
- Ma J, Lei Y, Ur Rehman K, Yu Z, Zhang J, Li W, Li Q, Tomberlin JK, Zheng L. 2018. Dynamic Effects of initial pH of substrate on biological growth and metamorphosis of black soldier fly (Diptera: Stratiomyidae). Environ Entomol. 47(1):159–165.): doi: 10.1093/ee/nvx186.
- Manurung R, Supriatna A, Esyanthi RR, Putra RE. 2016. Bioconversion of rice straw waste by black soldier fly larvae (Hermetia illucens L.): optimal feed rate for biomass production. J Entomol. Zool. Stud. 4(4):1036–1041.
- Meneguz M, Gasco L, Tomberlin JK. 2018. Impact of pH and feeding system on black soldier fly (Hermetia illucens, L; Diptera: Stratiomyidae) larval development. PLoS One. 13(8):e0202591.). doi: 10.1371/journal.pone.0202591.
- Mertens DR. 2002. Gravimetric determination of amylase-treated neutral detergent fiber in feeds with refluxing in beakers or crucibles: collaborative Study. J AOAC Int. 85(6):1217–1240.): doi: 10.1093/jaoac/85.6.1217.
- Oonincx DGAB, Finke MD. 2021. Nutritional value of insects and ways to manipulate their composition. JIFF. 7(5):639–659. doi: 10.3920/JIFF2020.0050.
- Purkayastha D, Sarkar S. 2021. Sustainable waste management using black soldier fly larva: a review. International J. Environ. Sci. Technol. (1). 19:12701–26. doi: 10.1007/s13762-021-03524-7.
- Siddiqui SA, Ristow B, Rahayu T, Putra NS, Widya Yuwono N, Nisa’ K, Mategeko B, Smetana S, Saki M, Nawaz A, et al. 2022. Black soldier fly larvae (BSFL) and their affinity for organic waste processing. Waste Manag. 140:1–13. (): doi: 10.1016/j.wasman.2021.12.044.
- Surendra KC, Tomberlin JK, van Huis A, Cammack JA, Heckmann LHL, Khanal SK. 2020. Rethinking organic wastes bioconversion: evaluating the potential of the black soldier fly (Hermetia illucens (L.))(Diptera: Stratiomyidae)(BSF). Waste Manag. (117);:58–80. doi: 10.1016/j.wasman.2020.07.050.
- Troch T, Lefébure É, Baeten V, Colinet F, Gengler N, Sindic M. 2017. Cow milk coagulation: process description, variation factors and evaluation methodologies. A review. Biotechnol Agron Soc Environ. 21:276–287. doi: 10.25518/1780-4507.13692.
- Tschirner M, Simon A. 2015. Influence of different growing substrates and processing on the nutrient composition of black soldier fly larvae destined for animal feed. JIFF. 1(4):249–259. doi: 10.3920/JIFF2014.0008.
- Yuan MC, Hasan HA. 2022. Effect of feeding rate on growth performance and waste reduction efficiency of black soldier fly larvae (Diptera: Stratiomyidae). Trop Life Sci Res. 33(1):179–199. doi: 10.21315/tlsr2022.33.1.11.
- Zhang YV, Ni J, Montell C. 2013. The molecular basis for attractive salt-taste coding in drosophila. Science. 340(6138):1334–1338. doi: 10.1126/science.1234133.