ABSTRACT
Fusobacterium nucleatum (Fn) infection is known to exacerbate ulcerative colitis (UC). However, the link between Fn-infected intestinal epithelial cell (IEC)-derived exosomes (Fn-Exo) and UC progression has not been investigated. Differentially expressed miRNAs in Fn-Exo and non-infected IECs-derived exosomes (Con-Exo) were identified by miRNA sequencing. Then, the biological role and mechanism of Fn-Exo in UC development were determined in vitro and in vivo. We found that exosomes delivered miR-129-2-3p from Fn-infected IECs into non-infected IECs, exacerbating epithelial barrier dysfunction and experimental colitis. Mechanically, Fn-Exo induces DNA damage via the miR-129-2-3p/TIMELESS axis and subsequently activates the ATM/ATR/p53 pathway, ultimately promoting cellular senescence and colonic inflammation. In conclusion, Exo-miR-129-2-3p/TIMELESS/ATM/ATR/p53 pathway aggravates cellular senescence, barrier damage, and experimental colitis. The current study revealed a previously unknown regulatory pathway in the progression of Fn-infectious UC. Furthermore, Exosomal-miR-129-2-3p in serum and TIMELESS may function as novel potential diagnostic biomarkers for UC and Fn-high-UC.
Introduction
Ulcerative colitis (UC) is an inflammatory bowel disease (IBD) distinct from Crohn’s disease (CD), characterized primarily by diffuse, superficial inflammation of the colon and rectumCitation1. Notably, UC increases the risk of disease progression over time, such as the increased risk of hospitalization, tumor development, and other extraintestinal complicationsCitation1. UC may be caused by multiple factors including intestinal flora dysbiosis, genetic background, mucosal immune dysregulation, and environment, but the exact etiology of UC remains unclear to dateCitation2,Citation3. Therefore, deeply exploring the pathogenesis of UC is urgently needed to effectively control disease progression, optimize targeted therapeutic regimens, and improve disease prognosis.
Fusobacterium nucleatum (Fn) is a Gram-negative bacterium enriched in the colonic tissue of UC patients. In our previous studyCitation4, Fn abundance has been shown to be strongly associated with the clinical course and clinical activity of UC. As for the mode of infection, Fn can not only invade the intestinal mucosa directly but also accelerate the UC process through its derived extracellular vesicles (EVs)Citation3–5. Exosomes are EVs with a diameter of 30–200 nm and are derived from almost all cellsCitation6,Citation7. Encapsulating RNA, DNA, proteins, and lipids, exosomes mediate a variety of physiological and pathological processes through transmission between different cellsCitation7. As small non-coding single-stranded RNAs, microRNAs (miRNAs) can be packaged in exosomes to prevent digestion by RNase and enter the circulation to act as potential biomarkers of diseaseCitation8. It has been reported that Fn-infected colorectal cancer cell-derived exosomes promote tumor metastasis by selectively carrying miRNAsCitation9. Moreover, another Gram-negative enteropathogenic bacterium, Enterotoxigenic Bacteroides fragilis (ETBF), exacerbates intestinal inflammation by downregulating miR-149-3p expression in exosomesCitation10. However, whether Fn can alter the expression profile of miRNAs in normal intestinal epithelial cells (IECs)-derived exosomes and thus regulate the development of UC is unknown.
Cellular senescence is a hallmark of aging, including premature senescence induced by external stress and replicative senescence, and has been demonstrated to be closely associated with a variety of pathological processes, including UCCitation11,Citation12. UC is recognized as a disease of premature colon aging, and the accumulation of DNA damage in the colon of UC patients is one of the main features of agingCitation12. Infection with a genotoxigenic Salmonella strain was found to induce DNA damage and cellular senescence in mouse intestineCitation13. However, the effect and mechanism of Fn infection on colonic senescence in mice have not been explored.
In the present study, we identified the miRNAs wrapped within exosomes by sequencing and found that Fn infection significantly altered the miRNAs profile in IECs-derived exosomes, particularly increasing the expression of Exosomal-miR-129-2-3p (Exo-miR-129-2-3p). More importantly, serum Exo-miR-129-2-3p may serve as a sensitive and specific biomarker for the diagnosis of UC and Fn-infected UC. Furthermore, Timeless circadian regulator (TIMELESS), determined as a direct downstream target of miR-129-2-3p, was shown to regulate DNA damage and cellular senescence in dextran sulfate sodium (DSS)-induced murine colitis. Our study collectively demonstrated that Fn-infected IECs-derived exosomes (Fn-Exo) activated the ataxia telangiectasia mutated (ATM)/(ataxia telangiectasia mutated and Rad3-related) ATR/p53 pathway via the miR-129-2-3p/TIMELESS axis, promoting cellular senescence, damaging the intestinal mucosal barrier, and exacerbating experimental colitis. These findings broaden the horizon for UC’s diagnosis, pathogenesis, and targeted therapy, especially for Fn-infected UC.
Results
Characterization of Con-Exo and Fn-Exo
To explore the characterization and function of exosomes derived from Fn-infected IECs, exosomes were isolated and purified from the culture supernatant of fetal human colon (FHC) cells co-cultured with Fn using ultracentrifugation (). Exosomes derived from FHC cells treated with an equal volume of PBS (Con-Exo) were used as controls (). Transmission electron microscopy (TEM) analysis and Nano Sight particle tracking analysis were performed to determine cup-shaped morphology, size, and concentration of purified Con-Exo and Fn-Exo (), as we described previouslyCitation8. We statistically found that the average size of Fn-Exo (119.90 ± 24.89 nm) is larger than that of Con-Exo (92.55 ± 14.37 nm, ). Fn was found to promote exosome secretion in FHC cells, as evidenced by a significantly higher average concentration of Fn-Exo (4.48 ± 0.84 107particles/mL) than Con-Exo (3.03 ± 0.74 107particles/mL, ). Markers of exosomes CD63 and CD9 were detected in both Fn-Exo and Con-Exo by Western blot analysis, indicating that the purified particles were exosomes (). Moreover, the protein expressions of CD63 and CD9 in Fn-Exo were significantly higher than those in Con-Exo of equal volume ( and Figure S1A). These results confirm that Fn-infected IECs secrete exosomes with larger sizes and higher concentrations.
Figure 1. Characterization of Con-Exo and Fn-Exo. (a) Con-Exo and Fn-Exo were extracted from the supernatants of PBS-treated FHC cells and Fn-infected FHC cells, respectively, by ultracentrifugation. (b) Representative TEM images of purified Con-Exo and Fn-Exo. Scale bar = 100 nm. (c) Nanoparticle tracking analysis of number and size distribution in Con-Exo and Fn-Exo. (d and e) Size (d) and concentration (e) of Con-Exo and Fn-Exo. (f) Western blotting analysis of exosomal markers CD63 and CD9 in Con-Exo and Fn-Exo, β-tubulin served as an internal control in whole-cell lysates. (g) Western blot analysis of Con-Exo and Fn-Exo proteins (CD63 and CD9) obtained from the same volume of FHC cells supernatants. Data are represented as means ± SEM. *p < 0.05, **p < 0.01.
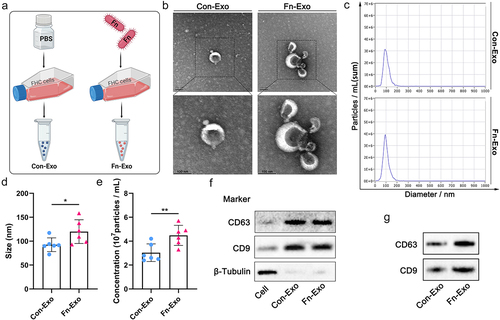
Promoting effect of Fn-Exo on barrier damage and experimental colitis
To further investigate the biological function of Fn-Exo, we co-cultured 10 μg of Dil-labeled Fn-Exo with 1 × 105 Caco-2 cells for 0, 6, 12, and 24 hours. As shown in , exosomes were taken up by Caco-2 cells starting from the 6th hour, with the highest uptake at the 24th hour. Therefore, exosomes were co-cultured with IECs cells in the subsequent cell experiments for 24 hours. Fn-Exo significantly reduced transepithelial electrical resistance (TEER) values in the Caco-2 monolayer cell model compared to the blank controls and Con-Exo treated groups (). Consistently, remarkably increased fluorescein isothiocyanate (FITC) – labeled dextran 4 kDa (FD4) permeability was detected in Caco-2 cells co-cultured with Fn-Exo (). Protein levels of the tight junction proteins (TJ proteins) ZO-1 and Occludin were significantly decreased in Fn-Exo-treated FHC and Caco-2 cells as suggested by Western blot analysis ( and Figure S1B). Immunofluorescence staining also showed that Fn-Exo, but not Con-Exo, significantly reduced the localization and expression of ZO-1 protein at the cell junctions of Caco-2 monolayers (). Thus, Fn-Exo promotes dysfunction and damage to the intercellular barrier of IECs.
Figure 2. Fn-Exo exacerbates barrier damage and experimental colitis. (a) Internalization of Dil-stained exosomes (red) in Caco-2 cells was visualized using a confocal laser scanning microscopy. DAPI (blue) was used to stain nuclei. Scale bar = 10 μm. (b) TEER values of the Caco-2 monolayer cell model. (c) Fold change of FD4 flux in Caco-2 monolayer cells. (d) Western blotting was performed to assess the expression of TJ proteins (ZO-1 and Occludin) in FHC and Caco-2 cells. (e) Representative immunofluorescence images of ZO-1 (red) in Caco-2 monolayers treated with PBS, Con-Exo, or Fn-Exo. Nuclei were stained blue using DAPI (blue). Scale bar = 20 μm. (f) Representative morphology and length of mice colons. (g and h) Body weight loss (g) and disease activity indices (DAI) (h) of experimental colitis mice and blank control animals (n = 8). (i) Intestinal barrier dysfunction in mice was evaluated by measuring plasma FITC-dextran levels (n = 8). (j) Mean ± SEM of mouse colonic histological scores (n = 8). (k) Hematoxylin and eosin (H&E) staining of mouse colon cross-sections. Scale bar = 50 μm. (l) Immunoblot analysis was performed to assess the expression of ZO-1 and Occludin in mouse colon tissue. (m) Immunohistochemistry (IHC) staining of ZO-1 in colon sections of mice. Scale bar = 20 μm. Data are represented as means ± SEM. *p < 0.05, **p < 0.01, ***p < 0.001, ****p < 0.0001.
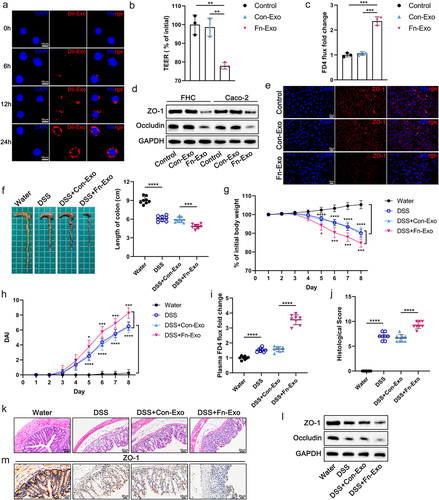
Next, we constructed an experimental murine colitis model to investigate the pro-inflammatory effects of Fn-Exo in vivo. We found that Fn-Exo infection significantly exacerbated DSS-induced colitis in mice, as manifested by shorter colon length (), more weight loss (), and higher disease activity index (DAI) scores (). Fn-Exo-gavaged colitis mice had significantly increased FD4 flux from the intestinal lumen to blood compared with DSS and Con-Exo-treated mice (). Histological analysis showed that Fn-Exo infection promoted inflammatory cell infiltration and severe damage to the intestinal epithelium in mouse colon (). The expression of TJ proteins (ZO-1 and Occludin) in colon tissue of DSS and Fn-Exo-stimulated mice was significantly lower than that of mice treated with water, DSS, or DSS and Con-Exo ( and Figure S1C). Immunohistochemical analysis of ZO-1 in mouse colon cross-sections also suggested that Fn-Exo infection decreased TJ protein expression (). However, no differences were found between the DSS-treated group and the DSS and Con-Exo-cotreated group for the above indicators. These data clarify that Fn-Exo aggravates cellular barrier damage and DSS-induced experimental colitis.
Exo-miR-129-2-3p may function as a potential Fn-high-UC diagnosis biomarker
We further performed miRNA sequencing analysis to identify differentially expressed miRNAs between Con-Exo and Fn-Exo, exploring the specific miRNA profile associated with Fn-Exo infection. A total of 100 differentially expressed miRNAs were identified, of which 99 miRNAs were expressed in both Con-Exo and Fn-Exo ( and Table S1). Compared with Con-Exo, 56 miRNAs were upregulated and 43 miRNAs were downregulated in Fn-Exo (). There were 18 miRNAs with significant differences in expression between Con-Exo and Fn-Exo (log2(|fold change|) > 2, Q-value <0.001, , and Table S2). The top 10 up-regulated miRNAs with the larger log2(|fold change|) values were selected for further screening. qRT-PCR validation analysis showed that among the exosomes derived from Fn-infected FHC cells, Caco-2 cells, and NCM460 cells, only miR-129-2-3p expression was significantly elevated ( and Figures S2A-S2C).
Figure 3. Exo-miR-129-2-3p may function as a potential Fn-high-UC diagnosis biomarker. (a) Venn diagram showing the unique and overlapping differential miRNAs between Con-Exo and Fn-Exo. (b) The up-and down-regulation of these 99 miRNAs. (c) Volcano plots of these 18 differentially expressed miRNAs. (d) Heatmap showing the profiles of differentially expressed miRNAs in Con-Exo and Fn-Exo. (e) Detection of relative expression levels of Exo-miR-129-2-3p in Con-Exo and Fn-Exo derived from 3 cell lines by qRT-PCR. (f and g) qRT-PCR analysis of Exo-miR-129-2-3p expression in serum of mice in different treatment groups (n = 6). (h) Relative Exo-miR-129-2-3p levels in healthy volunteers (n = 24) and UC patients (n = 72) serum. (i) The sensitivity and specificity of serum Exo-miR-129-2-3p for UC prediction were evaluated through receiver-operating characteristic (ROC) curve analysis. (j) qRT-PCR analysis of Exo-miR-129-2-3p expression in serum of healthy volunteers (n = 24), Fn-negative/low-UC patients (n = 30) and Fn-high-UC patients (n = 42). (k) ROC curve analysis assessed the sensitivity and specificity of serum Exo-miR-129-2-3p for the diagnosis of Fn-high-UC. Data are represented as means ± SEM. *p < 0.05, **p < 0.01, ***p < 0.001, ****p < 0.0001; NS, not significant.
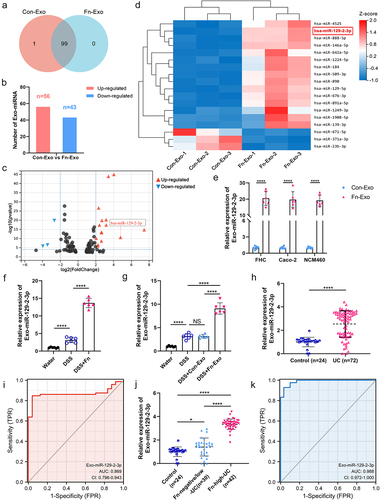
Subsequently, we extracted and characterized exosomes from human and mouse serum (Figures S3A-S3C). As shown in , both gavage of Fn and Fn-Exo significantly upregulated the expression of Exo-miR-129-2-3p in the serum of DSS-induced colitis mice. Exo-miR-129-2-3p was found to be significantly enriched in the serum of UC patients compared with healthy controls (). In addition, we assessed the correlation between the serum Exo-miR-129-2-3p levels and the clinicopathological features of UC patients listed in Table S3. A positive correlation was observed between serum Exo-miR-129-2-3p expression and the clinical course (p = 0.003), clinical activity of UC (p = 0.018), and refractory UC (p = 0.006, Table S3). The results of the ROC curve used to assess Exo-miR-129-2-3p diagnostic efficacy for UC determined an AUC of 0.869 (95%CI, 0.796–0.943), a sensitivity of 84.70%, and a specificity of 95.80% (). Importantly, the relative levels of Exo-miR-129-2-3p in serum of Fn-enriched (Fn > 20) UC patients (Fn-high-UC) were significantly higher than those of healthy controls and non-Fn-enriched (Fn < 20) UC patients (Fn-negative/low-UC, ). Then, the sensitivity and specificity of Exo-miR-129-2-3p for Fn-high-UC and Fn-negative/low-UC diagnosis were evaluated using ROC curves. As shown in , the AUC was 0.988 (95%CI, 0.972–1.000), with a sensitivity of 92.90% and a specificity of 96.70%. Hence, up-regulation of serum Exo-miR-129-2-3p expression is indicative of Fn infection and active, severe UC. Serum Exo-miR-129-2-3p may function as a potential diagnostic biomarker for UC, especially Fn-high-UC. Therefore, we select miR-129-2-3p for further investigation and suggest that Fn-Exo may regulate UC progression through Exo-miR-129-2-3p.
Exo-miR-129-2-3p facilitates barrier damage and DSS-induced colitis
In order to clarify whether Exo-miR-129-2-3p promoted colitis progression, we transfected FHC and Caco-2 cells with NC/miR-129-2-3p-mimics/inhibitor-NC/miR-129-2-3p-inhibitor, respectively. The relative expression of miR-129-2-3p in the above cells and cell-derived exosomes were examined separately to determine the effectiveness of transfection (Figures S4A, S4B). Exosomes extracted from the supernatant of transfected-FHC cells were subsequently co-cultured with Caco-2 cells for 24 h. Both significantly lower TEER values and notably increased FD4 flow in the Exo-mimics group compared to the Exo-NC group suggested that overexpression of Exo-miR-129-2-3p impaired the barrier function of Caco-2 monolayer cells (). Conversely, compared with the Exo-inhibitor-NC group, knockdown of Exo-miR-129-2-3p (Exo-inhibitor) enhanced cellular barrier function, exhibiting a marked increase in TEER values and a significant downregulation of plasma FD4 flux (). Additionally, western blot analysis and immunofluorescence staining showed that Exo-miR-129-2-3p-mimics damaged intercellular TJ proteins including ZO-1, whereas Exo-miR-129-2-3p-inhibitor protected intercellular tight junctions ( and Figure S1D).
Figure 4. Exo-miR-129-2-3p facilitates barrier damage and DSS-induced colitis. (a and b) Quantitative analysis of TEER values (a) and FD4 flux (b) in Caco-2 monolayer cell models with different treatments. (c) Western blot analysis of TJ proteins (ZO-1 and Occludin) in Caco-2 cells. (d) Representative immunofluorescence photomicrographs of fluorescent localization and expression of ZO-1 (red) in Caco-2 cells. Nuclei were stained with DAPI (blue). Scale bar = 20 μm. (e) Representative photographs and quantitative analysis of mice colons in different treatment groups (n = 8). (f-i) Effect of Exo-miR-129-2-3p on the body weight (f), DAI (g), plasma FD4 fold change (h), and histological score (i) in DSS-induced colitis mice (n = 8). (j) H&E – stained images of colon cross-sections in mice after different treatments. Scale bar = 50 μm. (k) Expression of ZO-1 and Occludin in mouse colon tissue was detected by Western blotting. (l) Representative images of IHC staining of ZO-1. Scale bar = 20 μm. Data are represented as means ± SEM. *p < 0.05, **p < 0.01, ***p < 0.001, ****p < 0.0001.
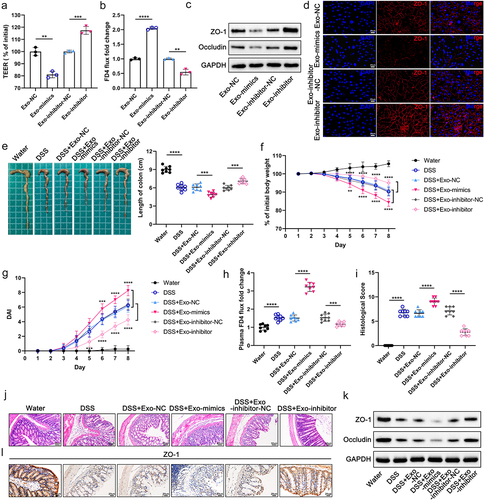
To further investigate the disruptive effect of Exo-miR-129-2-3p on the colonic mucosal barrier, we administered mice with differently transfected-FHC cells-derived exosomes by gavage using the same method as infection with Con-Exo and Fn-Exo. As speculated, in vivo experiments revealed that Exo-miR-129-2-3p-mimics promoted colonic shortening (), weight loss (), increased DAI scores (), and elevated FD4 flow () in DSS-induced colitis mice. Congruously, Hematoxylin and eosin (H&E)-stained images indicated that Exo-miR-129-2-3p-mimics upregulated histological scores and exacerbated DSS-induced colonic mucosal injury (). However, gavage of Exo-miR-129-2-3p-inhibitor attenuated DSS-induced colonic inflammatory symptoms and intestinal mucosal barrier damage in mice (.Exo-miR-129-2-3p-mimics aggravated the deficiency of ZO-1 and Occludin protein expression in colon tissue of colitis mice, whereas Exo-miR-129-2-3p-inhibitor rescued the reduction of TJ proteins ( and Figure S1E). The trend changes of ZO-1 immunohistochemical staining in were consistent with the results of the western blot analysis described above. These results confirm that Exo-miR-129-2-3p damages the barrier both in vitro and in vivo, facilitating DSS-induced colitis in mice, which is consistent with the trend of Fn-Exo’s role in colitis.
Identification of TIMELESS as the miR-129-2-3p target gene
It is known that miRNAs negatively regulate the mRNA levels of target genes by directly binding to their 3’-UTRCitation3,Citation14,Citation15. We conducted RNA sequencing to probe differential mRNA profiles between NC-transfected FHC cells and miR-129-2-3p-mimics-transfected FHC cells. A total of 12,643 differentially expressed mRNAs were identified by sequencing, among which 11,684 mRNAs were expressed in both groups of cells (5778 upregulated and 5906 downregulated, ). Further analysis revealed that 823 mRNAs were significantly under-expressed in FHC cells transfected with miR-129-2-3p-mimics (log2(fold change) < −1.5, Q-value <0.001, ). Prediction of potential target genes of miR-129-2-3p using a combination of RNA sequencing results and 3 bioinformatics tools (miRanda, RNAhybrid, and TargetScan, ). Among the 3 predicted potential target genes, only TIMELESS, but not SH2B1 or RCBTB2 was significantly low expressed in miR-129-2-3p-mimics-transfected IECs and significantly highly expressed in IECs transfected with miR-129-2-3p-inhibitor, as shown by qRT-PCR ( and Figures S5A, S5B). The protein level of TIMELESS also reduced with the upregulation of miR-129-2-3p expression, and increased with the decrease of miR-129-2-3p expression, in FHC and Caco-2 cells ( and Figure S1F).
Figure 5. Identification of TIMELESS as a direct downstream target of miR-129-2-3p. (a) The up-and down-regulation of differential mRNAs between FHC cells transfected with NC and miR-129-2-3p-mimics. (b) Volcano plot of differentially expressed mRNAs. (c) Heatmap showing differentially expressed mRNAs between FHC cells transfected with NC and miR-129-2-3p-mimics. (d) RNA-seq, miRanda, RNAhybrid, and TargetScan were used to predict miR-129-2-3p target genes. (e and f) qRT-PCR (e) and Western blot analysis (f) of TIMELESS expression in FHC and Caco-2 cells transfected with miR-129-2-3p-mimics/NC or miR-129-2-3p-inhibitor/inhibitor-NC. (g) TIMELESS expression in colon tissues of healthy controls (n = 24) and UC patients (n = 72) was analyzed by qRT-PCR. (h) The ROC curve to evaluate the diagnostic value of TIMELESS for UC. (i) qRT-PCR analysis of TIMELESS expression in colon tissues of healthy volunteers (n = 24), Fn-negative/low-UC patients (n = 30), and Fn-high-UC patients (n = 42). (j) The diagnostic value of TIMELESS for Fn-high-UC was evaluated by the ROC curve. (k) Western blot analysis of TIMELESS protein levels in colon tissues of healthy volunteers (n = 4), Fn-negative/low-UC patients (n = 4), and Fn-high-UC patients (n = 4). (l) Upper panel: representative FISH images to assess the abundance of Fn in tissues from healthy controls, Fn-negative/low-UC patients, and Fn-high-UC patients. Lower panel: representative images of IHC staining analysis of TIMELESS expression. Scale bar = 20 μm. (m) The binding site of wild-type TIMELESS with miR-129-2-3p (AAGGGCU), and mutant sequence of TIMELESS 3ʹ-UTR (UUCCCGA). (n) Dual-luciferase assay was performed to investigate the direct binding between miR-129-2-3p and TIMELESS in FHC and Caco-2 cells. Data are represented as means ± SEM. **p < 0.01, ***p < 0.001, ****p < 0.0001; NS, not significant.
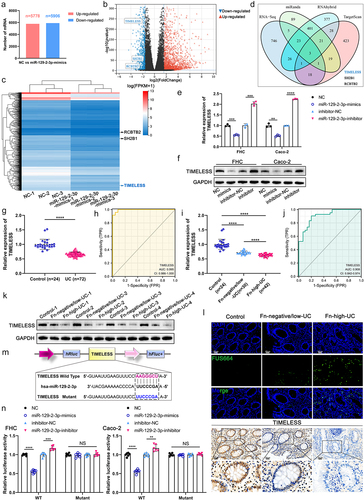
Circadian protein TIMELESS expression was reported to be decreased in mucosal epithelium of UC patientsCitation16. Here, significantly reduced TIMELESS mRNA levels were detected using qRT-PCR analysis in colon tissue of UC patients (). The AUC of 0.995 (95%CI, 0.986–1.000), sensitivity, and specificity both of 95.80% indicated the diagnostic efficacy of TIMELESS for UC (). In addition, the RNA level of TIMELESS in colon tissue of Fn-high-UC patients was found to be significantly lower, compared with that of healthy controls and Fn-negative/low-UC patients (). The AUC shown in was 0.908 (95%CI, 0.842–0.974). The sensitivity and specificity of using TIMELESS for diagnosing Fn-high-UC were 90.50% and 80.00%, respectively (). At the protein level, TIMELESS was significantly lacking in the colonic tissues of UC patients, notably in Fn-high-UC tissues ( and Figure S1G). We then examined Fn abundance and TIMELESS expression in UC tissue sections by FISH and immunohistochemical staining, respectively, and found that Fn invasion in UC tissues was accompanied by a dramatic downregulation of TIMELESS expression ().
According to the prediction of the TargetScan database, miR-129-2-3p has a direct binding site to TIMELESS, and the complementary binding sequence was shown in . The luciferase activity in FHC and Caco-2 cells overexpressing miR-129-2-3p was significantly reduced. Inversely, inhibition of miR-129-2-3p expression significantly improved the luciferase activity (). However, after mutating the binding site between miR-129-2-3p and TIMELESS, no effect of altered miR-129-2-3p levels on luciferase activity was detected (). The above results indicate that miR-129-2-3p directly targets TIMELESS, and the decrease of TIMELESS expression is strongly associated with Fn infection. In this context, we speculate that the exacerbating effect of Fn-Exo on colitis is associated with the miR-129-2-3p/TIMELESS axis.
Fn-Exo promotes cellular senescence and DNA damage via the miR-129-2-3p/TIMELESS axis
Nowadays, how TIMELESS regulates UC progress has not been reported. To explore the mechanism of TIMELESS in Fn-related colitis and the signal pathway involved, we further combined the STRING online database and DAVID online tool to conduct Kyoto Encyclopedia of Genes and Genomes (KEGG) pathway enrichment analysis on TIMELESS. 17 significantly enriched signaling pathways including cellular senescence were visualized in . Therefore, we hypothesized that TIMELESS is involved in the regulation of cellular senescence. We then constructed 3 siTIMELESS to transfect FHC and Caco-2 cells respectively, and 1 siTIMELESS with the best knockdown effect was selected for subsequent cell experiments by qRT-PCR and Western blot analysis (Figures S6A-S6D). SA-β-Gal was detected to accumulate abundantly in siTIMELESS-transfected FHC cells, indicating that inhibition of TIMELESS expression accelerates cellular senescence (). However, the increased proportion of SA-β-Gal-positive cells caused by siTIMELESS was observably reduced after suppressing miR-129-2-3p expression (). Similarly, the elevation of senescence-associated proteins (p53, p21, and p16) expression due to knockdown of TIMELESS was curbed by transfection with miR-129-2-3p-inhibitor ( and Figure S1H). Considering that DNA damage is a powerful trigger of cellular senescenceCitation17, we were curious whether TIMELESS-regulated cellular senescence is associated with DNA damage. We monitored the signal of canonical DNA damage marker γH2AX by immunoblotting and fluorescence confocal microscopy, and found that depletion of TIMELESS greatly promoted the accumulation of γH2AX protein ( and Figure S1H) and γH2AX foci (). On this basis, miR-129-2-3p-inhibitor accelerated the clearance of γH2AX, indicating efficient DNA repair ( and Figure S1H). Furthermore, Comet assays demonstrated that the severe DNA fragmentation triggered by siTIMELESS was prominently restored after miR-129-2-3p-inhibitor transfection (). Thus, TIMELESS depletion-induced cellular senescence and DNA damage are miR-129-2-3p-dependent.
Figure 6. Fn-Exo promotes cellular senescence and DNA damage via the miR-129-2-3p/TIMELESS axis. (a) KEGG pathway enrichment analysis of TIMELESS. (b) Quantitative analysis and representative images of SA-β-Gal staining in FHC cells. Scale bar = 20 μm. (c) The protein levels of TIMELESS, p53, p21, p16, γH2AX, and H2AX were detected by Western blot in FHC cells transfected with NC, siTIMELESS, or siTIMELESS and miR-129-2-3p-inhibitor. (d) Representative immunofluorescent images showing the γH2AX foci (red) in FHC cells transfected with NC, siTIMELESS, or siTIMELESS and miR-129-2-3p-inhibitor. DAPI (blue) was used to visualize nuclei. Scale bar = 10 μm. (e) Representative micrographs showing Comet analysis. Scale bar = 50 μm. (f) Quantitative analysis and representative images of SA-β-Gal staining of FHC cells in different treatment groups. Scale bar = 20 μm. (g) Western blot analysis of TIMELESS, p53, p21, p16, γH2AX, and H2AX protein levels in FHC cells with different treatments. (h) Immunofluorescence staining was performed to analyze the protein levels of γH2AX (red) in FHC cells. DAPI (blue) was used to visualize nuclei. Scale bar = 10 μm. (i) Representative micrographs showing Comet assay in 5 different treatment groups. Scale bar = 50 μm. Data are represented as means ± SEM. **p < 0.01, ***p < 0.001, ****p < 0.0001.
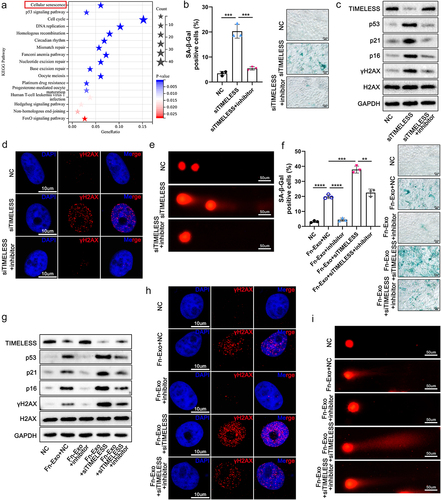
Increased invasion of Fn was observed in senescent human gingival fibroblastsCitation18, but whether Fn-Exo is involved in the regulation of senescence in IECs remains unknown. Herein, SA-β-Gal staining and detection of senescence markers p53, p21, and p16 confirmed the aggravation of FHC cell senescence by Fn-Exo ( and Figure S1I). However, suppression of miR-129-2-3p expression downregulated Fn-Exo-accentuated cellular senescence ( and Figure S1I). Importantly, further exacerbation of Fn-Exo-induced senescence by siTIMELESS was counteracted by miR-129-2-3p-inhibitor transfection ( and Figure S1I). Subsequently, the synergistic effect of Fn-Exo and siTIMELESS in promoting H2AX phosphorylation and γH2AX enrichment in the nucleus was attenuated with the knockdown of miR-129-2-3p ( and Figure S1I). Finally, as shown in Comet assays results in , the promoting effect of Fn-Exo on nuclear DNA breakage could be mitigated by miR-129-2-3p-inhibitor, even when cells were transfected with siTIMELESS, which promotes DNA damage. In sum, our finding is that Fn-Exo exacerbates miR-129-2-3p/TIMELESS axis-dependent cellular senescence and DNA damage.
TIMELESS contributes to cellular senescence through the ATM/ATR/p53 pathway
Then, protein-protein interaction network for TIMELESS and the other 16 genes involved in cellular senescence signaling pathways were constructed using the STRING online database and Cytoscape software, including a total of 17 nodes (). ATM, ATR, checkpoint kinase 2 (CHEK2), and checkpoint kinase 1 (CHEK1) were recognized as the 4 core targets of TIMELESS (). In the presence of DNA damage, ATM and ATR are activated by phosphorylation as key DNA damage response proteins, which phosphorylate checkpoint kinases (CHEK2 and CHEK1, respectively), thereby regulating cellular senescence, cell cycle, and other cells’ biological processesCitation11,Citation19,Citation20. In this context, we speculated that TIMELESS modulates cellular senescence via ATM/CHEK2 and/or ATR/CHEK1 signaling pathways. The Western blot analysis manifested that the phosphorylation levels of ATM, CHEK2, ATR, and CHEK1 increased as the TIMELESS protein level was down ( and Figure S1J). To further determine whether ATM or ATR activation is required for TIMELESS-regulated cellular senescence, we pretreated FHC cells with ATM inhibitor KU60019, ATR inhibitor AZD6738 and ATM/ATR co-inhibitor CGK733, respectively. Both KU60019 and AZD6738 pretreatment were proven to reduce the proportion of SA-β-Gal positive cells clearly (). Nevertheless, CGK733 showed significantly better efficiency than KU60019 and AZD6738 in a reversal of siTIMELESS-induced massive accumulation of SA-β-Gal in FHC cells (). Therefore, we believe that both ATM/CHEK2 and ATR/CHEK1 signaling pathways are involved in the regulation of cellular senescence by TIMELESS.
Figure 7. TIMELESS accelerates cellular senescence through the ATM/ATR/p53 pathway. (a) Protein-protein interaction networks for TIMELESS and 16 other genes involved in cellular senescence signaling pathways were constructed using the STRING online database and Cytoscape software. (b) Protein levels of TIMELESS, p-ATM, ATM, p-CHEK2, CHEK2, p-ATR, ATR, p-CHEK1, and CHEK1 in FHC cells transfected with NC or siTIMELESS were determined using western blotting assay. (c) Quantitative analysis and representative images of SA-β-Gal staining of FHC cells in 5 different treatment groups. Scale bar = 20 μm. (d) Western blot analysis of p-p53, p53, and p21 in FHC cells. The internal control was GAPDH. (e) Quantitative analysis and representative images of SA-β-Gal staining of FHC cells treated with NC and DMSO, siTIMELESS and DMSO, or siTIMELESS and Pifithrin-α. Scale bar = 20 μm. (f) Protein levels of p53 and p21 were evaluated by western blot analysis in FHC cells with different treatments. Data are represented as means ± SEM. **p < 0.01, ***p < 0.001, ****p < 0.0001.
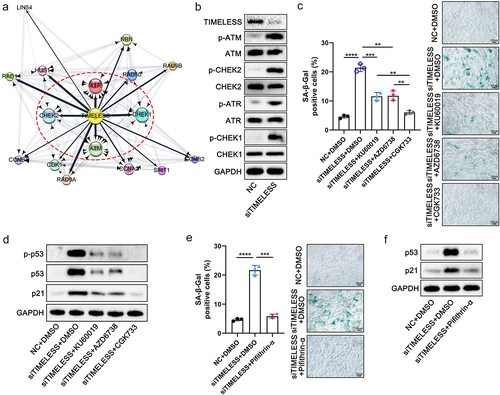
As one of the essential pathways during cellular senescence and DNA damage, the p53 signaling pathway was also identified in the KEGG pathway enrichment analysis illustrated in . We observed that the phosphorylated and total proteins of p53 and the protein level of p21 were markedly increased after transfection of siTIMELESS, whereas the inhibitors KU60019 and AZD6738, particularly CGK733, significantly decreased the expression of these proteins ( and Figure S1K). Pifithin-α, a small molecule inhibitor of p53 signaling, was shown to alleviate TIMELESS depletion-induced cellular senescence, as manifested by a distinct reduction in SA-β-Gal staining in FHC cells (). Pifithin-α significantly reduced p21 protein levels in TIMELESS-silenced cells, indicating that p21 expression here is p53-dependent and that TIMELESS modulates the canonical p53-p21 signaling pathway ( and Figure S1L). These results demonstrate that TIMELESS depletion exacerbates cellular senescence by activating both ATM and ATR signaling pathways and subsequently phosphorylating the p53 signaling pathway. Moreover, the upregulation of protein expression of cellular senescence-associated proteins (p53 and p21) closely related to the p53 pathway caused by Fn-Exo was significantly inhibited by Pifithin-α (Figures S7A, S7B). Hence, we suggest that the contribution of Fn-Exo to colitis may be closely related to ATM/ATR/p53 pathway activation and cellular senescence promoted by the miR-129-2-3p/TIMELESS axis.
Fn-Exo exacerbates experimental colitis by activating the ATM/ATR/p53 pathway via the miR-129-2-3p/TIMELESS axis
To determine the role and mechanism of the miR-129-2-3p/TIMELESS axis in mice with Fn-Exo-promoted colitis, we infected mice with adeno-associated virus (AAV)-miR-129-2-3p-sponge or AAV-shTIMELESS by enema, silencing miR-129-2-3p or TIMELESS expression in mouse colon tissue, respectively. By detecting and analyzing the colon length (), body weight (), DAI scores (), and histological scores of mice (), we found that AAV-miR-129-2-3p-sponge significantly alleviated the colitis of mice caused by DSS and Fn-Exo, whereas AAV-shTIMELESS aggravated the colitis. Notably, AAV-miR-129-2-3p-sponge rescued AAV-shTIMELESS damage to the colon of colitis mice (). As expected, AAV-miR-129-2-3p-sponge evidently mitigated the DSS- and Fn-Exo-induced reduction of TJ proteins in mice colon tissue ( and Figure S1M). Additionally, the negative regulation of TJ proteins expression by AAV-shTIMELESS was reversed by AAV-miR-129-2-3p-sponge infection ( and Figure S1M). Hence, we clarify that AAV-miR-129-2-3p-sponge attenuates the aggravating effect of Fn-Exo on DSS-induced colitis, while the promoting effect of AAV-shTIMELESS on Fn-Exo-related colitis in mice depends on miR-129-2-3p.
Figure 8. Fn-Exo exacerbates experimental colitis by activating the ATM/ATR/p53 pathway via the miR-129-2-3p/TIMELESS axis. (a-d) Representative morphology and length of colons (a), body weight loss (b), DAI (c), and histological score (d) of experimental colitis mice in 5 different treatment groups (n = 8). (e) H&E staining of mouse colon cross-sections after different treatments. Scale bar = 50 μm. (f) Representative images of IHC staining of ZO-1 in mouse colon cross-sections. Scale bar = 20 μm. (g) Immunoblot analysis was conducted to assess the expression of ZO-1 and Occludin using proteins extracted from colon tissues. GAPDH was used as an internal control. (h) Western blotting analysis of p53, p21, γH2AX, and H2AX in mice colon tissues. (i) SA-β-Gal staining of mouse colon cross-sections. Scale bar = 20 μm. (j-l) Representative immunofluorescence images showing the expression and distribution of p-ATM (red, j), p-ATR (red, k), and p-p53 (red, l) in mouse colonic epithelium. Nuclei were stained blue using DAPI. Scale bar = 20 μm. Data are represented as means ± SEM. **p < 0.01, ***p < 0.001, ****p < 0.0001.
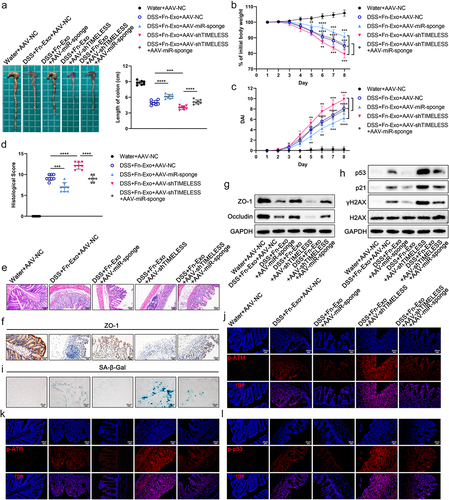
We next conducted Western blot analysis and SA-β-Gal staining, which showed that AAV-miR-129-2-3p-sponge significantly reduced the expression of p53, p21, and γH2AX proteins and the number of SA-β-Gal positive cells in colon tissue of DSS-and Fn-Exo-treated mice regardless of infection with AAV-shTIMELESS ( and Figure S1N). Moreover, the promotion of AAV-shTIMELESS on the above proteins’ expression as well as on the accumulation of SA-β-Gal could only be detected in the colon tissue of colitis mice not infected with AAV-miR-129-2-3p-sponge ( and Figure S1N). Thus, Fn-Exo facilitates cellular senescence in colitis mice through the miR-129-2-3p/TIMELESS axis. Compared to DSS, Fn-Exo, and AAV-NC-treated mice, p-ATM, p-ATR, and p-p53 proteins were significantly reduced in the colon cross-sections of mice infected with DSS, Fn-Exo, and AAV-miR-129-2-3p-sponge, whereas dramatically increased in the colon cross-sections of mice infected with DSS, Fn-Exo, and AAV-shTIMELESS (). Interestingly, activation of ATM, ATR, and p53 protein phosphorylation by AAV-shTIMELESS was detected to be significantly inhibited by AAV-miR-129-2-3p-sponge (). Together, these results indicate that Fn-Exo activates the ATM/ATR/p53 pathway via the miR-129-2-3p/TIMELESS axis, ultimately exacerbating experimental colitis.
Inhibition of the ATM/ATR/p53 pathway alleviates the exacerbating effect of Fn-Exo on experimental colitis and cellular senescence
To further elucidate the critical role of the ATM/ATR/p53 pathway in the acceleration of DSS-induced colitis progression by Fn-Exo, we inhibited ATM/ATR or p53 signaling in mice using CGK733 or Pifithin-α, respectively. Less colonic shortening (), higher body weight (), lower DAI scores (), lower histological scores (), and milder intestinal mucosal damage () were detected in both groups of mice treated with CGK733 and Pifithin-α. Moreover, the expression of TJ proteins was significantly increased in colonic tissue of mice additionally treated with CGK733 or Pifithin-α compared to mice stimulated with DSS and Fn-Exo ( and Figure S1O). We then assessed the expression of senescence marker proteins p53 and p21 in the colon of colitis mice by Western blot analysis and immunofluorescence staining, confirming that CGK733 and Pifithin-α effectively inhibited the upregulation of Fn-Exo on p53 and p21 proteins ( and Figure S1P). Consistently, SA-β-Gal staining suggested that both CGK733 and Pifithin-α significantly improved cellular senescence in colon cross-sections of DSS and Fn-Exo-treated mice (). Neither intestinal inflammation nor cellular senescence levels in colonic tissue were obviously different between CGK733 and Pifithin-α treated colitis mice. Overall, our results demonstrate that inhibiting the ATM/ATR/p53 pathway significantly alleviates Fn-Exo-exacerbated experimental colitis and cellular senescence in mice.
Figure 9. Inhibition of the ATM/ATR/p53 pathway alleviates the exacerbating effect of Fn-Exo on experimental colitis and cellular senescence. (a) Representative pictures of mouse colon morphology and statistical analysis of colonic length (n = 8). (b-d) Colitis severity in mice was evaluated by body weight loss (b), DAI (c), and histological score (d) (n = 8). (e) Representative images of H&E staining analyses. Scale bar = 50 μm. (f) Representative images of IHC staining of ZO-1 in mouse colon cross-sections. Scale bar = 20 μm. (g and h) Western blot assay was performed to measure the protein levels of ZO-1(g), Occludin (g), p53(h), and p21 (h) in colon tissues. GAPDH was used as an internal control. Groups are identified as shown. (i and j) The expression of p53 (i) and p21 (j) in colon cross-sections of mice was detected by fluorescence microscopy. Scale bar = 20 μm. (k) CGK733 and Pifithrin-α reduced the accumulation of SA-β-Gal in colon cross-sections of DSS- and Fn-Exo-treated mice. Scale bar = 20 μm. Data are represented as means ± SEM. *p < 0.05, **p < 0.01, ***p < 0.001, ****p < 0.0001.
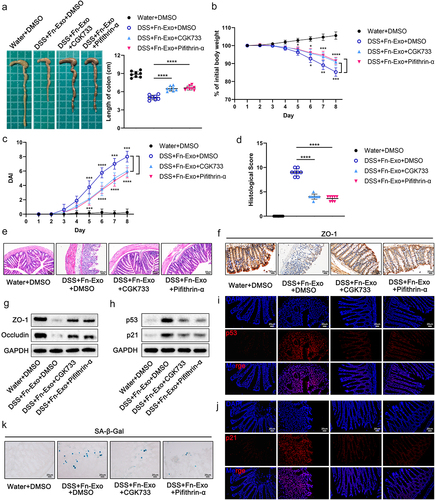
Discussion
UC is a type of chronic IBD with complex pathogenesisCitation2. It was widely believed that host-microbial cross-talk is essential for maintaining intestinal homeostasis. Gut dysbiosis accompanied by disruption of host-microbial mutualism has been implicated as one of the major etiologies of UCCitation21. Fn, a Gram-negative intestinal opportunistic pathogen, has been confirmed to be markedly enriched in the colon tissues of UC patientsCitation4. Mechanistically, Fn exacerbated UC through multiple pathways (including activation of the IL-17F/NF-κB signaling pathway and induction of autophagic cell death), as we previously reportedCitation4,Citation22. More importantly, studies have gradually realized that Fn could disrupt the intestinal mucosal barrier through its derived EVs (Fn-EVs), thereby promoting intestinal inflammationCitation5,Citation23. Our recently published study indicated that Fn-EVs exacerbated experimental colitis by regulating autophagy through the miR-574-5p/CARD3 axis. Targeting the Fn-EV-activated signaling pathway may be a potential therapeutic strategy for UCCitation3. Thus, an in-depth investigation of the pathogenic mechanism of Fn in UC is of great significance for intervening in UC development.
Exosomes are one of the major subtypes of EVs, which may be involved in the treatment and progression of UCCitation10,Citation24–26. Yang et alCitation24 reported that DSS-induced experimental colitis could be effectively relieved by oral administration of autologous exosomes obtained during the healing phase. A recent study detected exosome-like nanovesicles derived from edible turmeric with anti-inflammatory and colitis-preventive effectsCitation25. However, to date, there is a lack of research on the role of Fn-Exo in UC. Here, we found that Fn promoted the secretion of exosomes from FHC cells, which is similar to the discovery of Guo et alCitation9.Functionally, our data showed that Fn-Exo markedly exacerbated barrier damage and experimental colitis. Furthermore, M2 macrophage-derived Exosomal-miR-590-3p (Exo-miR-590-3p) has been discovered to promote epithelial repair and alleviate DSS-induced colitisCitation26. Another study revealed that ETBF regulated TH17 cell differentiation through inhibiting exosomes-packaged miR-149-3p, which subsequently promoted intestinal inflammationCitation10. Therefore, we put forward our hypothesis for the first time that Exo-miRNA derived from Fn-infected IECs may participate in regulating UC progression. In our study, we explored the differentially expressed miRNAs packaged within Con-Exo and Fn-Exo by miRNA sequencing, and found that Exo-miR-129-2-3p was significantly highly expressed in the culture supernatant of Fn-infected FHC cells.
Increasing evidence has shown that miRNAs are important in modulating inflammation-related diseases and even malignanciesCitation10,Citation26–28. For instance, miR-129-2-3p has been reported to affect wound healing through its involvement in diabetic-derived neutrophil dysfunctionCitation29. A previous study demonstrated that miR-129-2-3p was significantly suppressed in Epstein-Barr virus-associated gastric cancer (GC), and inhibition of miR-129-2-3p expression facilitated proliferation, migration, and invasion of GC cellsCitation30. Additionally, gastric juice miR-129-2-3p was suggested as a potential biomarker for screening GC, which may be used for a convenient noninvasive diagnosis of GCCitation31. DARS-AS1, a chronic unpredictable mild stress-responsive lncRNA, significantly enhanced triple-negative breast cancer migration and invasion by downregulating miR-129-2-3p expressionCitation32. However, it remains unclear whether and how miR-129-2-3p contributes to the DSS-induced intestinal pathogenic process. Here, our results clarified the role of Exo-miR-129-2-3p in the disruption of intercellular barrier function and the promotion of experimental colitis in mice. Notably, serum Exo-miR-129-2-3p was suggested to be a potential UC diagnostic biomarker with high sensitivity and specificity in this study.
Herein, by combining RNA sequencing, bioinformatics prediction, and qRT-PCR validation, TIMELESS, which was negatively regulated by miR-129-2-3p and significantly reduced in colon tissue of Fn-high UC patients, was identified as the direct target of miR-129-2-3p. Studies have shown that TIMELESS was involved in DNA damage repair after stress or injury, circadian rhythm regulation, and maintenance of telomere length, and changes in its expression may contribute to the occurrence and development of multiple diseasesCitation33–35. Moreover, silencing of TIMELESS, which has been defined as a key gene for maintaining genome stability, resulted in increased H2AX phosphorylation (γH2AX) levels, suggesting DNA damageCitation33,Citation36. This current study demonstrated that TIMELESS deletion aggravated Fn-Exo infection-induced DNA damage, which is consistent with the regulatory role of TIMELESS on DNA damage described in previous reportsCitation33,Citation36. We also found that Fn-Exo-accelerated barrier damage (both in vitro and in vivo) and colonic inflammation were miR-129-2-3p/TIMELESS axis-dependent, which had not been mentioned and investigated in previous studies.
Nowadays, it is gradually attracting attention that UC is closely related to agingCitation12,Citation37. According to Faye et alCitation37, IBD patients are at increased risk of aging-related diseases, and frailty resulting from physiologic decline is associated with adverse prognosis in IBD. However, the effects and regulatory mechanisms of Fn-Exo and miR-129-2-3p/TIMELESS axis on senescence in UC have yet to be elucidated. In this study, we predicted that TIMELESS may be involved in regulating cellular senescence based on KEGG pathway enrichment analysis. Consistent with previous findingsCitation38, our data indicated that silencing TIMELESS indeed promoted cellular senescence. Interestingly, the potentiation of siTIMELESS on cellular senescence was rescued by miR-129-2-3p-inhibitor, regardless of the presence or absence of Fn-Exo infection, as verified by our experiments both in vitro and in vivo. Accumulated evidence suggested that DNA damage response regulators ATM and ATR play a critical role in cellular senescenceCitation11,Citation39. It is well established that DNA damage activated ATM and ATR through phosphorylation, followed by phosphorylating CHEK2 and CHEK1, respectively, which may induce p53-dependent cellular senescenceCitation20,Citation40–42. This research raises an intriguing hypothesis that Fn-Exo, as an external stimulus, may accelerate cellular senescence by inducing DNA damage and subsequently activating the ATM/ATR/p53 signaling pathway. Herein, the key regulatory roles of Fn-Exo on cellular senescence, barrier damage, and phosphorylation activation of the ATM/ATR/p53 pathway have been confirmed in vitro and in vivo. Further experiments revealed that targeting the miR-129-2-3p/TIMELESS axis or inhibiting the ATM/ATR/p53 signaling pathway both significantly improved Fn-Exo-exacerbated cellular senescence and experimental colitis. In future studies, it is valuable to determine the specific origin of Exo-miR-129-2-3p in serum, as well as to construct miR-129-2-3p knockout mice to further clarify the critical role of miR-129-2-3p in Fn-Exo promoting UC. Furthermore, this study has some limitations. In addition to miR-129-2-3p, other miRNAs differentially expressed in FHC and Caco-2 cells may also be related to UC progression. And only male mice were utilized here.
In summary, we elucidated the biological role and mechanism of Fn-Exo in the progression of colitis for the first time. Our findings suggest that Fn-Exo activates the ATM/ATR/p53 signaling pathway via the miR-129-2-3p/TIMELESS axis, thereby promoting cellular senescence, barrier damage, and experimental colitis (). Targeting the miR-129-2-3p/TIMELESS axis or the ATM/ATR/p53 pathway may be valuable therapies for Fn-infected UC. In addition, serum-Exo-miR-129-2-3p and TIMELESS in humans may function as potential diagnostic biomarkers for UC and Fn-high-UC. Therefore, our study provides a solid foundation for improving our understanding of Fn-infectious UC pathogenesis and enhances diagnostic and molecular therapeutic approaches for UC.
Materials and methods
Cell culture
The human colonic epithelial cell lines FHC cells (CRL-1831) and Caco-2 cells (HTB-37) purchased from the American Type Culture Collection (ATCC, Rockville, USA). The human normal epithelial cell NCM460 cell line (BNCC339288) was purchased from the BeNa Culture Collection (BNCC, Beijing, China). Cell lines were cultured in Dulbecco’s modified Eagle medium (DMEM, Gibco, USA) supplemented with 10% fetal bovine serum (FBS, Gibco, USA) at standard condition (37°C, 5% COCitation2.
Bacterial culture
Fusobacterium nucleatum (Fn) obtained from the ATCC (ATCC 25,586, Rockville, USA) was incubated in fastidious anaerobic broth under anaerobic conditions at 37°C (10% H2, 5% CO2, and 85% N2), as described previouslyCitation4.
Human studies
Serum samples and colon tissue specimens from clinically and histologically confirmed therapy-naïve UC patients and sex- and age-matched healthy controls were obtained from Renmin Hospital of Wuhan University. Clinicopathological features of patients were acquired from hospital records. Informed consent was obtained from all participants. The project was approved by the Institutional Review Committee of the Renmin Hospital of Wuhan University (Approval number: 2018K-C089).
Exosomes isolation and characterization
FHC cells were infected with Fn suspension at a concentration of 1 × 108 colony-forming units (CFU)/mL for 48 hours at a multiplicity of infection of 10:1 (bacteria: cells) as in the previous studyCitation9. Then, the cell culture medium was replaced with a DMEM medium containing 10% exosome-depleted FBS. After 48 hours, culture supernatants of Fn-infected FHC cells and equal volumes of PBS-treated FHC cells were collected separately and then passed through 0.22 μm filters. Fn-Exo and Con-Exo were isolated and purified by repeated ultracentrifugation of the filtered supernatant twice at 120,000 g for 70 minutes at 4°C (Thermo Fisher Scientific, USA). Serum exosomes from humans (UC patients and healthy controls) and mice were extracted using the exoEasy Maxi Kit (Cat #76064, Qiagen, Germany) as we previously describedCitation8, following the manufacturer’s instructions. The size and concentration of exosomes were evaluated using the NanoSight NS300 system (Malvern Panalytical, United Kingdom). A transmission electron microscope (HT7800, Hitachi, Tokyo, Japan) was used to observe the morphology and size of exosomesCitation3,Citation8.
Fluorescence labeling and imaging of exosomes
We labeled purified exosomes with lipophilic fluorescent dye Dil (10 μM) to visualizeCitation3,Citation24. 10 μg of Dil-labeled exosomes were co-cultured with 1 × 105 Caco-2 cells, and images were captured using a laser scanning microscope (Leica TCS SP8, Leica Microsystems, Germany) after 0, 6, 12, and 24 hours of co-culture to determine the uptake of exosomes by the recipient cells.
Western blot analysis
Total protein extraction was performed using radioimmunoprecipitation assay (RIPA) buffer (Sigma-Aldrich, USA) and detection of protein concentration was performed with a bicinchoninic acid (BCA) Protein Assay Kit (Cat #23225, Thermo Fisher Scientific, USA). A mixture of loading buffer and cell lysate supernatant is loaded into the electrophoresis wells of sodium dodecyl sulfate-polyacrylamide gel electrophoresis (SDS-PAGE) gels and then transferred onto polyvinylidene fluoride membranes (Bio-Rad, Hercules, CA, USA). Primary antibodies were as follows: CD63 (Abcam ab217345; 1:1000); CD9 (Abcam ab236630; 1:1000); ZO-1 (Abcam ab216880; 1:1000); Occludin (CST 91,131; 1:1000); TIMELESS (Proteintech 14,421–1-AP; 1:1000); p-p53 (CST 9284; 1:1000); p53 (CST 2524; 1:1000); p21 (Abcam ab109199; 1:1000); p16 (Abcam ab108349; 1:1000); γH2AX (CST 9718; 1:1000); H2AX (CST 7631; 1:1000); p-ATM (CST 4526; 1:1000); ATM (CST 2873; 1:1000); p-CHEK2 (CST 2661; 1:1000); CHEK2 (CST 2662; 1:1000); p-ATR (CST 2853; 1:1000); ATR (CST 2790; 1:1000); p-CHEK1 (CST 2348; 1:1000); CHEK1 (CST 2360; 1:1000); β-Tubulin (Proteintech 10,094–1-AP; 1:5000); GAPDH (Proteintech 60,004–1-Ig; 1:50000). A ChemiDoc XRS System (Bio-Rad) was used for blot detection.
Transepithelial electrical resistance (TEER) measurement
Caco-2 monolayer cells were inoculated in transwell culture plate chambers (0.4 μm pore size, Corning Inc., USA) to monitor monolayer cell transepithelial resistance and assess the tightness of Caco-2 monolayer cell junctionsCitation43. The two electrodes of the Millicell-ERS system (Millipore, USA) were inserted into the top and bottom side of the transwell, respectively, and the whole procedure was performed at a constant temperature of 37°C. Three points in different directions were randomly selected for each transwell chamber and the detection was repeated for three times. The TEER value was expressed as Ω·cmCitation2.
Permeability assays in vitro and in vivo
Fluorescein isothiocyanate (FITC) – dextran 4 kDa (FD4, Sigma-Aldrich, USA) was added to transwell chambers seeded with Caco-2 monolayer cells and incubated for 2 hours. Fluorescein intensity in the lower chamber medium was then detected to analyze the permeability of the Caco-2 cellsCitation3. As previously described, we administered FD4 (Sigma-Aldrich, USA) to mice (n = 8 per group) at 400 μg/g body weight by gavageCitation44. Subsequently, the fluorescence of FITC in plasma samples was measured using a fluorescence enzyme labeling instrument (Thermo Fisher Scientific, USA), assessing the intestinal permeability in mice.
Immunofluorescence staining
For cell immunofluorescence staining, cells seeded on slides were washed 3 times with cold PBS and then fixed with 4% paraformaldehyde for 20 min at room temperature. Next, cells were permeabilized with 0.5% Triton X-100 and blocked with 3% goat serum for 1 hourCitation45, followed by incubation with primary antibody (ZO-1: Abcam ab221547; γH2AX: CST 9718) overnight at 4°C. The following primary antibodies were used for immunofluorescence staining of mouse colon cross-sections: p-ATM (Sigma-Aldrich 05–740-25UG); p-ATR (ABclonal AP0676); p-p53 (Proteintech 28,961–1-AP); p53 (CST 2524); p21 (Proteintech 27,296–1-AP). Nuclei were stained using DAPI (Beyotime, China). Immunofluorescence images were captured using a fluorescence microscope (Olympus FV1200, Japan) or a Leica TCS SP8 confocal laser scanning microscope (Leica Microsystems, Germany).
Mice
C57BL/6J wild-type mice (6–8-week-old, males) were purchased from Nanjing Biomedical Research Institute of Nanjing University (NBRI)Citation4. Mice were housed under standard specific pathogen-free barrier conditions with free access to food and waterCitation3,Citation4. All animal handling protocols in this study were approved by the Institutional Animal Care and Use Committee of Renmin Hospital of Wuhan University, China (Approval number: 20181001).
Induction and evaluation of experimental colitis
C57BL/6J male mice (6–8-week-old) were randomly divided into different treatment groups, with 8 mice in each group. Induction of experimental colitis in mice using sterile drinking water supplemented with 3% (w/v) DSS (MP Biomedicals, USA)Citation3. As previously describedCitation26, exosomes (Con-Exo, Fn-Exo, Exo-NC, Exo-miR-129-2-3p-mimics, Exo-inhibitor-NC, and Exo-miR-129-2-3p-inhibitor) were tail vein injected at 50 μg per mouse every 2 days starting 1 day before the mice received DSS treatment. Mice were observed and recorded daily for body weight, stool consistency, and severity of bloody stool to determine the DAI. After 7 days of treatment with DSS, mice were anesthetized and euthanized, and the colon was quickly and carefully separated for subsequent studies. 3–5 independent fields were randomly selected from each colon cross-section and the histological scores were calculated based on previously described scoring criteriaCitation4,Citation26.
Immunohistochemistry analysis
Tissue specimens were fixed with 4% paraformaldehyde (Biosharp, China), paraffin-embedded, and cut into 5-μm-thick sections. Then, an UltraSensitive SP Immunohistochemistry (IHC) Kit (Maxim Biotech, Fuzhou, China) was used following the same steps as we did previouslyCitation3,Citation4. Sections were incubated with primary antibodies against ZO-1 (Abcam ab221547) and TIMELESS (Proteintech 14,421–1-AP) overnight at 4°C. Finally, an Olympus FV1200 fluorescence microscope (Olympus, Japan) was used to acquire images for analysis.
Exosomal-miRNA-seq assay
Exosomes derived from Fn-infected FHC cells (Fn-Exo) and an equal volume of PBS-treated FHC cells (Con-Exo) were used for Exosomal-miRNA sequencing and library construction (BGI, Wuhan, China). We identified differentially expressed miRNAs in exosomes using DEGseq2Citation46,Citation47. Subsequently, data analysis and graphing were performed using the Dr. Tom Multi-Omics Data Mining System (https://biosys.bgi.com).
RNA isolation and quantitative real-time PCR (qRT-PCR) analysis
Total RNAs in cells, tissues, and exosomes were extracted using TRIzol reagent (Invitrogen). Next, miRNA and mRNA (500 ng) were reverse transcribed into cDNA using the PrimeScript RT Reagent Kit (Cat #RR037A, TaKaRa, Japan) and the PrimeScript Kit with gDNA Eraser (Cat #RR047A, TaKaRa, Japan), respectively, according to the manufacturer’s instructions. Analysis of miRNA and mRNA expression levels was performed using a TB Green Premix Ex Taq (Cat #RR820A, TaKaRa, Japan) on the BIO-RAD 7500 qRT-PCR Detection System. The usage of internal and external controls was consistent with the previous useCitation8,Citation28. The 2−ΔCT method was employed to determine miRNA and mRNA expression fold changes. Primers used for qRT-PCR in this study were listed in Table S4 (Sangon Biotech, China).
Cell transfection
Negative control RNA (NC), miR-129-2-3p-mimics, miR-129-2-3p-inhibitor/scrambled negative control RNA (inhibitor-NC), and siRNAs targeting the human TIMELESS gene (siTIMELESS) were purchased from GenePharma (Shanghai, China), the sequences of which were documented in Table S5. Cells were transfected in 6-well plates using Lipofectamine 3000 reagent (Invitrogen, USA) and Opti-MEM (Gibco, USA) according to the supplier’s instructions.
AAV9-mmiR-129-2-3p-sponge and AAV9-shRNA (TIMELESS) infection
The vector profiles of AAV9-NC-CAG-EGFP, AAV9-mmiR-129-2-3p-sponge-CAG-EGFP, and AAV9-shRNA (TIMELESS)-CAG-EGFP constructed and synthesized by Genechem Inc (Shanghai, China) are shown in Figure S8. As previously described, we achieved silencing of miR-129-2-3p and TIMELESS expression in the mouse colon by infecting mice with AAV9-mmiR-129-2-3p-sponge-CAG-EGFP and AAV9-shRNA (TIMELESS)-CAG-EGFP by enema 1 week before treatment with DSS respectively (1 × 10Citation11 AAV9 particles in 200 μL PBS/mouse)Citation3,Citation48. AAV9-NC-CAG-EGFP of equal volume and concentration was used as a control treatment.
RNA-seq assay
Total RNA was extracted using Trizol (Invitrogen, USA) from FHC cells transfected with NC or miR-129-2-3p-mimics, followed by mRNA enrichment and purification using oligo (dT)-attached magnetic beads. The purified and quality-checked double-stranded PCR products were heated denatured and then circularized using a splint oligo sequence to obtain the final library. The RNA sequencing was conducted using the MGISEQ-2000RS platform. Clustering, sequencing, and data analysis were done by BGI (Shenzhen, China).
Fluorescence in situ hybridization (FISH)
Microbial FISH was performed following the manufacturer’s instructions to assess the abundance of Fn in human colon tissue (Focobio, China). The sequence of the Fn targeting probe (FUS664; FITC-labeled) was 5’-CTT GTA GTT CCG C(C/T) TAC CTC-3’Citation4. Slides were photographed using the 400× lens on an Olympus FV1200 fluorescence microscope (Olympus, Japan). The average number of FUS664 probes per field of view was observed and calculated by three investigators who were blinded to the experimental protocol to evaluate Fn abundance. We defined Fn-negative, Fn-low, and Fn-high as samples with an average number of < 5, 5–20, and > 20 FUS664 probes visualized per field on average, respectivelyCitation4.
Luciferase reporter assay
The pmirGLO dual-luciferase miRNA targeting expression vector cloned with the binding sites between miR-129-2-3p and TIMELESS was constructed by GenePharma (Shanghai, China). FHC and Caco-2 cells were seeded in 24-well plates, and then co-transfected with TIMELESS wild-type (WT) or mutant plasmids and miR-129-2-3p-mimics/NC or miR-129-2-3p-inhibitor/inhibitor-NC using Lipofectamine 3000 (Invitrogen, USA) and Opti-MEM (Gibco, USA). Luciferase activities were detected 48 hours after co-transfection using a dual-luciferase reporter assay system (Promega, USA) and normalized against Renilla luciferase activity.
Senescence β-Galactosidase (SA-β-Gal) staining
We assessed β-galactosidase activity in 4% paraformaldehyde-fixed cells and freshly frozen colon cross-sections of mice using the Senescence β-Galactosidase Staining Kit (Cat #9860, CST), based on the previous descriptionsCitation11. An Olympus I×73microscope (Olympus, Japan) and an Olympus FV1200 microscope (Olympus, Japan) were utilized to capture SA-β-Gal positive cells and tissues, respectively. Next, SA-β-gal positive cells were quantified using ImageJ software.
Comet assay
Comet assay (single cell gel electrophoresis) was performed with the Trevigen Comet Assay Kit (Trevigen, USA) according to the manufacturer’s instructions, as described previouslyCitation49. Electrophoresis was carried out at 21 V for 30 minutes using the Comet Analytical Electrophoresis System II (Trevigen, USA). Slides were observed under an Olympus FV1200 upright microscope (Olympus, Japan) after staining with 50 μL SYBR Gold nucleic acid gel stain (Cat #S11494, Thermo Fisher Scientific, USA) for 30 minutes.
Statistical analysis
All data were presented as the mean ± SEM of at least 3 independent experiments. Statistical analyses were conducted using Student’s t-test or Chi-square test between 2 groups, while ANOVA was used between 3 or more groups. The sensitivity and specificity of diagnosis were evaluated by plotting the receiver-operating characteristic (ROC) curves using the Youden index (J)Citation8,Citation50. GraphPad Prism 9.0 (GraphPad Software, USA) and SPSS 22.0 (IBM, USA) were used to perform statistical analyses. p < 0.05 indicates statistical significance.
Author’s contributions
Conceptualization, W.D. and S.W.; Methodology, S.W., X.W., and M.C.; Formal Analysis, X.W., M.C., Z.X., and X. L.; Investigation, S.W., X.W., M.C., Z.X., and X. L.; Resources, J. Z., and W.D.; Writing – Original Draft, S.W.; Writing – Review and Editing, W.D.; Visualization, S.W., X.W., and M.C.; Supervision, J. Z., and W.D.; Funding Acquisition, W.D. All authors reviewed and commended on the manuscript.
Consent for publication
All authors have approved the content for publication in the journal.
Ethical approval and consent to participate
The project was approved by the Institutional Review Committee of the Renmin Hospital of Wuhan University (Approval number: 2018K-C089). All animal handling protocols in this study were approved by the Institutional Animal Care and Use Committee of Renmin Hospital of Wuhan University, China (Approval number: 20181001).
Supplemental Material
Download MS Word (1.2 MB)Acknowledgments
Not applicable.
Disclosure statement
No potential conflict of interest was reported by the author(s).
Data availability statement
All data generated or analyzed during this study are included in this published article and its supplementary information files. All sequencing data associated with this study have been uploaded to the NCBI (Sequence Read Archive) SRA database under the accession number PRJNA1000304 and PRJNA1000534.
Supplementary material
Supplemental data for this article can be accessed online at https://doi.org/10.1080/19490976.2023.2240035
Additional information
Funding
References
- Krugliak CN, Torres J, Rubin DT. What does disease progression look like in ulcerative colitis, and how might it be prevented? Gastroenterology. 2022;162(5):1396–25. doi:10.1053/j.gastro.2022.01.023.
- Kobayashi T, Siegmund B, Le Berre C, Wei SC, Ferrante M, Shen B, Bernstein CN, Danese S, Peyrin-Biroulet L, Hibi T. Ulcerative colitis. Nat Rev Dis Primers. 2020;6(1):74. doi:10.1038/s41572-020-0205-x.
- Wei S, Zhang J, Wu X, Chen M, Huang H, Zeng S, Xiang Z, Li X, Dong W. Fusobacterium nucleatum extracellular vesicles promote experimental colitis by modulating autophagy via the miR-574-5p/CARD3 axis. Inflamm Bowel Dis. 2022;29(1):9–26. doi:10.1093/ibd/izac177.
- Chen Y, Chen Y, Cao P, Su W, Zhan N, Dong W. Fusobacterium nucleatum facilitates ulcerative colitis through activating IL-17F signaling to NF-kappaB via the upregulation of CARD3 expression. J Pathol. 2020;250(2):170–182. doi:10.1002/path.5358.
- Liu L, Liang L, Yang C, Zhou Y, Chen Y. Extracellular vesicles of Fusobacterium nucleatum compromise intestinal barrier through targeting RIPK1-mediated cell death pathway. Gut Microbes. 2021;13(1):1–20. doi:10.1080/19490976.2021.1902718.
- Pegtel DM, Gould SJ. Exosomes. Annu Rev Biochem. 2019;88:487–514. doi:10.1146/annurev-biochem-013118-111902.
- Jeppesen DK, Fenix AM, Franklin JL, Higginbotham JN, Zhang Q, Zimmerman LJ, Liebler DC, Ping J, Liu Q, Evans R, et al. Reassessment of exosome composition. Cell. 2019;177(2):428–445.e18. doi:10.1016/j.cell.2019.02.029.
- Wei S, Peng L, Yang J, Sang H, Jin D, Li X, Chen M, Zhang W, Dang Y, Zhang G. Exosomal transfer of miR-15b-3p enhances tumorigenesis and malignant transformation through the DYNLT1/Caspase-3/Caspase-9 signaling pathway in gastric cancer. J Exp Clin Cancer Res. 2020;39(1):32. doi:10.1186/s13046-019-1511-6.
- Guo S, Chen J, Chen F, Zeng Q, Liu WL, Zhang G. Exosomes derived from Fusobacterium nucleatum-infected colorectal cancer cells facilitate tumour metastasis by selectively carrying miR-1246/92b-3p/27a-3p and CXCL16. Gut. 2020;70(8):1507–1519. doi:10.1136/gutjnl-2020-321187.
- Cao Y, Wang Z, Yan Y, Ji L, He J, Xuan B, Shen C, Ma Y, Jiang S, Ma D, et al. Enterotoxigenic bacteroidesfragilis promotes intestinal inflammation and malignancy by inhibiting exosome-packaged miR-149-3p. Gastroenterology. 2021;161(5):1552–1566. doi:10.1053/j.gastro.2021.08.003.
- Yan P, Li Z, Xiong J, Geng Z, Wei W, Zhang Y, Wu G, Zhuang T, Tian X, Liu Z, et al. LARP7 ameliorates cellular senescence and aging by allosterically enhancing SIRT1 deacetylase activity. Cell Rep. 2021;37(8):110038. doi:10.1016/j.celrep.2021.110038.
- Risques RA, Lai LA, Brentnall TA, Li L, Feng Z, Gallaher J, Mandelson MT, Potter JD, Bronner MP, Rabinovitch PS. Ulcerative colitis is a disease of accelerated colon aging: evidence from telomere attrition and DNA damage. Gastroenterology. 2008;135(2):410–418. doi:10.1053/j.gastro.2008.04.008.
- Martin O, Bergonzini A, Lopez CM, Paparouna E, Butter D, Theodorou S, Haykal MM, Boutet-Robinet E, Tebaldi T, Wakeham A, et al. Influence of the microenvironment on modulation of the host response by typhoid toxin. Cell Rep. 2021;35(1):108931. doi:10.1016/j.celrep.2021.108931.
- Peng L, Sang H, Wei S, Li Y, Jin D, Zhu X, Li X, Dang Y, Zhang G. circCUL2 regulates gastric cancer malignant transformation and cisplatin resistance by modulating autophagy activation via miR-142-3p/ROCK2. Mol Cancer. 2020;19(1):156. doi:10.1186/s12943-020-01270-x.
- Sang H, Zhang W, Peng L, Wei S, Zhu X, Huang K, Yang J, Chen M, Dang Y, Zhang G. Exosomal circRELL1 serves as a miR-637 sponge to modulate gastric cancer progression via regulating autophagy activation. Cell Death Disease. 2022;13(1):56. doi:10.1038/s41419-021-04364-6.
- Mosna K, Janega P, Sedlak J, Babal P. Complex changes of circadian proteins expression in inflammatory bowel disease. Bratisl Lek Listy. 2021;122(4):235–241. doi:10.4149/BLL_2021_038.
- D’Adda DFF. Living on a break: cellular senescence as a DNA-damage response. Nat Rev Cancer. 2008;8(7):512–522. doi:10.1038/nrc2440.
- Ahn SH, Cho SH, Song JE, Kim S, Oh SS, Jung S, Cho KA, Lee TH. Caveolin-1 serves as a negative effector in senescent human gingival fibroblasts during Fusobacterium nucleatum infection. Mol Oral Microbiol. 2017;32(3):236–249. doi:10.1111/omi.12167.
- Smith-Roe SL, Patel SS, Zhou Y, Simpson DA, Rao S, Ibrahim JG, Cordeiro-Stone M, Kaufmann WK. Separation of intra-S checkpoint protein contributions to DNA replication fork protection and genomic stability in normal human fibroblasts. Cell Cycle. 2013;12(2):332–345. doi:10.4161/cc.23177.
- Kondratov RV, Antoch MP. Circadian proteins in the regulation of cell cycle and genotoxic stress responses. Trends Cell Biol. 2007;17(7):311–317. doi:10.1016/j.tcb.2007.07.001.
- Li G, Lin J, Zhang C, Gao H, Lu H, Gao X, Zhu R, Li Z, Li M, Liu Z. Microbiota metabolite butyrate constrains neutrophil functions and ameliorates mucosal inflammation in inflammatory bowel disease. Gut Microbes. 2021;13(1):1968257. doi:10.1080/19490976.2021.1968257.
- Su W, Chen Y, Cao P, Chen Y, Guo Y, Wang S, Dong W. Fusobacterium nucleatum promotes the development of ulcerative colitis by inducing the autophagic cell death of intestinal epithelial. Front Cell Infect Microbiol. 2020;10:594806. doi:10.3389/fcimb.2020.594806.
- Engevik MA, Danhof HA, Ruan W, Engevik AC, Chang-Graham AL, Engevik KA, Shi Z, Zhao Y, Brand CK, Krystofiak ES, et al. Fusobacterium nucleatum secretes outer membrane vesicles and promotes intestinal inflammation. Mbio. 2021;12(2). doi:10.1128/mBio.02706-20
- Yang C, Zhang M, Sung J, Wang L, Jung Y, Merlin D. Autologous exosome transfer: A new personalised treatment concept to prevent colitis in a murine model. J Crohns Colitis. 2020;14(6):841–855. doi:10.1093/ecco-jcc/jjz184.
- Liu C, Yan X, Zhang Y, Yang M, Ma Y, Zhang Y, Xu Q, Tu K, Zhang M. Oral administration of turmeric-derived exosome-like nanovesicles with anti-inflammatory and pro-resolving bioactions for murine colitis therapy. J Nanobiotechnology. 2022;20(1):206. doi:10.1186/s12951-022-01421-w.
- Deng F, Yan J, Lu J, Luo M, Xia P, Liu S, Wang X, Zhi F, Liu D. M2 macrophage-derived exosomal miR-590-3p attenuates DSS-Induced mucosal damage and promotes epithelial repair via the LATS1/YAP/beta-catenin signalling axis. J Crohns Colitis. 2021;15(4):665–677. doi:10.1093/ecco-jcc/jjaa214.
- Tian Y, Xu J, Li Y, Zhao R, Du S, Lv C, Wu W, Liu R, Sheng X, Song Y, et al. MicroRNA-31 reduces inflammatory signaling and promotes regeneration in colon epithelium, and delivery of mimics in microspheres reduces colitis in mice. Gastroenterology. 2019;156(8):2281–2296. doi:10.1053/j.gastro.2019.02.023.
- Sun Y, Zhou Y, Shi Y, Zhang Y, Liu K, Liang R, Sun P, Chang X, Tang W, Zhang Y, et al. Expression of miRNA-29 in pancreatic beta cells promotes inflammation and diabetes via TRAF3. Cell Rep. 2021;34(1):108576. doi:10.1016/j.celrep.2020.108576.
- Umehara T, Mori R, Mace KA, Murase T, Abe Y, Yamamoto T, Ikematsu K. Identification of specific miRNAs in neutrophils of type 2 diabetic mice: Overexpression of miRNA-129-2-3p accelerates diabetic wound healing. Diabetes. 2019;68(3):617–630. doi:10.2337/db18-0313.
- Zhu M, Liang Q, Chen T, Kong Q, Ye G, Yu S, Li X, He Q, Liu H, Hu Y, et al. Identification and validation of methylated differentially expressed miRnas and immune infiltrate profile in EBV-associated gastric cancer. Clin Epigenetics. 2021;13(1):22. doi:10.1186/s13148-020-00989-0.
- Yu X, Luo L, Wu Y, Yu X, Liu Y, Yu X, Zhao X, Zhang X, Cui L, Ye G, et al. Gastric juice miR-129 as a potential biomarker for screening gastric cancer. Med Oncol. 2013;30(1):365. doi:10.1007/s12032-012-0365-y.
- Liu X, Zhang G, Yu T, He J, Liu J, Chai X, Zhao G, Yin D, Zhang C. Exosomes deliver lncRNA DARS-AS1 siRNA to inhibit chronic unpredictable mild stress-induced TNBC metastasis. Cancer Lett. 2022;543:215781. doi:10.1016/j.canlet.2022.215781.
- Young LM, Marzio A, Perez-Duran P, Reid DA, Meredith DN, Roberti D, Star A, Rothenberg E, Ueberheide B, Pagano M. TIMELESS forms a complex with PARP1 distinct from its complex with TIPIN and plays a role in the DNA damage response. Cell Rep. 2015;13(3):451–459. doi:10.1016/j.celrep.2015.09.017.
- Barnes JW, Tischkau SA, Barnes JA, Mitchell JW, Burgoon PW, Hickok JR, Gillette MU. Requirement of mammalian Timeless for circadian rhythmicity. Science. 2003;302(5644):439–442. doi:10.1126/science.1086593.
- Leman AR, Dheekollu J, Deng Z, Lee SW, Das MM, Lieberman PM, Noguchi E. Timeless preserves telomere length by promoting efficient DNA replication through human telomeres. Cell Cycle. 2012;11(12):2337–2347. doi:10.4161/cc.20810.
- Paulsen RD, Soni DV, Wollman R, Hahn AT, Yee MC, Guan A, Hesley JA, Miller SC, Cromwell EF, Solow-Cordero DE, et al. A genome-wide siRNA screen reveals diverse cellular processes and pathways that mediate genome stability. Mol Cell. 2009;35(2):228–239. doi:10.1016/j.molcel.2009.06.021.
- Faye AS, Colombel JF. Aging and IBD: A new challenge for clinicians and researchers. Inflamm Bowel Dis. 2022;28(1):126–132. doi:10.1093/ibd/izab039.
- Shen X, Li M, Mao Z, Yu W. Loss of circadian protein TIMELESS accelerates the progression of cellular senescence. Biochem Biophys Res Commun. 2018;503(4):2784–2791. doi:10.1016/j.bbrc.2018.08.040.
- Kang C, Xu Q, Martin TD, Li MZ, Demaria M, Aron L, Lu T, Yankner BA, Campisi J, Elledge SJ. The DNA damage response induces inflammation and senescence by inhibiting autophagy of GATA4. Science. 2015;349(6255):a5612. doi:10.1126/science.aaa5612.
- Yan S, Sorrell M, Berman Z. Functional interplay between ATM/ATR-mediated DNA damage response and DNA repair pathways in oxidative stress. Cell Mol Life Sci. 2014;71(20):3951–3967. doi:10.1007/s00018-014-1666-4.
- Barnes RP, de Rosa M, Thosar SA, Detwiler AC, Roginskaya V, Van Houten B, Bruchez MP, Stewart-Ornstein J, Opresko PL. Telomeric 8-oxo-guanine drives rapid premature senescence in the absence of telomere shortening. Nature Structural & Molecular Biology. 2022;29(7):639–652. doi:10.1038/s41594-022-00790-y.
- Chen TY, Huang BM, Tang TK, Chao YY, Xiao XY, Lee PR, Yang LY, Wang CY. Genotoxic stress-activated DNA-PK-p53 cascade and autophagy cooperatively induce ciliogenesis to maintain the DNA damage response. Cell Death Differ. 2021;28(6):1865–1879. doi:10.1038/s41418-020-00713-8.
- Lee J, Mo JH, Katakura K, Alkalay I, Rucker AN, Liu YT, Lee HK, Shen C, Cojocaru G, Shenouda S, et al. Maintenance of colonic homeostasis by distinctive apical TLR9 signalling in intestinal epithelial cells. Nat Cell Biol. 2006;8(12):1327–1336. doi:10.1038/ncb1500.
- Dawson PA, Huxley S, Gardiner B, Tran T, McAuley JL, Grimmond S, McGuckin MA, Markovich D. Reduced mucin sulfonation and impaired intestinal barrier function in the hyposulfataemic NaS1 null mouse. Gut. 2009;58(7):910–919. doi:10.1136/gut.2007.147595.
- Gross KM, Zhou W, Breindel JL, Ouyang J, Jin DX, Sokol ES, Gupta PB, Huber K, Zou L, Kuperwasser C. Loss of slug compromises DNA damage repair and accelerates stem cell aging in mammary epithelium. Cell Rep. 2019;28(2):394–407. doi:10.1016/j.celrep.2019.06.043.
- Love MI, Huber W, Anders S. Moderated estimation of fold change and dispersion for RNA-seq data with DESeq2. Genome Biol. 2014;15(12):550. doi:10.1186/s13059-014-0550-8.
- Qian Y, Arellano G, Ifergan I, Lin J, Snowden C, Kim T, Thomas JJ, Law C, Guan T, Balabanov RD, et al. ZEB1 promotes pathogenic Th1 and Th17 cell differentiation in multiple sclerosis. Cell Rep. 2021;36(8):109602. doi:10.1016/j.celrep.2021.109602.
- Liu X, Liu Y, Liu Z, Lin C, Meng F, Xu L, Zhang X, Zhang C, Zhang P, Gong S, et al. CircMYH9 drives colorectal cancer growth by regulating serine metabolism and redox homeostasis in a p53-dependent manner. Mol Cancer. 2021;20(1):114. doi:10.1186/s12943-021-01412-9.
- Guo P, Tian Z, Kong X, Yang L, Shan X, Dong B, Ding X, Jing X, Jiang C, Jiang N, et al. FadA promotes DNA damage and progression of Fusobacterium nucleatum-induced colorectal cancer through up-regulation of chk2. J Exp Clin Cancer Res. 2020;39(1):202. doi:10.1186/s13046-020-01677-w.
- Youden WJ. Index for rating diagnostic tests. Cancer-Am Cancer Soc. 1950;3(1):32–35. doi:10.1002/1097-0142(1950)3:1<32:aid-cncr2820030106>3.0.co;2-3.