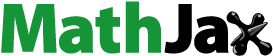
ABSTRACT
Within Malawi, and across the Global South, the adoption of stabilised soil blocks (SSBs) as a sustainable alternative to burnt clay bricks, has been hindered by the high cost of stabilisers. The purpose of this article is to evaluate the performance of cement as a stabiliser in soil stabilised blocks, and to propose cement ratios which both reduce the material cost while meeting building requirements for local applications. Using a brick production method replicable within a rural African context, we tested a range of cement contents for dry compressive strength, flexural strength, and water absorption properties. Our findings suggest that a cement content of 3.6% is adequate for single-story building applications, four times lower than that currently used in compressed brick production within the study area. Moreover, the elimination of the firing process – which represents half of burnt brick production costs – and the proposed cement ratios correspond to large cost savings, ranging from 20% to 77% depending on building application, for brick producers. These results suggest that the cost of manufacturing stabilised soil blocks can be considerably reduced and thus become financially viable; however further innovation is needed to achieve a sustainable means of production and use.
1. Introduction
With unparalleled population growth and urbanisation across the Global South, the demand for civil infrastructure, especially housing, has boomed in Africa’s growing population centres. This has resulted in an intensification of housing-related construction and the manufacture of building materials to feed the continent’s building boom (Bhairappanavar, Liu, and Shakoor Citation2021; Muboko et al. Citation2019). However, concerns over the enormous consumption of energy, materials, and water associated with the production of building materials have fuelled an emerging academic and practical conversation over the sustainability of current building practices. For instance, the manufacture of concrete produces 8.6% of global anthropogenic CO2 emissions (Miller, Horvath, and Monteiro Citation2016), while the production of cement emits 0.90 kg of CO2 per 1 kg of cement produced (Mahasenan, Smith, and Humphreys Citation2003). Moreover, the extraction of natural resources for traditional building materials, such as clay fired bricks, thatch, and timber has put severe pressure on tropical forests (Mahdjoub et al. Citation2021). To address these challenges, innovations in building technologies, including advancements in nanotechnology for structural steel, bio-receptive concrete for green facades, self-healing concrete, and recycled building materials have built demand for construction materials that are economically and environmentally sustainable (Bamigboye et al. Citation2019; Kylili and Fokaides Citation2017; Mahdjoub et al. Citation2021). Yet, while the literature focuses overwhelmingly on research done in the highly-resourced North, the greatest challenges and opportunities for innovation in the construction sector remain within the Global South (Denis Citation2020; Mulder Citation2014; Nagendra et al. Citation2018). The challenges that face the building material sector vary significantly between the North and the South, and also between diverse regions within the South. As such, it is difficult to apply existing innovations to low resourced Southern contexts, necessitating the need for contextually appropriate solutions.
Malawi is among the poorest countries in the world (Mahdjoub et al. Citation2021). Moreover, it is landlocked and densely populated, with a rapidly growing urban and rural population (Muboko et al. Citation2019; Mwathunga & Donaldson, Citation2022. Despite its challenges, it boasts a peaceful society and unspoilt natural beauty, with limitless tourism potential. The Malawian village of Chembe, located on Cape Maclear, is nestled between the south shore of Lake Malawi and the verdant, tree-covered hills of Lake Malawi National Park. Once a quiet fishing village and early mission station, Chembe has grown into the most famous tourist destinations in the country, popular for the warm lake, abundant with fish and wildlife, the quiet village setting, and the natural beauty of the surrounding National Park. Although significant potential for growth remains, tourism has already made its mark on Chembe, and the community is one of the most visited in the country. Moreover, the tourism industry has created hundreds of income-earning opportunities, drawing inhabitants from surrounding areas and encouraging young people to stay in the village rather than migrate to the city. As a result, the population of Chembe has exploded from 2,000 to over 20,000 individuals since the 1990s, many of whom work as tour guides, boat drivers, waiters, and small business owners: waged positions that are rare outside Malawi’s cities (Kalina et al. Citation2021). Furthermore, with the expansion of electricity, water, internet, and medical services to meet the needs of tourists and the expanding village, the standard of living for residents has improved substantially (Chimangeni Citation2015; Mahdjoub et al. Citation2021). The increases in tourism, population, and living standards have been mirrored by an ever-rising demand for housing and other structures, such as lodges, shops and event centres, which, as a result, spiked demand for building materials, like in cities across the Global South, but in contrast with Chembe’s peaceful rural setting. Burnt clay bricks dominate Chembe’s architecture. The few raw materials used to make burnt clay bricks include water, sand, and clay are widely available, while the aesthetics and functionality well-serve the village’s preferences for low-lying single story structures (Mahdjoub et al. Citation2021). Despite the ubiquity of the raw materials, however, the firing process used to harden clay bricks is expensive, inefficient, and requires large amounts of wood. Moreover, since Chembe is located within the protected Lake Malawi National Park, the only available supply of wood cannot be legally harvested. But with limited alternative methods to produce bricks, the harvesting of wood for brick firing from Lake Malawi National Park persists. As a result, forests within the national park are degrading rapidly, which has contributed to soil erosion, degradation of soil quality which leads to low agricultural yields, and flooding (Katumbi, Nyengere, and Mkandawire Citation2015; Nerfa and Rhemtulla Citation2019).
Stabilised soil blocks (SSBs), which do not require firing, have been introduced in Malawi, and in Chembe specifically, as an aesthetically desirable alternative to burnt clay bricks. Just like burnt clay bricks, stabilised soil blocks are made with water and clay, as well as cement which acts as a stabiliser. When combined through a compression process, the use of cement increases the strength and durability of the bricks as they cure, without the need for firing. A local vocation school was able to source a compressive machine in 2019, and has been manufacturing bricks using a cement ratio of 15%. However, due to Malawi’s landlocked status, cement is a very expensive input nationally, and even more so in Chembe due its remote location. The bricks being produced are far stronger than what is needed for local construction purposes, which consists almost entirely of single-story buildings. Moreover, the cost of the cement input has rendered the blocks financially unfeasible, with the school unable to competitively sell the blocks it manufactures. This raises the question: What cement ratios can be achieved that minimise the cement input and thus the cost of production, while still producing a strong, weather-resistant, and contextually feasible brick?
The purpose of this article is to assess the impact of cement in stabilised soil blocks on performance indicators, as a way of assessing the feasibility of SSBs as a sustainable alternative to fired bricks in Chembe. Our investigation follows in line with past literature on the production of compressed clay bricks, which shows that increased cement content corresponds with increased load-bearing capacity and durability. However, in contrast to other investigations, which have largely focused on compressed bricks that use soils with very high clay content, have an interlocking structure, or are suitable to larger building applications, our aim was to develop a product which would be contextually appropriate to Chembe, with its abundant supply of clayey sand and predominant single-story structures, while minimising cement inputs in order to reduce costs and increase potential usage. Our findings suggest that a cement content of 3.6%, which is four times lower than that currently used in compressed brick production in Chembe, is adequate for single-story building applications. The cost savings associated with using less cement make the future of building with stabilised soil blocks in Chembe more feasible, while serving as a valuable intervention towards combatting local deforestation, both in Chembe, and across Malawi, were such production methods to be more widely adopted.
2. Traditional building materials and soil stabilised bricks
Traditionally fired clay bricks are widespread as a building material in low-and-middle-income countries. In Malawi, 90% of housing is built through informal construction using traditional building materials, with a significant shift from mud walls to burnt clay brick occurring over the past two decades (Kloukinas et al. Citation2020). For people who depend on subsistence strategies for their livelihood, the production of burnt clay bricks is an attractive opportunity to earn money quickly. Few assets are needed to mix, form and fire the bricks, and the raw materials – clay, sand, and wood for firing – are inexpensive and easily accessible. Clay is particularly abundant near Chembe where a broad deposit of clay provides a seemingly inexhaustible supply of clay for local brick makers (see ). Most importantly in Chembe and other prosperous villages, however, is the aspirational value of burnt clay bricks, seen as an attainable upgrade from mud wall structures, and a sign of prosperity and continuity for a community that has relied on its quiet, rural aesthetics to attract tourists. As such, residents widely used burnt clay bricks to cultivate a traditional look in both private homes and tourist lodges, and the use of prefabricated concrete blocks has been resisted, even though they are commercially available within the village (Mahdjoub et al. Citation2021).
Yet, although burnt clay bricks are ubiquitous across Malawi, the firing process is problematic for a number of reasons. First, wood harvesting is a primary cause of deforestation (Geist and Lambin Citation2002; Muboko et al. Citation2019; Nerfa and Rhemtulla Citation2019; Ngwira and Watanabe Citation2019). In Chembe, protected woodlands in Lake Malawi National Park are the only available source of fuelwood, but are disappearing at an alarming rate (Abbot and Mace Citation1999; Katumbi, Nyengere, and Mkandawire Citation2015). Second, firing is expensive, as wood accounts for over 50% of production costs and is not burned efficiently (Alam and Starr Citation2009; Zingano Citation2005). Finally, irregularities in the firing process often result in poor quality bricks (Eires et al. Citation2012). Efforts to minimise the impact of brick firing have been largely ineffective. At-risk forests have been put under protection measures which are sometimes enforced by law enforcement patrols and penalty fines (Abbot and Homewood Citation1999; Liu et al. Citation2001). However, because the demand for wood continues to grow, restricting access to the supply has been increasingly difficult to monitor and enforce (Abbot and Mace Citation1999; Mauambeta Citation2010; Muboko et al. Citation2019). Improved kilns have been proposed to reduce the cost of wood and improve brick uniformity. However, high capital expenses, long payback periods and low government support have limited the viability of this potential solution (Alam and Starr Citation2009). In 2018, through the Sustainable Construction Materials Regulations of 2018, the Malawian government banned the use of burnt clay bricks in commercial and public building projects, in an attempt to spur the adoption of more environmentally friendly construction materials (National Construction Industry Council Citation2018). However, implementation of these measures, and a further ban on residential use, has been hampered by poor enforcement and a lack of contextually feasible alternatives across large parts of the country.
2.1. Stabilised soil blocks
Compressed earthen bricks first began appearing in scientific literature in the latter half of the 20th century, as concerns over the environmental impacts of burnt bricks escalated. The load-bearing capacity and durability of compressed earth bricks improves significantly with the addition of a stabiliser, most often cement (Eires et al. Citation2012; Guettala, Abibsi, and Houari Citation2006; Walker Citation1995), whereas bricks produced without stabilisers deteriorate quickly under adverse climatic conditions (Chan and Low Citation2010; Guettala, Abibsi, and Houari Citation2006). A significant body of literature has addressed the feasibility of using various organic alternatives to cement in SSBs such as bagasse (Alavéz-Ramírez et al. Citation2012; Dang, Fatahi, and Khabbaz Citation2016), eggshells (Oluwatuyi et al. Citation2018) rice husk ash (Moayedi et al. Citation2019; Taiwo et al. Citation2022), bone (Obianyo et al. Citation2021), and or palm bunch ash (Omoniyi Citation2019; Onyelowe Citation2019). However, these innovative additives are context specific and are feasible only when there is a consistent and affordable supply, which is not the case for Chembe which relies primarily on tourism, has only limited, subsistence farming, and intermittent electricity, which would limit the village’s ability to produce ash, if there was indeed any organic waste worth collecting. Despite the polluting effects of cement, the overall impacts of stabilised soil blocks are far less than those of burnt clay bricks, with five times lower CO2 emissions and ten times lower energy inputs (Guillaud Citation1995; Mpakati-Gama, Brown, and Sloan Citation2016; Oti and Kinuthia Citation2012; Walker Citation1995). Moreover, stabilised soil blocks use materials that are locally available, are simple to produce, and provide high thermal and acoustic insulation. In addition, stabilised soil blocks are more regular in shape than traditional burnt clay bricks, which reduces the amount of cement mortar needed in construction (Wambua and Malunga Citation2014).
Various cement ratios between 0% − 20% have been tested for compressive, flexural and tensile strength, and water absorption. These material tests have produced mixed results for interlocking or flat-surfaced bricks and for soils with varying particle size distributions, Atterberg limits, and other characteristic qualities (Chan and Low Citation2010; Danso et al. Citation2015; Eires et al. Citation2012; Guettala, Abibsi, and Houari Citation2006; Riza, Rahman, and Zaidi Citation2010; Walker Citation1995; Zingano Citation2005). In addition, the performance of cement-stabilised blocks increases with curing time, with up to a 10× increase in compressive strength from zero to 28 days (Sturm, Ramos, and Lourenço Citation2015). Although the performance of cement as a stabiliser has been highlighted, the high cost of cement in many of the contexts in which such stabilised soil blocks would be useful, has been noted as a common deterrent (Chan and Low Citation2010; Ngwira and Watanabe Citation2019; Oti and Kinuthia Citation2012).
In Malawi, the state and various NGOs have demonstrated a heightened interest in transitioning from burnt bricks to compressed earth bricks over the past decade. This interest has manifested in the creation of specific standards for Stabilised Soil Blocks, the Malawi Standard 777 for Stabilised Soil Blocks, as well as governmental proposals to locally manufacture brick presses (Eires et al. Citation2012; Ngwira and Watanabe Citation2019; Wambua and Malunga Citation2014). However, these efforts have been constrained by a lack of guidelines on how to optimise cement ratios and reduce the cost of stabilised soil blocks (Danso et al. Citation2015; Wambua and Malunga Citation2014; Zingano Citation2005). This is the specific gap this article speaks to: providing recommendations on optimal, financially feasible cement ratios for stabilised soil blocks that can be used to build single and double-story structures, and free-standing and retaining walls with low load-bearing requirements, like those that are typical in Chembe, in order to facilitate this transition to more sustainable building practices, in Malawi and across Global South contexts, where burnt clay bricks remain predominant.
3. Materials and methods
This study was inspired by the current manufacture of stabilised soil blocks by a local vocational school within Chembe. Utilising a mix of 15% cement, and approximately 80% clay soil and 5% water, they are making very strong stabilised soil blocks (290 mm in length, 140 mm in width, and 125 mm in height) that do not require firing, but as previously noted, they are unable to sell them due to the high sale price necessitated by the cost of the cement input (see ). In Mangochi District where Chembe is located, one 50 kg bag of Portland cement costs approximately 12,900 Malawian Kwacha (MK) (approximately $13 at the time of writing), but transport to Chembe from the supplier in nearby Monkey Bay adds an additional 500MK to the local price. Clay is not purchased, but rather collected from the clay seam in Chembe. Therefore, the only material cost for stabilised soil blocks comes from the cement. At a cement content of 15%, the cost of materials for each brick is approximately 418 MK. This is more than four times more expensive than a similarly sized fired brick, not even accounting for the labour costs of producing the stabilised soil blocks.
However, as we will demonstrate, the 15% cement content of these bricks far exceeds the strength and durability requirements for local building purposes. Single and double story structures with low load-bearing requirements, including lodges, shops, and homes predominate within Chembe. Lowering the cement content in the brick mix would lower production costs but also reduce the strength and durability of the bricks. The ideal cement ratio would be low enough to be economically practical, but high enough to ensure adequate brick strength and durability for relevant building requirements. Therefore, optimising the cement ratio is a key step in ensuring the viability of building with stabilised soil blocks within the community.
3.1. Material preparations
3.1.1. Soil characterisation
The strength and durability of stabilised soil bricks are influenced by the characteristics of the soil used in the brick mix. Geological formations vary widely across various regions in Malawi. Therefore, this experiment used soil from Chembe to most closely imitate the local manufacturing process. Three soil samples were collected from Chembe. The soil characterisation located in the results section is based on average values of the samples. The soil was classified according to the Unified Soil Classification System (USCS) based on the particle size distribution, liquid limit, plastic limit and plasticity index (8.4.2 Unified Soil Classification System Citationn.d.). The maximum dry densities and optimum moisture contents of the soil – mixed with incremental amounts of cement – were also determined by the Proctor compaction test. The benefits of soil compaction include increases in strength, load bearing capacity, and stability, characteristics which improve the overall performance of SSBs.
A sieve stack with the following opening sizes was used to determine the particle size distribution: 37.5 mm, 19 mm, 14 mm, 4.75 mm, 2.36 mm (No. 4), 1.18 mm, 0.6 mm, 0.425 mm, 0.3 mm, 0.212 mm, 0.150 mm, and 0.075 mm (No. 200). The percentage of particles passing the No. 4 and No. 200 sieves, as well as the Atterberg Limits, were determined to classify the soil according to the Unified Soil Classification System.
3.1.1.1. Preparation of specimens
A total of 145 bricks were manufactured in nine batches of 15 bricks each. The batches were produced with varying cement ratios of 0%, 2%, 4%, 6%, 8%, 10%, 12%, 14% and 16% by mass substitution. The brick mix was produced using class 42.5 CEM Portland cement, soil taken from Chembe Cape Maclear, and water. indicates the amounts of cement, soil, and water used in each batch, measured in percent mass. The water content added to each batch was based on the optimum moisture content determined by a Proctor test (see ).
Table 1. Material ratios of brick mix batches.
Table 2. Summary of Chembe soil characteristics.
Table 3. Maximum dry density (MDD) and optimum moisture content (OMC) of soil sample with varying cement ratios.
After mixing, the bricks were pressed in a compaction machine similar to the model being used in Chembe. The dimensions of the pressed bricks were 290 mm long, 140 mm wide, and 125 mm. Prior to testing, the bricks were cured under moist plastic sheets for 28 days.
3.2. Testing protocols
For each sample set of 15 bricks made with varying cement ratios, five were tested under compressive loading, five were tested for flexural strength, and five were tested for water absorption. The results from each sample set were averaged, with the averaged values guiding the discussion.
3.2.1. Compressive strength test
The dry compressive strength of the clay bricks was tested according to the Malawi Standard MS 777:2007. Specimens were axially loaded at a constant rate of 2 kN/min until failure. The compressive strength is given by Equation 1:
where CD is the dry compressive strength of the stabilised soil block in mega-Pascals, WD is the load applied at failure in Newtons, and A is the area on which the compressive load is applied in millimetres squared.
3.2.2. Flexural strength test
The flexural strength of the clay bricks was tested using a central-point bending strength test according to the Malawi Standard MS 777:2007. Specimens were simply supported across a span of 213 mm and transversely loaded at a constant rate of 2 kN/min until specimen failure. The flexural strength is given by Equation 2:
where S is stress in the stabilised soil block at midspan in mega-Pascals, W is the maximum load at failure in Newtons, l is the distance between the supports in millimetres, b is the width of the specimen in millimetres, and d is the depth of the specimen in millimetres.
3.2.3. Water absorption test
The water absorption of the clay bricks was tested according to the Malawi Standard MS 777:2007. After being dried to remove excess moisture, the specimens were immersed in clean water at room temperature for 24 hours. Then, specimens were weighed within three minutes after being removed from the water. The water absorption is given by Equation 3:
where AW is the water absorption as a percentage, m1 is the mass of the oven dry specimen in grams, and m2 is the mass of the saturated specimen after immersion in water in grams.
4. Results and discussion
4.1. Soil characterisation
The soil samples collected from Chembe consisted of 30.7% fine clay and silt particles (<0.075 mm), 68.6% sand particles (0.075 to 4.750 mm), and less than 1% gravel particles (>4.750 mm). summarises the soil characteristics of these samples and includes the percentage of soil types according to the particle size distribution, the Atterberg Limits, and the Proctor Test results.
shows the cumulative percentage of soil particles passing each sieve. The particle size distribution indicated that No. 4 cumulative percent finer was 95.0% and No. 200 cumulative percent finer was 30.7% ().Footnote1 The liquid limit of the sample was 29 and the plasticity index was 8.2. Thus, the soil can be classified as SC-CL, clayey sand with lean clay.
The Proctor test results for soil samples mixed with various percents of cement are displayed in . shows the optimal water content required for the preparation of specimens at each cement ratio as determined by the Proctor test results. The corresponding maximum dry density values confirm that the blocks meet the density requirement of 1600 kg/m3 as specified in the Malawi Standard for Stabilised Soil Blocks.
4.2. Brick test results
4.2.1. Compressive strength
The average dry compressive strengths of the stabilised soil blocks are illustrated in . The standard deviations from each five-specimen sample set are shown, together with the line of best fit (EquationEquation 4(4)
(4) ) and the R2 value.
Figure 5. Average dry compressive strengths of stabilised soil blocks at varying cement ratios, with error bars of the standard deviation of five samples.

Results suggest that the dry compressive strength of stabilised soil blocks increases linearly with the addition of cement for cement ratios between 0% and 16%, a finding which is supported by literature (Bahar, Benazzoug, and Kenai Citation2004; Danso et al. Citation2015). The relationship between dry compressed strengths and cement ratios can be quantified by Equation 4:
where CD is the dry compressive strength of the stabilised soil block in mega-Pascals, and x is the cement content as a percent mass. The R2 value of 0.988 indicates a strong correlation between cement content and dry compressive strength.
The Malawi Standard for Stabilised Soil Blocks specifies a minimum dry compressive strength of 2.5 MPa. Using EquationEquation 4(4)
(4) , it was determined that a cement ratio of 1.42% is required to meet the dry compressive strength specification. This ratio is lower than the findings of Bahar, Benazzoug, and Kenai (Citation2004), which correlate a cement content of approximately 3% to a dry compressive strength of 2.5 MPa. However, the soil used by Bahar et al had a clay content nearly double that of the soil from Chembe. Since compressive strength of stabilised soil blocks decreases with an increase in clay content (Walker Citation1995), the difference in results is likely due to the difference in soil and cement types used in each study.
4.2.2. Flexural strength
The average flexural strengths of the stabilised soil blocks are illustrated in .
Figure 6. Average flexural strengths of stabilised soil blocks at varying cement ratios, with error bars of the standard deviation of five samples.

Results suggest that the flexural strength of stabilised soil blocks increases linearly with the addition of cement for cement ratios between 0% and 16%. The relationship between flexural strength and cement ratios can be quantified by Equation 5:
where S is the flexural strength of the stabilised soil block in mega-Pascals, and x is the cement content as a percent mass. The R2 value of 0.9777 indicates a strong correlation between cement content and flexural strength. MS 777 specifies a minimum flexural strength of 0.5 MPa for stabilised soil blocks. Using EquationEquation 5(5)
(5) , we determined that a cement ratio of 3.52% is required to meet the flexural strength specification.
4.2.3. Water absorption
The average water absorptions of the stabilised soil blocks are illustrated in . The standard deviations from each five-specimen sample set are shown, together with the line of best fit (EquationEquation 6(6)
(6) ) and the R2 value.
Figure 7. Average water absorption of stabilised soil blocks at varying cement ratios, with error bars of the standard deviation of five samples.

Our results suggest that the percent water absorption by mass of stabilised soil blocks decreases linearly with the addition of cement for cement ratios between 2% and 16%, a finding which is consistent with Eires et al. (Citation2012). The SSB specimens with a cement ratio of 0% disintegrated during the water absorption test. The relationship between water absorption and cement ratios for cement ratios between 2–16% can be quantified by Equation 6, where AW is the water absorption of the stabilised soil block as a percent mass, and x is the cement content as a percent mass. The R2 value of 0.9526 indicates a strong correlation between cement content and water absorption.
The Malawi Standard for Stabilised Soil Blocks specifies a maximum water absorption of 15%. Results indicate that a minimum cement ratio of more than 0% but less than 2% is required to meet the water absorption specification. While the inverse linear relationship between water absorption and cement content corresponds with literature, the SSBs produced for this study indicate greater overall durability than SSBs tested by Eires et al. (Citation2012) and Guetalla et al (2006) at similar cement ratios. For example, Eires et al noted that blocks with a cement content of 3% broke down during immersion. The difference in durability between this study and other studies is not surprising, however, since brick performance can vary significantly due to a wide variety of factors including stabiliser type and content as well as clay content, particle size distribution, moisture content, compaction energy, and curing procedure (Danso et al. Citation2015).
5. Discussion
Load-bearing capacity, flexural strength, and durability were selected as indicators of brick performance for this study based on their significance in building applications for the Chembe region and their inclusion in the Malawi Standard for Stabilised Soil Blocks. Dry compressive strength is a universally accepted means of measuring the load-bearing capacity of bricks for building applications (Sturm, Ramos, and Lourenço Citation2015). Flexural strength is relevant to the seismic stability of buildings. Seismic hazards are non-negligible in Malawi, which lies along the East Africa Rift System (Eires et al. Citation2012; Kloukinas et al. Citation2020; Ngoma et al. Citation2019; Malawi Standard 820, Citation2010). Durability, measured by water absorption, is significant in areas like Chembe that experience heavy rainfall, which can lead to the erosion of poorly-manufactured earthen building materials (Danso et al. Citation2015).
Bricks require various strength and durability characteristics based on the building application. For example, bricks on the lower story of double story buildings must exhibit a higher load-bearing capacity than bricks on the upper story. The cement savings and associated cost savingsFootnote2 compare the proposed cement content with the cement content of 15% currently being used in Chembe as the baseline. For instance, bricks for single story buildings, which have a proposed cement content of 3.6%, use 1.188 kg less cement than generic bricks produced with a cement content of 15% and are therefore 318 MK cheaper to produce. The material and cost savings of manufacturing stabilised soil blocks with the proposed cement ratios are considerable. For example, bricks manufactured for a single story building are more than four times cheaper and use four times less cement than the blocks currently being produced. Therefore, manufacturing stabilised soil blocks for specific applications using the proposed cement ratios is more economically sustainable than manufacturing a generic, over-performing brick. provides proposed cement ratios for various building applications using the South African National Standards for building requirements, South African Bureau of Standards (Citation2011).
Table 4. Cement savings and associated cost savings based on building application.
Additionally, the Malawi Standard for Stabilised Soil Blocks specifies a minimum dry compressive strength of 2.5 N/mm2, a minimum flexural strength of 0.5 N/mm2, a maximum water absorption of 15%, and a minimum density of 1600 kg/m3. Based on the results obtained from compressive strength, flexural strength, and water absorption tests, we determined that a minimum cement ratio of 3.5% by mass is required to meet the MS 777 requirements. The density of blocks with a cement ratio of 3.5% is approximately equal to 2024 kg/m3, which is 27% greater than the minimum MS 777 specification of 1600 kg/m3. outlines the proposed material ratios of brick mixes for various building applications. The water content is based on the optimum moisture content for soil-cement mixes, using soil from Chembe, as determined by the soil characterisation tests. The proposed cement ratios for the building applications listed in all meet or exceed the requirements specified in the MS 777.
Table 5. Brick mix design based on building application.
These results identify flexural strength as the limiting factor for stabilised soil block performance and confirm the known low flexural strength of earthen building materials (Eires et al. Citation2012). Therefore, further research on improving the flexural strength of buildings constructed using SSBs would likely reduce the minimum cement ratio required and thus reduce the production costs of stabilised soil blocks. Improving flexural strength could include studies on the feasibility of introducing fibres into the brick mix or manufacturing bricks with holes for vertical reinforcement (Danso et al. Citation2015; Eires et al. Citation2012).
6. Conclusions and recommendations
The world’s growing population has contributed to a high demand for housing and pushed the construction sector to innovate building materials. In much of the Global South, the widespread use of burnt clay bricks has accelerated deforestation and contributed to anthropogenic CO2 emissions. With the emergence of alternative building materials, what future do stabilised soil blocks have for Malawi? How can the trade-off between material performance and cost be optimised to improve the viability of innovations in building materials for local communities?
The purpose of this article was to optimise the ratio of cement in compressed bricks made with clayey sand soils like those found in the growing tourist centre of Chembe, Malawi, and thus reduce the material costs for stabilised soil blocks. As noted, the effectiveness of cement to stabilise compressed bricks has been shown in past literature, however, most studies focused on bricks using very clayey soil or for bricks with high strength ratings for large structures. The intention was to minimise materials costs while still producing bricks that are appropriate for various building applications found in Chembe, primarily single-story structures.
The findings are consistent with the literature, which demonstrates that increasing the cement content in stabilised soil blocks improves their compressive strength, flexural strength, and durability properties. Specifically, cement contents of 3.6%, 12.2%, and 5.0% are respectively recommended for single-story, lower double-storey, and retaining wall construction in Chembe. These ratios exceed the strength and durability requirements for their respective applications, while optimising the material cost of the bricks. The proposed ratios are between 1.2 and four times lower than the current cement ratio being used in stabilised soil blocks in Chembe. In summary, the elimination of the firing process – which represents half of burnt brick production costs – and the proposed cement ratios correspond to large cost savings for brick producers who choose to adopt stabilised soil blocks in place of burnt clay bricks.
Further investigations could assess other parameters affecting the properties of stabilised soil blocks, such as the introduction of fibres or holes for vertical reinforcement to improve flexural strength, the production of interlocking blocks which eliminate the need for cement mortar, and the use of stabilisers other than cement, such as calcium carbide or lime. Finally, the availability and affordability of compression machines remains a space for innovation and a necessary intervention in order to apply these findings as broadly as possible across Malawi and similar Global South contexts.
Disclosure statement
No potential conflict of interest was reported by the author(s).
Additional information
Notes on contributors
Rachel Hershey
Rachel Hershey is a MSc candidate in the Department of Civil, Environmental and Geomatic Engineering (D-BAUG) at ETH Zürich. She holds a BSc in civil engineering from Cedarville University. Her interests are in humanitarian assistance and infrastructure development in low-and-middle-income countries, specifically in appropriate technologies for water supply and sanitation systems.
Marc Kalina
Marc Kalina is a Senior Scientist within the Chair of Global Health Engineering at ETH Zürich. As a critical human geographer, he specialises in participatory qualitative methodologies, as well as theoretically-grounded analysis around the ways in which waste and wasting are part of wider sociocultural-economic systems. His contemporary body of work has centred on human-waste relationships, particularly within South and Southern African contexts.
Innocent Kafodya
Innocent Kafodya is the Deputy Head of the Civil Engineering Department at the Malawi University of Business and Applied Sciences. He holds a PhD in Civil Engineering from the University of Johannesburg and a MSc in Structural Engineering from the Harbin Institute of Technology in China. His research has centred on Construction Materials, Earthquake Engineering and Structures and Geomechanics.
Elizabeth Tilley
Elizabeth Tilley is an Associate Professor and the Chair of Global Health Engineering at ETH Zürich. Thematically, her research centers on sanitation and waste management through a multi-disciplinary lens. Blending engineering, economics, and the social sciences, she is specifically interested in how engineered systems and technologies affect human, environmental, and societal health.
Notes
1. The D60 value of 0.538 mm was obtained by sieve analysis. D30 and D10 could not be determined due to the high percentage of particles passing through the 0.075 mm sieve.
2. The cost savings assume a 50 kg bag of cement costs 13,400 MK ($13 USD), including transportation, as of February 2022.
References
- Abbot, J. I. O., and K. Homewood. 1999. “A History of Change: Causes of Miombo Woodland Decline in a Protected Area in Malawi.” Journal of Applied Ecology 36 (3): 422–433. https://doi.org/10.1046/j.1365-2664.1999.00413.x.
- Abbot, J. I. O., and R. Mace. 1999. “Managing Protected Woodlands: Fuelwood Collection and Law Enforcement in Lake Malawi National Park.” Conservation Biology 13 (2): 418–421. https://doi.org/10.1046/j.1523-1739.1999.013002418.x.
- Alam, S. A., and M. Starr. 2009. “Deforestation and Greenhouse Gas Emissions Associated with Fuelwood Consumption of the Brick Making Industry in Sudan.” Science of the Total Environment 407 (2): 847–852. https://doi.org/10.1016/j.scitotenv.2008.09.040.
- Alavéz-Ramírez, R., P. Montes-García, J. Martínez-Reyes, D. C. Altamirano-Juárez, and Y. Gochi-Ponce. 2012. “The Use of Sugarcane Bagasse Ash and Lime to Improve the Durability and Mechanical Properties of Compacted Soil Blocks.” Construction and Building Materials 34:296–305. https://doi.org/10.1016/j.conbuildmat.2012.02.072.
- Bahar, R., M. Benazzoug, and S. Kenai. 2004. “Performance of Compacted Cement-Stabilised Soil.” Cement and Concrete Composites 26 (7): 811–820. https://doi.org/10.1016/j.cemconcomp.2004.01.003.
- Bamigboye, G. O., I. Davies, C. Nwanko, T. Michaels, G. Adeyemi, and O. Ozuor. 2019. “Innovation in Construction Materials-A Review.” IOP Conference Series: Materials Science and Engineering 640 (1): 012070. https://doi.org/10.1088/1757-899X/640/1/012070.
- Bhairappanavar, S., R. Liu, and A. Shakoor. 2021. “Eco-Friendly Dredged Material-Cement Bricks.” Construction and Building Materials 271:121524. https://doi.org/10.1016/j.conbuildmat.2020.121524.
- Chan, C.-M., and L.-P. Low. 2010. “Development of a Strength Prediction Model for “Green” Compressed Stabilised Earthbricks.” Journal of Sustainable Development 3 (3): 140. https://doi.org/10.5539/jsd.v3n3p140.
- Chimangeni, C. P. 2015. “The Benefits of Tourism from Community Perspective of Chembe Village.”
- Dang, L. C., B. Fatahi, and H. Khabbaz. 2016. “Behaviour of Expansive Soils Stabilized with Hydrated Lime and Bagasse Fibres.” Procedia Engineering 143:658–665. https://doi.org/10.1016/j.proeng.2016.06.093.
- Danso, H., B. Martinson, M. Ali, and C. Mant. 2015. “Performance Characteristics of Enhanced Soil Blocks: A Quantitative Review.” Building Research & Information 43 (2): 253–262. https://doi.org/10.1080/09613218.2014.933293.
- Denis, E. 2020. “More Urban Constructions for Whom? Drivers of Urban Built-Up Expansion Across the World from 1990 to 2015.” In Theories and Models of Urbanization: Geography, Economics and Computing Sciences, edited by D. Pumain, 235–258. Springer International Publishing. https://doi.org/10.1007/978-3-030-36656-8_13.
- Eires, R., T. Sturm, A. Camões, and L. Ramos. 2012. “Study of a New Interlocking Stabilised Compressed Earth Blocks. In Terra 2012 - XIth Int. Conf. on the Study and Conservation ofEarthern Architecture Heritage: Lima 2012, T6-024. Mechanical characterization of dry-stack interlocking compressed earth masonry. https://www.researchgate.net/publication/264267021_Mechanical_characterization_of_dry-stack_interlocking_compressed_earth_masonry
- Geist, H. J., and E. F. Lambin. 2002. “Proximate Causes and Underlying Driving Forces of Tropical DeforestationTropical Forests are Disappearing as the Result of Many Pressures, Both Local and Regional, Acting in Various Combinations in Different Geographical Locations.” BioScience 52 (2): 143–150. https://doi.org/10.1641/0006-3568(2002)052[0143:PCAUDF]2.0.CO;2.
- Guettala, A., A. Abibsi, and H. Houari. 2006. “Durability Study of Stabilized Earth Concrete Under Both Laboratory and Climatic Conditions Exposure.” Construction and Building Materials 20 (3): 119–127. https://doi.org/10.1016/j.conbuildmat.2005.02.001.
- Guillaud, H. 1995. Compressed Earth Blocks: A Publication of Deutsches Zentrum Für Entwicklungstechnologien - GATE, a Division of the Deutsche Gesellschaft Für Technische Zusammenarbeit (GTZ) GmbH in Coordination with the Building Advisory Service and Information Network - BASIN. 2: Manual of Design and Construction/Hubert Guillaud. Eschborn, Germany: Vieweg.
- Kalina, M., M. Ngcoya, B. Nkhoma, and E. Tilley. 2021. “Conceptualising Reuse in African Households: Perspectives from Chembe, Malawi.” Environment Development and Sustainability 24 (10): 12404–12426. https://doi.org/10.1007/s10668-021-01955-3.
- Katumbi, N., J. Nyengere, and E. Mkandawire. 2015. “Drivers of Deforestation and Forest Degradation in Dzalanyama Forest Reserve in Malawi.” International Journal of Science and Research 6 (889): e893.
- Kloukinas, P., V. Novelli, I. Kafodya, I. Ngoma, J. Macdonald, and K. Goda. 2020. “A Building Classification Scheme of Housing Stock in Malawi for Earthquake Risk Assessment.” Journal of Housing and the Built Environment 35 (2): 507–537. https://doi.org/10.1007/s10901-019-09697-5.
- Kylili, A., and P. A. Fokaides. 2017. “Policy Trends for the Sustainability Assessment of Construction Materials: A Review.” Sustainable Cities and Society 35:280–288. https://doi.org/10.1016/j.scs.2017.08.013.
- Liu, J., M. Linderman, Z. Ouyang, L. An, J. Yang, and H. Zhang. 2001. “Ecological Degradation in Protected Areas: The Case of Wolong Nature Reserve for Giant Pandas.” Science: Advanced Materials and Devices 292 (5514): 98–101. https://doi.org/10.1126/science.1058104.
- Mahasenan, N., S. Smith, and K. Humphreys 2003. “The Cement Industry and Global Climate Change: Current and Potential Future Cement Industry CO2 Emissions,” Greenhouse Gas Control Technologies—6th International Conference, edited by J. Gale and Y. Kaya, 995–1000. Pergamon. https://doi.org/10.1016/B978-008044276-1/50157-4.
- Mahdjoub, N., M. Kalina, A. Augustine, and E. Tilley. 2021. “Innovating Traditional Building Materials in Chembe, Malawi: Assessing Post-Consumer Waste Glass and Burnt Clay Bricks for Performance and Circularity.” International Journal of Sustainable Engineering 14 (4): 874–883. https://doi.org/10.1080/19397038.2021.1889706.
- Malawi Standard 820 Code of Practice of Design Loadings for Building. (2010).
- Manual on Subsurface Investigations (2nd Edition)—8.4.2 Unified Soil Classification System. (n.d.). Accessed February 3, 2023, from https://app.knovel.com/web/view/pdf/show.v/rcid:kpMSIE0002/cid:kt01323H93/viewerType:pdf//root_slug:manual-subsurface-investigations/url_slug:unified-soil-classification?cid=kt01323H93&b-q=astm%20d2487&include_synonyms=no&q=astm%20d2487&sort_on=default.
- Mauambeta, D. 2010. “Status of Forests and Tree Management in Malawi a Position Paper Prepared for the Coordination Union for Rehabilitation of the Environment (CURE).” https://doi.org/10.13140/2.1.3497.7926.
- Miller, S. A., A. Horvath, and P. J. M. Monteiro. 2016. “Readily Implementable Techniques Can Cut Annual CO2 Emissions from the Production of Concrete by Over 20%.” Environmental Research Letters 11 (7): 074029. https://doi.org/10.1088/1748-9326/11/7/074029.
- Moayedi, H., B. Aghel, M. M. Abdullahi, H. Nguyen, and A. Safuan A Rashid. 2019. “Applications of Rice Husk Ash as Green and Sustainable Biomass.” Journal of Cleaner Production 237:117851. https://doi.org/10.1016/j.jclepro.2019.117851.
- Mpakati-Gama, E. C., A. Brown, and B. Sloan. 2016. “Embodied Energy and Carbon Analysis of Urban Residential Buildings in Malawi.” International Journal of Construction Management 16 (1): 1–12. https://doi.org/10.1080/15623599.2015.1110274.
- Muboko, N., L. Zengeni, C. Mashapa, G. Chibememe, and E. Gandiwa. 2019. “The Social Dynamics of Illegal Wood Harvesting on Indigenous Woody Vegetation: A Case Study of Chimanimani National Park, Eastern Zimbabwe.” Open Journal of Forestry 9 (4): 381–396. https://doi.org/10.4236/ojf.2019.94021.
- Mulder, H. 2014. An analysis of the state of innovation in the South Africa construction industry. Dissertation, University of Pretoria. https://repository.up.ac.za/handle/2263/41014.
- Mwathunga, E., and R. Donaldson. 2022. “Urban Planning History of Malawi: Case Study of the Capital Lilongwe.” Planning Perspectives 37 (4): 713–733. https://doi.org/10.1080/02665433.2021.1988867.
- Nagendra, H., X. Bai, E. S. Brondizio, and S. Lwasa. 2018. “The Urban South and the Predicament of Global Sustainability.” Nature Sustainability 1 (7): 341–349. https://doi.org/10.1038/s41893-018-0101-5.
- National Construction Industry Council. 2018. Sustainable Construction Materials Regulations of 2018. Lilongwe, Malawi: National Construction Industry Council.
- Nerfa, L., and J. M. Rhemtulla. 2019. “Changes in Tree Species Diversity, Composition and Aboveground Biomass in Areas of Fuelwood Harvesting in Miombo Woodland Ecosystems of Southern Malawi.” Forests, Trees and Livelihoods 28 (3): 176–193. https://doi.org/10.1080/14728028.2019.1621777.
- Ngoma, I., I. Kafodya, P. Kloukinas, V. Novelli, J. Macdonald, and K. Goda. 2019. “Building Classification and Seismic Vulnerability of Current Housing Construction in Malawi.” Malawi Journal of Science & Technology 11 (1): Article 1. https://doi.org/10.4314/mjst.v11i1.
- Ngwira, S., and T. Watanabe. 2019. “An Analysis of the Causes of Deforestation in Malawi: A Case of Mwazisi.” Land 8 (3): 48. https://doi.org/10.3390/land8030048.
- Obianyo, I. I., A. A. Mahamat, E. N. Anosike-Francis, T. T. Stanislas, Y. Geng, K. C. Onyelowe, S. Odusanya, A. P. Onwualu, A. B. O. Soboyejo, and R. F. Colmenares. 2021. “Performance of Lateritic Soil Stabilized with Combination of Bone and Palm Bunch Ash for Sustainable Building Applications.” Cogent Engineering 8 (1): 1921673. https://doi.org/10.1080/23311916.2021.1921673.
- Oluwatuyi, O. E., B. O. Adeola, E. A. Alhassan, E. S. Nnochiri, A. E. Modupe, O. O. Elemile, T. Obayanju, and G. Akerele. 2018. “Ameliorating Effect of Milled Eggshell on Cement Stabilized Lateritic Soil for Highway Construction.” Case Studies in Construction Materials 9:e00191. https://doi.org/10.1016/j.cscm.2018.e00191.
- Omoniyi, T. E. 2019. “Potential of Oil Palm (Elaeisguineensis) Empty Fruit Bunch Fibres Cement Composites for Building Applications.” AgriEngineering 1 (2): 153–163. Article 2. https://doi.org/10.3390/agriengineering1020012.
- Onyelowe, K. C. 2019. “Nanosized Palm Bunch Ash (NPBA) Stabilisation of Lateritic Soil for Construction Purposes.” International Journal of Geotechnical Engineering 13 (1): 83–91. https://doi.org/10.1080/19386362.2017.1322797.
- Oti, J. E., and J. M. Kinuthia. 2012. “Stabilised Unfired Clay Bricks for Environmental and Sustainable Use.” Applied Clay Science 58:52–59. https://doi.org/10.1016/j.clay.2012.01.011.
- Riza, F. V., I. A. Rahman, and A. M. A. Zaidi 2010. A Brief Review of Compressed Stabilized Earth Brick (CSEB). 2010 International Conference on Science and Social Research (CSSR 2010), 999–1004. https://doi.org/10.1109/CSSR.2010.5773936.
- South African Bureau of Standards. (2011). SANS 10400-K: The Application of the National Building Regulations Part K: Walls. http://archive.org/details/za.sans.10400.k.2011.
- Sturm, T., L. F. Ramos, and P. B. Lourenço. 2015. “Characterization of Dry-Stack Interlocking Compressed Earth Blocks.” Materials and Structures 48 (9): 3059–3074. https://doi.org/10.1617/s11527-014-0379-3.
- Taiwo, L. A., I. I. Obianyo, A. O. Omoniyi, A. P. Onwualu, A. B. O. Soboyejo, and O. O. Amu. 2022. “Mechanical Behaviour of Composite Produced with Quarry Dust and Rice Husk Ash for Sustainable Building Applications.” Case Studies in Construction Materials 17:e01157. https://doi.org/10.1016/j.cscm.2022.e01157.
- Walker, P. J. 1995. “Strength, Durability and Shrinkage Characteristics of Cement Stabilised Soil Blocks.” Cement and Concrete Composites 17 (4): 301–310. https://doi.org/10.1016/0958-9465(95)00019-9.
- Wambua, B. N., and E. P. Malunga. 2014. “Infrastructural Development Using Stabilized Soil Blocks as a Tool for Climate Change Mitigation and Sustainable Development in Malawi.” Asian Journal of Engineering and Technology 2 (4): 346–355.
- Zingano, B. W. 2005. “The Problem of Fuel Wood Energy Demand in Malawi with Reference to the Construction Industry.” https://www.semanticscholar.org/paper/The-Problem-of-Fuel-Wood-Energy-Demand-in-Malawi-to-Zingano/a306c5747bcb840416c39ef3d8eb699c68ce345c.