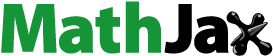
Abstract
Herbal plants have gained enormous interest due to their immune, antioxidant, and antibacterial activities. The present study proposed that white poplar (Populus alba) leave extract (WPE) might provide a promising alternative to traditional antibiotics to treat Aeromonas veronii infection in Nile tilapia (Oreochromis niloticus). WPE showed an in vitro antibacterial activity [22 ± 0.45 mm inhibition zone, with minimum inhibitory concentration (MIC) and minimum bactericidal concentration (MBC) of 60 µg/mL] against A. veronii. For the in-vivo study, a factorial (2 × 2) design was used to investigate the therapeutic effects of WPE on the antioxidant/immune status and blood biochemical parameters of Nile tilapia challenged with A. veronii. One-hundred sixty fish (33 ± 1.5 g) were assigned into four experimental groups, each with four replicates (4 glass tanks/group, 40 fish/group, 10 fish/tank) for 10 days. In the first (1st) and 2nd groups, 0 and 1.5 mg/L WPE were applied in tank water, respectively, without fish being challenged. In the 3rd and 4th groups, 0 and 1.5 mg/L WPE were applied in tank water, respectively, with fish intraperitoneal inoculated with 0.2 mL of A. veronii (0.5 × 107 CFU). Aeromonas veronii infection significantly diminished the survivability, hepatic catalase, lysozyme activity, nitric oxide, immunoglobulin M, phagocytic %, total protein, albumin, and globulin. Moreover, a significant rise in the hepatic malondialdehyde, serum hepatorenal function indicators, cortisol, and glucose levels were consequences of A. veronii challenge. Interestingly, the interaction between bacterial challenge and WPE application increased the survivability, antioxidant activity, and immune responses and reduced ALT levels of fish treated with WPE during the bacterial challenge. The serum creatinine, cortisol, and glucose levels were decreased in fish treated with WPE during the infection but still higher than in the non-infected fish either treated or not treated with WPE. In conclusion, WPE (1.5 mg/L) can be used as an antibacterial substance in fish farming to alleviate the detrimental impacts of A. veronii infection by enhancing the antioxidant/immune status of the infected fish. These outcomes could help reduce antibiotic usage in fish farms, which is conducive to the sustainable development of aquaculture.
HIGHLIGHTS
White poplar extract (WPE) had an in vitro antibacterial activity against Aeromonas veronii.
Aeromonas veronii infection inhibited the antioxidant/immune functions of Nile tilapia.
Aeromonas veronii infection altered the hepatorenal functions and biochemical parameters of Nile tilapia.
WPE at a level of 1.5 mg/L displayed a therapeutic effect in Nile tilapia against A. veronii.
Introduction
Today, aquaculture produces more than 49.2% of all fish consumed globally (FAO Citation2022). The speedy growth of fish production results in numerous dangerous problems, such as high-density culture incidents and the shortage of effective disease prevention approaches. Consequently, this extension may initiate stressful conditions, suppressing the immune system and increasing the fish’s vulnerability to infectious agents (Harikrishnan et al. Citation2011a; Lieke et al. Citation2020). In addition, various stressors that result from environmental changes and human activity can pose problems for fish (Schreck and Tort Citation2016; Reid et al. Citation2019). Fish immune systems and antioxidant capability are compromised by these stressors, which causes reproductive dysfunction and potentially catastrophic financial losses (Ahmed et al. Citation2022).
Treatment of bacterial pathogens using antibiotics in aquaculture is not endorsed due to their residues in fish, increased resistant pathogenic bacteria, and adverse environmental impacts that harm human well-being (El-Nobi et al. Citation2021). Among these pathogens was the Aeromonas veronii (A. veronii) infection, which causes ulcerative lesions and haemorrhagic septicaemia (Amal et al. Citation2018; Abd El Latif et al. Citation2019; Youssuf et al. 2020). The significant threats behind A. veronii infection in tilapia culture are the massive mortalities and resistant to various antibiotics like neomycin, oxytetracycline, norfloxacin, nalidixic acid, lincomycin, ampicillin, gentamycin, and tetracycline (Lazado and Zilberg Citation2018; Abd El Latif et al. Citation2019). Thus, it is critical to find a different strategy to enhance the general health of fish and reduce antibiotic usage (Ibrahim et al. Citation2024). As a result, improving disease resistance and enhancing fish’s antioxidant and immune capacity have become necessary in healthful aquaculture (Amer et al. Citation2022; Abdel Rahman et al. Citation2023). Currently, aquaculture uses plant supplements as exogenic immune-prophylactics and antioxidants (Thanigaivel et al. Citation2016; Alagawany et al. Citation2020; Ahmadifar et al. Citation2021). The majority of plants and their extracts have quinones, phenolic glycosides, polyphenols, terpenoids, flavonoids, alkaloids, polypeptides, tannins, and polysaccharides substances that are efficient substitutes for antibiotics and synthetical composites (Abdou Said et al. Citation2021; Nik Mohamad Nek Rahimi et al. Citation2022). Besides, Medicinal plants are tremendous sources of nutrients for animals, eco-friendly, economical, locally available, and can fend against a wide range of bacteria (Tadese et al. Citation2022; Nguyen et al. Citation2023).
Among these medicinal plants, white poplar, Populus alba (P. alba), is a medicinal herb of the Salicaceae (willow) family, which is frequently grown in temperate and subtropical areas (Tawfeek et al. Citation2019). Its native range extends from the Atlas Mountains in Africa through most of southern and central Europe to Central Asia. It has been introduced into various temperate and humid regions around the world. It grows on moist sites, often next to water, in hot summers and cold to mild winters (Mifsud 2002; Fussi et al. Citation2010; Costa et al. Citation2011; Caudullo and De Rigo 2016). Worldwide, 91% of poplars are found in natural forests (Singh and Kumar Citation2012); nevertheless, introduced forests are small but quickly developing components of poplar forests. There are ∼5 million hectares of poplar plantations worldwide and another 1.5 million hectares in agroforestry systems (Kutsokon et al. Citation2015). Most (73% of the global population) are farmed in China (FAO Citation2005). One of the most desirable characteristics of poplar trees is their rapid growth. In the United States, particular studies specify a range of production rates from 5.4 to 30 mg/ha/year, and findings depend on many factors (Riemenschneider et al. Citation2001; Geyer Citation2006; Goerndt and Mize Citation2008).
The efficacy of ethanolic extracts of P. alba leaves against various microorganisms has been established (Nassima et al. Citation2019). The effective substances from the genus Populus are identified in conventional medication for diverse biological activities involving antioxidant, antiseptic, antiviral, antifungal, and antitumoral (Tebbi and Debbache-Benaida Citation2022). Incorporating P. alba extract into an agar medium showed the antimycobacterial effect against the growth of three types of mycobacteria due to their content of flavonoids and polyphenols (Haouat et al. Citation2013). An earlier study showed that chitosan white poplar nanocapsule had an in vitro antibacterial activity against Streptococcus agalactiae (Abdel Rahman et al. Citation2023). We designated this study based on the detrimental impacts of A. veronii in tilapia culture, the resistance of this strain to various antibiotics, the urgency to reduce antibiotic usage in aquaculture, and the potential therapeutic effect of white poplar extract (WPE). The in vitro antibacterial action of WPE against A. veronii was assessed. In addition, the potential therapeutic effects of WPE on the antioxidant activity, immune status, and blood biochemical parameters of Nile tilapia challenged with A. veronii were evaluated.
Materials and methods
Preparation of WPE
Fresh white poplar leaves were obtained from the Faculty of Agriculture, Benha University, Egypt, and were identified by the classification unit at the Desert Research Centre, Cairo, Egypt. Dehydrated white poplar leaves were ground into a powder with milling; then, 10 g of flour was mixed with 100 mL acidified ethanol (70%) solution [0.1 M HCl (v/v)] to prepare the ethanolic extract according to Zhao et al. (Citation2008).
Determination of the phytochemical constituents of the plant extract
The WPE's qualitative phytochemical components, including its flavonoids, phenols, terpenes, steroids, alkaloids, cardiac glycosides, saponins, and tannins, were evaluated using Trease and Evans’ standard methodology (Trease and Evans Citation1989).
Gas chromatography-mass spectrometry analysis (GC-MS)
At the Central Laboratories Network, National Research Centre, Cairo, Egypt, a gas chromatograph (GC) (7890B) and mass spectrometer detector (5977 A) were part of the GC-MS system (Agilent Technologies). An HP-5MS column with dimensions of 30 × 0.25 mm internal diameter and 0.25 μm film thickness was installed in the GC. The following temperature program was used during the analysis, with hydrogen serving as the carrier gas at a rate of 1.0 mL/min at a splitless injection volume of 1 µl (temperature: 50 °C for 1 min; rising at 10 °C/min to 300 °C and held for 20 min). The injector and detector were kept at 250 °C. Mass spectra were generated by electron ionisation (EI) at 70 eV with a spectral range of m/z 30–700 and a solvent delay of 9 min. The Quad was 150 °C, but the majority was 230 °C. Identifying numerous chemicals by contrasting the spectrum fragmentation pattern with those seen in the Wiley and NIST Mass Spectral Library data was feasible.
Isolation of the bacterial strain
Aeromonas veronii strain (131TF-ID) was isolated (in the Aquatic Animal Medicine, Faculty of Veterinary Medicine, Zagazig University, Egypt) from sick O. niloticus. The strain was identified using the VITEK® 2 system (BioM' Erieux Inc., NC, USA) and the universal bacterial primers 27F (5′-AGAGTTTGATCMTGGCTCAG-3′) and 1492R (5′-TACGGYTACCTTGTTACGACTT-3′) according to Reda et al. (Citation2022). The isolate was grown for 24 h at 27 °C on tryptic soy agar, and then one colony was incubated in tryptic soy broth for another 24 h at 27 °C. The pellet from the A. veronii cultured broth was collected and suspended in a sterile phosphate-buffered saline solution after centrifuging for 10 min at 3000 rpm at 4 °C. Aeromonas veronii’s lethal dosage 50 (LD50) was established (Ibrahim et al. Citation2024). Five groups were created using 100 fish with two replicates (10 fish/replicate; 20 fish/group). The fish were deprived of food for 24 h before the experimental infection. The fish groups were intraperitoneally (IP) injected with bacterial suspension at various concentrations, which were adjusted by the McFarland standard tube. A 0.2 mL of A. veronii (105–108 CFU/fish) was injected into the first through fourth groups, and 0.2 mL of sterile saline was injected into the fifth group. Four days following the injection, the fish deaths were noted. The LD50 was calculated to be 2 × 107 CFU/mL based on the results of the Probit Analysis Programme (version 1.5). A sub-lethal dosage of 0.5 × 107 was determined and used in the treatment study.
In vitro study for determining the antibacterial activity
Disc diffusion assay
The disc diffusion technique was used to determine the zone of inhibition during the antibacterial screening of the extracts (Liu et al. Citation2016). On Muller Hinton agar plates (Oxoid, England), 100 µL of a bacterial suspension containing 0.5 × 107 CFU/mL (equal to 0.5 McFarland turbidity) was uniformly streaked. The striped plates were placed in the incubator and dried for 20 min at 37 °C. Onto sterile Whatman paper discs (5 mm in diameter) were soaked in 10 µL of WPE solution (1.5 µg/mL). The discs were also dried in an incubator. The cefotaxime antibiotic disc (10 µg, Oxoid, England) (Vila et al. Citation2002) was used as a positive control, and a disc soaked in ultra-purified water (10 µL) was utilised as a negative control. The dried discs were added to the inoculation plates and incubated for 24 h at 37 °C. The inhibitory zones were then calibrated to the nearest millimetre (mm). The assay was carried out in triplicates.
Minimum inhibitory concentration (MIC)
WPE was subjected to the MIC procedure (Swain et al. Citation2014). In brief, we prepared serial dilutions (0, 10, 20, 30, 40, 50, 60, 70, 80, 90, and 100 µg/mL) from the extract. The cultures containing 0.5 × 107 CFU/mL of the bacterial isolate were added, and the 96-well plate was incubated, as mentioned before. The MIC level was at which there was no sign of bacterial growth on the plate.
Minimum bactericidal concentration (MBC)
MBC was achieved by sub-culturing from each well onto a nutrient agar plate. For that test strain, the plate with the lowest extract concentration that failed to produce growth on subculture was regarded as MBC (Ibrahim et al. Citation2023).
Fish
Two hundred sixty O. niloticus (33 ± 1.5 g) were acquired from the Central Laboratory of Aquaculture Research in Abbasa, Egypt. Following CCAC (Citation2005) recommendations, they were examined and stocked as ten fish per glass tank (50 × 40 × 60 cm) with 90 L water volume. The fish were initially held for a 14-day adaptation period, during which they were fed a commercial diet and monitored for disease and mortality. The physiochemical characteristics of water were observed according to APHA (Citation1992). Every day at 9:00 am, the water parameters were measured using an oxygen metre (970 portable DO metre, Jenway, London, UK) for dissolved oxygen (DO) and water temperature (T; °C). A pH metre (Digital Mini-pH Metre, model 55, Fisher Scientific, Denver, CO, USA) was used to measure the pH. Salinity was measured using a refractometer (Erma, Japan). Using the High Range Ammonia Colorimeter—Checker® HC HI733 (HANNA, Egypt), the ammonia was estimated. The temperature range was 26 ± 1.5 °C, dissolved oxygen was 6.5 ±0.3 mg L−1, and ammonia was 0.02 ± 0.003 mg L−1. Each tank’s water was physically sucked out every other day and replenished with fresh water.
Determination of the therapeutic dose of WPE
For determination of the therapeutic concentration of WPE, one hundred fish were divided into 10 groups and subjected to 10 grading concentrations of WPE (0, 0.5, 1, 1.5, 2, 2.5, 3, 3.5, 4, and 4.5 mg/L) for 10 days (Table ). The WPE was dissolved in 0.03% dimethyl sulfoxide (DMSO) before being added to the aquarium water. Daily siphoning of the excretory debris was conducted during the exposure period, and clinical signs and mortalities were recorded. The 0.5–1.5 mg/L range was safe for WPE concentration limits, as it did not result in any abnormal clinical signs or mortality. A concentration of 1.5 mg/L WPE was chosen as the therapeutic dosage.
Table 1. Effect of water exposure to different white poplar extract (WPE) levels on mortality and clinical signs of O. niloticus for 10 days.
Experimental design and protocol
The experimental design is shown in Figure . One hundred sixty fish were assigned into four experimental groups (4 glass tanks/group, 40 fish/group, 10 fish/tank) for ten days. In the first and second groups, 0 and 1.5 mg/L WPE were applied in tank water, respectively, without fish being challenged. In the 3rd and 4th groups, 0 and 1.5 mg/L WPE were applied in tank water, respectively, with fish intraperitoneal inoculated with 0.2 mL of A. veronii (0.5 × 107 CFU). Throughout the experiment, the fish were given a commercial meal three times a day until satisfied. Fish were kept under constant observation for any clinical symptoms or death.
Sampling
After the experiment (10 days), fish were starved for 24 h and sedated by a 100 mg/L benzocaine solution (Lugo et al. Citation2008). Blood samples were taken from the caudal vessels of 12 fish/group into two batches. The first batch was taken using a 1 mL plastic syringe and left at room temperature (23 °C) for 4 h before being centrifuged at 1750 ×g for 10 min to separate the serum. Sera was then stored at −20 °C until use in biochemical and immunological testing. A 1 mL heparinised syringe was used to collect the second batch of blood samples for the phagocytic activity assay. To analyse the hepatic oxidant/antioxidant assays, liver samples (n = 12) from the deceased fish were also taken.
Liver antioxidant indices
The hepatic content of malondialdehyde (MDA) was spectrophotometrically measured in the homogenate using a specific kit (Bio-diagnostic company, Giza, Egypt; Catalog No. MD 25 29), while the catalase activity (CAT) was measured as described by Aebi (Citation1984).
Immunological indices
The kits from Cusabio Co. (Houston, TX, USA; Catalogue no. CSB-E12045Fh) were used to measure the immunoglobulin M (IgM) serum level. Following Ghareghanipoora et al. (Citation2014), with minor changes, we assessed serum lysozyme (LYZ) activity using the lysis of Micrococcus lysodeikticus (Sigma Co., MO, USA). We combined the serum with the M. lysodeikticus solution (0.2 mg/mL in 0.05 M PBS, pH 6.2) at 25 °C for 5 min. We employed a 5010 photometer from the BM Co. in Berlin, Germany, to measure the optical density at 540 nm for 5 min at 1-min intervals using various dilutions of lyophilised chicken egg-white lysozyme (Sigma Co., MO, USA). Following Bryan and Grisham (Citation2007) methodology, nitric oxide (NO) was evaluated. Based on the methodology of Cai et al. (Citation2004), leukocytes’ phagocytic activity (PA%) was assessed.
Serum biochemical indices
The serum activities of aspartate aminotransferase (AST) and alanine aminotransferase (ALT) were measured using the kits of Biotrend Co., MD, USA (Catalog no. EK12276 and MBS038444, respectively). The creatinine level was determined as described by Fossati et al. (Citation1983). The electrophoretic distribution of serum proteins, including total proteins (TP), albumins, and globulins, was also measured (Badawi Citation1971). The serum glucose level was assessed following the technique described by Hyvarinen and Nikkila (Citation1962). The serum cortisol level was measured according to Odhiambo et al. (Citation2020).
Statistical analysis
Shapiro-Wilk’s test was used to check the data’s normality. The outcomes of the antioxidant/immune and biochemical indices were examined using a two-way analysis of variance (ANOVA) in SPSS version 18 (SPSS, Chicago, IL, USA). Tukey’s multiple range tests were used to discover differences between means (n = 12/group). The data variation was represented as pooled SEM, and the significance level was established at p < 0.05.
Results
Phytochemical composition of the plant extract
The active phytochemical compounds of WPE are presented in Table . WPE had abundant terpenoids and moderate amounts of phenolics and steroids, then low amounts of alkaloids, tannins, flavonoids, saponins, and glycosides.
Table 2. Phytochemical compounds in white poplar extract (WPE).
GC-MS results
The result of GC-MS of WPE is shown in Table and Figure . GC-MS analysis detected 17 bioactive compounds in WPE. The major substances are 9,12,15-Octadecatrienoic acid, methyl ester, (Z, Z, Z)-(19.96%), Phytol (16.67%), beta.-Amyrone (14.59%), Methyl tetradecadienoate (10.44%), Hexadecanoic acid, ethyl ester (10.08%), γ-Sitosterol (9.24%), Phosphoric acid (7.63%), Stearic acid (2.53%), β-Sitosterol, TMS derivative (2.44%), Vitamin E (1.65%).
Table 3. Bioactive compounds in white poplar extract (WPE) determined by GC-MS.
In-vitro antibacterial activity of WPE
Figure showed that WPE had an inhibition zone of 22 ± 0.45 mm against A. veronii. Meanwhile, cefotaxime gave a 24 ± 0.33 mm inhibitory zone. The MIC and MBC of WPE against A. veronii were 60 µg/mL.
Clinical signs and survival
The non-infected fish treated with 0 and 1.5 mg/L WPE exhibited normal clinical signs with a 100% survival rate. While the infected fish treated with 0 mg/L WPE displayed decreased swimming activity with a manifestation of skin haemorrhage and fin rot as well as the lowest survival rate (67.5%) (Figure ). Interestingly, the infected fish treated with 1.5 mg/L WPE retrieved the previous clinical manifestations, only for slight fin rot with enhanced survivability (90%) compared to the infected-nontreated group.
Antioxidant-immune status
The effect of WPE application on the antioxidant-immune status of O. niloticus challenged with A. veronii is shown in Table . Regardless of WPE application, challenging the fish with A. veronii resulted in detrimental effects on the antioxidant-immune status of fish, indicated by a rise in the oxidative status (increased hepatic MDA level) and reduction in the antioxidant activity (reduced hepatic CAT activity) (p < 0.01). Furthermore, the reduction in the immune status of fish is represented by lowered LYZ, NO, IgM, and PA % (p < 0.01). Regardless of A. veronii challenge, WPE enhanced both antioxidant and immune responses in fish (p < 0.01). The interaction between bacterial challenge and WPE application showed boosted antioxidant activity (p < 0.01) and immune responses (p < 0.05) of fish treated with WPE during the bacterial challenge.
Table 4. Effects of white poplar extract (WPE) application on immunological parameters of O. niloticus challenged with A. veronii.
Blood biochemical indices
Regardless of the WPE application, the challenge with A. veronii increased the hepatorenal indices (ALT, AST, and creatinine), cortisol, and glucose with a significant decline in TP and albumins (p < 0.01) with no significant effect on the serum globulin level (p > 0.05) (Table ). Regardless of A. veronii challenge, WPE application decreased the serum levels of hepatorenal indices, cortisol, and glucose and increased serum total protein and albumin levels (p < 0.01). The interaction between the bacterial infection and WPE application revealed increased ALT levels in infected fish (not treated with WPE) while decreased in fish treated with WPE during the bacterial challenge. The serum creatinine, cortisol, and glucose levels were increased in infected fish (not treated with WPE). In contrast, these levels were decreased in fish treated with WPE during the infection but still higher than in the non-infected fish either treated or not treated with WPE (p < 0.01). The opposite was observed in the serum levels of TP and albumin. The interaction did not affect Serum AST and globulin levels (p > 0.05).
Table 5. Effects of white poplar extract (WPE) application on blood biochemical parameters of O. niloticus challenged with A. veronii.
Discussion
Providing safe substitutes to antibiotics for treating bacterial pathogens in aquaculture is a must for reducing the antibiotic resistance problem. Phytotherapeutics are effective and eco-friendly ways to treat fish diseases without compromising the sustainability of the environment. Herbal extracts can be used as impressive natural alternatives to antibiotics or chemical drugs for treating fish illness, reducing drug resistance and residual accumulation problems in fish and consumers. The current study proposed a water additive (WPE) for controlling the A. veronii challenge in Nile tilapia. The group infected with A. veronii displayed decreased swimming activity, diseased signs (fin rot and skin haemorrhage), and the lowest survivability (67.5%). These outcomes might be due to the bacteria’s virulence factors (phospholipase, adhesin, and aerolysin), which possess cytotoxic and haemolytic activities; these virulence factors are held responsible for the development of such clinical manifestation (Li et al. Citation2020). Treatment of the infected fish with WPE retrieved the previous clinical manifestation, only for slight fin rot and improved survivability (90%). These results could be due to WPE antibacterial activity, which was approved by the in-vitro investigations. WPE had an inhibitory zone against A. veronii of 22 ± 0.45, with MIC and MBC of 60 µg/mL. The antibacterial activity of WPE in the current study could related to its principal constituent, ɑ-linolenic acid (9,12,15-Octadecatrienoic acid, methyl ester, (Z, Z, Z), which was previously documented to have antibacterial activity against Bacillus subtilis (Kusumah et al. Citation2020) and Mycobacterium tuberculosis (Masoko et al. Citation2016). Phytol, detected in our GC/MS analysis, had antibacterial activity against Pseudomonas aeruginosa (Lee et al. Citation2016). Previous studies showed that WPE displayed an in vitro antibacterial activity against Mycobacterium tuberculosis (Haouat et al. Citation2013), Escherichia coli, Pseudomonas aeruginosa, Staphylococcus aureus, and Enterococcus faecalis (Nassima et al. Citation2019). A previous study carried out by Abo El-Fadl et al. (Citation2022) documented that WPE has various active constituents which have antimicrobial, anti-inflammatory, antioxidant, and immunomodulatory effects.
Extreme reactive oxygen species (ROS) production can damage biomolecules, including lipids, nucleic acid, and proteins, which oxidate lipids and protein carbons (Zheng et al. Citation2019). Aeromonas veronii infection significantly decreased the hepatic CAT. It increased the hepatic MDA (lipid peroxidation marker) level. In this line, Elgendy et al. (Citation2022) observed that Nile tilapia, challenged with A. veronii showed a reduction in the antioxidants and an increase in MDA level. Herein, increased MDA levels and lowered CAT activity could be attributed to bacterial toxins’ ROS overproduction in the cell membrane, resulting in such oxidative damage (Bandeira Junior and Baldisserotto Citation2021). Treating the infected fish with WPE ameliorated the antioxidant activity compared to the infected-nontreated fish. The enhanced antioxidant status of infected fish by WPE treatment could be related to its primary compound [ɑ-linolenic acid (19.96%), phytol (16.67%), and vitamin E (1.65%)]. ɑ-linolenic acid has antioxidant potential by improving the antioxidant enzyme (CAT) and reducing the MDA level (Yuan et al. Citation2022). Also, phytol was reported to have antioxidant properties (Santos et al. Citation2013; Okpala et al. Citation2022). Vitamin E is a non-enzymatic antioxidant molecule with antioxidant properties in spotted seabass (Lateolabrax maculatus) (Li et al. Citation2023). Also, WPE contains a mixture of flavonoids and phenolic compounds that can resist oxidation, eliminate free radicals, and enhance immune function (Tian et al. Citation2019). Many medicinal plants have a strong free radicals scavenging ability because their structure contains multiple phenolic hydroxyl groups (hydrogen donors and singlet oxygen quenchers) and indirectly raises the stress resistance ability (Hoseinifar et al. Citation2017; Alagawany et al. Citation2020). Phenols and flavonoids can scavenge most oxidative fragments and other free radicals associated with many diseases (Mbokane and Moyo Citation2018).
The fish’s antioxidant defense system strongly correlates to the immune system and health condition (Zhang et al. Citation2020). LYZ is an immune protein in mucus, Plasma, and lymphoid tissue (Saurabh and Sahoo Citation2008). LYZ has a role in antibacterial activity, among other defensive processes (Maqsood et al. Citation2010). Nitric oxide is involved in the immune function of vertebrates and invertebrates (Sharma et al. Citation2010). Nitric oxide safeguards the host from bacterial or viral illnesses (Srivastava and Pandey Citation2015). IgM, the predominant immunoglobulin in teleosts, can immediately protect fish against illnesses (Magnadottir Citation2010). In addition, Blood protein levels, notably globulin, are assumed to reflect the fish’s improved nutritional condition and immunological response (Sahoo et al. Citation2021). The current study showed that A. veronii infection significantly decreased the immunological parameters (LYZ, NO, IgM, and PA %) and decreased TP, albumin, and globulins. The decreased immunological biomarker in the current experiment caused by A. veronii infection was similar to those obtained by Reyes-Becerril et al. (Citation2015), who found comparable outcomes in Pacific red snapper (Lutjanus peru) challenged with A. veronii. Treatment of the A. veronii-challenged fish with WPE improved the immune status and protein profile significantly compared to the infected-nontreated fish. The immunostimulant properties of WPE could be related to its active constituent; Phytol had immunostimulant activity by improving the serum level of LYZ and IgM in common carp (Cyprinus carpio) (Hoseini et al. Citation2021). Also, WPE has phytochemical constituents like phenols, terpenes, tannins, and alkaloids. Phenolics could inhibit pathogenic bacterial growth (Citarasu Citation2010). Terpenes may also have immune-modulating effects by promoting T and B cell activation (Harikrishnan et al. Citation2011b). Tannins had an immune-stimulatory impact on Beluga sturgeon (Huso huso) by boosting LYZ levels (Safari et al. Citation2020). In the Blunt snout bream (M. amblycephala), alkaloids, another biological component of WPE, were found to have an immune-stimulatory impact by raising IgM levels and boosting resistance to Aeromonas hydrophila (Ye et al. Citation2019).
In the current study, A. veronii infection significantly increased the serum levels of hepatorenal indices. Elgendy et al. (Citation2022) achieved similar outcomes. The elevated hepatorenal indices caused by A. veronii infection could be due to damaged hepatocytes and renal cells by A. veronii virulence components, increasing these indicators in the blood (Abd El Latif et al. Citation2019). Treatment of the infected fish with WPE ameliorated these indices compared to the infected-non-treated one. These results may be attributed to the protecting abilities of WPE. WPE may enhance cell membrane stability and protect tissues from toxic damage caused by free radicals through the antioxidant properties of its active substances, resulting in decreased levels of ALT and AST.
Cortisol and glucose are considered stress indicators in fish (Ahmed et al. Citation2022; Ibrahim et al. Citation2023). Aeromonas veronii infection elevated the serum cortisol and glucose. Water exposure of the infected fish to WPE reduced these stress biomarkers. These indicated the anti-stress and anti-inflammatory properties of WPE due to its active constituents (flavonoids, terpenes, and phenolics). Terpenes and phenols have anti-inflammatory activities (Aggarwal et al. Citation2006). Also, flavonoids decreased the level of cortisol and glucose in rainbow trout (Gesto et al. Citation2008).
Conclusions
From the obtained results, we can conclude that WPE has in vitro antibacterial activity (22 ± 0.45 mm inhibition zone, with MIC and MBC of 60 µg/mL) against A. veronii). In addition, WPE (1.5 mg/L) can be used as an antibacterial substance in fish farming to alleviate the detrimental impacts of A. veronii infection by enhancing the antioxidant/immune status of the infected fish indicated by the increase in the serum levels of CAT, LYZ, NO, IgM, and PA % and reducing MDA level. WPE application could reduce the harmful effects of A. veronii infection on the hepato-renal function and reduce stress caused by the infection. These outcomes could help reduce antibiotic usage in fish farms and alleviate Nile tilapia’s health and immune responses during A. veronii challenge. Additional studies are required to examine the effect of WPE as an affordable product for farmers at the fish farm level.
Ethical approval
The experimental protocol received ethical approval from Zagazig University’s Institutional Animal Care and Use Committee (Approval No. ZU-IACUC/2/F/136/2021). All animal experiments were carried out in accordance with the instructions outlined in ‘The Guide for the Care and Use of Laboratory Animals in Scientific Investigations’, and the study followed the relevant institutional standards. All animal experiments were carried out in accordance with the ARRIVE guidelines.
Acknowledgements
This work was supported by the Researches Supporting Project (RSP2024R36), King Saud University, Riyadh, Saudi Arabia. The authors thank Professor Rasha M. Reda for her kind help and providing the A. veronii strain. The authors thank the Aquatic Animal Medicine Department, Faculty of Veterinary Medicine, Zagazig University, for their kind help during the experimental procedures.
Disclosure statement
No potential conflict of interest was reported by the author(s).
Data availability statement
The datasets generated or analysed during the current study are not publicly available but are available upon reasonable request from the corresponding author.
References
- Abd El Latif A, Elabd H, Amin A, Eldeen AN, Shaheen A. 2019. High mortalities caused by Aeromonas veronii: identification, pathogenicity, and histopathologicalstudies in Oreochromis niloticus. Aquacult Int. 27(6):1725–1737. doi: 10.1007/s10499-019-00429-8.
- Abdel Rahman AN, Ismail SH, Fouda MM, Abdelwarith AA, Younis EM, Khalil SS, El-Saber MM, Abdelhamid AE, Davies SJ, Ibrahim RE. 2023. Impact of Streptococcus agalactiae challenge on immune response, antioxidant status and hepatorenal indices of Nile tilapia: the palliative role of chitosan white poplar nanocapsule. Fishes. 8(4):199. doi: 10.3390/fishes8040199.
- Abdel Rahman AN, Amer SA, Masoud SR, El-Saber MM, Osman A, Younis EM, Abdelwarith AA, Davies SJ, Khamis T, Ibrahim RE. 2023. Neem seed protein hydrolysate as a fishmeal substitute in Nile tilapia: effects on antioxidant/immune pathway, growth, amino acid transporters-related gene expression, and Aeromonas veronii resistance. Aquaculture. 573:739593. doi: 10.1016/j.aquaculture.2023.739593.
- Abdou Said A, Reda RM, Abd, El-Hady HM. 2021. Overview of herbal biomedicines with special reference to coriander (Coriandrum sativum) as new alternative trend for the development of aquaculture. Egypt J Aquatic Biol Fish. 25(2):539–550. doi: 10.21608/ejabf.2021.165900.
- Abo El-Fadl RE, El-Saber MM, Ahmed ME, Abd Elaziem TM, El-Sayed AA. 2022. Impact on growth and secondary metabolites in white poplar (Populus alba L.) callus using SeNPs@ Moringa. Curr Mater Sci. 15(2):175–191. doi: 10.2174/2666145414666211125093640.
- Aebi H. 1984. Catalase in vitro. Methods Enzymol. 105:121–126. doi: 10.1016/s0076-6879(84)05016-3.
- Aggarwal BB, Ichikawa H, Garodia P, Weerasinghe P, Sethi G, Bhatt ID, Pandey MK, Shishodia S, Nair MG. 2006. From traditional Ayurvedic medicine to modern medicine: identification of therapeutic targets for suppression of inflammation and cancer. Expert Opin Ther Targets. 10(1):87–118. doi: 10.1517/14728222.10.1.87.
- Ahmadifar E, Pourmohammadi Fallah H, Yousefi M, Dawood MA, Hoseinifar SH, Adineh H, Yilmaz S, Paolucci M, Doan HV. 2021. The gene regulatory roles of herbal extracts on the growth, immune system, and reproduction of fish. Animals. 11(8):2167. doi: 10.3390/ani11082167.
- Ahmed SA, Ibrahim RE, Elshopakey GE, Khamis T, Abdel-Ghany HM, Abdelwarith AA, Younis EM, Davies SJ, Elabd H, Elhady M. 2022. Immune-antioxidant trait, growth, splenic cytokines expression, apoptosis, and histopathological alterations of Oreochromis niloticus exposed to sub-lethal copper toxicity and fed thyme and/or basil essential oils enriched diets. Fish Shellfish Immunol. 131:1006–1018. doi: 10.1016/j.fsi.2022.11.013.
- Alagawany M, Farag MR, Salah AS, Mahmoud MA. 2020. The role of oregano herb and its derivatives as immunomodulators in fish. Rev Aquac. 12(4):2481–2492. doi: 10.1111/raq.12453.
- Amal MN, Koh CB, Nurliyana M, Suhaiba M, Nor-Amalina Z, Santha S, Diyana-Nadhirah KP, Yusof MT, Ina-Salwany MY, Zamri-Saad M. 2018. A case of natural co-infection of Tilapia Lake Virus and Aeromonas veronii in a Malaysian red hybrid tilapia (Oreochromis niloticus × O. mossambicus) farm experiencing high mortality. Aquaculture. 485:12–16. doi: 10.1016/j.aquaculture.2017.11.019.
- Amer SA, El-Araby DA, Tartor H, Farahat M, Goda NI, Farag MF, Fahmy EM, Hassan AM, Abo El-Maati MF, Osman A. 2022. Long-term feeding with curcumin affects the growth, antioxidant capacity, immune status, tissue histoarchitecture, immune expression of proinflammatory cytokines, and apoptosis indicators in Nile tilapia, Oreochromis niloticus. Antioxidants. 11(5):937. doi: 10.3390/antiox11050937.
- APHA. 1992. WPCF (American Public Health Association, American Waterworks Association, Water Pollution Control Federation) Standard methods for the examination of water and wastewater. 17, 1193.
- Badawi HJ. 1971. Electrophoretic studies of serum proteins of four Tilapia species (Pisces). Marine Biol. 8(2):96–98. doi: 10.1007/BF00350923.
- Bandeira Junior G, Baldisserotto B. 2021. Fish infections associated with the genus Aeromonas: a review of the effects on oxidative status. J Appl Microbiol. 131(3):1083–1101. doi: 10.1111/jam.14986.
- Bryan NS, Grisham MB. 2007. Methods to detect nitric oxide and its metabolites in biological samples. Free Radic Biol Med. 43(5):645–657. doi: 10.1016/j.freeradbiomed.2007.04.026.
- Cai WQ, Li SF, Ma JY. 2004. Diseases resistance of Nile tilapia (Oreochromis niloticus), blue tilapia (Oreochromis aureus) and their hybrid (female Nile tilapia × male blue tilapia) to Aeromonas sobria. Aquaculture. 229(1–4):79–87. doi: 10.1016/S0044-8486(03)00357-0.
- Caudullo G, De Rigo D. 2016. Populus alba in Europe: Distribution, habitat, usage and threats. European Atlas of Forest Tree Species. In: San-Miguel-Ayanz J, de Rigo D, Caudullo G, Houston Durrant T, Mauri A, editors; p. 134–135.
- CCAC. 2005. Canadian Council on Animal Care guidelines on: the care and use of fish in research, teaching and testing. Ottawa: CCAC; p. 87 [accessed 2016 Jul 18]. http://www.ccac.ca/Documents/Standards/Guidelines/Fish.pdf.
- Citarasu T. 2010. Herbal biomedicines: a new opportunity for aquaculture industry. Aquacult Int. 18(3):403–414. doi: 10.1007/s10499-009-9253-7.
- Costa JC, Neto C, Capelo J, Lousã M, Rivas-Martinez S. 2011. A global view on the riparian forests with Salix neotricha and Populus alba in the Iberian Peninsula (Portugal and Spain). Plant Biosystems-An International Journal Dealing with all Aspects of Plant Biology. 145(3):553–569.
- El-Nobi G, Hassanin M, Khalil AA, Mohammed AY, Amer SA, Montaser MM, El-Sharnouby ME. 2021. Synbiotic effects of Saccharomyces cerevisiae, mannan oligosaccharides, and β-glucan on innate immunity, antioxidant status, and disease resistance of Nile tilapia, Oreochromis niloticus. Antibiotics. 10(5):567. doi: 10.3390/antibiotics10050567.
- Elgendy MY, Shaalan M, Abdelsalam M, Eissa AE, El‐Adawy MM, Seida AA. 2022. Antibacterial activity of silver nanoparticles against antibiotic‐resistant Aeromonas veronii infections in Nile tilapia, Oreochromis niloticus (L.), in vitro and in vivo assay. Aquac Res. 53(3):901–920. doi: 10.1111/are.15632.
- FAO. 2005. International Poplar Commission Report of the 22nd Session of the Commission and of the 42nd Session of its Executive Committee. http://www.fao.org/forestry/9497-0be6293ee294ca6ff64cd8f5f570e1c1b.pdf.
- FAO. 2022. The state of world fisheries and aquaculture 2022. Towards blue transformation. The State of World Fisheries and Aquaculture (SOFIA). Rome: FAO. doi: 10.4060/cc0461en.
- Fossati P, Prencipe L, Berti G. 1983. Enzymic creatinine assay: a new colorimetric method based on hydrogen peroxide measurement. Clin Chem. 29(8):1494–1496. doi: 10.1093/clinchem/29.8.1494.
- Fussi B, Lexer C, Heinze B. 2010. Phylogeography of Populus alba (L.) and Populus tremula (L.) in Central Europe: secondary contact and hybridisation during recolonisation from disconnected refugia. Tree Genetics & Genomes. 6:439–450.
- Gesto M, Soengas JL, Míguez JM. 2008. Acute and prolonged stress responses of brain monoaminergic activity and plasma cortisol levels in rainbow trout are modified by PAHs (naphthalene, β-naphthoflavone and benzo (a) pyrene) treatment. Aquat Toxicol. 86(3):341–351. doi: 10.1016/j.aquatox.2007.11.014.
- Geyer W. 2006. Biomass production in the Central Great Plains USA under various coppice regimes. Biomass Bioenergy. 30(8–9):778–783. doi: 10.1016/j.biombioe.2005.08.002.
- Ghareghanipoora M, Akbary P, Akhlaghi M, Fereidouni M. 2014. Non-specific immune responses and immune related genes expression of rainbow trout (Oncorhynchus mykiss, Walbaum) fed Zataria multiflora boiss extract. Bull Environ Pharm Life Sci. 3(5):140.
- Goerndt ME, Mize C. 2008. Short-rotation woody biomass as a crop on marginal lands in Iowa. North J Appl For. 25(2):82–86. doi: 10.1093/njaf/25.2.82.
- Haouat AC, El Guendouzi S, Haggoud A, David S, Sqalli H, Ibnsouda S, Iraqui M. 2013. Antimycobacterial activity of Populus alba leaf extracts. J Med Plants Res. 7(16):1015–1021.
- Harikrishnan R, Balasundaram C, Heo MS. 2011a. Impact of plant products on innate and adaptive immune system of cultured finfish and shellfish. Aquaculture. 317(1–4):1–15. doi: 10.1016/j.aquaculture.2011.03.039.
- Harikrishnan R, Balasundaram C, Heo MS. 2011b. Diet enriched with mushroom Phellinus linteus extract enhances the growth, innate immune response, and disease resistance of kelp grouper, Epinephelus bruneus against vibriosis. Fish Shellfish Immunol. 30(1):128–134. doi: 10.1016/j.fsi.2010.09.013.
- Hoseini SM, Gharavi B, Taheri Mirghaed A, Hoseinifar SH, Van Doan H. 2021. Effects of dietary phytol supplementation on growth performance, immunological parameters, antioxidant and stress responses to ammonia exposure in common carp, Cyprinus carpio (Linnaeus, 1758). Aquaculture. 545:737151. doi: 10.1016/j.aquaculture.2021.737151.
- Hoseinifar SH, Zou HK, Miandare HK, Van Doan H, Romano N, Dadar M. 2017. Enrichment of common carp (Cyprinus carpio) diet with medlar (Mespilus germanica) leaf extract: effects on skin mucosal immunity and growth performance. Fish Shellfish Immunol. 67:346–352. doi: 10.1016/j.fsi.2017.06.023.
- Ibrahim RE, Elbealy MA, Salem GA, Abdelwarith AA, Younis EM, Wagih E, Elkady AA, Davies SJ, Rahman ANA. 2023. Acute mancozeb-fungicide exposure induces neuro-ethology disruption, health disorders, and immune-oxidative dysfunction in Nile tilapia (Oreochromis niloticus). Aquat Toxicol. 261:106630. doi: 10.1016/j.aquatox.2023.106630.
- Ibrahim RE, Elshopakey GE, Abdelwarith AA, Younis EM, Ismail SH, Ahmed AI, El-Saber MM, Abdelhamid AE, Davies SJ, El-Murr A, et al. 2023. Chitosan neem nanocapsule enhances immunity and disease resistance in Nile tilapia (Oreochromis niloticus). Heliyon. 9(9):e19354. doi: 10.1016/j.heliyon.2023.e19354.
- Ibrahim RE, Elshobaky G, ElHady M, Abdelwarith AA, Younis EM, Rhouma NR, Murad SK, Yassin EMM, Khamis T, Ismail SH, et al. 2024. Nelumbo nucifera synthesized selenium nanoparticles modulate the immune-antioxidants, biochemical indices, and pro/anti-inflammatory cytokines pathways in Oreochromis niloticus infected with Aeromonas veronii. Fish Shellfish Immunol. 144:109287. doi: 10.1016/j.fsi.2023.109287.
- Kusumah D, Wakui M, Murakami M, Xie X, Yukihito K, Maeda I. 2020. Linoleic acid, α-linolenic acid, and monolinolenins as antibacterial substances in the heat-processed soybean fermented with Rhizopus oligosporus. Biosci Biotechnol Biochem. 84(6):1285–1290. doi: 10.1080/09168451.2020.1731299.
- Kutsokon NK, Jose S, Holzmueller E. 2015. A global analysis of temperature effects on Populus plantation production potential. Am J Plant Sci. 06(01):23–33. doi: 10.4236/ajps.2015.61004.
- Lazado CC, Zilberg D. 2018. Pathogenic characteristics of Aeromonas veronii isolated from the liver of a diseased guppy (Poecilia reticulata). Lett Appl Microbiol. 67(5):476–483. doi: 10.1111/lam.13057.
- Lee W, Woo E-R, Lee DG. 2016. Phytol has antibacterial property by inducing oxidative stress response in Pseudomonas aeruginosa. Free Radic Res. 50(12):1309–1318. doi: 10.1080/10715762.2016.1241395.
- Li T, Raza SHA, Yang B, Sun Y, Wang G, Sun W, Qian A, Wang C, Kang Y, Shan X. 2020. Aeromonas veronii infection in commercial freshwater fish: a potential threat to public health. Animals. 10(4):608. doi: 10.3390/ani10040608.
- Li X, Sun J, Wang L, Song K, Lu K, Zhang L, Ma X, Zhang C. 2023. Effects of dietary vitamin E levels on growth, antioxidant capacity and immune response of spotted seabass (Lateolabrax maculatus) reared at different water temperatures. Aquaculture. 565:739141. doi: 10.1016/j.aquaculture.2022.739141.
- Lieke T, Meinelt T, Hoseinifar SH, Pan B, Straus DL, Steinberg CE. 2020. Sustainable aquaculture requires environmental‐friendly treatment strategies for fish diseases. Rev Aquac. 12(2):943–965. doi: 10.1111/raq.12365.
- Liu C, Wang L, Xu H, Wang S, Gao S, Ji X, Xu Q, Lan W. 2016. “One pot” green synthesis and the antibacterial activity of g-C3N4/Ag nanocomposites. Mater Lett. 164:567–570. doi: 10.1016/j.matlet.2015.11.072.
- Lugo JM, Rodriguez A, Helguera Y, Morales R, Gonzalez O, Acosta J, Besada V, Sanchez A, Estrada MP. 2008. Recombinant novel pituitary adenylate cyclase-activating polypeptide from African catfish (Clarias gariepinus) authenticates its biological function as a growth-promoting factor in low vertebrates. J Endocrinol. 197(3):583–597. doi: 10.1677/JOE-07-0555.
- Magnadottir B. 2010. Immunological control of fish diseases. Mar Biotechnol. 12(4):361–379. doi: 10.1007/s10126-010-9279-x.
- Maqsood S, Singh P, Samoon MH, Balange AK. 2010. Effect of dietary chitosan on non-specific immune response and growth of Cyprinus carpio challenged with Aeromonas hydrophila. Int Aquac Res. 2(2):77.
- Masoko P, Mabusa IH, Howard RL. 2016. Isolation of alpha-linolenic acid from Sutherlandia frutescens and its inhibition of Mycobacterium tuberculosis’ shikimate kinase enzyme. BMC Compl Altern Med. 16(1):1–8.
- Mbokane E, Moyo N. 2018. A preliminary investigation into the potential effect of Artemisia afra on growth and disease resistance in sub-adults of Oreochromis mossambicus. Aquaculture. 482:197–202. doi: 10.1016/j.aquaculture.2017.09.047.
- Nassima B, Nassima B, Riadh K. 2019. Antimicrobial and antibiofilm activities of phenolic compounds extracted from Populus nigra and Populus alba buds (Algeria). Braz J Pharm Sci. 55:e18114. doi: 10.1590/s2175-97902019000218114.
- Nguyen DTC, Van Tran T, Nguyen TTT, Nguyen DH, Alhassan M, Lee T. 2023. New frontiers of invasive plants for biosynthesis of nanoparticles towards biomedical applications: a review. Sci Total Environ. 857(Pt 2):159278. doi: 10.1016/j.scitotenv.2022.159278.
- Nik Mohamad Nek Rahimi N, Natrah I, Loh JY, Ervin Ranzil FK, Gina M, Lim SHE, Lai KS, Chong CM. 2022. Phytocompounds as an alternative antimicrobial approach in aquaculture. Antibiotics. 11(4):469. doi: 10.3390/antibiotics11040469.
- Hyvarinen A, Nikkila EA. 1962. Specific determination of blood glucose with off-toluidine. Clin Chim Acta. 7:140–143. doi: 10.1016/0009-8981(62)90133-x.
- Odhiambo E, Angienda PO, Okoth P, Onyango D. 2020. Stocking density induced stress on plasma cortisol and whole blood glucose concentration in Nile tilapia fish (Oreochromis niloticus) of lake Victoria, Kenya. Int J Zool. 2020:1–8. doi: 10.1155/2020/9395268.
- Okpala EO, Onocha PA, Ali MS. 2022. Antioxidant activity of phytol dominated stem bark and leaf essential oils of Celtis zenkeri Engl. Trends Phytoch Res. 6(2):137–144.
- Reda RM, El‐Murr A, Abd Elhakim Y, El‐Shahat W. 2022. Aeromonas veronii detection in Egyptian fish farms with summer tilapia mortality outbreaks and the role of formic acid in limiting its spread. Aquac Res. 53(3):940–956. doi: 10.1111/are.15635.
- Reid AJ, Carlson AK, Creed IF, Eliason EJ, Gell PA, Johnson PTJ, Kidd KA, MacCormack TJ, Olden JD, Ormerod SJ, et al. 2019. Emerging threats and persistent conservation challenges for freshwater biodiversity. Biol Rev Camb Philos Soc. 94(3):849–873. doi: 10.1111/brv.12480.
- Reyes-Becerril M, Angulo C, Ascencio F. 2015. Humoral immune response and TLR9 gene expression in Pacific red snapper (Lutjanus peru) experimentally exposed to Aeromonas veronii. Fish Shellfish Immunol. 42(2):289–296. doi: 10.1016/j.fsi.2014.11.002.
- Riemenschneider DE, Isebrands J, Berguson WE, Dickmann DI, Hall RB, Mohn CA, Stanosz GR, Tuskan GA. 2001. Poplar breeding and testing strategies in the north-central US: demonstration of potential yield and consideration of future research needs. For Chronicle. 77(2):245–253. doi: 10.5558/tfc77245-2.
- Safari R, Hoseinifar SH, Imanpour MR, Mazandarani M, Sanchouli H, Paolucci M. 2020. Effects of dietary polyphenols on mucosal and humoral immune responses, antioxidant defense and growth gene expression in Beluga sturgeon (Huso huso). Aquaculture. 528:735494. doi: 10.1016/j.aquaculture.2020.735494.
- Sahoo S, Banu H, Prakash A, Tripathi G. 2021. Chapter 1: Immune system of fish: an evolutionary perspective. In: Antimicrobial immune response. Rijeka: IntechOpen. doi: 10.5772/intechopen.99541.
- Santos CCMP, Salvadori MS, Mota VG, Costa LM, de Almeida AAC, de Oliveira GAL, Costa JP, de Sousa DP, de Freitas RM, de Almeida RN. 2013. Antinociceptive and antioxidant activities of phytol in vivo and in vitro models. Neurosci J. 2013:949452. doi: 10.1155/2013/949452.
- Saurabh S, Sahoo P. 2008. Lysozyme: an important defence molecule of fish innate immune system. Aquac Res. 39(3):223–239. doi: 10.1111/j.1365-2109.2007.01883.x.
- Schreck CB, Tort L. 2016. The concept of stress in fish. In: Fish physiology. Vol 35. Academic Press, Elsevier; p. 1–34. https://doi.org/10.1016/B978-0-12-802728-8.00001-1
- Sharma D, Sandur SK, Rashmi R, Maurya D, Suryavanshi S, Checker R, Krishnan S, Sainis KB. 2010. Differential activation of NF-Κb and nitric oxide in lymphocytes regulates in vitro and in vivo radiosensitivity. Mutat Res. 703(2):149–157. doi: 10.1016/j.mrgentox.2010.08.010.
- Singh A, Kumar A. 2012. Poplars and willows for increasing the livelihood and rural development: a review. Report on International Poplar Commission 24th Session, Dehradun, 29 October–2 November 2012; p. 155.
- Srivastava PK, Pandey AK. 2015. Role of immunostimulants in immune responses of fish and shellfish. Biochem Cell Arch. 15(1):47–73.
- Swain P, Nayak S, Sasmal A, Behera T, Barik S, Swain S, Mishra S, Sen A, Das J, Jayasankar P. 2014. Antimicrobial activity of metal based nanoparticles against microbes associated with diseases in aquaculture. World J Microbiol Biotechnol. 30(9):2491–2502. doi: 10.1007/s11274-014-1674-4.
- Tadese DA, Song C, Sun C, Liu B, Liu B, Zhou Q, Xu P, Ge X, Liu M, Xu X, et al. 2022. The role of currently used medicinal plants in aquaculture and their action mechanisms: a review. Rev Aquac. 14(2):816–847. doi: 10.1111/raq.12626.
- Tawfeek N, Sobeh M, Hamdan DI, Farrag N, Roxo M, El-Shazly AM, Wink M. 2019. Phenolic compounds from Populus alba L. and Salix subserrata Willd.(Salicaceae) counteract oxidative stress in Caenorhabditis elegans. Molecules. 24(10):1999. doi: 10.3390/molecules24101999.
- Tebbi SO, Debbache-Benaida N. 2022. Phytochemistry, chemical composition and therapeutic uses of Populus nigra L. aerial parts from 1991–2021 onwards: an overview. Sust Chem Pharm. 30:100880.
- Thanigaivel S, Chandrasekaran N, Mukherjee A, Thomas J. 2016. Seaweeds as an alternative therapeutic source for aquatic disease management. Aquaculture. 464:529–536. doi: 10.1016/j.aquaculture.2016.08.001.
- Tian C, Chang Y, Zhang Z, Wang H, Xiao S, Cui C, Liu M. 2019. Extraction technology, component analysis, antioxidant, antibacterial, analgesic and anti-inflammatory activities of flavonoids fraction from Tribulus terrestris L. leaves. Heliyon. 5(8):e02234. doi: 10.1016/j.heliyon.2019.e02234.
- Trease GE, Evans WC. 1989. A textbook of pharmacognosy. 13th ed. Vol. 585. London: Bailliere Tindall Ltd.
- Vila J, Marco F, Soler L, Chacon M, Figueras MJ. 2002. In vitro antimicrobial susceptibility of clinical isolates of Aeromonas caviae, Aeromonas hydrophila and Aeromonas veronii biotype sobria. J Antimicrob Chemother. 49(4):701–702. doi: 10.1093/jac/49.4.701.
- Ye Q, Feng Y, Wang Z, Zhou A, Xie S, Zhang Y, Xiang Q, Song E, Zou J. 2019. Effects of dietary Gelsemium elegans alkaloids on growth performance, immune responses and disease resistance of Megalobrama amblycephala. Fish Shellfish Immunol. 91:29–39. doi: 10.1016/j.fsi.2019.05.026.
- Yuan Q, Xie F, Huang W, Hu M, Yan Q, Chen Z, Zheng Y, Liu L. 2022. The review of alpha‐linolenic acid: sources, metabolism, and pharmacology. Phytother Res. 36(1):164–188. doi: 10.1002/ptr.7295.
- Zhang X, Sun Z, Cai J, Wang G, Wang J, Zhu Z, Cao F. 2020. Dietary supplementation with fermented Moringa oleifera leaves inhibits the lipogenesis in the liver of meat ducks. Anim Feed Sci Tech. 260:114336. doi: 10.1016/j.anifeedsci.2019.114336.
- Zhao X, Corrales M, Zhang C, Hu X, Ma Y, Tauscher B. 2008. Composition and thermal stability of anthocyanins from Chinese purple corn (Zea mays L.). J Agric Food Chem. 56(22):10761–10766. doi: 10.1021/jf8025056.
- Zheng X, Chi C, Xu C, Liu J, Zhang C, Zhang L, Huang Y, He C, He C, Jia X, et al. 2019. Effects of dietary supplementation with icariin on growth performance, antioxidant capacity and non-specific immunity of Chinese mitten crab (Eriocheir sinensis). Fish Shellfish Immunol. 90:264–273. doi: 10.1016/j.fsi.2019.04.296.