ABSTRACT
The use of engineered nanoparticles (NPs) in industrial and household applications will very likely lead to the release of such materials into the environment. Assessing the risks of these NPs in the environment requires an understanding of their mobility, reactivity, ecotoxicity, and persistency. Today there is a growing need for alternative and clean fuels that can replace our growing dependence on fossil fuels for vehicular propulsion. One such fuel is hydrogen, the most abundant element in the universe. It also creates no pollutants when burned as a fuel. However, due to its explosiveness, an efficient storage method is needed for hydrogen to become a replacement for gasoline. We study the structural, properties thermodynamic of absorption H2 on nanocrystalline silicon germanium films (Si/Ge) and across in length Si/Ge nanotube by calculation computed in different temperatures. Carbon nanotubes are a possible solution to the storage of hydrogen in fuel cell–powered vehicles due to their low density, high strength, and hydrogen adsorption characteristics. Carbon nanotubes (CNTs) are well-ordered, high-aspect-ratio allotropes of carbon. Density function theory (DFT) method was carried out to investigate the H2 adsorption in the (4,4) armchair single-walled carbon nanotube (SWCNT) in different temperatures. Current work surrounding hydrogen storage in carbon nanotubes is aimed at investigating the mechanisms of hydrogen adsorption into carbon nanotubes by GUSSIAN 03 Package program.
INTRODUCTION
Particles in the nano-size range have been present on earth for millions of years and have been used by mankind for thousands of years. Soot for instance, as part of the Black Carbon continuum, is a product of the incomplete combustion of fossil fuels and vegetation; it has a particle size in the nanometer–micrometer range and therefore falls partially within the nanoparticle (NP) domain. Pollutants from such diverse sources as oil and chemical spills, pesticide and fertilizer runoff, abandoned industrial and mining sites, and airborne gaseous and particulate matter from automobiles exacerbate the situation on a daily basis.[ Citation 1 ] The forecasted huge increase in the manufacture and use of NPs makes it likely that increasing human and environmental exposure to NPs will occur. As a result, NPs are beginning to come under scrutiny and the discussion about the potential adverse effects of NPs has increased steadily in recent years.[ Citation 2 , Citation 3 , Citation 4 , Citation 5 ]
Nanoscale materials find use in a variety of different areas such as electronic, biomedical, pharmaceutical, cosmetic, energy, environmental, catalytic, and material applications. Because of the potential of this technology, there has been a worldwide increase in investment in nanotechnology research and development.[ Citation 6 ]
Careful comparison of silicon and germanium chemical properties, however, shows subtle but indisputable differences, with Ge exhibiting distinct lithopile, siderophile, or organophile behavior depending on its environment. These specific properties are responsible for Ge geochemical cycles in the ocean and continental environments not being simple analogues of corresponding silica cycles.[ Citation 7 ] This requires Ge to be enriched in the secondary phases that form during the weathering of primary silicates.
One such fuel is hydrogen, the most abundant element in the universe. It also creates no pollutants when burned as a fuel. However, due to its explosiveness, an efficient storage method is needed for hydrogen to become a replacement for gasoline. The four original technologies are being explored for hydrogen storage[ Citation 8 ]: compressed gas, liquefaction metal hydrides, and physisorption.[ Citation 9 ] Carbon nanotubes are a possible solution to the storage of hydrogen in fuel cell–powered vehicles due to their low density, high strength, and hydrogen adsorption characteristics. Current work surrounding hydrogen storage in carbon nanotubes is aimed at investigating the mechanisms of hydrogen adsorption into carbon nanotubes.
It has been proposed that hydrogen can be adsorbed by carbon nanotubes () in two ways, physisorption and chemisorption. Physisorption of hydrogen occurs in carbon nanotubes by trapping hydrogen molecules inside the cylindrical structure of the nanotube or by trapping hydrogen in the interstitial sites between nanotubes.
Its results are completed to Si/Ge (nanofilm, nanotube) that Si/Ge is used for the adsorption of gases that is to form nanofilm and nanotube (). It is important to study the e-beam-induced phenomena in silica-based glasses because germanium-doped silica is one of the most used optical materials for production of integrated optical devices in which e-beam writing is foreseen. The integrated circuit fabrication by direct e-beam writing offers several advantages compared to the traditional photolithography techniques, in particular its large flexibility, which allow the mastering of section of refractive index waveguides.[ Citation 7 ]
We used the B3LYP method for calculating proportion thermodynamic by density function theory (DFT) for them. The basis set used 6-31G by GUSSIAN 03 Package.
CALCULATION DETAILS
We have performed density function theory (DFT) calculations using the Gaussian 03 revision B.03 package. In DFT, the energy has the form
RESULTS AND DISCUSSION
Of the various types of carbon nanotubes producible, single-walled nanotubes (SWNTs) are the simplest, being but a single graphite plane rolled into a thin tube. SWNTs have large theoretical surface areas that are on the order of those for high-surface-area activated carbons.[ Citation 4 ] Activated carbons have been shown to have very high hydrogen adsorption capabilities, higher than those of carbon nanotubes in many cases.[ Citation 8 ] Hydrogen can be stored in bundles of SWNTs, where H2 molecules are physisorbed at the exterior surfaces of SWNTs, which interstitial spaces between SWNTs are separating the intertube distances by high pressure of hydrogen gas.[ Citation 11 ] At low-pressure adsorption of hydrogen on SWNT, material saturates like that of the saran carbon, scaled by the surface area of the ropes. At pressures above 40 bar, however, the isotherms indicate that the SWNT material undergoes a transition to a new state of hydrogen coverage.[ Citation 12 ] This is consistent with the hydrogen permeating into the ropes, separating them into individual SWNT, with full exposure of their outer and passing in SWNT, and physisorbing onto the carbon surfaces. The thermodynamic properties are summarized in .
TABLE 1 The thermodynamic properties of H2 adsorption on surface and passing in SWNT (4,4)
This high-density phase must involve tube decohesion, because the hydrogen coverage is high and the attractive van der Waals. The thermodynamic driving force for this tube decohesion is the high chemical potential of the hydrogen gas at high pressure.
In , the hydrogen molecules that adsorb on the surface of the SWNT undergo an increase in chemical potential. The equality of chemical potential in two-phase equilibrium requires the reduction of the chemical potential of hydrogen gas in the high-capacity phase to be equal to the loss of van der Waals cohesive energy.
When hydrogen adsorbs to a SWNT material, there are different geometries possible. During chemisorption, the hydrogen molecules will exothermically adsorb to the top sites of carbon atoms on the tube wall, forming an arch-type geometry, essentially hydrogen atoms bonded to every carbon atom on the outside of the tube wall.
Another stable geometry occurs when molecular hydrogen is stored in the empty space inside a carbon nanotube. At pressures mean 10 bar, simultaneously H2 is adsorbed and is passed in SWNT; thermodynamic properties of these interactions are shown in . shows the total energy (MJ/mol) of adsorption and passing simultaneously H2 molecules. Excessive hydrogen storage will result, we conclude that these repulsive energies determine the maximum storage capacity of hydrogens inside nanotubes and the stability of the tubes.
TABLE 2 The thermodynamic properties of adsorption and passing simultaneously H2 in SWNT (4,4)
This suggests that Li may act as a catalytic active center for the H2 dissociative adsorption. The dissociated hydrogen atoms may spill over from Li sites to the carbon network, and finally become bonded to carbon atoms.[ Citation 12 ]
Efficient hydrogen storage in carbon nanotubes requires control of tube size, pressure, and temperature. With current technology it is possible to obtain solid carbon nanotubes with small distributions of tube size. Pressure and temperature remain as the two main control factors that need to be addressed.[ Citation 13 ]
Hydrogen is an elementary adsorption and at the same time it plays an important technological role. However, despite of intensive experimental and theoretical study of hydrogen interaction with a Si/Ge surface, the details of mechanisms of H2 adsorption and adsorption from a Si/Ge surface remain contradictory.
It is found that the binding energy of the physisorbed H2 on Si/Ge nanofilm surface is weak, and it can increase significantly in a nanotube. The character of the binding dramatically changes when passing molecular H2 functionalizes through it, as shown in . Out of two adsorbed H2, the first one can be adsorbed dissociatively and the second one is chemisorbed molecularly. The nature of binding has a very weak physisorption in the third adsorbed H2. The Si/Ge, also, promotes the chemisorptions of H2 with smaller binding energy. The present results reveal the important effect of passing the molecular H2 through nanotube. These results will increase our understanding of the molecular and dissociative adsorption of hydrogen ().
TABLE 3 The thermodynamic properties of H2 adsorption on surface Si/Ge and passing in Si/Ge nanotube
FIGURE 5 The total energy (MJ/mol) for H2 adsorption on surface Si/Ge and passing in Si/Ge nanotube.
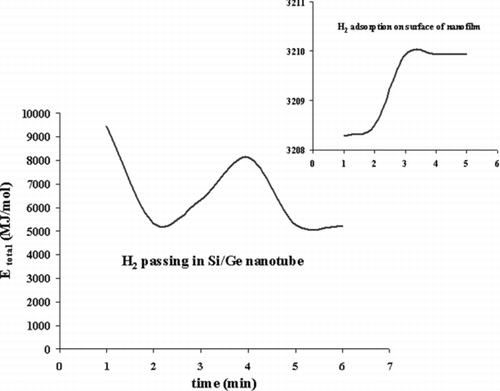
On the other hand, this method of increasing hydrogen adsorption is feasible and possible at ambient temperature. This storage capacity is well above the goals of the Department of Energy (DOE) Hydrogen Plan. Single-walled carbon nanotubes offer better adsorption properties than that of other nanotubes because of their maximum in surface area. Because they can now be produced with such a low distribution of tube size, they provide a good foundation for future research into the application of carbon nanotubes for hydrogen fuel storage. Research into this area could lead to very efficient and affordable fuel cells that would be applicable to the automotive industry.
CONCLUSIONS
Carbon nanotubes are a promising material for use in fuel cells and hydrogen storage. However, all mechanisms and condition of hydrogen adsorption onto carbon nanotubes are not yet clearly understood. At this point it is not economically feasible to produce fuel cells utilizing carbon nanotubes, but results indicate that they may not be far away. The main areas of research needed to advance this material into widespread use or the trash can, consist of tube size, doping agents, temperature affects on hydrogen adsorption and desorption, and the affects of pressure on hydrogen adsorption and desorption.
Nanotubes are stronger than steel but very flexible and lightweight. These exceptional mechanical characteristics make them suitable as “tips” for scanning probe microscopes or enforcing fibers for nanotube–polymer composite materials.[ Citation 14 ]
Depending on the construction, nanotubes can behave like electrical conductors or semiconductors, which makes them extremely useful for nanoscale electronics applications. The electronic characteristics of carbon nanotubes are strongly coupled to the mechanical properties and provide great opportunity to develop novel electromechanical nanodevices.[ Citation 15 ]
In this work, we present a detailed analysis of the H2 adsorption and passing on CNT and Si/Ge nanotube. These calculations are done by DFT by GUSSIAN 03 Package program.
We found that the binding energy between H2 molecule and outer surface of a bare Si/Ge is very weak and the physisorption bond can easily be broken. The H-H interaction when absorbed on the surface is weakened, and, therefore, the results have shown that the bond in the molecular interaction of H2 is relatively stronger with a shorter bond length, so energy changes are regular when passing H2 through nanotube. The binding energy would increase if H2 molecule were directly attached to Si/Ge surface. However, the case with the Si/Ge atom leads to a relative weaker interaction and smaller binding energies.
REFERENCES
- Maynard , A. D. 2006 . Nanotechnology: A Research Strategy for Addressing Risk; , Washington , DC : Woodrow Wilson International Center for Scholars .
- Obare , S. O. and Meyer , G. J. 2004 . Nanostructured materials for environmental remediation of organic contaminants in water . J. Environ. Sci. Health Part A , 39 : 2549 – 2582 .
- Nowack , B. D. and Bucheli , T. 2007 . Occurrence, behavior and effects of nanoparticles in the environment . Environ. Pollut. , 150 : 5 – 22 .
- Oberdörster , G. , Oberdörster , E. and Oberdörster , J. 2005 . Nanotoxicology: an emerging discipline evolving from studies of ultrafine particles . Environ. Health Perspect. , 113 : 823 – 839 .
- Nel , A. , Xia , T. , Mädler , L. and Li , N. 2006 . Toxic potential of materials at the nanolevel . Science , 311 : 622 – 627 .
- Guzman , K. A. D. , Taylor , M. R. and Banfield , J. F. 2006 . Environmental risks of nanotechnology: national nanotechnology initiative funding, 2000–2004 . Environ. Sci. Technol. , 40 : 1401 – 1407 .
- Monajjemi , M. , Mahdavian , L. and Mollaamin , F. 2008 . Characterization of nanocrystalline Silicon Germanium film and nanotube in adsorption gas by: Monte Carlo and Langevin Dynamic simulation . Bull. Chem. Soc. Ethiop. , 22 : 1 – 10 .
- Chan , S. P. , Chen , G. , Gong , X. G. and Liu , Zh. F. 2001 . Chemisorption of hydrogen molecules on carbon nanotubes under high pressure . Phys. Rev. Lett. , 87 : 205502 – 205506 .
- Rzepka , M. , Lamp , P. and de la Casa-Lillo , M. A. 1998 . Physisorption of hydrogen on microporous carbon and carbon nanotubes . J. Phys. Chem. B , 102 : 10894 – 10898 .
- Accelrys Inc. 2001 . Materials Studio CASTEP , San Diego : Accelrys Inc. .
- Lee , S. M. , An , K. H. , Lee , Y. H. , Seifert , G. and Frauenheim , T. 2001 . A hydrogen storage mechanism in single-walled carbon nanotubes . J. Am. Chem. Soc. , 123 : 5059 – 5063 .
- Ye , Y. , Ahn , C. C. , Witham , C. , Fultz , B. , Liu , J. , Rinzler , A. G. , Colbert , D. , Smith , K. A. and Smalley , R. E. 1999 . Hydrogen adsorption and cohesive energy of single-walled carbon nanotubes . Appl. Phys. Lett. , 74 : 2307 – 2309 .
- Chen , P. , Wu , X. , Lin , J. and Tan , K. L. 1999 . High H2 uptake by alkali-doped carbon nanotubes under ambient pressure and moderate temperatures . Science , 285 : 91 – 93 .
- Environmental Futures Research in Nanoscale Science, Engineering and Technology, Science To Achieve Results (STAR) Program, National Center for Environmental Research . http://es.epa.gov/ncer/rfa/archive/grants/02/02nanotech.html
- Golberg , D. , Dorozhkin , P. , Bando , Y. , Hasegawa , M. and Dong , Z. C. 2002 . Semiconducting B–C–N nanotubes with few layers . Chem. Phys. Lett. , 359 : 220 – 228 .