ABSTRACT
The gut microbiota and bile acid metabolism are key determinants of the response of inflammatory bowel disease to biologic therapy. However, the molecular mechanisms underlying the interactions between the response to anti-α4β7-integrin therapy and the gut microbiota and bile acid metabolism remain unknown. In this research, we investigated the role of gut microbiota-related bile acid metabolism on the response to anti-α4β7-integrin therapy in a humanized immune system mouse model with colitis induced by 2,4,6-trinitrobenzene sulfonic acid. We found that anti-α4β7-integrin significantly mitigated intestinal inflammation, pathological symptoms, and gut barrier disruption in remission-achieving colitis mice. Whole-genome shotgun metagenomic sequencing demonstrated that employing baseline microbiome profiles to predict remission and the treatment response was a promising strategy. Antibiotic-mediated gut microbiota depletion and fecal microbiome transplantation revealed that the baseline gut microbiota contained common microbes with anti-inflammatory effects and reduced mucosal barrier damage, improving the treatment response. Targeted metabolomics analysis illustrated that bile acids associated with microbial diversity were involved in colitis remission. Furthermore, the activation effects of the microbiome and bile acids on FXR and TGR5 were evaluated in colitis mice and Caco-2 cells. The findings revealed that the production of gastrointestinal bile acids, particularly CDCA and LCA, further directly promoted the stimulation of FXR and TGR5, significantly improving gut barrier function and suppressing the inflammatory process. Taken together, gut microbiota-related bile acid metabolism-FXR/TGR5 axis may be a potential mechanism for impacting the response to anti-α4β7-integrin in experimental colitis. Thus, our research provides novel insights into the treatment response in inflammatory bowel disease.
Introduction
Inflammatory bowel disease (IBD), including Crohn’s disease (CD) and ulcerative colitis (UC), is a life-limiting and lifelong, relapsing and remitting inflammatory disorder that affects the intestine.Citation1 Although the etiology of IBD is unidentified, environmental and genetic factors render individuals susceptible.Citation2,Citation3 To date, over 250 host susceptibility sites have been found in genomics studies of IBD.Citation4–6 In addition to these genetic sites, environmental variables and other host factors are also significant in the pathogenesis and development of particular phenotypes that affect the response of IBD to treatment.Citation5
There are numerous novel pharmacological targets in IBD,Citation7,Citation8 and emerging therapeutic techniques indicate that an early therapy initiation favors a better response.Citation9 Personalizing therapies to determine the best strategy for disease management is essential in IBD, as each subsequent treatment may result in a reduced likelihood of a response.Citation8 The mainstay treatment for moderate-to-severe IBD patients is anti-tumor necrosis factor (TNF) therapy; however, non-response to this biologic therapy in IBD patients remains a dilemma, with a documented incidence of 30%.Citation10 Biomarkers that affect the response to anti-TNF are currently being researched, which may include the use of clinical indicators such as age, body mass index, or disease duration.Citation11 Some promising biomarkers, such as oncostatin M, Il13RA2, and TREM-1, have also been discovered but have not been employed in the clinic.Citation12–14 Recently, anti-α4β7-integrin, which blocks leukocyte trafficking, has become widely available, revolutionizing our capacity for achieving remission and reducing the morbidity rate.Citation15 Until now, the only anti-α4β7-integrin medication authorized for the induction and maintenance therapy for IBD is vedolizumab (VDZ).Citation16 VDZ has been demonstrated to prevent lymphocyte homing by inhibiting the transmembrane protein α4β7-integrin, which is required for lymphocyte attachment and motility to the intestinal endothelium.Citation17 Excitingly, a recent study revealed that VDZ outperformed anti-TNF therapy (adalimumab) in a comparison of 52-week clinical response rates.Citation18 Despite a superior initial response, only a small number of patients experienced persistent remission. Moreover, when it comes to randomized controlled trials or observational cohort studies, individual clinical variables do not have a high enough predictive value to evaluate response to this therapy, and no significant model building has been done to improve response to this mechanism of action.
Throughout the past decade, the pivotal role of the intestinal microbiome has been emphasized in numerous immune-mediated disorders.Citation3,Citation19–21 In IBD, the diversity of gut microbiota decreased, pro-inflammatory bacteria (i.e., Enterobacteriaceae and Fusobacteriaceae) increased, and anti-inflammatory bacteria (i.e., Firmicutes) decreased.Citation3,Citation19,Citation21 Recently, the baseline gut microbiota was used to predict the treatment response in a prospective study of IBD patients receiving anti-α4β7-integrin as an initial treatment, and the researchers discovered that remission-achieving CD patients at week 14 had higher baseline levels of Roseburia inulinivorans and Burkholderiales sp.Citation22 Additionally, community α-diversity among remission-achieving CD patients was markedly higher, but β-diversity was lower among responders at baseline. In short, the differences in the baseline gut microbiota are related to the manner in which IBD patients respond to anti-α4β7-integrin therapy.
Furthermore, in IBD patients, alterations in the gut microbiota have been linked to variations in the concentration and diversity of metabolites, such as bile acids (BAs).Citation23–26 BAs are a kind of unique chemical property of steroids that are mostly formed by the breakdown of cholesterol.Citation27 Resident bacteria in the intestinal tract convert primary BAs into secondary BAs during digestion, which unequivocally shows that intestinal dysbiosis linked to IBD primarily influences the gut microbiota and BA metabolism. Moreover, functional characterizations and gene-wide association analyses have revealed that impaired BA signaling pathways are pathogenic in the progression of IBD.Citation28 Interestingly, in UC patients who achieved remission, the secondary BAs increased dramatically following fecal microbiota transplantation (FMT), indicating that BA metabolic pathways correlated to microbial diversity were involved in promoting remission.Citation29
Additionally, the primary and secondary BAs are targeted and stimulated by various receptors, such as FXR and TGR5. The majority of BA-triggered receptors are broadly distributed in the digestive tract, and the expression of these receptors is influenced by the gut microbiota, which is drastically altered during colitis.Citation30 Animals lacking the nuclear receptor FXR or the membrane receptor TGR5 are more susceptible to colitis, indicating that BA-triggered receptors are essential for sustaining intestinal homeostasis.Citation31,Citation32 Therefore, the stimulation of FXR/TGR5 signaling pathways through BAs can alleviate the clinical symptoms of IBD.
Following the information provided above, we hypothesized that gut microbiota-related BA metabolism-FXR/TGR5 axis might contribute to the development of a comprehensive predictive model for the response of IBD to anti-α4β7-integrin therapy. We used the humanized mouse model of colitis induced by 2,4,6-trinitrobenzene sulfonic acid (TNBS) and combined metagenomics and metabolomics to investigate the treatment response. Our results revealed that anti-α4β7-integrin could markedly improve pathological symptoms, intestinal inflammation, and gut barrier disruption in remission-achieving colitis mice. Whole-genome shotgun metagenomic sequencing demonstrated that baseline microbiome profiles could be used to predict remission and response to anti-α4β7-integrin in colitis. Subsequently, the gut microbiota depletion caused by antibiotics and FMT in pseudo-germ-free (PGF) mice from clinical donors showed that the response to anti-α4β7-integrin was impacted by focusing on the gut microbiota. At the same time, the targeted metabolomics analysis showed that BAs correlated to microbial diversity were involved in the remission of colitis. Furthermore, the activation effects of the microbiome and BAs on FXR, TGR5, and their downstream genes were evaluated in colitis mice and Caco-2 cells. Taken together, our research revealed that gut microbiota-related BA metabolism-FXR/TGR5 axis may be a potential mechanism for impacting response to anti-α4β7-integrin therapy in IBD.
Materials and methods
Humanized immune system mouse model
Since VDZ only affects immunological cells from humans, we utilized the humanized immune system mouse model for our investigation. Female NOD.Cg-PrkdcscidIl2rgem1Smoc mice were reconstituted using human CD34+ hematopoietic stem cells by Shanghai Model Organism Center, Inc. (Shanghai, China) and sent to our facilities following verification of effective engrafting and existence of the humanized immune system. Thus, the well-established mouse model [huHSC-(M-NSG)] has a humanized immune system and an implanted population of human immune cells.Citation33 The mice were given 7 days to acclimate on arrival. Mice were housed in separate ventilated cages in groups of five in a specified pathogen-free environment with access to food and water ad libitum. The experiments were carried out in accordance with the Animal Experiment Guidelines of Guangxi Medical University (SCXK-2020–0003) and approved by the Animal Care and Welfare Committee (Approval No. GXMU202204090).
Chemicals and reagents
TNBS (P2297–10 ml) was obtained from Sigma-Aldrich (MO, USA). VDZ (28713–300 mg) was purchased from Takeda Pharmaceuticals (Tokyo, Japan). Vancomycin, metronidazole, ampicillin, and neomycin sulfate were obtained from Solarbio Technology (Beijing, China; purity>95%). All BA standards were obtained from Shanghai ZZBIO (Shanghai, China; purity>95%). Fetal bovine serum, DMEM/F12, and TRIzol reagent were purchased from Thermo Fisher Scientific (MA, USA). All reagents for protein measurement and extraction were purchased from Beyotime Biotechnology (Shanghai, China).
Experimental colitis and drug administration
The following were the three animal experimentation procedures:
In the interest of determining the response to anti-α4β7-integrin in IBD, the humanized mice were assigned at random to the control (n = 10), model (n = 10), and VDZ-treated groups (n = 20). Colitis was induced in the mice by TNBS, as described previously.Citation34 Briefly, after pre-sensitizing the shaved skin with 150 μl of 1% (wt/v) TNBS-olive oil-acetone solution, 100 μl of 2.5% (wt/v) TNBS in 50% ethanol solution was injected into the colon of 24-hour starved mice 7 days later. Healthy mice received an equal amount of phosphate-buffered saline (PBS). The VDZ was injected intraperitoneally at 50 mg/kg 2 days prior to the first day of TNBS induction and at 30 mg/kg on days 0 and 4 of the trial, according to the previous report.Citation35 Due to the delayed effects shown after receiving VDZ in the clinic, pretreatment was administered. The control group and model group received the same volume of PBS. Finally, according to whether pathological symptoms, intestinal inflammation, and gut-barrier disruption improved, the VDZ-treated colitis mice were divided into the remission and non-remission groups. Stool samples at baseline were collected for whole-genome shotgun metagenomic sequencing and targeted metabolomics analysis before starting the treatment with VDZ. Mice were euthanized under anesthesia, and the pertinent samples were gathered on the 7th day.
To verify whether intestinal microbiome was involved in the response to anti-α4β7-integrin in IBD, the PGF mouse model was developed by giving the antibiotic cocktail (ABX) containing vancomycin (0.5 g/L), ampicillin (1 g/L), metronidazole (1 g/L), and neomycin sulfate (1 g/L) beginning 7 days prior to TNBS administration until the end of this trial to exhaust the intestinal flora in accordance with the previous method.Citation36 Induction of colitis and VDZ administration were outlined in the experimental procedure of Part I. Five groups of humanized mice (n = 10 in each group) were established: the control group (control), the model group (model), the VDZ-treated group (VDZ), the antibiotic-disposed model group (ABX-model), and the antibiotic-disposed VDZ-treated model group (ABX-VDZ). Stool samples were collected both before and after antibiotic administration for 16S-rDNA sequencing. The pertinent samples were obtained on the 14th day after the mice were anesthetized and sacrificed. The entire experimental process is detailed in .
To further confirm that the gut microbiota is associated with the response to anti-α4β7-integrin in IBD, we established the humanized gut microbiota through FMT. The FMT proceeds with a few minor changes, as originally described.Citation37 Feces were collected from clinical donors. At week 0 (baseline), the donors provided stool samples for FMT and 16S-rDNA sequencing. To guarantee that the majority of intestinal flora in the recipient mice (n = 10 for each group) were eliminated, ABX was administered for 14 days, after which the mice received induction with TNBS and, in the meantime, were transplanted every day with baseline intestinal flora from CD patients (FMT-model), week 14 remission patients (FMT-remission), and week 14 non-remission patients (FMT-non-remission) for 7 days. Besides, fresh human feces transplanted from healthy individuals were used as the controls (FMT-control). Induction of colitis and VDZ administration were conducted as described above. Stool samples of the recipient mice were collected for 16S-rDNA sequencing after FMT. The comprehensive experimental procedure is displayed in .
Evaluation of general condition
The status of the animals was checked daily, and the severity of the induced colitis was assessed using the disease activity index (DAI) score, as previously described.Citation38 The entire colon was removed from the mice after they were euthanized, and it was then assessed, weighed, and checked for indications of mucosal ulcers. The colonic mucosa injury index (CMDI) score was assessed on a scale from 0 to 4. A score of 0 indicated that there was no colon inflammation. The detailed scoring criteria were applied as reported previously.Citation39 The distal colon was cut into a 1 cm segment for histological assessment and immunofluorescence staining, and then rapid liquid nitrogen freezing of the remaining colon was performed for molecular analysis. A small incision was made in the tail to draw blood, and the serum was then frozen for the inflammatory cytokine assay.
Establishment of humanized gut microbiota
Stool samples were transplanted as previously described.Citation37 As some of the immunological and histological traits mimic elements of CD, TNBS-induced colitis has been widely employed to study a range of topics that may be pertinent to this disease.Citation40 Consequently, we included all donor patients with CD for a more rigorous and accurate analysis of the treatment response. The inclusion criterion was CD patients starting VDZ as part of their regular clinical treatment. Patients received 300 mg of VDZ intravenously at weeks 0 and 2 and then subsequently every 8 weeks. Patients with ileostomies or J-pouches were excluded from the study because disease activity could not be reliably estimated in these individuals. Utilizing the Harvey Bradshaw index (HBI), disease activity was evaluated at each infusion. HBI scoring was performed again at week 14 after the start of therapy during the prospective follow-up. The previously established criteria state that an HBI score of < 4 indicates clinical remission, and scores over 4 indicate active disease.Citation41 A total of ten CD individuals who did not receive VDZ treatment, ten patients in remission after treatment with VDZ, and ten patients who were not in remission after treatment were enrolled in this study. In addition, 10 healthy volunteers were included. The characteristics of the donors are listed in detail in Table S1. All participants signed written informed consent documentation. The study protocol was approved by the Ethical Committee of the First Affiliated Hospital of Guangxi Medical University (Approval No. 2023-E070-01).
Feces (5 g) were collected from the donors mentioned above, and combined feces were then homogenized for 1 minute. The particles were eliminated by creating a liquid slurry and subsequent centrifugation at 500 g for 3 minutes. To avoid changes in gut microbiota composition, the supernatant was collected in anaerobic circumstances and delivered to each recipient mouse at a volume of 200 μL within 10 minutes.
Histological assessment
The colon samples were embedded in paraffin and cut into 5 μm slices. Hematoxylin and eosin staining was performed on one set of paraffin slices, and the inflammation-related histological scores were calculated using previously reported criteria.Citation42
Measurement of inflammation cytokines
The manufacturer’s recommendations were followed while using specialist enzyme-linked immunosorbent assay kits (R&D Systems, MN, USA) to measure the human forms of serum interleukin (IL)-6, IL-10, and TNF-α levels. The cytokine concentration was determined according to the standard curve after measuring the absorbance (OD value) at 450 nm using a microplate reader (BioTek Instruments, VT, USA).
Immunofluorescence staining
Using the standard protocol, immunofluorescence staining was conducted with primary antibodies targeting mouse anti-CD3 (1:250, Cat #MA5–12577; Invitrogen™, CA, USA), mouse anti-CD68 (1:200, Cat #MA5–13324; Invitrogen™), rabbit anti-Zonula occludens-1 (ZO-1) (1:200, Cat #61–7300; Invitrogen™) and rabbit anti-Claudin-1 (1:200, Cat #PA5–16833; Invitrogen™). After the samples had been incubated with the primary antibodies overnight at 4°C, they were washed with PBS and then probed with the Alexa Fluor™ 594 goat anti-mouse secondary antibody (1:500, Cat #A11005; Invitrogen™), Alexa Fluor™ 488 goat anti-rabbit secondary antibody (1:500, Cat #A27034; Invitrogen™), and Alexa Fluor™ 594 goat anti-rabbit secondary antibody (1:500, Cat #A11012; Invitrogen™) for 1 h at 37°C in the dark. The samples were counterstained with DAPI and observed by laser scanning confocal microscopy (Zeiss LSM880, Jena, Germany).
Whole-genome shotgun metagenomic sequencing and data analysis
The same research technician isolated microbial genomic DNA from all four cohorts with the QIAamp Fast DNA Stool Mini Kit (Hilden, Germany) per the manufacturer’s instructions. DNA isolation was carried out using the QIAcube automated sample preparation device. With the Illumina NovaSeq 6000 platform (San Diego, CA, USA), a total of 8 Gb of 150 base pair readings per sample were generated by whole-genome shotgun metagenomic sequencing. Samples with fewer than 10 million readings were eliminated. The Illumina adapters were eliminated from raw readings using the kneadData tool (version 0.5.1), and the readings were then trimmed to PHRED quality 30. The FastQC tool (version 0.11.7) was deployed to evaluate the metagenomes’ quality. The MetaPhlAn3 tool (version 2.7.3), which used the marker gene database, profiled the taxonomic and composition of metagenomes. Fisher’s Alpha was determined using the normalized read count from the marker gene database for the signatures at the levels of phylum, class, order, family, genus, and species. On the same taxonomic ranks, Bray-Curtis dissimilarity was estimated within and between groups. ANOSIM analysis and non-metric multidimensional scaling (NMDS) were carried out on the basis of operational classification units (OTUs) by using R software (version 3.6.3). According to the median relative abundance across all samples, the top taxonomic categories and pathways were selected. The LEfSe algorithm was employed to perform differential abundance analyses to identify the taxonomic traits of important species using LDA coefficient cutoff values of 2.5 and FDR correction cutoff values of 0.05.Citation43 Student’s t-test was employed to determine whether any baseline taxonomic group or diversity measures substantially changed between individuals who achieved remission and those who did not.
16S-rDNA gene high-throughput sequencing and data analysis
Complete microbiological genomic DNA was extracted from each 200 mg fecal sample as described above. Specific primers were used to amplify the V3-V4 sections of the bacterial 16S-rDNA gene. The procedure was performed as previously stated.Citation36 The Amplicon Sequence Variants were combined with signature sequences to create the equivalent of 100% clustering OTUs. QIIME 2 was used to estimate the α-diversity and β-diversity following SILVA classifier (release 132) normalization. The Basic Local Alignment Search Tool was used to assess the signature sequence alignment. R software (version 3.6.3) was used to generate graphs.
Targeted metabolomics analysis
A liquid chromatography-mass spectrometry (LC-MS) system (AB Sciex, MA, USA) with negative ion scanning was used to measure the fecal levels of 27 BAs. Briefly, 900 μL of ultrapure water was utilized to suspend 100 mg of feces homogenized in liquid nitrogen. Thereafter, 500 μL of acetonitrile/methanol at a ratio of 8:2 was applied. Following the 30-second vortex, the mixture was centrifuged at 4°C and 12,000 rpm for 10 minutes. The supernatant was retained in the sample vial and separated on a chromatography column following homogenization and centrifugation. Multiple reaction monitoring was employed to detect and quantify BA metabolites, and the corresponding standard curves served as the basis for measurement. Microsoft Excel was used to analyze the SCIEX OS software-processed MS data. SIMCA 13.0 (Umetrics, Sweden) was implemented to visualize the multivariate data matrix.
Real-time quantitative polymerase chain reaction (RT-qPCR))
TRIzol reagent was applied to extract total RNA from approximately 50 mg of colon tissue. Subsequently, the RNA was quantified using a spectrophotometer at 260 nm. Reverse transcription was performed to obtain complementary DNA with the PrimeScript™ RT Reagent Kit (Cat #RR047A, Takara Biotechnology, Shiga, Japan). qPCR was conducted using the TB Green Premix Ex Taq™ II Kit (Cat #RR820A, Takara Biotechnology) on the ABI 7500 system (Applied Biosystems, CA, USA). Primers were synthesized by Takara Biotechnology. Table S2 shows the list of primer sequences. GAPDH gene expression was used as the endogenous control, and the RT-qPCR data were analyzed using the 2−ΔΔCt method.
Western blotting (WB)
The radioimmunoprecipitation assay lysis buffer was applied to extract the total protein from about 50 mg of colon tissue. After that, the BCA protein assay kit was used to quantify it. WB was carried out as statedCitation44 with the following antibodies: rabbit anti-ZO-1 (1:1000, Cat #NBP1–85046; Novus Biologicals, CO, USA), rabbit anti-Claudin-1 (1:1000, Cat #NBP1–77036, Novus Biologicals), rabbit anti-FXR (1:1000, Cat #12295, Cell Signaling Technology, MA, USA), rabbit anti-TGR5 (1:1000, Cat #NBP2–23669, Novus Biologicals), rabbit anti-β-tubulin (1:1000, Cat #2146, Cell Signaling Technology), and goat anti-rabbit HRP-linked secondary antibody (1:3000, Cat #7074, Cell Signaling Technology). Using the Western BrightTM ECL kit (Advansta, CA, USA), the membranes were visualized.
Cell culture and drug intervention
Human Caco-2 cells were provided by the American Type Culture Collection (MD, USA). In DMEM/F12 (1:1) medium with 10% fetal bovine serum, cells were cultured at 37°C in 5% CO2. The cells were passaged when they reached approximately 80% confluency, and the medium was changed every 2 days. To administer medications, cells were plated at the 8 × 10Citation5 cells/well density and exposed to different concentrations of VDZ (1, 5, and 10 nM), CDCA (50 μM), and LCA (10 μM) for 12 hours. Cells were then rinsed, and RNA and proteins were extracted.
Statistical analysis
The data is shown as the mean ± SD. GraphPad Prism 9.0 (CA, USA) was employed for statistical analysis. One-way analysis of variance followed by the least significant difference test (assuming equal variances) or the Tam-hance’s T2 test (not assuming equal variances) was performed to determine statistical significance across multiple groups. Student’s t test was used for comparison between the two groups. P < .05 was considered significant.
Results
Anti-α4β7-integrin mitigates pathological symptoms and intestinal inflammation in remission-achieving colitis mice
The common clinical manifestations of IBD, including diarrhea, hematochezia, and weight loss, were effectively simulated by TNBS-induced intestinal inflammation.Citation45 Following TNBS administration, the weight loss and DAI scores were significantly increased, indicating that colitis was successfully induced in the humanized mice. After the treatment with anti-α4β7-integrin, colitis mice in the remission group showed the significant improvement in weight loss and DAI scores. As the modeling process progressed, some mice’s weight stabilized or slightly increased, and the DAI scores gradually dropped as a result of the body’s tendency for self-healing (). Severe pathological symptoms and high CMDI scores were found in the model and non-remission groups; however, the above symptoms and scores were significantly improved in the remission group (). Previous literature has shown that anti-α4β7-integrin therapy reduces the expression of CD3+ T cells and CD68+ macrophages with or without remission,Citation35 and we obtained the similar results, indicating that anti-α4β7-integrin functioned in colitis mice (). Furthermore, as shown in (, the inflammation cytokines IL-6 and TNF-α demonstrated significantly lower mRNA and protein expression levels, and IL-10 demonstrated significantly higher mRNA and protein expression levels in the remission mice than in the non-remission mice. Regrettably, not all colitis mice demonstrated an improved inflammatory phenotype with anti-α4β7-integrin. In short, some humanized mice with colitis did not respond to anti-α4β7-integrin.
Figure 1. Anti-α4β7-integrin therapy mitigates inflammation symptoms in remission-achieving colitis mice. (a) Daily variations in body weight and the weight ratio of Day 7/Day 0 (n = 9–11). (b) Daily variations in disease activity index (DAI) and DAI on Day 7 (n = 9–11). (c) Macroscopic observation of the colon and the colonic mucosa injury index (CMDI) (n = 9–11). (d) Hematoxylin and eosin (h&e) staining images and histological scores related to inflammation. Scale bar = 200 μm (n = 6). (e and f) Immunofluorescence images displaying CD3 and CD68 in situ expression. Scale bar = 50 μm (n = 4). (g) CD3 and CD68 mRNA expression levels (n = 6). (h) Inflammatory cytokine mRNA expression levels (n = 6). (i) Inflammatory cytokine levels in serum (n = 8). Mean ± SD is shown. One-way analysis of variance (ANOVA) was applied. nsp > .05, *p < .05, **p < .01, ***p < .001, and ****p < .0001.
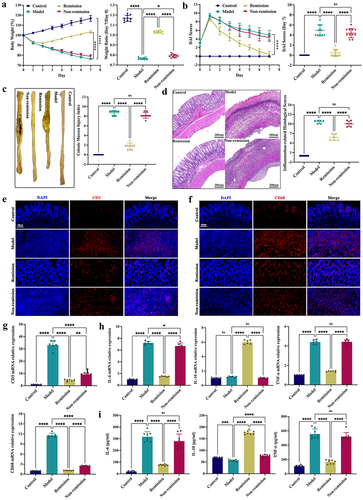
Anti-α4β7-integrin restores gut barrier function in remission-achieving colitis mice
As part of the therapy process, the tight junctions (TJs), including ZO-1, occludin, claudin-1, and claudin-4, are principally examined to determine the structural integrity of the intestinal epithelium. In contrast to the non-remission group, the remission group had considerably higher mRNA expression levels for TJs, as shown in . Moreover, the localization of ZO-1 and claudin-1 proteins on the epithelial cell membrane of healthy intestines was revealed by immunofluorescence staining; however, in mice with colitis, these TJ proteins were largely depleted. Interestingly, the mucosal layers of the remission group displayed noticeably higher levels of TJ proteins than the non-remission group (). Similar results were obtained using WB (). These results revealed that anti-α4β7-integrin therapy can repair gut barrier damage in colitis mice that achieve remission.
Figure 2. Anti-α4β7-integrin therapy repairs intestinal barrier damage in remission-achieving colitis mice. (a) Tight junctions mRNA expression levels (n = 6). (b and c) Immunofluorescence images displaying Zonula occludens-1 (ZO-1) and claudin-1 in situ expression. Scale bars = 100, 50, and 20 μm (n = 4). (d) Immunoblots showing ZO-1 and claudin-1 protein expression levels (n = 6). Mean ± SD is shown. One-way ANOVA was applied. nsp > .05, *p < .05, **p < .01, ***p < .001, and ****p < .0001.
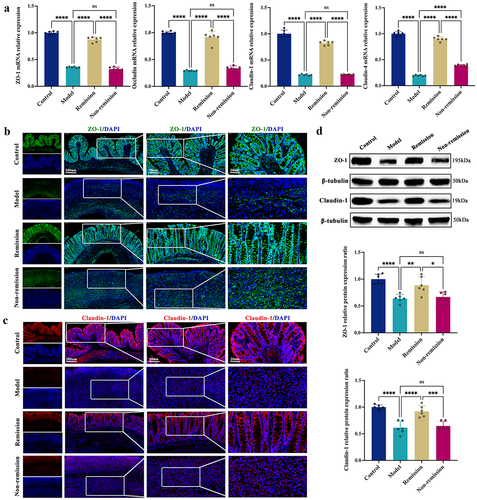
Baseline microbiome profiles can be used to predict remission and response to anti-α4β7-integrin in humanized mice with colitis
Given that intestinal microecological disorder is a key determinant in the propagation of IBD, we used the metagenomic sequencing to examine the treatment response. As shown in Table S3, in contrast to colitis mice who failed to achieve remission, remission-achieving colitis mice had higher baseline community diversity. The PCA score plot illustrated that the OTUs in the non-remission group were distant from those in the remission group (). Consistent with this finding, the NMDS plot also showed a clear separation of the OTUs between the groups with and without remission (). Besides, the ANOSIM analysis indicated that the difference in inter-group was greater than that in intra-group, indicating that grouping was meaningful (). Excitingly, the differences in the composition of gut microbiota were found between the groups in and out of remission at all levels (). We further examined the differences in colitis mice treated with VDZ using the LEfSe tool. The results revealed that at the genus level, Paraprevotella, Prevotella, Roseburia, Lactobacillus, and Clostridium were enriched in the remission group, whereas Parabacteroides, Ruthenibacterium, Ruminococcus, Bacteroides, Emergencia, and Blautia were enriched in the non-remission group (). Moreover, there were significant differences in the abundance of gut microbiota at the species level between the remission and non-remission groups (). Collectively, employing baseline microbiome profiles to predict remission and response to treatment was shown to be a promising strategy.
Figure 3. Baseline microbiome profiles can predict the treatment response. (a) PCA score plot. (b) NMDS plot. (c) ANOSIM analysis. (d-i) Fecal microbiota relative abundance at all levels. (j) Bacteria significantly varied at the genus level between groups with and without remission. (k) Bacteria significantly varied at the species level between groups with and without remission. (n = 3 in each group). The LEfSe algorithm was employed for performing differential abundance analyses using LDA coefficient cutoff values of 2.5 and FDR correction cutoff values of 0.05.
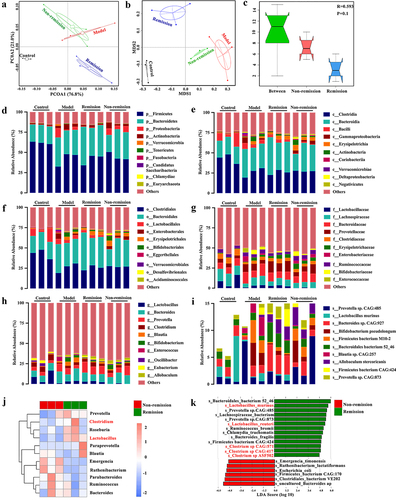
Response to anti-α4β7-integrin is impacted by targeting the gut microbiota in humanized mice with colitis
To confirm whether microbiome signatures were involved in the response to anti-α4β7-integrin in IBD, we induced colitis in PGF mice (). Through 16S-rDNA sequencing, we discovered that PGF mice demonstrated a less varied gut microbiome in general, indicating that most of the bacteria had been depleted (). In PGF mice with colitis, the beneficial effects of anti-α4β7-integrin, including an increase in body weight, a lower DAI score, and the reversal of severe pathological symptoms, were partially abolished (). Anti-α4β7-integrin did not significantly alter the levels of serum inflammation cytokines () and had limited effects on the expression of TJs ().
Figure 4. Gut microbiota depletion and fecal microbiota transplantation (FMT). (a) Schematic illustration of gut microbiota depletion caused by antibiotics (ABX). (b) Multiple sample PCoA. (c) Community α-diversity presented by the Chao 1, Shannon, and Simpson indices. (d) Schematic illustration of FMT to colitis mice. (e) Multiple sample PCoA in different groups. (f-i) Community α-diversity presented by the Chao 1, Shannon, and Simpson indices in different groups. (n = 4 in each group). Mean ± SD is shown. Student’s t test was applied. nsp > .05, *p < .05, and **p < .01.
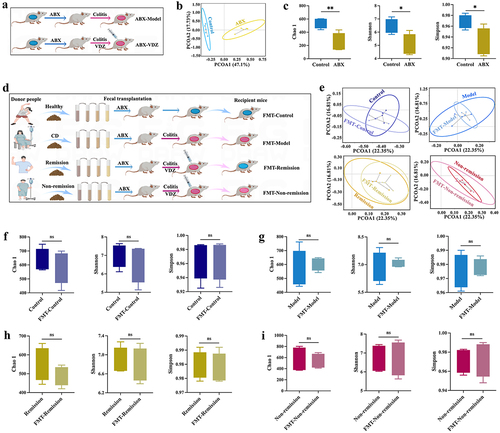
Figure 5. Baseline gut microbiota contain common bacteria with anti-inflammatory effects. (a and c) Daily variations in body weight and the weight ratio of Day 7/Day 0 (n = 10). (b and d) Daily variations in DAI and DAI on Day 7 (n = 10). (e and f) H&E staining images and histological scores related to inflammation. Scale bar = 200 μm (n = 6). Mean ± SD is shown. One-way ANOVA was applied. nsp > .05, **p < .01, and ****p < .0001.
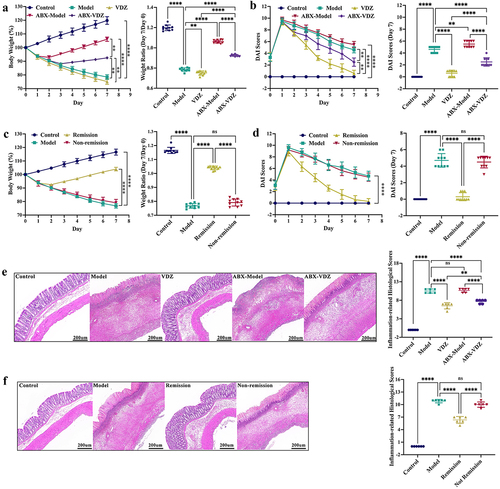
Figure 6. Baseline gut microbiota reduce mucosal barrier damage. (a and d) Tight junctions mRNA expression levels (n = 6). (b and e) Inflammatory cytokine levels in serum (n = 8). (c and f) Immunoblots showing ZO-1 and claudin-1 protein expression levels (n = 6). Mean ± SD is shown. One-way ANOVA was applied. nsp > .05, *p < .05, **p < .01, ***p < .001, and ****p < .0001.
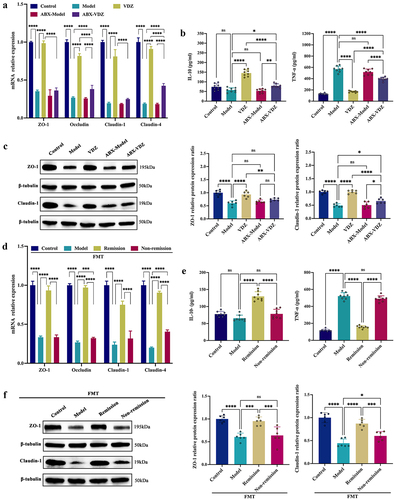
To further verify the fundamental significance of microbiome signatures for the response to anti-α4β7-integrin in IBD, we administered FMT to PGF mice with colitis (). We sequenced the baseline feces of clinical donors and recipient mice for 16S-rDNA, and the results showed no significant difference, which indicated that the human intestinal flora was successfully transplanted into the mice of each group (). In the recipient mice, FMT from remission patients increased body weight, decreased DAI scores, and mitigated the pathological symptoms. In contrast, FMT from non-remission patients produced no discernible response to treatment (). Moreover, in the FMT-remission group, the levels of serum inflammation cytokines were significantly changed (), and the expression of TJs was also considerably increased (). However, the above indicators were not substantially altered in the FMT-non-remission group. To summarize, the baseline gut microbiota contained common bacteria with anti-inflammatory effects and reduced mucosal barrier damage, which improved the treatment response.
BA metabolism modulation is involved in the response of humanized mice with colitis to anti-α4β7-integrin
Since BAs play a role in regulating intestinal homeostasis,Citation46,Citation47 we explored whether fecal BA levels affect the response to anti-α4β7-integrin in IBD. In this research, a total of 27 BAs were detected by targeted metabolomics analysis (). BAs were clearly clustered in each group according to the PCA score plot, and the clusters between the remission and non-remission groups were distant (). As well, the concentration of secondary BAs and the ratio of secondary to primary BAs were both significantly higher (), and considerably higher baseline levels of CDCA (a natural agonist of FXR) and LCA (a natural agonist of TGR5) were discovered in the remission group than in the non-remission group. Furthermore, the level of isoLCA was decreased, and the levels of TCDCA, α-MCA, β-MCA, UDCA, and HDCA were all increased in mice that achieved remission (). Therefore, we demonstrated that BAs correlated to microbial diversity were involved in the remission of colitis in humanized mice.
Figure 7. Baseline bile acid (BA) metabolism modulation is involved in the treatment response. (a) PCA score plot. (b) the level of secondary BAs and the ratio of secondary/primary BAs. (c) Composition of BAs. (d) Comparison of the relative diversity of significantly changed BAs across groups. (n = 6 in each group). Mean ± SD is shown. One-way ANOVA was applied. nsp > .05, *p < .05, **p < .01, ***p < .001, and ****p < .0001.
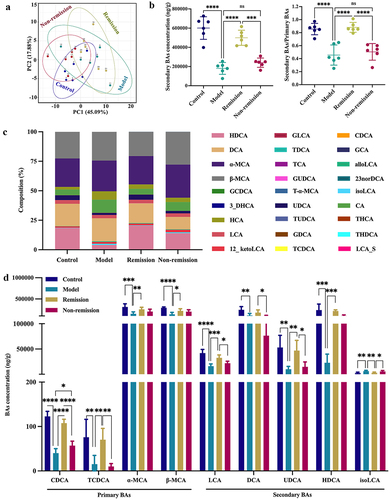
Gut microbiota-related BA metabolism-FXR/TGR5 axis impacts the response of humanized mice with colitis to anti-α4β7-integrin
As microbial BAs are FXR and TGR5 receptor agonists, and the levels of CDCA and LCA varied considerably between groups, we next determined whether the stimulation of colonic FXR and TGR5 was associated with the response to anti-α4β7-integrin in IBD. As depicted in , the expression levels of FXR and TGR5 were significantly increased, and their target genes (FGF15 for FXR and YAP for TGR5), which are essential for alleviating colonic inflammation and improving gut barrier dysfunction,Citation30,Citation48 were also upregulated in the remission group versus the non-remission group. However, the depletion of intestinal flora mediated by antibiotics partially attenuated the regulatory effects of anti-α4β7-integrin (). Additionally, in the PGF mice with colitis, FMT from remission patients markedly elevated the mRNA and protein expression levels of FXR and TGR5, whereas FMT from non-remission patients had limited effects (). Thus, the altered microbiome-mediated activation of FXR and TGR5 has a causal role in predicting the treatment response in colitis.
Figure 8. Ability to alter gut microbiota is essential for activating colonic FXR and TGR5. (a-c) FXR, TGR5, and downstream genes mRNA expression levels. (d – f) Immunoblots showing FXR and TGR5 protein expression levels. (n = 6 in each group). Mean ± SD is shown. One-way ANOVA was applied. nsp > .05, *p < .05, **p < .01, ***p < .001, and ****p < .0001.
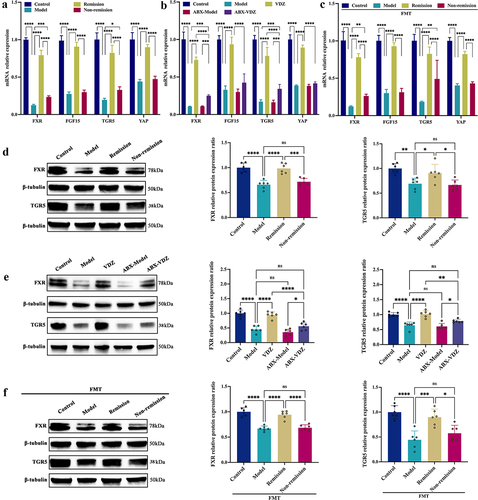
To determine whether BAs have a direct effect on FXR and TGR5, human Caco-2 cells were cultured with the FXR agonist CDCA and the TGR5 agonist LCA.Citation49,Citation50 As shown in , the mRNA expression levels of cognate receptors were increased by the agonists; however, anti-α4β7-integrin had no effect on the expression of FXR and TGR5 or target genes FGF19 and YAP, even at high dosages. Consistently, the protein expression levels of FXR and TGR5 were both increased after agonists stimulation, but anti-α4β7-integrin failed to yield any effects (). In summary, the production of microbial BAs, particularly CDCA and LCA, have a direct stimulatory effect on FXR and TGR5.
Figure 9. Production of microbial BAs has a direct stimulatory effect on FXR and TGR5. (a) FXR, TGR5, and downstream genes mRNA expression levels in Caco-2 cells. (b) Immunoblots showing FXR and TGR5 protein expression levels in Caco-2 cells. (n = 3 in each group). Mean ± SD is shown. One-way ANOVA was applied. **p < .01 and ***p < .001.
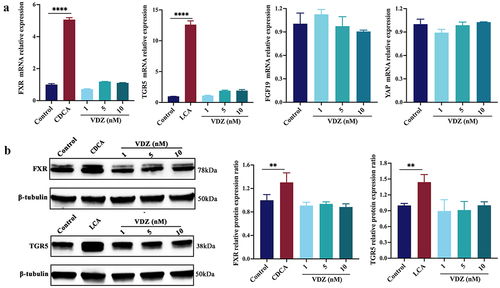
Discussion
Many therapeutic targets are now available, highlighting the value of individualized treatment and the necessity of predicting response to each mechanism of action. The inability to predict in advance which treatments will benefit patients most may cause delays in establishing remission, low response rates, and long-term morbidity. Hence, it is of utmost clinical and scientific significance to comprehend the determinants that affect the response to one therapeutic class. Initial efforts to do so that relied solely on clinical factors yielded disappointing outcomes.Citation51 The capacity of genetics to impact the therapeutic response is also incompletely understood.Citation51 Target organ (the intestine in IBD) genomic expression profiles initially demonstrated promise, but their level of predictability remains modest,Citation52 underscoring the requirement to uncover novel determinants for impacting the treatment response.
The gut microbiota is crucial for the initiation and propagation of intestinal inflammation;Citation3,Citation19,Citation21,Citation53 variables that decrease the gut microbiota diversity, such as antibiotics, increase the likelihood of IBD.Citation54,Citation55 Currently, a couple of bacteria linked to clinical remission have demonstrated promise as stand-alone treatments for the microbiome. In experimental colitis, B. ovatus monotherapy was better than FMT for achieving remission.Citation56 A prospective cohort study by Shaw et al. revealed differences in the gut microbiome species (i.e., Fusobacterium, Adlercreutzia, and Akkermansia) between remission and non-remission patients. They were able to predict the responder status with 76.5% accuracy utilizing microbiome data.Citation57 In a trial of CD patients, an increase in B. longum was linked to maintaining remission.Citation58 In addition, earlier research correlated microbial metacommunities and anti-integrin (i.e., VDZ) therapy, indicating that remission patients initially treated with anti-integrin showed increased abundance of beneficial Bifidobacterium longum and Bacteroides, promoting intestinal homeostasis.Citation59
Here, we established correlations between baseline microbiome profiles and remission and illustrated the value of a predictive model incorporating microbiome signatures to identify the treatment response. We further proposed that the trajectory of early microbiome alterations may serve as biomarkers of response to this therapy in IBD. As expected, significant variations in bacterial abundance existed between the groups with and without remission, and the gut microbiota depletion caused by antibiotics could limit the effect of anti-α4β7-integrin on alleviating intestinal inflammation, indicating that the baseline microbiome profiles are a key determinant of remission. Additionally, FMT from remission patients mitigated the symptoms of colitis in recipient animals, highlighting that the response to anti-α4β7-integrin relied on reshaping the bacterial abundance.
As already stated, we found that the baseline gut microbiota, which contained common microbes, was linked to the treatment response. Taxonomically, remission was predicted by the baseline relative abundance of Lactobacillus and Clostridium in our study. Lactobacillus is a significant bacterial genus implicated in BA metabolism and exploits bile-salt hydrolase to deconjugate BAs to their corresponding unconjugated free forms.Citation60 A high level of bile-salt hydrolase in the intestinal tract also benefits gut barrier function and prevents colitis.Citation61,Citation62 In addition, via CYP7A1-mediated 7α-dehydroxylation, both Lactobacillus and Clostridium are capable of converting primary BAs into secondary BAs. Citation27,Citation47 Thus, the elevated levels of CDCA and LCA, which have been utilized to demonstrate anti-inflammatory effects on the intestinal tract,Citation63,Citation64 partly result from the dysregulated microbiome’s improvement, which led to a rise in the abundance of Lactobacillus and Clostridium in remission-achieving colitis mice. In brief, the diversity of BAs in colitis mice that respond to anti-α4β7-integrin may be affected by alterations in the abundance of gut microbiota brought on by mucosal inflammation and IBD activity.
At the same time, we also determined BA-targeted metabolic signatures that predicted remission in TNBS-induced colitis mice undergoing anti-α4β7-integrin therapy. Our data revealed that the difference in baseline BAs might be a determinant of gut microbiota impacting the treatment response. BAs, which are endogenous signaling molecules, can regulate local immunological function, intestinal hormone secretion, and gastrointestinal motility by interacting with receptors, such as FXR and TGR5.Citation65 The primary endogenous ligands for FXR and TGR5 are unconjugated BAs, including CDCA, DCA, LCA, and CA. Among these, CDCA has the strongest effect on FXR activation,Citation66 while LCA has the highest capacity to activate TGR5.Citation60 Our result was in agreement with previous research in that gut microbiome disorder stimulation markedly reduced the amounts of LCA and CDCA in the feces.Citation67 We also discovered that the remission group not only had high baseline BA levels but also had active FXR and TGR5 signaling pathways. In the lack of intestinal flora, FXR and TGR5 were partially activated; however, this activation effect was reversed by the subsequent FMT from patients in remission. These findings strongly imply that the ability to restore the gut microbiota is essential for the activation of FXR and TGR5. Furthermore, BA-cultured Caco-2 cells showed a direct stimulatory effect on FXR and TGR5. Studies have shown that BA-mediated activation of the FXR/TGR5 was protective in colitis.Citation67 However, the absence of secondary BAs decreased FXR and TGR5 activation, which may weaken gut barrier integrity and anti-inflammatory signaling pathways, eventually contributing to the pathophysiology of IBD.Citation61,Citation67 Hence, the activation of FXR and TGR5 signaling pathways through BAs may increase the response to anti-α4β7-integrin therapy in IBD.
Although FXR and TGR5 play critical roles in preserving gut homeostasis, their agonists’ therapeutic potential is constrained by their significant adverse effects. Itching and other negative effects are connected to the usage of FXR ligands and worsen with increasing doses.Citation68 Upon intestinal FXR activation, these ligands also hinder the synthesis of BAs in the liver.Citation69 Moreover, pruritus develops in mice after topical TGR5-specific BAs administration, and TGR5 ablation alleviates this condition.Citation70 Diarrhea is yet another adverse effect connected with the application of TGR5 ligands.Citation28 Studies have shown that taking supplements containing LCA and CDCA helps alleviate gut barrier dysfunction and intestinal inflammation,Citation63,Citation71 but high physiological concentrations of BAs have a number of serious adverse effects such as DNA damage, apoptosis, and oxidative/nitrative stress.Citation72,Citation73 Therefore, the indirect induction of FXR and TGR5 activation by restoring the composition of gut microbiota increases the response to anti-α4β7-integrin therapy, which is a safer therapeutic avenue for managing IBD than the application of particular BAs or synthetic BA agonists to directly activate the target pharmacologically.
Nevertheless, in the current research, further experimental data are required to confirm that signaling pathways of FXR and TGR5 are primarily responsible for the IBD response to anti-α4β7-integrin therapy. Collectively, testing the response to this therapy in colitis using FXR/TGR5 antagonists or the dual-gene deletion animal model would be a viable and promising avenue. Therefore, our upcoming research on this subject will be primarily concentrated on developing a dual-gene knockout animal model with colitis, which is anticipated to deliver more accurate results and conclusively establish whether the mechanism of improving the response to anti-α4β7-integrin therapy in IBD is linked to the activation of FXR and TGR5 signaling pathways.
In light of these findings, we can tentatively suggest the potential mechanism involved in the response to anti-α4β7-integrin therapy in TNBS-induced colitis as follows. The baseline gut microbiota in remission-achieving colitis mice contained common microbes that could regulate microbial BA levels, especially the beneficial genera of Lactobacillus and Clostridium, which are linked to increased gastrointestinal BAs. Moreover, the production of gastrointestinal BAs, particularly CDCA and LCA, led to further stimulation of FXR and TGR5, which significantly improved gut barrier function and mitigated intestinal inflammation ().
Figure 10. Illustration of the mechanism impacting the response to anti-α4β7-integrin therapy in humanized mice with colitis. The baseline gut microbiota in remission-achieving colitis mice contained common microbes that could regulate microbial BA levels. Moreover, the increased BA levels led to further stimulation of FXR and TGR5, which significantly improved gut barrier function and mitigated intestinal inflammation.
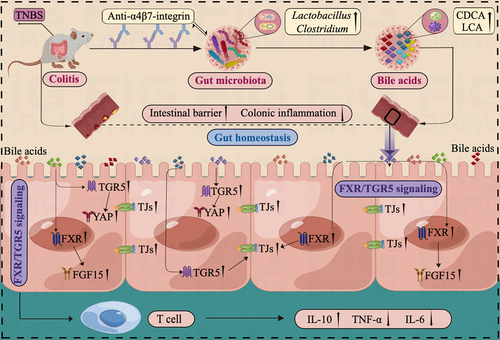
Taken together, our findings have several important implications. First, our results show that microbially encoded pathways may play a role in the treatment response in addition to microbial taxonomy. The pathways have been confirmed by alterations in the diversity of BA metabolism. Our results additionally demonstrate a role for BA metabolism correlated to microbial diversity as a determinant in impacting the treatment response. Specifically, our results suggest that the direct activation of FXR and TGR5 signaling pathways through BAs increases the treatment response. Overall, the gut microbiota-related BA metabolism-FXR/TGR5 axis may be a potential mechanism for impacting the response to anti-α4β7-integrin therapy in colitis mice. The discovery of predictive microbial signals can lead to the development of new probiotics with specific anti-inflammatory activities or that trigger anti-inflammatory metabolic pathways. The omics signatures discovered in the present research can also be exploited as targets for more advanced treatments and provide additional insight into the pathogenesis and development of these complicated diseases.
Authors contribution
Bing Han and Xiaoping Lv designed and conceived the study. Bing Han, XiaoDan Lv, Gengfeng Liu, Shiquan Li, Junhua Fan, Lan Chen, Zhixi Huang, Guangfu Lin, Xiaofang Xu, and Ziqian Huang performed the experiments. Bing Han, XiaoDan Lv, and Gengfeng Liu analyzed and collated the data. Bing Han wrote the first draft. Xiaoping Lv and Lingling Zhan revised the paper. The final paper was reviewed and approved by all authors.
Supplemental Material
Download MS Word (14.7 KB)Supplemental Material
Download MS Word (17.5 KB)Supplemental Material
Download MS Word (22.5 KB)Acknowledgments
in this study was created by Figdraw (www.figdraw.com).
Disclosure statement
No potential conflict of interest was reported by the author(s).
Data availability statement
The data generated during whole-genome shotgun metagenomic sequencing and 16S-rDNA gene high-throughput sequencing were deposited in the National Centre for Biotechnology Information (NCBI) Sequence Read Archive (SRA; https://www.ncbi.nlm.nih.gov/) under BioProject numbers PRJNA941025, PRJNA941019 and PRJNA941022.
Supplementary material
Supplemental data for this article can be accessed online at https://doi.org/10.1080/19490976.2023.2232143
Additional information
Funding
References
- Chang JT, Longo DL. Pathophysiology of inflammatory bowel diseases. N Engl J Med. 2020;383(27):2652–23. doi:10.1056/NEJMra2002697.
- Ananthakrishnan AN. Epidemiology and risk factors for IBD. Nat Rev Gastroenterol Hepatol. 2015;12:205–217. doi:10.1038/nrgastro.2015.34.
- Knights D, Lassen KG, Xavier RJ. Advances in inflammatory bowel disease pathogenesis: linking host genetics and the microbiome. Gut. 2013;62:1505–1510. doi:10.1136/gutjnl-2012-303954.
- Tysk C, Lindberg E, Järnerot G, Flodérus-Myrhed B. Ulcerative colitis and Crohn’s disease in an unselected population of monozygotic and dizygotic twins. A study of heritability and the influence of smoking. Gut. 1988;29:990–996. doi:10.1136/gut.29.7.990.
- Jostins L, Ripke S, Weersma RK, Duerr RH, McGovern DP, Hui KY, Lee JC, Schumm LP, Sharma Y, Anderson CA, et al. Host–microbe interactions have shaped the genetic architecture of inflammatory bowel disease. Nature. 2012;491(7422):119–124. doi:10.1038/nature11582.
- Liu JZ, van Sommeren S, Huang H, Ng SC, Alberts R, Takahashi A, Ripke S, Lee JC, Jostins L, Shah T, et al. Association analyses identify 38 susceptibility loci for inflammatory bowel disease and highlight shared genetic risk across populations. Nat Genet. 2015;47:979–986. doi:10.1038/ng.3359.
- Olivera P, Danese S, Peyrin-Biroulet L. Next generation of small molecules in inflammatory bowel disease. Gut. 2017;66(2):199–209. doi:10.1136/gutjnl-2016-312912.
- Danese S, Vuitton L, Peyrin-Biroulet L. Biologic agents for IBD: practical insights. Nat Rev Gastroenterol Hepatol. 2015;12(9):537–545. doi:10.1038/nrgastro.2015.135.
- Peyrin-Biroulet L, Reinisch W, Colombel J, Mantzaris G, Kornbluth A, Diamond R, Rutgeerts P, Tang L, Cornillie F, Sandborn W. Clinical disease activity, C-reactive protein normalisation and mucosal healing in Crohn’s disease in the SONIC trial. Gut. 2014;63:88‐95. doi:10.1136/gutjnl-2013-304984.
- Ben-Horin S, Kopylov U, Chowers Y. Optimizing anti-TNF treatments in inflammatory bowel disease. Autoimmun Rev. 2014;13(1):24–30. doi:10.1016/j.autrev.2013.06.002.
- Boyapati RK, Kalla R, Satsangi J, Ho GT. Biomarkers in search of precision medicine in IBD. Am J Gastroenterol. 2016;111(12):1682–1690. doi:10.1038/ajg.2016.441.
- West NR, Hegazy AN, Owens BMJ, Bullers SJ, Linggi B, Buonocore S, Coccia M, Gortz D, This S, Stockenhuber K, et al. Oncostatin M drives intestinal inflammation and predicts response to tumor necrosis factor–neutralizing therapy in patients with inflammatory bowel disease. Nat Med. 2017;23(5):579–589. doi:10.1038/nm.4307.
- Verstockt B, Verstockt S, Creyns B, Tops S, Van Assche G, Gils A, Ceuppens JL, Vermeire S, Ferrante M, Breynaert C. Mucosal IL13RA2 expression predicts nonresponse to anti-TNF therapy in Crohn’s disease. Aliment Pharmacol Ther. 2019;49(5):572–581. doi:10.1111/apt.15126.
- Gaujoux R, Starosvetsky E, Maimon N, Vallania F, Bar-Yoseph H, Pressman S, Weisshof R, Goren I, Rabinowitz K, Waterman M, et al. Cell-centred meta-analysis reveals baseline predictors of anti-TNFα non-response in biopsy and blood of patients with IBD. Gut. 2019;68(4):604–614. doi:10.1136/gutjnl-2017-315494.
- Veny M, Fernández-Clotet A, Panés J. Controlling leukocyte trafficking in IBD. Pharmacol Res. 2020;159:105050. doi:10.1016/j.phrs.2020.105050.
- Kobayashi T, Hibi T. Improving IBD outcomes in the era of many treatment options. Nat Rev Gastroenterol Hepatol. 2023;20(2):79–80. doi:10.1038/s41575-022-00738-z.
- Wyant T, Fedyk E, Abhyankar B. An overview of the mechanism of action of the monoclonal antibody vedolizumab. J Crohns Colitis. 2016;10(12):1437–1444. doi:10.1093/ecco-jcc/jjw092.
- Sands B, Peyrin-Biroulet L, Loftus E, Danese S, Colombel J, Törüner M, Jonaitis L, Abhyankar B, Chen J, Rogers R, et al. Vedolizumab versus adalimumab for moderate-to-severe ulcerative colitis. N Engl J Med. 2019;381(13):1215–1226. doi:10.1056/NEJMra1905725.
- Becker C, Neurath MF, Wirtz S. The Intestinal microbiota in inflammatory bowel disease. Ilar J. 2015;56(2):192–204. doi:10.1093/ilar/ilv030.
- Forbes JD, Van Domselaar G, Bernstein CN. The gut microbiota in immune-mediated inflammatory diseases. Front Microbiol. 2016;7:1081. doi:10.3389/fmicb.2016.01081.
- Kostic AD, Xavier RJ, Gevers D. The microbiome in inflammatory bowel disease: current status and the future ahead. Gastroenterology. 2014;146(6):1489–1499. doi:10.1053/j.gastro.2014.02.009.
- Ananthakrishnan AN, Luo C, Yajnik V, Khalili H, Garber JJ, Stevens BW, Cleland T, Xavier RJ. Gut microbiome function predicts response to anti-integrin biologic therapy in inflammatory bowel diseases. Cell Host & Microbe. 2017;21(5):603–610.e3. doi:10.1016/j.chom.2017.04.010.
- Franzosa EA, Sirota-Madi A, Avila-Pacheco J, Fornelos N, Haiser HJ, Reinker S, Vatanen T, Hall AB, Mallick H, McIver LJ, et al. Gut microbiome structure and metabolic activity in inflammatory bowel disease. Nature Microbiol. 2019;4(2):293–305. doi:10.1038/s41564-018-0306-4.
- Jacobs JP, Goudarzi M, Singh N, Tong M, McHardy IH, Ruegger P, Asadourian M, Moon BH, Ayson A, Borneman J, et al. A disease-associated microbial and metabolomics state in relatives of pediatric inflammatory bowel disease patients. Cell Mol Gastroenterol Hepatol. 2016;2(6):750–766. doi:10.1016/j.jcmgh.2016.06.004.
- Torres J, Palmela C, Brito H, Bao X, Ruiqi H, Moura-Santos P, Pereira da Silva J, Oliveira A, Vieira C, Perez K, et al. The gut microbiota, bile acids and their correlation in primary sclerosing cholangitis associated with inflammatory bowel disease. United European Gastroenterol J. 2018;6(1):112–122. doi:10.1177/2050640617708953.
- Jones ML, Martoni CJ, Prakash S. Letter to the editor regarding the report of Duboc et al: Connecting dysbiosis, bile-acid dysmetabolism and gut inflammation in inflammatory bowel disease. Gut. 2013;62:654–655. doi:10.1136/gutjnl-2012-303867.
- Chiang JY. Bile acids: regulation of synthesis. J Lipid Res. 2009;50(10):1955–1966. doi:10.1194/jlr.R900010-JLR200.
- Fiorucci S, Carino A, Baldoni M, Santucci L, Costanzi E, Graziosi L, Distrutti E, Biagioli M. Bile acid signaling in inflammatory bowel diseases. Dig Dis Sci. 2021;66(3):674–693. doi:10.1007/s10620-020-06715-3.
- Paramsothy S, Nielsen S, Kamm MA, Deshpande NP, Faith JJ, Clemente JC, Paramsothy R, Walsh AJ, van den Bogaerde J, Samuel D, et al. Specific bacteria and metabolites associated with response to fecal microbiota transplantation in patients with ulcerative colitis. Gastroenterology. 2019;156(5):1440–1454.e2. doi:10.1053/j.gastro.2018.12.001.
- Sorrentino G, Perino A, Yildiz E, El Alam G, Bou Sleiman M, Gioiello A, Pellicciari R, Schoonjans K. Bile acids signal via TGR5 to activate intestinal stem cells and epithelial regeneration. Gastroenterology. 2020;159(3):956–968.e8. doi:10.1053/j.gastro.2020.05.067.
- Cipriani S, Mencarelli A, Chini MG, Distrutti E, Renga B, Bifulco G, Baldelli F, Donini A, Fiorucci S. The bile acid receptor GPBAR-1 (TGR5) modulates integrity of intestinal barrier and immune response to experimental colitis. PLoS One. 2011;6(10):e25637. doi:10.1371/journal.pone.0025637.
- Gadaleta RM, van Erpecum KJ, Oldenburg B, Willemsen EC, Renooij W, Murzilli S, Klomp LW, Siersema PD, Schipper ME, Danese S, et al. Farnesoid X receptor activation inhibits inflammation and preserves the intestinal barrier in inflammatory bowel disease. Gut. 2011;60(4):463–472. doi:10.1136/gut.2010.212159.
- Billerbeck E, Barry WT, Mu K, Dorner M, Rice CM, Ploss A. Development of human CD4+FoxP3+ regulatory T cells in human stem cell factor–, granulocyte-macrophage colony-stimulating factor–, and interleukin-3–expressing NOD-SCID IL2Rγnull humanized mice. Blood. 2011;117(11):3076–3086. doi:10.1182/blood-2010-08-301507.
- Wirtz S, Popp V, Kindermann M, Gerlach K, Weigmann B, Fichtner-Feigl S, Neurath MF. Chemically induced mouse models of acute and chronic intestinal inflammation. Nat Protoc. 2017;12(7):1295–1309. doi:10.1038/nprot.2017.044.
- Manzini R, Schwarzfischer M, Atrott K, Laimbacher A, Lang S, Wawrzyniak M, Rickenbacher A, Turina M, Hruz P, Lissner D, et al. Combination of vedolizumab with tacrolimus is more efficient than vedolizumab alone in the treatment of experimental colitis. Inflamm Bowel Dis. 2021;27(12):1986–1998. doi:10.1093/ibd/izab063.
- Hou Q, Huang J, Zhao L, Pan X, Liao C, Jiang Q, Lei J, Guo F, Cui J, Guo Y, et al. Dietary genistein increases microbiota-derived short chain fatty acid levels, modulates homeostasis of the aging gut, and extends healthspan and lifespan. Pharmacol Res. 2023;188:106676. doi:10.1016/j.phrs.2023.106676.
- Jin JH, Xu Z, Zhang LN, Zhang C, Zhao XD, Mao YX, Zhang HJ, Liang XG, Wu JL, Yang Y, et al. Gut-derived β-amyloid: Likely a centerpiece of the gut–brain axis contributing to Alzheimer’s pathogenesis. Gut Microbes. 2023;15(1):2167172. doi:10.1080/19490976.2023.2167172.
- Yousefi-Ahmadipour A, Rashidian A, Mirzaei MR, Farsinejad A, PourMohammadi-Nejad F, Ghazi-Khansari M, Ai J, Shirian S, Allahverdi A, Saremi J, et al. Combination therapy of mesenchymal stromal cells and sulfasalazine attenuates trinitrobenzene sulfonic acid induced colitis in the rat: the S1P pathway. J Cell Physiol. 2019;234(7):11078–11091. doi:10.1002/jcp.27944.
- Millar AD, Rampton DS, Chander CL, Claxson AW, Blades S, Coumbe A, Panetta J, Morris CJ, Blake DR. Evaluating the antioxidant potential of new treatments for inflammatory bowel disease using a rat model of colitis. Gut. 1996;39(3):407–415. doi:10.1136/gut.39.3.407.
- Kiesler P, Fuss IJ, Strober W. Experimental models of inflammatory bowel diseases. Cell Mol Gastroenterol Hepatol. 2015;1(2):154–170. doi:10.1016/j.jcmgh.2015.01.006.
- Harvey RF, Bradsha JM. A simple index of Crohn’s-disease activity. The Lancet. 1980;315(8167):514. doi:10.1016/s0140-6736(80)92767-1.
- Chen G, Ran X, Li B, Li Y, He D, Huang B, Fu S, Liu J, Wang W. Sodium butyrate inhibits inflammation and maintains epithelium barrier integrity in a TNBS-induced inflammatory bowel disease mice model. EBioMedicine. 2018;30:317–325. doi:10.1016/j.ebiom.2018.03.030.
- Segata N, Izard J, Waldron L, Gevers D, Miropolsky L, Garrett WS, Huttenhower C. Metagenomic biomarker discovery and explanation. Genome Biol. 2011;12:R60. doi:10.1186/gb-2011-12-6-r60.
- Li Y, Dong JL, Xiao HW, Zhang SQ, Wang B, Cui M, Fan SJ. Gut commensal derived-valeric acid protects against radiation injuries. Gut Microbes. 2020;11(4):789–806. doi:10.1080/19490976.2019.1709387.
- Wirtz S, Neufert C, Weigmann B, Neurath MF. Chemically induced mouse models of intestinal inflammation. Nat Protoc. 2007;2(3):541–546. doi:10.1038/nprot.2007.41.
- Larabi AB, Hugo LPM, Bäumler AJ. Bile acids as modulators of gut microbiota composition and function. Gut Microbes. 2023;15(1):2172671. doi:10.1080/19490976.2023.2172671.
- Wahlstrom A, Sayin SI, Marschall HU, Backhed F. Intestinal crosstalk between bile acids and microbiota and its impact on host metabolism. Cell Metab. 2016;24:41–50. doi:10.1016/j.cmet.2016.05.005.
- Zhou X, Cao L, Jiang C, Xie Y, Cheng X, Krausz KW, Qi Y, Sun L, Shah YM, Gonzalez FJ, et al. PPARα-UGT axis activation represses intestinal FXR-FGF15 feedback signalling and exacerbates experimental colitis. Nat Commun. 2014;5(1):4573. doi:10.1038/ncomms5573.
- Song M, Ye J, Zhang F, Su H, Yang X, He H, Liu F, Zhu X, Wang L, Gao P, et al. Chenodeoxycholic acid (CDCA) protects against the lipopolysaccharide-induced impairment of the intestinal epithelial barrier function via the FXR-MLCK pathway. J Agric Food Chem. 2019;67(32):8868–8874. doi:10.1021/acs.jafc.9b03173.
- Li S, Qiu M, Kong Y, Zhao X, Choi HJ, Reich M, Bunkelman BH, Liu Q, Hu S, Han M, et al. Bile acid G protein-coupled membrane receptor TGR5 modulates aquaporin 2–mediated water homeostasis. J Am Soc Nephrol. 2018;29(11):2658–2670. doi:10.1681/ASN.2018030271.
- Siegel CA, Melmed GY. Predicting response to anti-TNF agents for the treatment of Crohn’s disease. Therap Adv Gastroenterol. 2009;2(4):245–251. doi:10.1177/1756283X09336364.
- Arijs I, Li K, Toedter G, Quintens R, Van Lommel L, Van Steen K, Leemans P, De Hertogh G, Lemaire K, Ferrante M, et al. Mucosal gene signatures to predict response to infliximab in patients with ulcerative colitis. Gut. 2009;58(12):1612–1619. doi:10.1136/gut.2009.178665.
- Gevers D, Kugathasan S, Denson LA, Vazquez-Baeza Y, Van Treuren W, Ren B, Schwager E, Knights D, Song SJ, Yassour M, et al. The treatment-naive microbiome in new-onset Crohn’s disease. Cell Host & Microbe. 2014;15:382–392. doi:10.1016/j.chom.2014.02.005.
- Lewis JD, Chen EZ, Baldassano RN, Otley AR, Griffiths AM, Lee D, Bittinger K, Bailey A, Friedman ES, Hoffmann C, et al. Inflammation, antibiotics, and diet as environmental stressors of the gut microbiome in pediatric Crohn’s disease. Cell Host & Microbe. 2015;18:489–500. doi:10.1016/j.chom.2015.09.008.
- Ungaro R, Bernstein CN, Gearry R, Hviid A, Kolho KL, Kronman MP, Shaw S, Van Kruiningen H, Colombel JF, Atreja A. Antibiotics associated with increased risk of new-onset Crohn’s disease but not ulcerative colitis: a meta-analysis. Am J Gastroenterol. 2014;109:1728–1738. doi:10.1038/ajg.2014.246.
- Ihekweazu FD, Fofanova TY, Queliza K, Nagy-Szakal D, Stewart CJ, Engevik MA, Hulten KG, Tatevian N, Graham DY, Versalovic J, et al. Bacteroides ovatus ATCC 8483 monotherapy is superior to traditional fecal transplant and multi-strain bacteriotherapy in a murine colitis model. Gut Microbes. 2019;10:504–520. doi:10.1080/19490976.2018.1560753.
- Shaw KA, Bertha M, Hofmekler T, Chopra P, Vatanen T, Srivatsa A, Prince J, Kumar A, Sauer C, Zwick ME, et al. Dysbiosis, inflammation, and response to treatment: a longitudinal study of pediatric subjects with newly diagnosed inflammatory bowel disease. Genome Med. 2016;8:75. doi:10.1186/s13073-016-0331-y.
- Kong L, Lloyd-Price J, Vatanen T, Seksik P, Beaugerie L, Simon T, Vlamakis H, Sokol H, Xavier RJ. Linking strain engraftment in fecal microbiota transplantation with maintenance of remission in Crohn’s disease. Gastroenterology. 2020;159(6):2193–2202.e5. doi:10.1053/j.gastro.2020.08.045.
- Lee JWJ, Plichta D, Hogstrom L, Borren NZ, Lau H, Gregory SM, Tan W, Khalili H, Clish C, Vlamakis H, et al. Multi-omics reveal microbial determinants impacting responses to biologic therapies in inflammatory bowel disease. Cell Host & Microbe. 2021;29(8):1294–1304.e4. doi:10.1016/j.chom.2021.06.019.
- Jia W, Xie G, Jia W. Bile acid–microbiota crosstalk in gastrointestinal inflammation and carcinogenesis. Nat Rev Gastroenterol Hepatol. 2018;15(2):111–128. doi:10.1038/nrgastro.2017.119.
- Duboc H, Rajca S, Rainteau D, Benarous D, Maubert MA, Quervain E, Thomas G, Barbu V, Humbert L, Despras G, et al. Connecting dysbiosis, bile-acid dysmetabolism and gut inflammation in inflammatory bowel diseases. Gut. 2013;62:531–539. doi:10.1136/gutjnl-2012-302578.
- Hua YL, Jia YQ, Zhang XS, Yuan ZW, Ji P, Hu JJ, Wei YM. Baitouweng Tang ameliorates DSS-induced ulcerative colitis through the regulation of the gut microbiota and bile acids via pathways involving FXR and TGR5. Biomed Pharmacother. 2021;137:111320. doi:10.1016/j.biopha.2021.111320.
- Lajczak-McGinley NK, Porru E, Fallon CM, Smyth J, Curley C, McCarron PA, Tambuwala MM, Roda A, Keely SJ. The secondary bile acids, ursodeoxycholic acid and lithocholic acid, protect against intestinal inflammation by inhibition of epithelial apoptosis. Physiol Rep. 2020;8:e14456. doi:10.14814/phy2.14456.
- Goyal N, Rana A, Bijjem KR, Kumar P. Effect of chenodeoxycholic acid and sodium hydrogen sulfide in dinitro benzene sulfonic acid (DNBS) – Induced ulcerative colitis in rats. Pharmacol Rep. 2015;67(3):616–623. doi:10.1016/j.pharep.2014.12.018.
- Baars A, Oosting A, Knol J, Garssen J, van Bergenhenegouwen J. The gut microbiota as a therapeutic target in IBD and metabolic disease: a role for the bile acid receptors FXR and TGR5. Microorganisms. 2015;3:641–666. doi:10.3390/microorganisms3040641.
- Ke J, Li Y, Han C, He R, Lin R, Qian W, Hou X. Fucose ameliorate intestinal inflammation through modulating the crosstalk between bile acids and gut microbiota in a chronic colitis murine model. Inflamm Bowel Dis. 2020;26:863–873. doi:10.1093/ibd/izaa007.
- Sinha SR, Haileselassie Y, Nguyen LP, Tropini C, Wang M, Becker LS, Sim D, Jarr K, Spear ET, Singh G, et al. Dysbiosis-induced secondary bile acid deficiency promotes intestinal inflammation. Cell Host & Microbe. 2020;27(4):659–670.e5. doi:10.1016/j.chom.2020.01.021.
- Carino A, Biagioli M, Marchiano S, Fiorucci C, Bordoni M, Roselli R, Di Giorgio C, Baldoni M, Ricci P, Monti MC, et al. Opposite effects of the FXR agonist obeticholic acid on Mafg and Nrf2 mediate the development of acute liver injury in rodent models of cholestasis. Biochim Biophys Acta Mol Cell Biol Lipids. 2020;1865(9):158733. doi:10.1016/j.bbalip.2020.158733.
- Fiorucci S, Distrutti E. The pharmacology of bile acids and their receptors. Handb Exp Pharmacol. 2019;256:3–18. doi:10.1007/164_2019_238.
- Alemi F, Poole DP, Chiu J, Schoonjans K, Cattaruzza F, Grider JR, Bunnett NW, Corvera CU. The receptor TGR5 mediates the prokinetic actions of intestinal bile acids and is required for normal defecation in mice. Gastroenterology. 2013;144:145–154. doi:10.1053/j.gastro.2012.09.055.
- Ward JBJ, Lajczak NK, Kelly OB, O’Dwyer AM, Giddam AK, Ni Gabhann J, Franco P, Tambuwala MM, Jefferies CA, Keely S, et al. Ursodeoxycholic acid and lithocholic acid exert anti-inflammatory actions in the colon. Am J Physiol Gastrointest Liver Physiol. 2017;312:G550–G558. doi:10.1152/ajpgi.00256.2016.
- Ajouz H, Mukherji D, Shamseddine A. Secondary bile acids: an underrecognized cause of colon cancer. World J Surg Oncol. 2014;12:164. doi:10.1186/1477-7819-12-164.
- Payne CM, Bernstein C, Dvorak K, Bernstein H. Hydrophobic bile acids, genomic instability, Darwinian selection, and colon carcinogenesis. Clin Exp Gastroenterol. 2008;1:19–47. doi:10.2147/ceg.s4343.