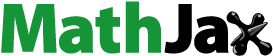
Abstract
A smooth transition phase is the key for optimal dairy cows’ performance and reduced antibiotics use. Therefore, the objective of our Our study was to compare the effect of an antibiotic growth promoter and a nutraceutical bolus, onhealth, colostrum, milk production, and profitability in transition dairy cows. Seventy-five animals blocked by parity, previous milk yield, lactation length, and body condition score (BCS) were assigned in a randomised design to 1 of 3 groups: control (CON; N = 26) receiving no treatment; monensin (MON; N = 27) receiving, a slow-releasing intraruminal bolus of sodic monensin at −20 d relative to expected calving; nutraceutical (ECS; N = 22) receiving, at −5 d before the expected calving an intraruminal bolus of Echinacea purpurea dry extract, vitamin E, l-carnitine and Silybum marianum released within 24h. Colostrum yield and density were recorded; its composition and immunoglobulins content were analysed. Every 20 days BCS was recorded; individual milk yield was recorded daily until 70DIM and monthly thereafter until 305 DIM. Milk quality was tested monthly. An economic evaluation until 70DIM was performed. Colostrum and BCS were analysed by analysis of covariance. Economics and BCS were analysed with ANOVA, and milk yield and quality with ANOVA for repeated measures. The MON and ECS had lower colostrum protein and His, Arg, Ala, Met, Val and Ile content, and in higher milk yield until 35 (ECS) and 70 (MON) DIM compared to the CON. A trend for a higher partial income was observed for MON and ECS compared to the CON group, suggesting a positive impact of the treatments.
HIGHLIGHTS
Monensin and nutraceutical boluses alter colostrum composition and increase milk yield.
Nutraceutical bolus is a promising alternative.
Introduction
In transition cows, negative energy balance is one of the main drivers for diseases and/or reduced production affecting metabolism and immune function, along with other stressors associated with parturition and lactations adaptations (Esposito et al. Citation2020; Mezzetti et al. Citation2021). Furthermore, several cow and management-related factors, such as parity, pre-partum nutrition and dry period length can impair colostrum composition (McCarthy, Yasui, Ryan, Pelton, et al. Citation2015) and, indirectly affect the passive transfer of immunity and offspring health (Abuelo et al. Citation2019; Cardoso et al. Citation2021). Therefore, several strategies have been investigated to address these challenges.
Among these, nutrients active on intermediate metabolism, such as rumen protected polyunsaturated fatty acids (n-3 and n-6), have been reported to increase immune function by exerting pro- or anti-inflammatory actions (Lessard et al. Citation2004; Esposito et al. Citation2014). Moreover, different dietary protein levels and nutrients supplementation (choline and methionine) have been suggested to improve the transition period by reducing both, β-hydroxybutyrate (BHBA) levels and fatty liver incidence (Cadórniga-Valiño et al. Citation1997; Coleman et al. Citation2019) and affecting immune cell function (Batistel et al. Citation2017; Zhou et al. Citation2018).
Furthermore, antibiotic growth promoters have been used to shift rumen fermentation towards more efficient capture of energy and nitrogen (Hungate Citation1975), therefore reducing the effect of imbalanced energy metabolism and, consequently, enhancing productivity in cattle diets (Duffield et al. Citation2008). In Europe, since 2013, the antimicrobial rumen modifier monensin (a carboxylic polyether ionophore) has been approved, under veterinary prescription, for the use as controlled-release capsule in dairy cattle to prevent postpartum diseases (mainly ketosis) in high-producing animals (European Medicine Agency Citation2013). This is because monensin increases propionate production in the rumen, with consequent reduced blood BHBA concentration, improved liver function and reduced incidence of peripartum diseases (Mammi et al. Citation2021). However, the overall heightened concern on the use of antibiotics in livestock has led the research towards the investigation of nutraceutical alternatives to modulate energy metabolism. Among these, live yeast and yeast-based products, have been shown to modulate rumen and metabolic response -exerting some activity also on mucosal and systemic immunity- and, consequently, cattle performance (Lopreiato et al. Citation2020).
Lastly, phytochemicals as feed additives, can be potentially used as antioxidants, antimicrobials (Hammer et al. Citation1999), modulators of appetite, rumen fermentation and digestive activities (Yoruk and Marshall Citation2003; Wallace Citation2004). They interact with the immune, endocrine and metabolic systems, potentially enhancing animal performance (Lopreiato et al. Citation2020).
A commercial nutraceutical bolus containing herbal extracts (Echinacea purpurea, Silibum marianum) and synthetic molecules (carnitine and vitamin E), has been developed as a preventive tool against transition cows’ diseases. This product is intended to act as intermediate metabolism enhancer through: (i) immunomodulatory effect of the Echinacea purpurea (Seckin et al. Citation2018; McNeil et al. Citation2023); (ii) hepatoprotective function of the silymarin extracted from Silybum marianum (Hermenean et al. Citation2015; Ulger et al. Citation2017); (iii) antioxidant action of the vitamin E (Haga et al. Citation2021; Xiao et al. Citation2021), which reduces the incidence of mastitis and reproductive diseases and also modifies colostrum and milk fatty acids profile (Weiss Citation2017) and (iv) energy metabolism facilitating Carnitine, which contributes in preventing fatty liver during negative energy balance in dairy cows (Ringseis et al. Citation2018).
Therefore, our hypothesis was that the ECS bolus could have improved the energy utilisation during the transition and early lactation periods by supporting the intermediate metabolism and the immune system, thus acting as alternative to a glucogenic, antibiotic-based rumen environment modifier. Hence, the objective of this study was to assess the effect of monensin or a nutraceutical bolus administration, in transition dairy cows, on colostrum yield composition, milk yield and quality, health status and reproductive performance.
Materials and methods
The study complied with the Italian laws on animal experimentation and ethics (DL 04/03/2014 n. 26).
Products tested
The following products have been tested:
Monesin (Kexxtone, Elanco Italia Animal Health, Sesto Fiorentino, Florence, Italy), a carboxylic polyether ionophore, delivered as a controlled-release capsule. Each capsule contains 12 subunits, each containing 2.7 g of monensin (equivalent to 2.9 g monensin sodium), thus supplying an average of 335 mg/d of the active compound for 95 d in the rumen environment.
Commercial nutraceutical bolus comprised of 10 g of vitamin E, 5 g of Echinacea purpurea dry extract, 5 g of l-carnitine and 5 g of silymarin (Silybum marianum) released in the rumen between 20 and 24h after administration w (PRI-X, Biotrade, Malavasi Claudio & C. SNC, Mirandola, Modena, Italy). The bolus ’components are registered as category 2b (Echinacea purpurea and Silybum marianum) and 3a (l-carnitine and vitamin E) in the Annexe I of the Register of Feed Additives (Regulation (EC) 1831/2003).
Experimental design, animals and diets
The trial was conducted from October 2018 to May 2019 in a commercial dairy herd located in northern Italy (45°11'27.0"N 10°00'16.1"E).
Seventy-five Italian Holstein dairy cattle, housed in a free stall pen, were blocked by parity (3.271.26), previous milk yield (31.04
4.24 kg/d), previous lactation length (357.97
58.17 days in milk - DIM) and body condition score (BCS; 3.42
0.35), and assigned, in a randomised design, to one of the following treatments: 1. control (CON; N = 26 cows; parity: 3.12
1.11; previous milk yield: 30.65
3.92 kg/d; previous lactation length: 358.46
51.67 DIM; BCS: 3.37
0.27), receiving no treatment; 2. monensin (MON; N = 27 cows; parity: 3.56
1.16; previous milk yield: 31.48
5.14 kg/d; previous lactation length: 369.96
77.16 DIM; BCS: 3.43
0.36), receiving a bolus of monensin, as a controlled release capsule, 3 wk before the expected calving date as per suggested protocol; 3. nutraceutic (ECS; N = 22 cows; parity: 3.10
1.25; previous milk yield: 30.76
4.57 kg/d; previous lactation length: 351.65
62.08 DIM; BCS: 3.40
0.43), receiving an intra-ruminal nutraceutical bolus 5 d before the expected calving date in order to make its components available to the cows around parturition. The animals, housed in the same pen, were observed from 20 d before the expected calving date (−20 d) until 305 lactating days, and they all received the same dry and lactating total mixed rations (TMR). The TMR, formulated to meet 100% of the NRC requirements, was delivered once daily at 7:00 am and pushed-up in the feed bunk twice daily at 12:00 pm and 18:00 pm. From drying off to the calving day, cows were fed a dry diet (Table ). After calving, cows were individually housed in a calving pen with permanent straw bedding for 24h. Here colostrum was milked twice, with the first milking within 6h after parturition and the second within 12h from the first. Following, the animals were moved to the post-partum pen and milked twice daily at 3.30 h and 14.30 h. Here, the animals were allowed to gradually adjust to the “fresh cow” diet which was offered at 75% of the formulated ration together with ad libitum hay until 10 DIM. From 11 DIM the animals were moved in the fresh cows’ pen, provided with sand-bedded stalls and fed the fresh cow diet (105% of the “fresh cow” TMR to ensure at least 5% of refusals) until 100 DIM. From 101 to 180 DIM, the cows were transferred to the lactation group where they were fed 105% of the lactating diet and to the late lactation group until the dry off period where they were fed the late lactation diet in the same proportion. Diets and their chemical composition are reported in Table .
Table 1. Ingredient and chemical composition of dry and lactating diets.
Measurements, sampling and data collection
All the TMR were collected monthly, whereas the forages were sampled at any stock change. The samples were labelled and stored at −20° C until analysed.
Individual colostrum yield was measured as the sum of the first and second milking produced within the first 24h post-partum. Moreover, colostrum quality was measured through the refractive index (BRIX degrees - °Bx), assessed on the first and second milking, with an ATC refractometer (Gebruiksaanwijzing Brix Refractometer-Heberex, Enschede), having a working range of 0 to 35°Bx. From each cow, a sample of colostrum (200 mL) from the first milking was collected and stored at − 20 °C prior to freeze-drying for further analyses.
Daily milk yield was measured for the first 70 DIM through the lactometer installed in the milking parlour (EnneEffe Boumatic, Cremona) and recorded electronically through the Herd Metrix software (Smart Dairy management system). Moreover, official test-day recording milk yield and composition (fat, protein and somatic cell count - SCC) were obtained until 305 DIM. Monthly milk yield was corrected for energy content (energy corrected milk - ECM) as described by Tyrrell and Reid (Citation1965).
The animals’ BCS was recorded every 20 d from −20 d to 80 DIM. Animals were scored according to the scoring system described by Edmonson et al. (Citation1989), by two different operators blinded to the treatments, and their average was recorded. The concentration of BHBA in blood was tested by a specialised veterinary practitioner as a farm routine analysis at 5 DIM (according to McArt et al. Citation2012). Briefly, a single drop of blood was collected from the ear capillary of each animal and analysed using a test strip in a handheld device (GlucoMen Lx PLUS+, A. Menarini diagnostic, Firenze).
Information regarding the prevalence of diseases and the therapeutic approaches used were recorded. Finally, reproductive performance, measured as calving to first service, calving to pregnancy and number of inseminations per pregnancy were recorded using the Cincinnato software (Italservice S.r.l. Cremona, Italy).
The economics of the experimental treatments was evaluated for the first 70DIM. For each cow, based on the milk yield (MY), the dry matter intake (DMI) was predicted according to Allen et al. (Citation2019) using the following equation:
Where:
NDF = neutral detergent fibre
ADF = acid detergent fibre
fNDF = forage NDF
fNDFD = forage NDF digestibility
Furthermore, the following costs were considered: cost of the diet from 0 to 70 DIM (€0.22 kg of expected DMI/cow/d), cost of the experimental treatments (CON =00€/cow; MON =35€/cow; ECS =15€/cow), cost of any veterinary treatment. Individual milk income was calculated considering the individual daily production for the first 70 DIM with a milk price of 0.41 €/kg. The partial income/cow was calculated as difference between milk income and feed, drugs, veterinary and experimental treatments costs.
Samples analyses
The TMR samples were dried for 48 h at 55 °C, then grounded in a Retch SK mill (Bauknecht, Stuttgart, Germany) to pass 1 mm screen. The TMR chemical analysis were performed as described by Righi et al. (Citation2017). Starch content was assessed by polarimetric method. The fibre fractions were analysed according to Mertens et al. (Citation2002) with the use of heat-stable amylase, without sodium sulphite and expressed inclusive of residual ash. The nitrogen (N) content was measured following the Dumas method (AOAC 968.03; AOAC Citation2005) by the combustion digestion of the samples at 900 °C in excess of oxygen by Dumatherm® (Gerhardt GmbH & Co,Königswinter, Germany). Neutral detergent fibre (NDF) digestibility after 24 h of incubation was assessed as described by Righi et al. (Citation2017).
Colostrum total solids (TS, %) were determined by drying the sample at 103 °C (AOAC Citation2000; 990.20). The ash content was determined by sample ignition at 550 °C (AOAC Citation2000; 945.46), whereas fat and protein content were determined according to Cequier-Sánchez et al. (Citation2008) and via Kjeldhal method (AOAC Citation2000; 991.20), respectively. Furthermore, Ala, Arg, Asp, Glu, Gly, His, Ile, Leu, Lys, Met, Phe, Pro, Ser, Thr, Tyr, Val and minerals were determined chemically with gold standards as described in Goi et al. (Citation2023); whereas total immunoglobulins (Ig) and IgG concentrations were evaluated by NIR spectroscopy as described by Franzoi et al. (Citation2022).
Statistical analysis
Statistical analysis was conducted using SAS 9.4. All analysis of covariance (ANCOVA) assumption were checked with Levene test and Shapiro test.
The treatment effect on colostrum yield, density, IgG, total Ig, AA and mineral concentration and on the ΔBCS (−20 d to 0 DIM, −20 d to 20 DIM, 0 to 20 DIM, 0 to 40 DIM and −20 d to 40 DIM) and on BHBA level has been estimated by ANCOVA. Colostrum data were adjusted for parity and length of dry period (covariate) in the general linear model whereas BCS and BHBA data were adjusted for parity, BCS at −20 d before calving and length of dry period.
In case of repeated measurements, the PROC MIXED was used and the cow’s genetic merit was included using the Italian official genetic selection index of Holstein breed (performances, functionality and type index - PFT). Briefly, for:
BCS: treatment was included as fixed effect as well as the three covariates (BCS at −20 d, length of dry period and DIM) and their interactions.
Milk yield and composition: PFT (covariate) and parity were used as fixed effect.
The prevalence of diseases and of the use of veterinary treatments was tested through the contingency procedure with the Fisher’s exact test. The treatment effect on reproductive performance and the economics evaluation were tested by analysis of variance (ANOVA). For the reproductive performance, the model accounted for the cow’s PFT, parity, previous milk yield, length of dry period and their interactions.
Results
After parturition, 12 animals have been removed from the trial due to complications unrelated to the study. Therefore, the final groups numerosity resulted of 23, 22 and 18 cows in the CON, MON and ECS respectively.
Colostrum
Colostrum yield and density (Figure ) and Ig content (Table ) were not affected by treatments; the total colostrum yield was 16.2 ±0.61 kg, 15.77 ± 0.61 kg and 15.97 ± 0.61 kg for CON, MON and ECS, respectively.
Figure 1. Colostrum yield (a), density (b) and (c) immunoglobulin content for the control (CON), monensin (MON) and nutraceutical (ECS) group (values are reported as least square means ± standard error).

Table 2. First milking colostrum chemical composition, amino acids and mineral content in the control (CON), monensin (MON) and nutraceutical (ECS) group (values are reported as least square means).
The first colostrum total protein content was lower in the treated groups compared to the control (p = .019). Consequently, the first colostrum AA content resulted in lower His, Arg, Ala, Met, Val and Ile in the treated groups compared to the CON (p05); whereas, there was only a trend (p ≤
1) for lower Ser, Asp, Glu, Thr, Pro, Lys, Tyr, Cys, Leu and Phe, content in the treated cows compared to the CON (Table ). The treatments resulted in higher Si, B and Ba content of the first colostrum compared to the control (Table ; p < .05).
BCS and BHBA concentration
The treatments did not affect BCS (Figure ). However, when the ΔBCS at specific time intervals were considered (−20 d to 0 DIM; 0 to 20 DIM; 0 to 40 DIM; −20 d to 40 DIM and 0 to 80 DIM), a trend for the biggest BCS loss in the interval from −20 d to 20 DIM was observed in animals treated with MON (p = .07; Table ). The treatments had no effect on blood BHBA concentration, which was within normal levels in all the groups.
Figure 2. Body condition score (BCS) observed from −20 to 80 DIM for control (CON), monensin (MON) and nutraceutical (ECS) group (values are reported as least square means ± standard error).

Table 3. Body condition score variations (ΔBCS) at different time intervals and beta-hydroxybutyrate (BHBA) blood levels in control (CON), monensin (MON) and nutraceutical (ECS) group (values are reported as least square means).
Milk yield and composition
The weekly milk yield until 70 DIM was higher in both treated groups (p < .001) compared to the CON (LSM ± SE of average milk yield during the first 70 DIM: 46.9 ± 0.78 and 45.3 ± 0.78 versus 41.87 ± 0.78 kg for the MON, ECS and CON), and no differences between MON and ECS during the first 28 DIM were found. From 35 DIM onwards, only MON resulted in higher milk yield compared to the CON and ECS (Figure , Table of Supplementary Material). No treatment effect was observed on milk yield and on the ECM of the entire lactation when considering 305 DIM (Figure ). Furthermore, MON resulted in a tendency for higher fat % (p = .1) and fat yield (p = .058) compared to the CON and the ECS group (Figure ).
Figure 3. Average daily milk yield (kg/d) expressed weekly until 70 DIM ((values are reported as least square means ± standard error).

Diseases prevalence and reproductive performance
All the groups presented an overall diseases prevalence within the expected prevalence for a commercial farm in the northern Italy, although the control group had a prevalence in the upper range. Nonetheless, a trend for an overall lower prevalence of diseases in the MON and ECS compared to the CON was recorded (Figure ); 9.38%, 4.84% versus 18.75% respectively; p = .071). The treatments influenced the calving to first service interval (95.2337.49, 72.89
37.49 and 81.41
37.49 for CON, MON and ECS respectively; p = .011); however, a significant interaction between dry period length and treatment was observed in the calving to first service interval (p = .006; Figure ). In fact, despite an initial balancing of the groups for DIM, after the involuntary removal of 12 animals from the study, MON resulted to be the group with the longest dry period (44.73
7.43; 55.44
15.41; 46.75
3.97 for CON, MON and ECS respectively). This could have partially affected the outcome of the calving to first service interval.
Economic evaluation
The cost of the veterinary interventions, the total costs and the milk and partial income are reported in Table . No differences among groups were observed for the costs (p = .40). Although the MON had a numerically higher milk income compared to CON and ECS, this was not significantly different from the others (p = .65); therefore, the partial income resulted similar among the groups (p = .62).
Table 4. Average veterinary costs and income for cows in control (CON), monensin (MON) and nutraceutical (ECS) group from −20 to 70 DIM (values are reported as least square means).
Discussion
The objective of the study was to compare the effect of two products that, through different mechanisms of action (alteration of rumen metabolism for the monensin and action on the intermediate metabolism for the ECS) aim at enhancing the cows’ ability to cope with the physiological challenges of the transition phase. However, the different half-life of the two products tested (monensin is released for 95 d-, whereas the ECS is released in the rumen between 20 and 24 h after administration, with its compounds having an average half-life of 6–12h), together with the intention of minimising the stress from handling of the animals and of keeping the same number of interventions for treatments administration between groups, has led to different treatments administration times between groups.
Colostrum yield was in line with what reported by previous research (Vasquez et al. Citation2021) with no differences among groups. To the best of our knowledge, there are no studies evaluating the effect of monensin and of the nutraceutical compounds included in the ECS on colostrum yield. However, as reported by Ort et al. (Citation2018), colostrum yield is closely associated with DMI which has been reported not to be altered by monensin (Drong et al. Citation2016), or by the components used in the nutraceutical bolus tested in this study (Carlson et al. Citation2007; Chandra et al. Citation2013), unless used in doses higher than those tested in this trial (e.g. 10 g/d of carnitine, Baghbadorani et al. Citation2022). This let us suppose that the lack of difference in colostrum yield among groups can be related to a lack of changes in feed intake among groups during the transition phase. Colostrum chemical composition was comparable to those reported by other authors (Righi et al. Citation2016; Playford and Weiser Citation2021); furthermore, colostrum density, total Ig and IgG were similar among the groups. However, the protein content of the first milking colostrum of the MON and ECS was lower compared to the CON. To the best of our knowledge, there is no data available describing the effect of monenisin, Echinacea purpurea, vitamin E, carnitine and Silybum marianum on colostrum composition; however, whereas no evidence of monensin altering milk chemical composition has been found, evidences of a possible negative effect of the nutraceutical components on overall mammary gland protein synthesis has been reported (Rekleweska et al. Citation2004; Shedeed et al. Citation2023). Furthermore, Ulger et al. (Citation2017) observed a reduction in milk CP content and in BCS loss when feeding Silybum marianum to dairy cattle, speculating that the hepatoprotective and antioxidant activity of the Silybum marianum ameliorate the transition period through a better nutrient partitioning. In this study, consistently with other researchers (Danesh Mesgaran et al. Citation2021), we did not observe significant differences among groups for the BCS, even though the ECS had a numerically higher BCS 3 wk post-partum, and MON cows had a tendency for a higher BCS loss in the time interval −20 to 20 DIM. Furthermore, no differences were observed among groups for BHBA which is known to be directly related to the degree of lipolysis (Barletta et al. Citation2017). Our results are, therefore, contrasting the hypothesis of Ulger et al. (Citation2017) regarding a possible better nutrient partitioning caused by the ECS.
The lower content of CP and of many AA observed in the treated groups could be explained by the mechanism of action of the treatments themselves. For instance, in the transition period, monensin reduces the action of protein deaminating bacteria in the rumen, increasing the flux of rumen undegraded proteins to the intestine thus, increasing the subsequent use of absorbed AA for gluconeogenesis in animals with energy deficit (Duffield et al. Citation1998). Furthermore, El-Basiony et al. (Citation2015), observed that Echinacea purpurea improves nutrient digestibility in dairy goats with increasing blood serum total protein. According to the authors, this may be due to the antioxidant activity of the polyphenolic components of E. purpurea (Cervellati et al. Citation2002) and or to their antibacterial and antiviral effect and its protectants effect against reactive oxygen species (Bergeron et al. Citation2000). Both activities provide a suitable environment for the rumen microbial growth, therefore enhancing nutrients digestibility. It should be noted, however, that in cited studies the plant products were administered for longer periods compared to the present trial.
The overall milk yield of the first 70 DIM resulted higher for MON and ECS compared to the CON group. Both MON and ECS resulted in similar milk yield until 35 DIM; after that, only monensin resulted in higher production until 70 DIM. However, there were no difference among groups during the whole lactation (LSM of average production: CON = 37.2 ± 1.15; MON = 39.2 ± 1.15; ECS = 38.1 ± 1.15 kg/d). The results are in accordance with other studies reporting the effect of monensin in increasing milk yield until nine weeks of lactation (Arieli et al. Citation2008; McCarthy, Yasui, Ryan, Mechor, et al. Citation2015). Conversely, the ECS treatment increased milk yield only up to 35DIM suggesting that its nature, of fast release product, could explain the shorter effect. These results are in line with other studies which reported an increased milk yield in goats supplemented with Echinacea Purpurea (El-Basiony et al. Citation2015) and in cows supplemented with silymarin (Tedesco, Tava. et al. Citation2004). In accordance with other research (El-Basiony et al. Citation2015; Mammi et al. Citation2021), milk composition wasn’t affected by treatments; however, few study reported a lowering effect of monensin on milk components (Phipps et al. Citation2000; Mezzetti et al. Citation2019), whereas other studies reported evidences of a possible negative effect of the components used in the ECS on overall mammary gland protein synthesis (Rekleweska et al. 2004; Shedeed et al. Citation2023).
Monensin is reported to reduce the risk of ketosis (e.g. animals with high BCS at parturition), by increasing propionate availability (Mammi et al. Citation2021). In our study, no cows were remarkably over-condition before parturition therefore, it could be speculated that the monensin-related increased availability of propionate, reflected in an increased availability of acetate for milk fat synthesis, which translated in a slightly increased milk fat content.
Reklewska et al. (Citation2004) reported a transient reduction of milk protein content coupled with an increment of milk lactoferrin followed by increased milk yield in dairy goats supplemented with Echinacea purpurea. On the other hand, the supplementation with silymarin-rich extract in dairy goats alters milk composition by increasing β-casein, αs1-casein and αs2-casein (Shedeed et al. Citation2023). In both cited studies however, the treatments have been administered for longer period than what done in our study; therefore, the lack of difference in protein content of the ECS group may be related to its nature, of fast release product.
According to Ribeiro and Carvalho (Citation2017), about 33% of dairy cows has at least one clinical disease in the first three weeks of lactation, with the most common diseases being metritis, mastitis, digestive problems, lameness and respiratory problems. In our study, the disease prevalence was below 20%, a percentage within the expected prevalence for a commercial farm in the northern Italy, with the most prevalent diseases being the same listed by Ribeiro and Carvalho (Citation2017). However, a trend for a lower diseases prevalence in the treated groups was observed with only 9.38% and 4.84% of the cows affected by one or more diseases in the MON and ECS vs the 18.75% of the CON cows. This could be explained by an overall increased stress resilience of the treated animals. In fact, by ameliorating cows’ energy balance (Duffield et al. Citation2003; Hausmann et al. Citation2018), monensin could have exerted beneficial effects on the transition cows’ immune status (Bekuma Citation2019). On the other hand, the ECS treatment comprised Echinacea purpurea, reported to modulate innate and adaptive immune response (LaLone et al. Citation2007; Seckin et al. 2018); vitamin E, that neutralises reactive oxygen species (Bass Citation1999); carnitine, that is involved in oxidation of long-chain fatty acids, regulation of ketosis, support of the immune system, enhancement of the antioxidant system (Citil et al. Citation2009; Pirestani and Aghakhani Citation2018); silymarin, assisting in metabolic adaptation to the onset of lactation due to its hepatoprotective properties (Tedesco, Domeneghini, et al. Citation2004; Tedesco, Tava, et al. Citation2004).
Consistently with the study of Mohammadi et al. (Citation2012), the MON cows had the shortest calving to first service interval. Although it could have been speculated that this result might have been related to a change in the propionic acid available for synthesis of glucose (Neto et al. Citation2009), it needs to be kept in mind that the MON was also the group with the longest dry period prior to the beginning of this study, therefore definitive conclusions cannot be drawn.
Despite the higher milk production of both, the MON and the ECS over the first 70 DIM, the economical evaluation of the groups within this period showed no significative differences for the costs and only a trend for a higher milk income and partial income of the MON and ECS compared to the CON. This can be explained, in part, by the higher veterinary treatment costs of the MON group (25.91€more than the ECS) and by the higher cost of the monensin product (35 vs 15€for MON and ECS), and in part by the higher estimated intake, calculated based on the milk yield, which increased the feed costs for the more producing groups. Therefore, the MON and the ECS groups generated a daily partial income of 1.895 and 1.868€more than the CON. Considering an average milk yield of 45 kg/d, the treatments increased the partial income/kg of milk produced of 0.042€(MON) and 0.041€(ECS), respectively, thus making the ECS a valid alternative to the use of antibiotic-based products.
Conclusions
Our findings strongly suggest that the combination of molecules contained in the ECS are effective in improving the energy utilisation during the transition and early lactation periods and can be an alternative to a glucogenic, antibiotic-based rumen environment modifier. The results also indicate the need to test further protocols of the nutraceutical administration, capable guarantee the availability of the molecules for longer period. Further studies, enrolling bigger sample size and including in-depth molecular analyses are necessary to corroborate these results.
Ethical approval
The study complied with the Italian laws on animal experimentation and ethics (DL 04/03/2014 n. 26). All research reported in this research has been conducted in an ethical and responsible manner.
Supplemental Material
Download MS Word (14 KB)Disclosure statement
No potential conflict of interest was reported by the author(s).
Data availability statement
The data that support the findings of this study are available from the corresponding author, [S.M.], upon reasonable request.
References
- Abuelo A, Hernández J, Benedito JL, Castillo C. 2019. Redox biology in transition periods of dairy cattle: role in the health of periparturient and neonatal animals. Antioxidants. 8(1):20. doi: 10.3390/antiox8010020.
- Allen MS, Sousa DO, VandeHaar MJ. 2019. Equation to predict feed intake response by lactating cows to factors related to the filling effect of rations. J Dairy Sci. 102(9):7961–7969. doi: 10.3168/jds.2018-16166.
- AOAC. 2000. Official Methods of Analysis. 17th ed. Gaithersburg, MD, USA: The Association of Official Analytical Chemists.doi: 10.1007/s10811-008-9326-4.
- AOAC (2005) Official method of analysis. 18th ed. Washington DC, USA: Association of Official Analytical Chemists. doi: 10.1093/jaoac/85.5.1187.
- Arieli A, Dicken U, Dagoni I, Spirer Y, Zamwel S. 2008. Production and health of cows given monensin prepartum and a high-energy diet postpartum. J Dairy Sci. 91(5):1845–1851. doi: 10.3168/jds.2007-0795.
- Baghbadorani MK, Mehrzad J, Vodjgani M, Khosravi A, Akbarinejad V. 2022. Evaluation of biochemical and hematological parameters in postpartum Holstein dairy cows following supplementation of Immunofin® herbal extract. Iran J Vet Med. 16:274–287. doi: 10.22059/IJVM.2022.334623.1005210.
- Barletta RV, Maturana Filho M, Carvalho PD, Del Valle TA, Netto AS, Rennó FP, Mingoti RD, Gandra JR, Mourão GB, Fricke PM, et al. 2017. Association of changes among body condition score during the transition period with NEFA and BHBA concentrations, milk production, fertility, and health of Holstein cows. Theriogenology. 104:30–36. doi: 10.1016/j.theriogenology.2017.07.030.
- Bass RT. 1999. Effects of vitamin E supplementation in late gestation cattle and evaluation of vitamin E, cholesterol, and phospholipid relationships in bovine serum and serum lipoproteins. Doctoral dissertation, Virginia Polytechnic Institute and State University.
- Batistel F, Arroyo JM, Garces CIM, Trevisi E, Parys C, Ballou MA, Cardoso FC, Loor JJ. 2017. Ethyl-cellulose rumen-protected methionine alleviates inflammation and oxidative stress and improves neutrophil function during the periparturient period and early lactation in Holstein dairy cows. J Dairy Sci. 101(1):480–490. doi: 10.3168/jds.2017-13185.
- Bekuma A. 2019. Combating negative effect of negative energy balance in dairy cows: comprehensive review. Approach Poult Dairy Vet Sci. 6:1–4. doi: 10.31031/apdv.2019.06.000633.
- Bergeron C, Livesey JF, Awang DVC, Arnason JT, Rana J, Baum BR, Letchamo W. 2000. A quantitative HPLC method for the quality assurance of Echinacea products on the North American market. Phytochem Anal. 11(4):207–215. doi: 10.1002/1099-1565(200007/08)11:4<207::AID-PCA519>3.0.CO;2-T.
- Cadórniga-Valiño C, Grummer RR, Armentano LE, Donki SS, Bertics SJ. 1997. Effects of fatty acids and hormones on fatty acid metabolism and gluconeogenesis in bovine hepatocytes. J Dairy Sci. 80(4):646–656. doi: 10.3168/jds.S0022-0302(97)75983-6.
- Cardoso CL, King A, Chapwanya A, Esposito G. 2021. Growth and puberty of calves—a review. Animals. 11(5):1212–1224. doi: 10.3390/ani11051212.
- Carlson DB, McFadden JW, D'Angelo A, Woodworth JC, Drackley JK. 2007. Dietary L-carnitine affects periparturient nutrient metabolism and lactation in multiparous cows. J Dairy Sci. 90(7):3422–3441. doi: 10.3168/jds.2006-811.
- Cequier-Sánchez E, Rodríguez C, Ravelo ÁG, Zárate R. 2008. Dichloromethane as a solvent for lipid extraction and assessment of lipid classes and fatty acids from samples of different natures. J Agric Food Chem. 56(12):4297–4303. doi: 10.1021/jf073471e.
- Cervellati R, Renzulli C, Guerra MC, Speroni E. 2002. Evaluation of antioxidant activity of some natural polyphenolic compounds using the Briggs-Rauscher reaction method. J Agric Food Chem. 50(26):7504–7509. doi: 10.1021/jf020578n.
- Chandra G, Aggarwal A, Singh AK, Kumar M, Upadhyay RC. 2013. Effect of vitamin E and zinc supplementation on energy metabolites, lipid peroxidation, and milk production in peripartum sahiwal cows. Asian-Australas J Anim Sci. 26(11):1569–1576. doi: 10.5713/ajas.2012.12682.
- Citil M, Karapehlivan M, Erdogan HM, Yucayurt R, Atakisi E, Atakisi O. 2009. Effect of orally administered l-carnitine on selected biochemical indicators of lactating Tuj-ewes. Small Rumin Res. 81(2-3):174–177. doi: 10.1016/j.smallrumres.2008.12.001.
- Coleman DN, Alharthi A, Lopreiato V, Trevisi E, Miura M, Pan YX, Loor JJ. 2019. Choline supply during negative nutrient balance alters hepatic cystathionine β-synthase, intermediates of the methionine cycle and transsulfuration pathway, and liver function in Holstein cows. J Dairy Sci. 102(9):8319–8331. doi: 10.3168/jds.2019-16406.
- Danesh Mesgaran M, Kargar H, Danesh Mesgaran S, Javadmanesh A. 2021. Peripartal rumen-protected L-carnitine manipulates the productive and blood metabolic responses in high-producing Holstein dairy cows. Front Vet Sci. 8:769837. doi: 10.3389/fvets.2021.769837.
- Drong C, Meyer U, von Soosten D, Frahm J, Rehage J, Breves G, Dänicke S. 2016. Effect of monensin and essential oils on performance and energy metabolism of transition dairy cows. J Anim Physiol Anim Nutr. 100(3):537–551. doi: 10.1111/jpn.12401.
- Duffield TF, LeBlanc S, Bagg R, Leslie K, Ten Hag J, Dick P. 2003. Effect of a monensin controlled release capsule on metabolic parameters in transition dairy cows. J Dairy Sci. 86(4):1171–1176. doi: 10.3168/jds.S0022-0302(03)73700-X.
- Duffield TF, Rabiee AR, Lean IJ. 2008. A meta-analysis of the impact of monensin in lactating dairy cattle. Part 3. Health and reproduction. J Dairy Sci. 91(6):2328–2341. doi: 10.3168/jds.2007-0801.
- Duffield TF, Sandals D, Leslie KE, Lissemore K, McBride BW, Lumsden JH, Dick P, Bagg R. 1998. Effect of prepartum administration of monensin in a controlled-release capsule on postpartum energy indicators in lactating dairy cows. J Dairy Sci. 81(9):2354–2361. doi: 10.3168/jds.S0022-0302(98)70126-2.
- Edmonson AJ, Lean IJ, Weaver LD, Farver T, Webster G. 1989. A body condition scoring chart for Holstein dairy cows. J Dairy Sci. 72(1):68–78. doi: 10.3168/jds.S0022-0302(89)79081-0.
- El-Basiony A, Khattab H, Kholif A, Hadhoud F, El-Alamy H. 2015. Effect of using Echinacea purpurea, Nigella sativa and Chicorium intybus in dairy goats’ diet on milk production and quality: 2- effect on digestibility, some blood parameters and milk production and quality. Egypt J Nutr Feed. 18(2):137–145. doi: 10.21608/ejnf.2015.104436.
- Esposito G, Medina VA, Schneider A, Gilbert RO, Butler WR. 2014. Effect of dietary conjugated linoleic acid (CLA) on the metabolism and reproduction of dairy cows. SA J an Sci. 43(5):33–37. doi: 10.4314/sajas.v43i5.6.
- Esposito G, Raffrenato E, Lukamba SD, Adnane M, Irons PC, Cormican P, Tasara T, Chapwanya A. 2020. Characterization of metabolic and inflammatory profiles of transition dairy cows fed an energy-restricted diet. J Anim Sci. 98(1):1–15. doi: 10.1093/jas/skz391.
- European Medicine Agency. 2013. European public assessment reports (EPAR). [accessed 2024 Jan 1]. https://ec.europa.eu/health/documents/community-register/2013/20130128125085/anx_125085_it.pdf.
- Franzoi M, Costa A, Goi A, Penasa M, De Marchi M. 2022. Effectiveness of visible – near infrared spectroscopy coupled with simulated annealing partial least squares analysis to predict immunoglobulins G, A, and M concentration in bovine colostrum. Food Chem. 371:131189. doi: 10.1016/j.foodchem.2021.131189.
- Goi A, De Marchi M, Costa A. 2023. Minerals and essential amino acids of bovine colostrum: phenotypic variability and predictive ability of mid- and near-infrared spectroscopy. J Dairy Sci. 106(12):8341–8356. doi: 10.3168/jds.2023-23459.
- Haga S, Ishizaki H, Roh S. 2021. The physiological roles of vitamin e and hypovitaminosis e in the transition period of high-yielding dairy cows. Animals. 11(4):1088. doi: 10.3390/ani11041088.
- Hammer KA, Carson CF, Riley TV. 1999. Antimicrobial activity of essential oils and other plant extracts. J Appl Microbiol. 86(6):985–990. doi: 10.1046/j.1365-2672.1999.00780.x.
- Hausmann J, Deiner C, Patra AK, Immig I, Starke A, Aschenbach JR. 2018. Effects of a combination of plant bioactive lipid compounds and biotin compared with monensin on body condition, energy metabolism and milk performance in transition dairy cows. PLoS One. 13(3):e0193685. doi: 10.1371/journal.pone.0193685.
- Hermenean A, Stan M, Ardelean A, Pilat L, Mihali CV, Popescu C, Nagy L, Deák G, Zsuga M, Kéki S, et al. 2015. Antioxidant and hepatoprotective activity of milk thistle (Silybum marianum L. Gaertn.) seed oil. Open Life Sci. 10(1):147–158. doi: 10.1515/biol-2015-0017.
- Hungate RE. 1975. The rumen microbial ecosystem. Annual Review of Ecology and Systematics 6(1):39–66.
- LaLone CA, Hammer KDP, Wu L, Bae J, Leyva N, Liu Y, Solco AKS, Kraus GA, Murphy PA, Wurtele ES, et al. 2007. Echinacea species and alkamides inhibit prostaglandin E2 production in RAW264.7 mouse macrophage cells. J Agric Food Chem. 55(18):7314–7322. doi: 10.1021/jf063711a.
- Lessard M, Gagnon N, Godson DL, Petit HV. 2004. Influence of parturition and diets enriched in n-3 or n-6 polyunsaturated fatty acids on immune response of dairy cows during the transition period. J Dairy Sci. 87(7):2197–2210. doi: 10.3168/jds.S0022-0302(04)70040-5.
- Lopreiato V, Mezzetti M, Cattaneo L, Ferronato G, Minuti A, Trevisi E. 2020. Role of nutraceuticals during the transition period of dairy cows: a review. J Anim Sci Biotechnol. 11(1):96. doi: 10.1186/s40104-020-00501-x.
- Mammi LME, Guadagnini M, Mechor G, Cainzos JM, Fusaro I, Palmonari A, Formigoni A. 2021. The use of monensin for ketosis prevention in dairy cows during the transition period: a systematic review. Animals. 11(7):1988. doi: 10.3390/ani11071988.
- McArt JAA, Nydam DV, Oetzel GR. 2012. Epidemiology of subclinical ketosis in early lactation dairy cattle. J Dairy Sci. 95(9):5056–5066. doi: 10.3168/jds.2012-5443.
- McCarthy MM, Yasui T, Ryan CM, Mechor GD, Overton TR. 2015. Performance of early-lactation dairy cows as affected by dietary starch and monensin supplementation. J Dairy Sci. 98(5):3335–3350. doi: 10.3168/jds.2014-8820.
- McCarthy MM, Yasui T, Ryan CM, Pelton SH, Mechor GD, Overton TR. 2015. Metabolism of early-lactation dairy cows as affected by dietary starch and monensin supplementation. J Dairy Sci. 98(5):3351–3365. doi: 10.3168/jds.2014-8821.
- McNeil BK, Renaud DL, Steele MA, Keunen AJ, DeVries TJ. 2023. Effects of Echinacea purpurea supplementation on markers of immunity, health, intake, and growth of dairy calves. J Dairy Sci. 106(7):4949–4965. doi: 10.3168/jds.2022-22862.
- Mertens DR, Allen M, Carmany J, Clegg J, Davidowicz A, Drouches M, Frank K, Gambin D, Garkie M, Gildemeister B, et al. 2002. Gravimetric determination of amylase-treated neutral detergent fiber in feeds with refluxing in beakers or crucibles: collaborative study. J AOAC Int. 85(6):1217–1240.
- Mezzetti M, Cattaneo L, Passamonti MM, Lopreiato V, Minuti A, Trevisi E. 2021. The transition period updated: a review of the new insights into the adaptation of dairy cows to the new lactation. Dairy. 2(4):617–636. doi: 10.3390/dairy2040048.
- Mezzetti M, Piccioli-Cappelli F, Bani P, Amadori M, Calamari L, Minuti A, Loor JJ, Bionaz M, Trevisi E. 2019. Monensin controlled-release capsule administered in late-pregnancy differentially affects rumination patterns, metabolic status, and cheese-making properties of the milk in primiparous and multiparous cows. Ital J Anim Sci. 18(1):1271–1283. doi: 10.1080/1828051X.2019.1645623.
- Mohammadi MC, Ghasrodashti AR, Tamadon A, Behzadi MA. 2012. Effects of prepartum monensin feeding on energy metabolism and reproductive performance of postpartum high-producing Holstein dairy cows. Pak Vet J. 32:45–49.
- Neto GB, Berndt A, Nogueira JR, Nogueira JC, Demarchi JJAA. 2009. Volatile fatty acids in cattle supplemented with protein-enriched salt and sodium monensin. S Afr J Anim Sci. 39:284–288.
- Ort SB, Aragona KM, Chapman CE, Shangraw E, Brito AF, Schauff DJ, Erickson PS. 2018. The impact of direct-fed microbials and enzymes on the health and performance of dairy cows with emphasis on colostrum quality and serum immunoglobulin concentrations in calves. J Anim Physiol Anim Nutr. 102(2):e641–e652. doi: 10.1111/jpn.12806.
- Phipps RH, Wilkinson JID, Jonker LJ, Tarrant M, Jones AK, Hodge A. 2000. Effect of monensin on milk production of Holstein-Friesian dairy cows. J Dairy Sci. 83(12):2789–2794. doi: 10.3168/jds.S0022-0302(00)75176-9.
- Pirestani A, Aghakhani M. 2018. The effects of rumen-protected choline and L-carnitine supplementation in the transition period on reproduction, production, and some metabolic diseases of dairy cattle. J Appl Anim Res. 46(1):435–440. doi: 10.1080/09712119.2017.1332632.
- Playford RJ, Weiser MJ. 2021. Bovine colostrum: its constituents and uses. Nutrients. 13(1):265. doi: 10.3390/nu13010265.
- Regulation (EC) No 1831/2003 of the European Parliament and of the council. 2023. Regulation of 22 September 2003 on the additives for use in animal nutrition. OJ L. 268:29.
- Reklewska B, Bernatowicz E, Ryniewicz Z, Pinto RR, Zdziarski K. 2004. Preliminary observations on the Echinacea-induced lactoferrin production in goat milk. Anim Sci Pap Rep. 22:17–25.
- Ribeiro ES, Carvalho MR. 2017. Impact and mechanisms of inflammatory diseases on embryonic development and fertility in cattle. Anim Reprod. 14(3):589–600. doi: 10.21451/1984-3143-AR1002.
- Righi F, Simoni M, Malacarne M, Summer A, Costantini E, Quarantelli A. 2016. Feeding a free choice energetic mineral-vitamin supplement to dry and transition cows: effects on health and early lactation performance. Large Anim Rev. 22:161–170.
- Righi F, Simoni M, Visentin G, Manuelian CL, Currò S, Quarantelli A, De Marchi M. 2017. The use of near infrared spectroscopy to predict faecal indigestible and digestible fibre fractions in lactating dairy cattle. Livest Sci. 206:105–108. doi: 10.1016/j.livsci.2017.10.006.
- Ringseis R, Keller J, Eder K. 2018. Regulation of carnitine status in ruminants and efficacy of carnitine supplementation on performance and health aspects of ruminant livestock: a review. Arch Anim Nutr. 72(1):1–30. doi: 10.1080/1745039X.2017.1421340.
- Seckin C, Alpun Kalayci G, Turan N, Yilmaz A, Cizmecigil UY, Aydin O, Richt JA, Yilmaz H. 2018. Immunomodulatory effects of echinacea and pelargonium on the innate and adoptive immunity in calves. Food and Agricultural Immunology. 29(1):744–761. doi: 10.1080/09540105.2018.1444738.
- Shedeed SM, ElSabaawy EH, Mohamed MI, Mabrok HB, Abd El-Wahab WM. 2023. Impact of feeding ration supplemented with silymarin-rich extract on milk quality of goat and utilization of milk in producing functional soft-cheese. Egypt J Chem. 0(0):0–0. doi: 10.21608/ejchem.2022.132914.5871.
- Tedesco D, Domeneghini C, Sciannimanico D, Tameni M, Steidler S, Galletti S. 2004. Silymarin, a possible hepatoprotector in dairy cows: biochemical and histological observations. J Vet Med A Physiol Pathol Clin Med. 51(2):85–89. doi: 10.1111/j.1439-0442.2004.00603.x.
- Tedesco D, Tava A, Galletti S, Tameni M, Varisco G, Costa A, Steidler S. 2004. Effects of silymarin, a natural hepatoprotector, in periparturient dairy cows. J Dairy Sci. 87(7):2239–2247. doi: 10.3168/jds.S0022-0302(04)70044-2.
- Tyrrell HF, Reid JT. 1965. Prediction of the energy value of cow’s milk. J Dairy Sci. 48(9):1215–1223. doi: 10.3168/jds.S0022-0302(65)88430-2.
- Ulger I, Onmaz AC, Ayaşan T. 2017. Effects of silymarin (Silybum marianum) supplementation on milk and blood parameters of dairy cattle. S Afr J Anim Sci. 47(6):758–765. doi: 10.4314/sajas.v47i6.3.
- Vasquez JA, McCarthy MM, Richards BF, Perfield KL, Carlson DB, Lock AL, Drackley JK. 2021. Effects of prepartum diets varying in dietary energy density and monensin on early-lactation performance in dairy cows. J Dairy Sci. 104(3):2881–2895. doi: 10.3168/jds.2020-19414.
- Wallace RJ. 2004. Antimicrobial properties of plant secondary metabolites. Proc Nutr Soc. 63(4):621–629. doi: 10.1079/pns2004393.
- Weiss WP. 2017. A 100-year review: from ascorbic acid to zinc—mineral and vitamin nutrition of dairy cows. J Dairy Sci. 100(12):10045–10060. doi: 10.3168/jds.2017-12935.
- Xiao J, Khan MZ, Ma Y, Alugongo GM, Ma J, Chen T, Khan A, Cao Z. 2021. The antioxidant properties of selenium and vitamin e; their role in periparturient dairy cattle health regulation. Antioxidants. 10(10):1555. doi: 10.3390/antiox10101555.
- Yoruk R, Marshall MR. 2003. Physicochemical properties and function of plant polyphenol oxidase: a review. J Food Biochem. 27(5):361–422. doi: 10.1111/j.1745-4514.2003.tb00289.x.
- Zhou Z, Ferdous F, Montagner P, Luchini DN, Corrêa MN, Loor JJ. 2018. Methionine and choline supply during the peripartal period alter polymorphonuclear leukocyte immune response and immunometabolic gene expression in Holstein cows. J Dairy Sci. 101(11):10374–10382. doi: 10.3168/jds.2018-14972.