ABSTRACT
Vibrio cholerae utilizes the Type VI secretion system (T6SS) to gain an advantage in interbacterial competition by delivering anti-prokaryotic effectors in a contact-dependent manner. However, the impact of T6SS and its secreted effectors on physiological behavior remains poorly understood. In this study, we present Tle1Vc, a phospholipase effector in atypical pathogenic V. cholerae E1 that is secreted by T6SS via its interaction with VgrG1E1. Tle1Vc contains a DUF2235 domain and belongs to the Tle1 (type VI lipase effector) family. Bacterial toxicity assays, lipase activity assays and site-directed mutagenesis revealed that Tle1Vc possessed phospholipase A1 activity and phospholipase A2 activity, and that Tle1Vc-induced toxicity required a serine residue (S356) and two aspartic acid residues (D417 and D496). Cells intoxication with Tle1Vc lead to membrane depolarization and alter membrane permeability. Tli1tox-, a cognate immunity protein, directly interacts with Tle1Vc to neutralize its toxicity. Moreover, Tle1Vc can kill multiple microorganisms by T6SS and promote in vivo fitness of V. cholerae through mediating antibacterial activity. Tle1Vc induces bacterial motility by increasing the expression of flagellar-related genes independently of functional T6SS and the tit-for-tat (TFT) response, where Pseudomonas aeruginosa uses its T6SS-H1 cluster to counterattack other offensive attackers. Our study also demonstrated that the physical puncture of E1 T6SS can induce a moderate TFT response, which is essential to the Tle1Vc-mediated strong TFT response, maximizing effector functions. Overall, our study characterized the antibacterial mechanism of phospholipase effector Tle1Vc and its multiple physiological significance.
Introduction
Vibrio cholerae, a gram-negative bacterium, is the causative pathogen of cholera, a severe infectious disease.Citation1 They can be divided into toxigenic and non-toxigenic strains based on whether they carry the cholera toxin ctxAB genes.Citation2,Citation3 The main mode of cholera transmission is through the consumption of water and food contaminated with toxigenic V. cholerae, with the typical symptom being watery diarrhea that can lead to death in severe cases. Secretion systems, such as the type III secretion system and the type VI secretion system (T6SS),Citation4,Citation5 are widely used by bacteria to overcome host and competitor challenges. T6SS is a bacterial nanoweapon that can kill or inhibit adjacent cells by injecting toxic effectors through the telescopic structure of a needle tubeCitation6. T6SS enables microorganisms to obtain a competitive advantage over competitors in the same ecological niche, enhancing their environmental adaptability and pathogenicity to the host.Citation7
T6SS was first discovered in Pseudomonas aeruginosa and V. cholerae in 2006Citation8,Citation9 and is present in approximately 25% of all sequenced gram-negative bacteria.Citation10 The T6SS nanomachine can be divided into three main parts: the membrane complex, baseplate, and contractile sheath, which perform injection functions.Citation6 These structures are conserved among different species.Citation11,Citation12 The tubular structure can be divided into an Hcp inner tube and outer tube composed of VipA/B.Citation13 The inner Hcp tube can be secreted by the contraction of VipA/B.Citation14,Citation15 Fast injection of the Hcp inner tube delivers the tip structure, including VgrG, PAAR protein, and accompanying effectors, into the target cell.Citation16,Citation17 The killing effect of the T6SS is mainly attributed to the effectors loaded on the tip structure. Although effectors are extremely diverse, catalytic activity can be generally classified into lipase, peptidoglycan-degrading enzymes, proteases, nucleases, and pore-forming effectors, etc., which can exert their corresponding cytotoxic effect after being injected into neighboring cells.Citation18 These effectors are secreted by T6SS in diverse ways, including covalently or non-covalently binding to VgrG, Hcp, and PAAR to achieve transportation. Occasionally, these interactions are aided by specific adaptors or chaperones.
Type VI lipase effectors (Tle) are highly abundant and can be found in a broad spectrum of bacterial species.Citation17,Citation19–21 Tle can be divided into five divergent families (Tle1–5).Citation19 Tle1 and Tle2 are enriched in aquatic metagenomes, especially in Vibrio species, and have been shown to be important for interbacterial competition in the complex microbial community.Citation20 In addition to their antibacterial activity, lipase effectors also regulate multiple physiological roles. For example, Tle1AH and Tle2Vc have been shown to affect biofilm formation and trigger tit-for-tat (TFT) responses in which Pseudomonas aeruginosa uses its T6SS encoded by T6SS-H1 cluster to counterattack other offensive attackers, respectively.Citation21,Citation22
T6SS effector proteins belonging to the Tle1 family have been extensively studied in Gram-negative bacteria.Citation19 Previous investigations have primarily focused on the antibacterial activity of Tle1, along with exploring other biological functions such as their impact on biofilm formation and colonization in host.Citation19,Citation21,Citation23,Citation24 It is worth noting that the enzymatic activity and antibacterial mechanisms of Tle1 family proteins encoded by different bacteria can vary significantly. For instance, Tle1BT of Burkholderia thailandensis BTH_I2698 and Tle1PA of Pseudomonas aeruginosa have been shown to possess phospholipase A2 (PLA2) activity,Citation19,Citation25 while Tle1EAEC of entero-aggregative Escherichia coli exhibits both phospholipase A1 (PLA1) activity and a lower level of PLA2 activity.Citation24 However, limited information is available regarding the molecular mechanism underlying Tle1Vc-mediated killing activity in non-toxigenic V. cholerae strains, as well as its biological roles in virulence and in vivo fitness within host organisms. In this study, we report a lipase effector Tle1Vc in an atypical pathogenic O1 serogroup V. cholerae E1 lacking the typical virulence factors (cholera toxin and toxin co-regulated pilus), but encoding a type III secretion system responsible for intestinal colonization of non-O1, non-O139 V. cholerae.Citation26,Citation27 Tle1Vc is a phospholipase effector with phospholipase A1 activity and A2 activity secreted by T6SS and is predicted to contain two conserved motifs in addition to the known GXSXG motif, which are essential for its catalytic toxicity. We also found that an immunity protein, Tli1tox- can specifically abolish Tle1Vc-mediated toxicity by direct interaction. It is possible that Tle1Vc is secreted through noncovalent binding to the structural protein VgrG1E1. T6SS-mediated delivery of Tle1Vc demonstrated broad-spectrum antibacterial activity, providing V. cholerae E1 with a competitive advantage against multiple microorganisms. Colonization analysis revealed that V. cholerae E1 can colonize the adult mouse cecum and utilize its T6SS to enhance in vivo fitness. Moreover, our study found that Tle1Vc regulates bacterial motility and elicits a strong tit-for-tat response from P. aeruginosa PAO1. Notably, physical puncturing of the T6SS can induce a moderate TFT response, which is critical to the Tle1Vc-induced robust TFT response. Overall, our investigation has unveiled the vital physiological roles played by the T6SS of V. cholerae E1 and its substrate, Tle1Vc, which is a member of the phospholipase Tle1 family.
Materials and methods
Bacterial strains and growth conditions
All the strains and plasmids used in this study are listed in Table S1. Strains were grown at 37°C in Luria – Bertani medium or with 1.2% agar. The final concentrations of antibiotics in the growth media were 10 μg/mL chloramphenicol (Cm) for E. coli, 2 μg/mL Cm for V. cholerae, 100 μg/mL ampicillin (Amp), 50 μg/mL kanamycin (Km), and 10 μg/mL nalidixic acid (Nal).
Plasmid construction
Primers used in this study are listed in Table S2. Gene deletion and chromosomal mutation of V. cholerae were performed using the suicide plasmid pWM91 or pDS132 containing a sacB counter-selectable mark.Citation28,Citation29 Plasmid pK18 was used for gene deletion in Pseudomonas aeruginosa PAO1.Citation30
The tle1Vc (Gene accession number: OQ980252) and vgrG1E1 genes were directly cloned into the pBAD24 plasmid for complementation (cytoplasmic expression).Citation31 The effector and its variants were also fused with an N-terminal periplasmic Tat (twin-arginine translocation pathway) signal (periplasmic expression) into the pBAD24 plasmid for V. cholerae and E. coli or the pPSV37 plasmidCitation32 for P. aeruginosa PAO1. Immunity proteins were cloned into the pSRKKm vector for the complementation assays.Citation33 When required, these genes were introduced into the pETM3C plasmid with a 6× His purification tag for protein purification.
Bacterial competition assays
Overnight cultures of bacterial predators and prey cells were diluted to an OD600 of 1.0 and mixed in a 1:1 (predator: prey) ratio. Mixtures (5 μL) were spotted onto 0.22 μm polyvinylidene fluoride (PVDF) membranes overlaid on an LB agar plate and incubated at 37°C. After 4 h, cells were resuspended in 1 mL of 0.8% NaCl, and 5 μL of serially diluted cells was spotted onto selective LB agar plates to determine the number of surviving prey.
Protein secretion and Western blots analysis
Overnight bacterial cultures were subcultured in fresh LB and grown to OD600 0.9 at 37°C. The culture was centrifuged, and the supernatant was filtered through a 0.22 μm PVDF filter. For each 1.8 mL of culture supernatant, 200 μL of 100% trichloroacetic acid was added, and the mixture was incubated on ice for 30 min before centrifugation at 20,000 g for 15 min. The resulting precipitate was then washed twice with ice-cold acetone. Protein samples were separated by 12% SDS-PAGE gel and transferred onto PVDF membrane, which was then blocked with 5% nonfat milk. The proteins were probed with anti-rabbit Tle1Vc, anti-rabbit Hcp, and anti-mouse RpoB (Biolegend) primary antibodies overnight at 4°C, and further incubated with fluorescent secondary antibodies (LI-COR, Odyssey). The signal was screened using a two-color infrared laser imager. Polyclonal antibodies against Hcp and Tle1Vc were purchased from Shanghai Youlong Biotech.
Bacterial toxicity assays
E. coli BL21(DE3) cells carrying the effectors and their variants were cultured overnight in LB medium. The cultures were then adjusted to an optical density at 600 nm (OD600) of 1.0 and serially diluted in 0.8% NaCl at a 10-fold dilution. Subsequently, 5 μL of the resulting dilution was spotted onto LB agar plates containing either 0.2% arabinose or 0.2% glucose, followed by overnight incubation.
For the growth curve assays, bacterial cells expressing both the effector and immunity proteins were grown in LB medium overnight. The cultures were then inoculated at a 1% ratio into LB medium supplemented with 0.2% arabinose and 0.03 mM IPTG. The optical density was measured at the indicated time points.
Expression and purification of proteins
The effector gene tle1Vc, the immunity genes tli1tox- and tli1tox+ were cloned into a pETM3C plasmid with a 6× His-tag and transformed into E. coli BL21(DE3). Overnight cultures were inoculated into the LB medium and grown to an OD600 0.6. After adding IPTG to a final concentration of 0.1 mM, the culture was incubated at 16°C for 16 h. Cells were collected by centrifugation, resuspended in lysis buffer (50 mM tris HCl, 100 mM NaCl, 30 mM imidazole, 1 mM PMSF, pH 7.8) and finally lysed by ultrasonication. Recombinant proteins with a 6× His tag were trapped on a Ni2+-NTA column (Sangon Biotech). The column containing the target protein was washed twice in wash buffer (50 mM tris HCl, 100 mM NaCl, 30 mM imidazole, pH 7.8) and eluted with elution buffer (50 mM tris HCl, 100 mM NaCl, 300 mM imidazole, pH 7.8). The eluted proteins were further purified using the AKTA FPLC system.
Enzyme activity assays
The lipase activity of purified Tle1Vc and its inactivated mutants was detected using Tween 20 as degrading substrate.Citation34,Citation35 The enzyme activity using protein (50 ng) and Tween 20 as the substrate was assayed at 37°C in a 200 µL reaction buffer containing 25 mM Tris-HCl, pH 7.8), 1.8% (v/v) Tween 20, and 3 mM CaCl2. The optical density at 500 nm was measured over a 60-min period.
For phospholipase A activity assays were performed using PED-A1 (sn-1-labeled) and PED6 (sn-2-labeled) fluorescent substrates according to manufacturer’s directions (Invitrogen). Activity of Tle1Vc and its variants on these substrates was measured at an enzyme concentration of 3 nM at 37°C.
Analytical gel filtration chromatography coupled with static light scattering
Molar mass measurements were performed using an AKTA FPLC system (GE Healthcare) coupled with a static light scattering detector (mini-DAWN, Wyatt) and a differential refractive index detector (Optilab, Wyatt). Protein samples (50 μM) were loaded onto a Superdex 200 Increase 10/300 GL column (GE Healthcare) pre-equilibrated with a buffer (50 mM Tris-HCl (pH 7.5), 100 mM NaCl, 1 mM EDTA, and 1 mM DTT). The effector and immunity proteins were mixed at a 1:1 ratio.
Isothermal titration calorimetry (ITC) assay
ITC experiments were performed using a MicroCal ITC200 calorimeter (Malvern, UK) at 25°C. Proteins at high concentrations (100 μM) were loaded into the syringe and titrated into cells containing the corresponding interactors at low concentrations (10 μM). The sample in the syringe was subsequently injected into the cell with a time interval of 120 s (0.5 μL for the first injection and 2 μL each for the following 18 injections). Titration data were analyzed using Origin 7.0 software and fitted with the one-site binding model.
Surface plasmon resonance (SPR) experiments
Surface plasmon resonance (SPR) experiments were conducted to measure the binding kinetic parameters between proteins. The surface of a CM5 sensor chip (GE Healthcare) was activated using NHS and EDC (1:1, v/v), followed by immobilization of the protein through standard amine-coupling at 25°C. The ligand was diluted to a concentration of 10 μg/μL in sodium acetate buffer (pH 4.0), and the protein immobilization level was set to approximately 1000 RU (response unit; 1 RU is roughly equivalent to a 1 pg/mm2 change in the concentration of the bound substance on the chip surface). Subsequently, ethanolamine was used to block any unreacted NHS ester groups. To assess the activity of the immobilized protein on the chip surface, a potential interactive protein was utilized, and regeneration was performed using glycine hydrochloride at pH 1.5.
Different concentrations of analytes were sequentially injected into the channel to evaluate the binding kinetic parameters. A reference channel was activated and blocked to account for nonspecific binding of analytes to the chip surface. To remove any remaining sample from the different analytes, the chip was washed twice with PBS. The on-rates and off-rates of the compounds were determined by fitting the data to a 1:1 Langmuir binding model. Kinetic and affinity measurements were calculated based on the data after subtracting the reference channel signal using the Biacore T200 Evaluation Software (GE Healthcare).
Motility assays
Overnight cultures were adjusted to OD600 ~1.0 and the 1 µL suspension was spotted on the center of the 0.3% agar LB plates containing appropriate antibiotics and 0.2% arabinose, then incubated at room temperature. The diameter of the migration areas was measured at the indicated time points.
RT-qPCR analysis
Bacterial cells grown on 0.3% agar LB plate were collected and total RNA was extracted using the RZ solution (TIANGEN). Single-stranded cDNA was synthesized from 1 μg of total RNA using HiScript® III RT SuperMix (Vazyme, R323) with random hexamers as primers in a 20 μL reaction mixture. The resulting cDNA mixture was diluted to 10 ng/μL as a template for the subsequent quantification assays. Reverse transcription-quantitative PCR (RT-qPCR) was performed using a LightCycler® 96 SW (Roche) with specific primers, as described in Table S2. To standardize the results, the relative abundance of V. cholerae 16S gene was used as the internal standard. The fold change in gene transcription was determined using the comparative threshold cycle (CT) method.Citation36
Electron microscopy
Overnight bacteria were inoculated into 0.3% agar LB plate containing appropriate antibiotics and 0.2% arabinose and incubated at room temperature overnight. Strains were collected, adsorbed on a carbon type B 300-mesh copper grid (Ted Pella, Inc.), and stained with 3% uranyl acetate. Samples were imaged using a 120 kV transmission electron microscope (Talos L120C G2).
Fluorescence microscopy imaging assays
BL21(DE3) cells periplasmically expressing Tle1Vc and its catalytic mutant were grown to OD600 1.0, resuspended in 0.5× PBS and spotted on 1.5% agarose-LB pads containing 0.2% arabinose and fluorescence dyes. DiBAC4(3) and SYTOX blue, two commonly used cell membrane integrity dyes, were used to evaluate the dynamic process of bacterial deathCitation37. DiBAC4(3) (Invitrogen) is sensitive to membrane potential and will aggregate and show a bright green fluorescent signal when the cell membrane loses its potential. SYTOX blue (Invitrogen) can enter cells after the cell membrane loses selective permeability and stain nucleic acids in bright blue. All microscopy images were obtained using a ZEISS LSM900 (Carl Zeiss) inverted microscope with a Definite Focus 2.0 system to acquire stable long-period images and 100× oil immersion objective lens (Carl Zeiss). Each sample was observed continuously for 2 h, and images were captured every 20 s.
Bioinformatics analysis
All nucleotide and protein sequences of the microorganisms were acquired from the National Center for Biotechnology Information (NCBI) database. The genome of V. cholerae E1 can be found in the NCBI database (GenBank accession numbers: chromosome 1: CP110188.1; chromosome 2: CP110189.1). A total of 1338 V. cholerae genomes were downloaded from the NCBI database. BLAST analysis was performed using Tle1Vc as the query, and the cutoff value for Tle1Vc-positive was an HMMER bit score of 20 over the overall sequence comparison. Phylogenetic tree reconstruction was performed using MEGA 7.0, and sequence logos were generated using the Weblogo tool.
In vivo colonization assays
The animal experiments were approved by the Ethics Committee on Animal Experimentation of the Southern University of Science and Technology (project code: SUSTech-JY202203087).
The adult mouse model was used as previously described with the following modificationsCitation38. Five-week-old CD1 mice were fed water containing streptomycin (0.5%) and aspartame (0.4%) for 12 h before inoculation. For single colonization assays, V. cholerae wild-type and mutant V. cholerae were orally inoculated at 108 CFU into mice pretreated with streptomycin. The feces were collected daily. After 3-day post-infection, mice were sacrificed, and the small intestines, cecum, and large intestines were harvested and homogenized, and colonizing bacteria were counted by plating on LB agar plates. For competitive colonization assays, a 1:1 mixture of the wild type (LacZ−) and mutant (LacZ+) was orally inoculated with 108 CFU into mice, which were enumerated by plating on LB agar plates containing 40 μg/mL X-Gal (5-bromo-4-chloro-3-indolyl-β-Dgalactopyranoside). The competitive index (i.e., the output ratio of mutant to wild-type over the input ratio of mutant to wild-type) was calculated.
Results
Tle1Vc is a phospholipase antibacterial effector belonging to the Tle1 family
In a previous study, we isolated an environmental V. cholerae strain E1 with constitutive T6SS expression.Citation27 Using comparative genomic analysis, we predicted a new effector-immunity pair in the Aux1 cluster of E1 (no contain TseL effector) by comparing it to the N16961 reference genome (). Through Pfam database analysis, we predicted that this potential effector contained a DUF2235 domain, which belongs to the phospholipase effector Tle1 subfamily (), and named it Tle1Vc. We found that V. cholerae Tle1Vc homologs were mainly distributed in non-toxigenic V. cholerae and rarely in toxigenic V. cholerae (), indicating that Tle1Vc may be more important in non-toxigenic V. cholerae in complex environments. Phylogenetic analysis revealed that Tle1Vc is closely related to the known Tle1AH effector from Aeromonas hydrophila NJ-35 (, left) and that Tle1AH-mediated antibacterial activity depends on its delivery into the periplasm of the target bacteria.Citation21 To determine whether Tle1Vc functions in a similar way, we expressed Tle1Vc with or without a twin-arginine periplasmic translocation signal (Tat) in E. coli BL21 cells using an arabinose-inducible pBAD vector. The results showed that Tat-Tle1Vc, but not Tle1Vc, was toxic to E. coli BL21 ().
Figure 1. Tle1Vc is a lipase effector with antibacterial activity.
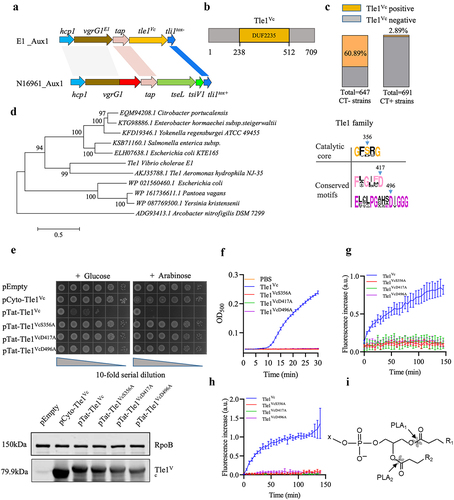
Our analysis of multiple sequence homology comparisons revealed two conserved motifs, FXGXXD (X is for any amino acid) and EXXXPGXXXDIG, in addition to the known lipase catalytic center motif GXSXG (, right). To determine whether these motifs are essential for Tle1Vc-induced toxicity, we mutated two aspartic acid residues (D417 and D496) and a serine residue (S356) to alanine within these motifs and tested the toxicity of Tle1Vc and its variants in the periplasm of E. coli BL21. Heterologous expression of Tat-Tle1Vc resulted in reduced survival, whereas mutant variants Tat-Tle1VcS356A, Tat-Tle1VcD417A, and Tat-Tle1VcD496A grew as well as the empty control (). Western blot analysis indicated that the expression level of these mutant proteins in E. coli BL21 cells were comparable to wild type (, below), suggesting that loss of catalytic activity was caused by active site mutations rather than instability of proteins expressed. To determine the enzymatic activity of Tle1Vc, we purified the effector and performed a lipase activity assay using Tween 20 as the catalytic substrate. We also purified the Tle1Vc mutant alleles for the enzyme activity assay. Tle1Vc showed significant catalytic activity, whereas the catalytic mutants Tle1VcS356A, Tle1VcD417A, and Tle1VcD496A were unable to hydrolyze the substrate as buffer control ( and S1a). Next we asked whether Tle1Vc possesses phospholipase activity. Using vesicle substrates doped with fluorescent phospholipid derivatives, our results showed that Tle1Vc had both PLA2 activity and PLA1 activity (). In summary, these data demonstrated that Tle1Vc is a phospholipase effector belonging to the Tle1 family, and its toxicity requires two conserved motifs in addition to the known GXSXG motif.
Tle1Vc alters membrane potential and permeability of target cell
TseL, a lipase effector previously reported in V. cholerae V52, belongs to the Tle2 family and has been shown to impairs cell membrane integrity by degrading phospholipids.Citation19,Citation22 To investigate whether Tle1Vc also induces membrane damage, we assessed its effect on membrane potential and permeability using voltage-dependent DiBAC4(3) dye and SYTOX blue nucleic acid dye, respectively, which can bind to the depolarized membrane and visualize cell death via time-lapse microscopy.Citation37 E. coli BL21 cells carrying Tat-Tle1Vc, Tat-Tle1VcS356A, Tat-Tle1VcD417A, or Tat-Tle1VcD496A were spotted individually on a thin 1.5% agarose LB pad containing the two dyes and 0.2% arabinose as an inducer of pBAD promoter. Under toxin-inducing conditions, E. coli BL21 cells expressing Tat-Tle1Vc showed simultaneous green and blue fluorescence, stained by DiBAC4(3) and SYTOX blue dye, respectively, compared with the empty vector (). In contrast, no fluorescence signal was observed in the cells expressing the three variants (). Quantitative analysis showed that the percentage of fluorescence signal of cells (a 30 × 30 µm field of at least 30 cells) periplasmically expressed Tle1Vc protein was notably higher than cells expressed its mutants or vector control (). These data demonstrated that Tle1Vc triggers membrane depolarization and permeabilization, resulting in cell death.
Figure 2. Tle1Vc effector impairs the integrity of bacterial membrane.
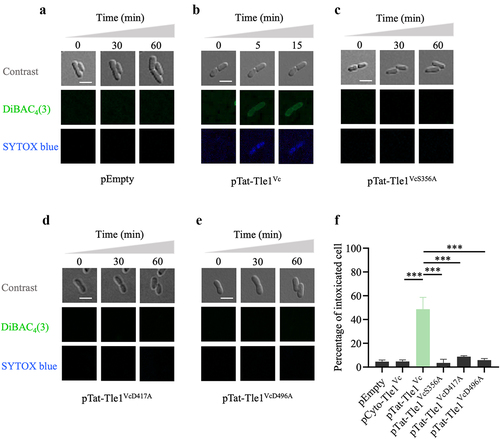
Tli1tox- specifically neutralizes Tle1Vc-induced toxicity
Genes encoding T6SS effectors and their corresponding immunity proteins are often found in pairs, with immunity genes located downstream of the effector genes. In the case of tle1Vc, an unannotated gene was identified downstream of the effector gene, which was designated Tli1tox- due to its homology to the Tli1tox+ protein encoded by toxigenic V. cholerae ( and S2). To investigate whether Tle1Vc-induced antibacterial activity could be neutralized by Tli1tox-, we generated a Δtle1Vc/tli1tox- mutant as prey in a competitive killing assay. Co-culturing the prey cells with E1 wild-type, Δtle1Vc, Δtle1Vc+pTle1Vc, and ΔvasK (inactive T6SS) as predators showed that cells lacking the tli1tox- immunity gene were susceptible to Tle1Vc positive predators, as the Δtle1Vc mutant and ΔvasK did not kill the Δtle1Vc/tli1tox- prey cells, whereas the wild type and Δtle1Vc+pTle1Vc markedly reduced their survival (). However, introducing tli1tox- into the Δtle1Vc/tli1tox- prey cells using an IPTG-inducible pSRKKm vector provided effective protection after induction (). These results demonstrate that Tle1Vc toxicity can be neutralized by Tli1tox-.
Figure 3. Tli1tox- specifically neutralizes the toxicity of Tle1Vc.
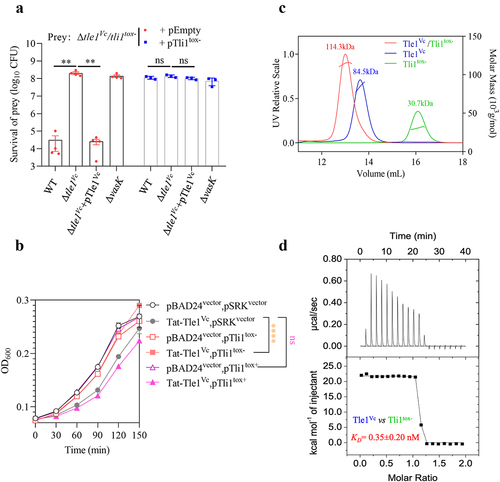
Bioinformatics analysis identified a hypothetical protein (Tli1tox+) encoded by the second gene downstream of tle2Vc (tseL) within the Aux1 cluster of in toxigenic V. cholerae N16961, which shares ~ 80% identity with Tli1tox- at the amino acid level ( and S2). To determine whether Tli1tox+ plays a defensive role against Tle1Vc toxicity, we co-expressed Tli1tox- and Tli1tox+ using an IPTG-inducible Ptac promoter and Tat-Tle1Vc using an arabinose-inducible PBAD promoter in E. coli BL21 cells. The results showed that cells expressing Tli1tox- grew well under Tle1Vc-induced toxic conditions as compared with the empty control, whereas cells expressing Tli1tox+ failed to do so (), indicating that Tli1tox- specifically neutralizes the toxicity of Tle1Vc.
Furthermore, we purified the effector and the two immunity proteins (Figure S1) to investigate their interaction with Tle1Vc using analytical gel filtration chromatography coupled with static light scattering and the isothermal calorimetric titration. When Tle1Vc, Tli1tox+, and Tli1tox- were added individually to the column, their individual unique peaks and molecular weights could be detected by laser scatter. However, when we mixed Tle1Vc with Tli1tox- and loaded the mixture into the column, the peaks representing individual proteins disappeared, and a new peak with a higher molecular weight appeared. The new complex peak corresponded to a molecular weight of 113.3 kDa, which was approximately equal to the combined molecular weight of Tle1Vc (84.5 kDa) and Tli1tox- (30.7 kDa) (). In contrast, Tli1tox+ failed to form a stable complex with Tle1Vc (Figure S3a). Moreover, the intensity of interaction was measured by the Isothermal Titration Calorimetry (ITC) assays. Tle1Vc and Tli1tox- binding was clearly endothermic and tight, at least in the low nanomolar KD range (~0.35 ± 0.20 nM) (), while no significant interaction was observed between the Tli1tox+ and Tle1Vc (Fig. S3B). These data suggested that Tli1tox- can specifically protect cells from Tle1Vc-induced intoxication by direct interaction with Tle1Vc.
Tle1Vc delivery requires T6SS and the carrier VgrG1E1
To confirm whether functional T6SS is necessary for Tle1Vc secretion, western blotting was performed to detect Tle1Vc secretion in both the wild-type strain and the ΔvasK (T6SS-inactive) strain. The results showed that Tle1Vc was present in the pellets of both strains, but only in the supernatant of the wild type, indicating that Tle1Vc secretion strictly depends on functional T6SS ().
Figure 4. Tle1Vc delivery requires functional T6SS and VgrG1E1.
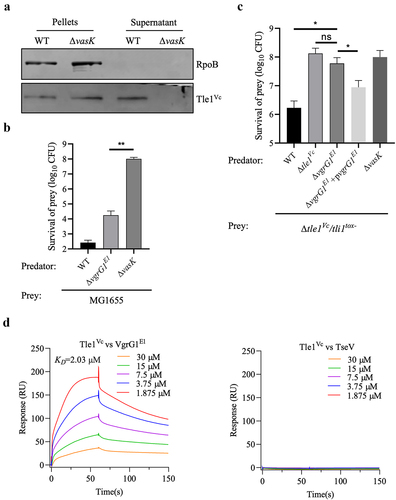
Since T6SS effectors require interaction with adjacent structural proteins such as PAAR, Hcp, and VgrGCitation6, we assessed the effect of the upstream protein VgrG1E1 on Tle1Vc delivery via bacterial competition assays. As expected, the ΔvgrG1E1 mutant, which retained T6SS activity, abolished the killing effect of the effector-immunity mutant Δtle1Vc/tli1tox- compared to the wild type. However, complementation of the ΔvgrG1E1 mutant with vgrG1E1 restored the killing ability of Δtle1Vc/tli1tox- prey (), indicating that VgrG1E1 acts as a carrier for Tle1Vc secretion. To further confirm this conclusion, VgrG1E1 and Tle1Vc were purified (Figure S1a,c), and their interaction intensity was analyzed using the Surface Plasmon Resonance (SPR) assays. Results showed that Tle1Vc can bind to VgrG1E1 with a KD of 2.03 μM, but can not interact with negative control TseV (an unpublished T6SS effector) (). The results indicated that Tle1Vc could directly bind to VgrG1E1 for delivery.
T6SS-delivered Tle1Vc contributes to Vibrio cholerae intra-/inter- species antagonism
Previous studies have demonstrated that the Type VI secretion system (T6SS) plays a crucial role in intra- and inter-species competition in Vibrio cholerae.Citation39,Citation40 In V. cholerae, T6SS function was abolished by completely deleting multiple effectors but not by point mutagenesis, which inactivates multiple effectors.Citation41 Among the four effectors encoded by V. cholerae E1, Tle1Vc is a lipase effector, VasX is a pore-forming effector, VgrG3cp targets the cell wall, and TseV is an uncharacterized effector.Citation27,Citation42 To assess the role of Tle1Vc in bacterial competition, we created a mutant strain (4eff) that inactivated the catalytic mutants of vgrG3cpD867ACitation27 and tle1VcD417A, deleted vasXΔC16Citation42, and deleted tseV. This mutant strain retained T6SS secretion but abolished the antibacterial killing toward effector-cognate immunity mutants (Figure S4a,b). In competitive killing assays, we co-incubated the 3eff predator strain (only Tle1Vc remained active) and the 4eff predator strain with prey cells, such as V. cholerae C6706, V. vulnificus, V. parahaemolyticus 1360, E. coli MG1655, A. hydrophila, and E. aerogenes. The results demonstrated that the 3eff predator strain effectively killed all the prey cells tested, whereas the 4eff strain exhibited killing activity similar to that of the ΔvasK mutant in all competition assays (). Therefore, the T6SS-secreted Tle1Vc effector can promote intra- and inter-species competition in Vibrio cholerae E1.
Figure 5. Tle1Vc contributes to Vibrio cholerae intra- and interspecies antagonism.
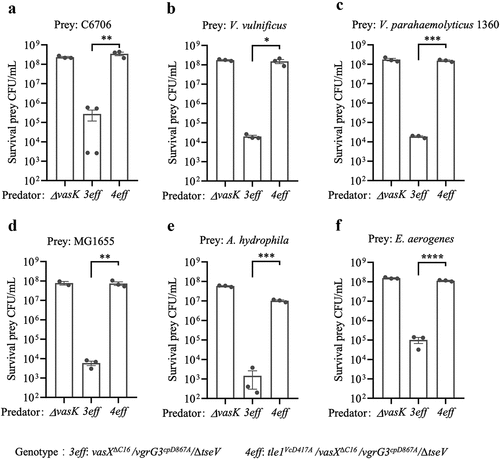
Physical puncture of T6SS and catalytic activity of effector are essential to Tle1Vc-induced strong tit-for-tat response of P. aeruginosa
To test whether Tle1Vc could induce a tit-for-tat (TFT) response, we performed experiments with Pseudomonas aeruginosa PAO1, which uses its T6SS to counterattack other attacking bacteria.Citation43 The results showed that a tle1Vc-inactivated strain of V. cholerae led to a higher number of surviving CFUs in PAO1 than in the wild type, whereas complementation with tle1Vc in the inactivated strain reduced the survival number to that of the wild-type group (). When the T6SS-H1 cluster of PAO1 was inactivated, we failed to observe counterattack from PAO1 ΔtssB1 after a T6SS attack by V. cholerae E1 wild-type and Δtle1Vc+pTle1Vc strains (). This suggests that Tle1Vc induces a strong TFT response in PAO1 via its T6SS-H1 cluster counterattack. We further evaluated the toxicity of Tle1Vc on PAO1 and found that cells expressing Tat-Tle1Vc grew poorly compared to the other Tat-Tle1Vc variants and the Tat-GFP control (). However, the surviving CFU of cells expressing Tat-Tle1Vc in LB-agar plates were comparable to those of the three Tat-Tle1Vc variants and Tat-sfGFP control (). We also tested whether endogenous Tle1Vc was sufficient to stimulate the TFT response of PAO1. The results showed that Tle1Vc could not directly induce a TFT response (), which differs from the lipase effector TseL (or Tle2Vc).Citation44 Thus, we speculate that the Tle1Vc-induced strong TFT response may require the presence of physical puncture or the synergy of other T6SS effectors. We used the multiple-effector-inactivation mutant 4eff (retained T6SS physical puncture and lost the killing assay; Figure S4a,b) and ΔvasK (no physical puncture) to test the first hypothesis. V. cholerae E1 4eff and ΔvasK were co-incubated with PAO1 expressing pPSV37 empty vector or its derivatives of Tle1Vc. As expected, the CFU of surviving 4eff after a T6SS attack by P. aeruginosa expressing Tat-Tle1Vc obviously decreased compared to the empty vector control, whereas the CFU of surviving ΔvasK after the same T6SS attack did not change (), suggesting that T6SS physical puncture is essential for Tle1Vc-induced strong TFT response. Notably, a single physical puncture can directly stimulate the TFT response of PAO1, but the effect was moderate compared to the presence of the effector Tat-Tle1Vc (). We further assessed the role of the catalytic activity of Tle1Vc on TFT response. The survival of V. cholerae E1 4eff and ΔvasK after a T6SS attack by PAO1 expressing three Tle1Vc mutant variants were examined, and the results indicated that the CFU of surviving 4eff was obviously increased in the three Tle1Vc variant groups compared to the Tle1Vc group and was comparable to that of the empty plasmid group (), suggesting that the catalytic activity of Tle1Vc was also found to be vital to TFT response. Collectively, these data demonstrate that Tle1Vc triggers TFT response, which is dependent on its catalytic activity, the T6SS-H1 cluster of PAO1, and physical puncture of V. cholerae E1 T6SS.
Figure 6. Tle1vc-induced strong tit-for-tat response requires the T6SS-H1 of PAO1, catalytic activity of effector and the physical puncture of T6SS.
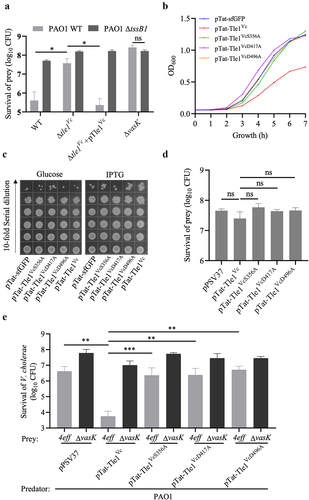
Tle1Vc mediates motility of Vibrio cholerae E1 by controlling flagellar synthesis
In addition to its antibacterial and anti-eukaryotic effects, T6SS also plays a role in the function of multiple virulence factors.Citation45–47 The effect of Tle1Vc on bacterial motility and biofilm formation was tested, and the results showed no significant difference in biofilm formation between the wild-type and Δtle1Vc strains. However, the motility of the Δtle1Vc mutant was noticeably decreased compared to that of the wild-type, and this phenotype could be restored after the Δtle1Vc mutant was complemented with tle1Vc (Δtle1Vc +pTle1Vc) (). Notably, the ΔvasK mutant maintained motility similar to that of the wild-type, indicating that Tle1Vc-dependent motility does not require T6SS function. Since the catalytic activity of Tle1Vc is vital to the tit-for-tat response and antibacterial activity, the effect of catalytic activity on motility was further evaluated. The Tle1Vc-inactivated chromosomal mutant tle1VcD417A showed attenuated motility compared to the wild type, indicating that Tle1Vc-mediated motility requires its catalytic activity.
Figure 7. Phospholipase effector Tle1Vc mediates bacterial motility through modulating flagellar gene expression.
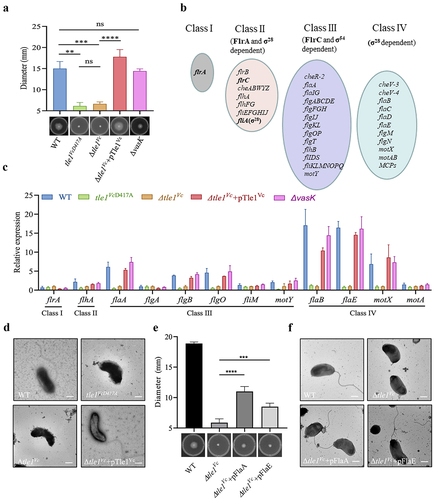
Vibrio cholerae is motile through a single polar flagellumCitation48, which is typically composed of three major structural components encoded by multiple genes: the basal body, hook, and filament.Citation49,Citation50 The expression of V. cholerae flagellar genes is regulated by a four-tiered transcriptional hierarchyCitation48 (). Therefore, the effect of Tle1Vc on flagellar gene expression was examined by qPCR. As expected, the expression of several flagellar genes was downregulated at the transcriptional level in the Δtle1Vc and tle1VcD417A mutants compared to that in the wild type (). However, the expression of downregulated flagellar genes was restored to the expression level of the wild-type in the Δtle1Vc +pTle1Vc strain (). Electron microscopy imaging assays showed that a single bacterium of the Δtle1Vc and tle1VcD417A mutants lost the singular flagellum, whereas the Δtle1Vc +pTle1Vc strain recovered the singular flagellum, as observed in the wild type (). To further determine whether Tle1Vc-mediated motility defect could be restored by complementing downregulated flagellar genes in Δtle1Vc mutant, we evaluated two representative flagellar genes, flaA and flaE, whose expressions are important for flagellar synthesis of Vibrio choleraeCitation51. Results of complementation assays showed that expression of flaA or flaE could partially restore motility of Δtle1Vc (), which was consistent with the result of electron microscopy imaging (), suggesting that the flagellar synthesis is responsible for motility defect mediated by the Tle1Vc.
Antimicrobial activity mediated by Tle1Vc contribute to in vivo fitness of V. cholerae E1
Toxigenic V. cholerae is a noninvasive pathogen that colonizes the small intestine through the expression of the toxin-co-regulated pilus (TCP), an essential colonization factor of Vibrio cholerae.Citation44 Although environmental V. cholerae E1 lacks known virulence factors such as TCP and CT, it was found to colonize the cecum rather than the small intestine of adult mice (Figure S5a,b). T6SS has been reported to regulate bacterial colonization in the host.Citation52,Citation53 To investigate whether the T6SS of E1 plays a role in in vivo fitness, we orally infected mice pretreated with streptomycin (to suppress gut resident microbes) with a 1:1 mixture of E1 wild-type (T6SS+; LacZ−) strain and ΔvasK (T6SS−; LacZ+) mutant. We observed a significant colonization defect of the T6SS− strain compared to the T6SS+ strain in the cecum as well as in the large intestine (Figure S5c,d). As the growth rate of T6SS− mutant is similar to wild type (Figure S5e), suggesting that E1 can utilize its T6SS to increase in vivo competitiveness.
Next, we assessed the role of Tle1Vc in the gut colonization of E1 by comparing the number of bacteria colonized by the wild-type and Δtle1Vc mutant strains. We observed a significant colonization defect of the Δtle1Vc mutant in the cecum compared to the wild-type strain (). As Tle1Vc mediates both motility and antibacterial abilities in E1, motility plays a significant role in V. cholerae colonization. To investigate the importance of motility, we generated a motility-deficient mutant of E1, designated as ΔflaA, and compared its motility ability to that of the wild type. The results demonstrated that ΔflaA lost its motility ability (). Subsequently, we evaluated the impact of motility on E1 colonization. In comparison to the wild type, the colonization level of ΔflaA in the cecum was similar (), indicating that motility of E1 was not essential for cecum colonization. By performing competitive colonization assays with the wild-type (LacZ−) and sensitive Δtle1Vc/tli1tox- mutant (LacZ+). We found that the number of colonies of the Δtle1Vc/tli1tox- mutant gradually decreased over infection time compared to that of the wild-type strain in feces (), and the Δtle1Vc/tli1tox- mutant showed a huge colonization defect in the cecum (), showing that Tle1Vc is active in vivo. These results suggest that Tle1Vc-mediated antibacterial activity contributes to in vivo fitness rather than motility.
Figure 8. The effect of Tle1Vc on E1’s in vivo fitness and survival of immunity mutant.
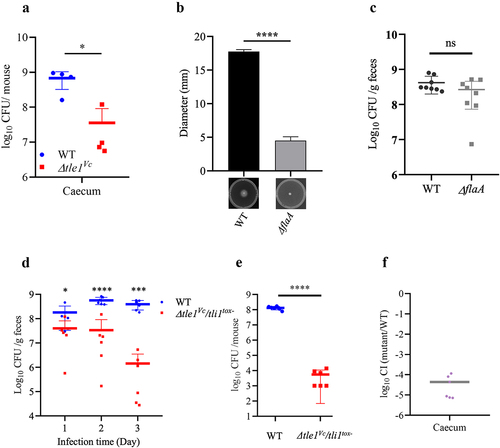
Discussion
The type VI secretion system (T6SS) delivers effectors into neighboring cells to enhance competitiveness, and the cell membrane is a common target for these effectors. For example, Tle1 of Burkholderia thailandensis and Aeromonas hydrophila, Tle2 and VasX of V. cholerae, Tle1, Tle4 and Tse4 of P. aeruginosa, and Tle1 and Tle5 of K. pneumoniae all have been shown to hydrolyze the membrane phospholipids or act on membrane by pore-forming.Citation19,Citation21,Citation25,Citation42,Citation54–56 In this study, we identified a putative T6SS lipase effector Tle1Vc in Aux1 cluster of the atypical pathogenic V. cholerae E1 based on bioinformatics analysis. By analyzing its amino acid sequence, we identified a conserved DUF2235 domain and lipase catalytic core motif GXSXG, which are widely distributed in Tle1 lipase family. Tle1 proteins have been established as a subfamily of T6SS phospholipase effectors, exhibiting either phospholipase A2 activity or a combination of phospholipase A1 and A2 activities.Citation19,Citation24 In this study, we demonstrated that Tle1Vc possesses both phospholipase A2 activity and a similar phospholipase A1 activity. Conversely, another Tle1 effector, Tle1EAEC, has been shown to possess phospholipase A1 activity and a lower level of phospholipase A2 activity.Citation24 These findings underscore the importance of characterizing the phospholipase activity profile of the Tle1 family in elucidating the antibacterial mechanisms and biological roles of other Tle1 family effectors.
According to multiple sequence alignment, two Tle1 family conserved motifs FXGXXD and EXXXPGXXXDIG in addition to catalytic center motif GXSXG were identified in Tle1Vc. By heterologous expression assays, we determined that the S356, D417 and D496 residues of Tle1Vc were indispensable for Tle1Vc-catalyzed toxicity, which was consistent with the result of enzyme activity assays. The structure and active domain of Tle1PA of P. aeruginosa have been shown that the catalytic triad (S235-D279-H377) is vital for its toxicity.Citation25 Although Tle1PA and Tle1Vc only share ~ 16% identity in their amino acid sequences, the D417 and S356 residues of FXGXXD and GXSXG motifs corresponds to D279-S235 of Tle1PA, and the D496 residue of Tle1Vc and the H377 residue of Tle1PA are adjacent within EXXXPGXHXDIG motif, showing that Tle1Vc may use the same catalytic mode to degrade phospholipids as Tle1PA. After being injected into neighboring cells by the T6SS, Tle1BT and Tle2Vc are able to increase cellular permeability by hydrolyzing the membrane phospholipids of target cells.Citation19 Using time-lapse microscopy, we also demonstrate that Tle1Vc increased the permeability of cell membrane and caused cell death. While P. aeruginosa PAO1 cells demonstrated differential resistance to Tle1Vc under solid and liquid LB culturing conditions, the toxicity mediated by Tle1Vc was notably milder in liquid medium compared to solid medium. These discrepancies may be attributed to the presence of metal ions in agar, as previous studies have reported their involvement in mediating the killing activity of T6SS effectors.Citation57 PAO1 has evolved unknown pathways to counteract T6SS effectors (VgrG3 and VasX) of V. cholerae, in contrast to E. coli MG1655.Citation22 Furthermore, PAO1 encodes over 1000 more proteins than MG1655, which could contribute to its enhanced protective capacity against phospholipase-mediated membrane damage inflicted by Tle1Vc. Further investigations are required to fully understand the underlying mechanisms.
In the present study, we identified two putative immunity proteins, Tli1tox- and Tli1tox+, encoded by genes downstream of tle1Vc and tle2Vc, respectively, in environmental V. cholerae E1 and toxigenic N16961 strains. Bioinformatics analysis revealed that these cognate proteins belong to the DUF2931 family and are predicted to possess signal peptide sequences at their N-termini, consistent with the localization of Tle1Vc. However, functional analysis demonstrated that Tli1tox- was essential for neutralizing the toxicity of Tle1Vc through direct interaction, whereas Tli1tox+ did not exhibit this protective role, despite sharing approximately 80.24% sequence identity. It has been reported that mutations in immunity proteins can lead to a decrease in V. cholerae‘s intraspecies competition capacity.Citation58 Further investigation is needed to elucidate whether variations in amino acid residues in Tli1tox+ resulted in the loss of interaction with Tle1Vc.
In addition to conferring interbacterial competition, the T6SS may also play multiple physiological roles, including bacterial motility, protease activity, biofilm formation and can provoke tit-for-tat response from Pseudomonas aeruginosa.Citation22,Citation59 In this study, we demonstrated that inactivation or deletion of Tle1Vc impacts motility of V. cholerae E1 by downregulating the expression of flagellar relative genes such as flgB, flgO, flaABE and motXY, whose expression is essential for flagellar synthesis.Citation48,Citation50,Citation51 And the motility of Δtle1Vc could be partially restored by complementing with flagellar gene flaA or flaE. These data were consistent with the result of electron microscopy imaging assays. Furthermore, motility induced by Tle1Vc is independent of the functional T6SS but relies on Tle1Vc-catalytic activity. As T6 effectors, VgrG3 and TseL, despite lacking a classical Sec or TAT secretion signal, have been observed in the periplasm through heterologous expression in E. coli, even in the absence of T6SS. This suggests that T6 effectors can reach the periplasm independently of T6SS,Citation60 potentially leading to Tle1Vc-mediated motility independent of an active T6SS. The regulation of flagellum biosynthesis is a complex process. Cell envelope stress response systems and defense-related genes have been identified as factors influencing flagellum-driven motility.Citation61 For instance, the Rcs phosphorelay system, which responds to outer membrane damage, as well as two-component regulatory systems BaeSR and WigKR, which participate in the cellular response to T6SS effectors and provide protection against foreign toxins,Citation62–64 suggest that cell envelope stress response systems may integrate effector damage as a signal to initiate a cascade response, potentially associated with Tle1Vc-driven motility. Additionally, T6SS-dependent Tle1Vc also triggers tit-for-tat (TFT) response and this process depends on T6SS-H1 counterattack of P. aeruginosa and its catalytic activity, as observed in Tle2VcCitation22. Interestingly, we found that physical puncture of V. cholerae T6SS can moderately induce T6SS-H1-mediated counterattack of P. aeruginosa, showing that single physical penetration is sufficient for inducing TFT response. Furthermore, the expression of Tle1Vc in P. aeruginosa PAO1 could not stimulate TFT response and Tle1Vc-mediated strong TFT response was dependent on the physical puncture of V. cholerae E1, which differs from Tle2Vc-mediated TFT response that the expression of Tle2Vc alone in PAO1 was sufficient for inducing T6SS-H1-mediated counterattack.Citation22 As Tle1Vc and Tle2Vc have various phospholipase activities responsible for different bactericidal capacity toward P. aeruginosa and E. coli,Citation19,Citation22,Citation32 which may result in distinct TFT responses stimulated by them. Taken together, these findings indicate that the effects of T6SS and Tle1Vc proteins on microorganism physiology are multidimensional and complicated.
In addition to being an anti-bacterial weapon, T6SS allows pathogens to compete with commensal microbiota during host colonization.Citation52,Citation53,Citation65 To better understand the role of E1 T6SS, we carried out mouse colonization assay and verified that T6SS could promote E1’s in vivo fitness. T6SS-delivered Tle1Vc is shown to present bactericidal activity in vivo and confer V. cholerae E1 a competitive advantage in the cecum of adult mice, which was also further confirmed by competitive colonization experiments in which the E1 wild type strain showed a significant competitive advantage (>104 fold, ) over Δtle1Vc/tli1tox- prey strain in the cecum, similar to in vitro killing efficiency (). E1 T6SS had stronger dynamic in vivo than toxigenic strain which has a much lower in vivo killing rate (<10 fold).Citation57,Citation65 These results imply that the atypical pathogenic V. cholerae E1 has more higher in vivo killing capacity than toxigenic V. cholerae strain. In conclusion, the data presented here provide a strong evidence that Tle1Vc of V. cholerae E1 serves as a T6SS effector belonging to Tle1 lipase family and its homologs are widely distributed among different bacterial species. Our finding suggested that Tle1Vc not only acts as a antimicrobial toxin that plays important roles in antagonistic bacterium-bacterium interactions, also is involved in a number of cellular processes.
Supplemental Material
Download Zip (3.8 MB)Acknowledgments
We thank Prof. Zhaoqing Luo for helpful discussion and Prof. Liang Yang for providing us with the P. aeruginosa PAO1 strain.
Disclosure statement
No potential conflict of interest was reported by the author(s).
Data availability statement
The authors confirm that the data supporting the findings of this study are available within the article and its supplementary materials.
Supplementary material
Supplemental data for this article can be accessed online at https://doi.org/10.1080/19490976.2023.2241204
Additional information
Funding
References
- Clemens JD, Nair GB, Ahmed T, Qadri F, Holmgren J. Cholera. Lancet. 2017 Sep 23;390(10101):1539–22. doi:10.1016/S0140-6736(17)30559-7.
- Sack DA, Sack RB, Nair GB, Siddique AK. Cholera. Lancet. 2004 Jan 17;363(9404):223–233. doi:10.1016/S0140-6736(03)15328-7.
- Faruque SM, Albert MJ, Mekalanos JJ. Epidemiology, genetics, and ecology of toxigenic Vibrio cholerae. Microbiol Mol Biol Rev. 1998 Dec;62(4):1301–1314. doi:10.1128/MMBR.62.4.1301-1314.1998.
- Fu Y, Waldor MK, Mekalanos JJ. Tn-Seq analysis of Vibrio cholerae intestinal colonization reveals a role for T6SS-mediated antibacterial activity in the host. Cell Host & Microbe. 2013 Dec 11;14(6):652–663. doi:10.1016/j.chom.2013.11.001.
- Dong TG, Dong S, Catalano C, Moore R, Liang X, Mekalanos JJ. Generation of reactive oxygen species by lethal attacks from competing microbes. Proc Natl Acad Sci USA. 2015 Feb 17;112(7):2181–2186. doi:10.1073/pnas.1425007112.
- Cherrak Y, Flaugnatti N, Durand E, Journet L, Cascales E. Structure and activity of the Type VI secretion system. Microbiol Spectr. 2019 Jul;7(4). doi:10.1128/microbiolspec.PSIB-0031-2019
- Yu KW, Xue P, Fu Y, Yang L. T6SS mediated stress responses for bacterial environmental survival and host adaptation. Int J Mol Sci. 2021 Jan 6;22(2):478. doi:10.3390/ijms22020478.
- Mougous JD, Cuff ME, Raunser S, Shen A, Zhou M, Gifford CA, Goodman AL, Joachimiak G, Ordoñez CL, Lory S, et al. A virulence locus of Pseudomonas aeruginosa encodes a protein secretion apparatus. Science. 2006 Jun 9;312(5779):1526–1530. doi:10.1126/science.1128393.
- Pukatzki S, Ma AT, Sturtevant D, Krastins B, Sarracino D, Nelson WC, Heidelberg JF, Mekalanos JJ. Identification of a conserved bacterial protein secretion system in Vibrio cholerae using the Dictyostelium host model system. Proc Natl Acad Sci USA. 2006 Jan 31;103(5):1528–1533. doi:10.1073/pnas.0510322103.
- Boyer F, Fichant G, Berthod J, Vandenbrouck Y, Attree I. Dissecting the bacterial type VI secretion system by a genome wide in silico analysis: what can be learned from available microbial genomic resources? Bmc Genom. 2009 Mar 12;10(1):104. doi:10.1186/1471-2164-10-104.
- Cherrak Y, Rapisarda C, Pellarin R, Bouvier G, Bardiaux B, Allain F, Malosse C, Rey M, Chamot-Rooke J, Cascales E, et al. Biogenesis and structure of a type VI secretion baseplate. Nature microbiol. 2018 Dec;3(12):1404–1416. doi:10.1038/s41564-018-0260-1.
- Nguyen VS, Logger L, Spinelli S, Legrand P, Huyen Pham TT, Nhung Trinh TT, Cherrak Y, Zoued A, Desmyter A, Durand E, et al. Type VI secretion TssK baseplate protein exhibits structural similarity with phage receptor-binding proteins and evolved to bind the membrane complex. Nature microbiol. 2017 Jun 26;2(9):17103. doi:10.1038/nmicrobiol.2017.103.
- Bonemann G, Pietrosiuk A, Mogk A. Tubules and donuts: a type VI secretion story. Mol Microbiol. 2010 May;76(4):815–821. doi:10.1111/j.1365-2958.2010.07171.x.
- Kapitein N, Bonemann G, Pietrosiuk A, Seyffer F, Hausser I, Locker JK, Mogk A. ClpV recycles VipA/VipB tubules and prevents non-productive tubule formation to ensure efficient type VI protein secretion. Mol Microbiol. 2013 Mar;87(5):1013–1028. doi:10.1111/mmi.12147.
- Brackmann M, Wang J, Basler M. Type VI secretion system sheath inter-subunit interactions modulate its contraction. EMBO Rep. 2018 Feb;19(2):225–233. doi:10.15252/embr.201744416.
- Nguyen VS, Douzi B, Durand E, Roussel A, Cascales E, Cambillau C. Towards a complete structural deciphering of Type VI secretion system. Curr Opin Struct Biol. 2018 Apr;49:77–84. doi:10.1016/j.sbi.2018.01.007.
- Ma J, Pan Z, Huang J, Sun M, Lu C, Yao H. The Hcp proteins fused with diverse extended-toxin domains represent a novel pattern of antibacterial effectors in type VI secretion systems. Virulence. 2017 Oct 3;8(7):1189–1202. doi:10.1080/21505594.2017.1279374.
- Jurenas D, Journet L. Activity, delivery, and diversity of Type VI secretion effectors. Mol Microbiol. 2021 Mar;115(3):383–394. doi:10.1111/mmi.14648.
- Russell AB, LeRoux M, Hathazi K, Agnello DM, Ishikawa T, Wiggins PA, Wai SN, Mougous JD. Diverse type VI secretion phospholipases are functionally plastic antibacterial effectors. Nature. 2013 Apr 25;496(7446):508–512. doi:10.1038/nature12074.
- Egan F, Reen FJ, O’Gara F. Tle distribution and diversity in metagenomic datasets reveal niche specialization. Environ Microbiol Rep. 2015 Apr;7(2):194–203. doi:10.1111/1758-2229.12222.
- Ma S, Dong Y, Wang N, Liu J, Lu C, Liu Y. Identification of a new effector-immunity pair of Aeromonas hydrophila type VI secretion system. Vet Res. 2020 May 24;51(1):71. doi:10.1186/s13567-020-00794-w.
- Kamal F, Liang X, Manera K, Pei T-T, Kim H, Lam LG, Pun A, Hersch SJ, Dong TG. Differential cellular response to translocated toxic effectors and physical penetration by the Type VI secretion system. Cell Rep. 2020 Jun 16;31(11):107766. doi:10.1016/j.celrep.2020.107766.
- Crisan CV, Chande AT, Williams K, Raghuram V, Rishishwar L, Steinbach G, Watve SS, Yunker P, Jordan IK, Hammer BK, et al. Analysis of Vibrio cholerae genomes identifies new type VI secretion system gene clusters. Genome Biol. 2019 Aug 12;20(1):163. doi:10.1186/s13059-019-1765-5.
- Flaugnatti N, Le TT, Canaan S, Aschtgen M-S, Nguyen VS, Blangy S, Kellenberger C, Roussel A, Cambillau C, Cascales E, et al. A phospholipase A1 antibacterial Type VI secretion effector interacts directly with the C-terminal domain of the VgrG spike protein for delivery. Mol Microbiol. 2016 Mar;99(6):1099–1118. doi:10.1111/mmi.13292.
- Hu H, Zhang H, Gao Z, Wang D, Liu G, Xu J, Lan K, Dong Y. Structure of the type VI secretion phospholipase effector Tle1 provides insight into its hydrolysis and membrane targeting. Acta Crystallogr D Biol Crystallogr. 2014 Aug;70(8):2175–2185. doi:10.1107/S1399004714012899.
- Tam VC, Serruto D, Dziejman M, Brieher, W., Mekalanos, J J. A type III secretion system in Vibrio cholerae translocates a formin/spire hybrid-like actin nucleator to promote intestinal colonization. Cell Host & Microbe. 2007 Apr 19;1(2):95–107. doi:10.1016/j.chom.2007.03.005.
- Liu M, Zhao MY, Wang H, Wang Z-H, Wang Z, Liu Y, Li Y-P, Dong T, Fu Y. Pesticin-like effector VgrG3 cp targeting peptidoglycan delivered by the Type VI secretion system contributes to Vibrio cholerae interbacterial competition. Microbiol Spectr. 2023 Jan 10;11(1):e0426722. doi:10.1128/spectrum.04267-22.
- Philippe N, Alcaraz JP, Coursange E, Geiselmann J, Schneider D. Improvement of pCVD442, a suicide plasmid for gene allele exchange in bacteria. Plasmid. 2004 May;51(3):246–255. doi:10.1016/j.plasmid.2004.02.003.
- Metcalf WW, Jiang W, Daniels LL, Kim S-K, Haldimann A, Wanner BL. Conditionally replicative and conjugative plasmids CarryinglacZα for cloning, mutagenesis, and allele replacement in bacteria. Plasmid. 1996 Jan;35(1):1–13. doi:10.1006/plas.1996.0001.
- Choi KH, Schweizer HP. An improved method for rapid generation of unmarked Pseudomonas aeruginosa deletion mutants. BMC Microbiol. 2005 May 23;5(1):30. doi:10.1186/1471-2180-5-30.
- Guzman LM, Belin D, Carson MJ, Beckwith J. Tight regulation, modulation, and high-level expression by vectors containing the arabinose PBAD promoter. J Bacteriol. 1995 Jul;177(14):4121–4130. doi:10.1128/jb.177.14.4121-4130.1995.
- Liang X, Kamal F, Pei TT, Xu P, Mekalanos JJ, Dong TG. An onboard checking mechanism ensures effector delivery of the type VI secretion system in Vibrio cholerae. Proc Natl Acad Sci USA. 2019 Nov 12;116(46):23292–23298. doi:10.1073/pnas.1914202116.
- Khan SR, Gaines J, Roop RM 2nd, Farrand SK. Broad-host-range expression vectors with tightly regulated promoters and their use to examine the influence of TraR and TraM expression on Ti plasmid quorum sensing. Appl Environ Microbiol. 2008 Aug;74(16):5053–5062. doi:10.1128/AEM.01098-08.
- von Tigerstrom RG, Stelmaschuk S, Tigerstrom RGV. The use of Tween 20 in a sensitive turbidimetric assay of lipolytic enzymes. Can J Microbiol. 1989 Apr;35(4):511–514. doi:10.1139/m89-079.
- Zottig X, Meddeb-Mouelhi F, Beauregard M. Development of a high-throughput liquid state assay for lipase activity using natural substrates and rhodamine B. Anal Biochem. 2016 Mar 1;496:25–29. doi:10.1016/j.ab.2015.11.020.
- Livak KJ, Schmittgen TD. Analysis of relative gene expression data using real-time quantitative PCR and the 2−ΔΔCT method. Methods. 2001 Dec;25(4):402–408. doi:10.1006/meth.2001.1262.
- Quereda JJ, Meza-Torres J, Cossart P, Pizarro-Cerdá J. Listeriolysin S: A bacteriocin from epidemic Listeria monocytogenes strains that targets the gut microbiota. Gut Microbes. 2017;8(4):384–391. doi:10.1080/19490976.2017.1290759.
- Liu M, Hao G, Li Z, Zhou Y, Garcia-Sillas R, Li J, Wang H, Kan B, Zhu J, et al. CitAB two-component system-regulated citrate utilization contributes to Vibrio cholerae competitiveness with the gut microbiota. Infect Immun. 2019 Mar;87(3). doi:10.1128/IAI.00746-18.
- Drebes Dorr NC, Blokesch M. Interbacterial competition and anti-predatory behaviour of environmental Vibrio cholerae strains. Environ Microbiol. 2020 Oct;22(10):4485–4504. doi:10.1111/1462-2920.15224.
- Unterweger D, Miyata ST, Bachmann V, Brooks TM, Mullins T, Kostiuk B, Provenzano D, Pukatzki S. The Vibrio cholerae type VI secretion system employs diverse effector modules for intraspecific competition. Nat Commun. 2014 Apr 1;5(1):3549. doi:10.1038/ncomms4549.
- Dong TG, Ho BT, Yoder-Himes DR, Mekalanos JJ. Identification of T6SS-dependent effector and immunity proteins by Tn-seq in Vibrio cholerae. Proc Natl Acad Sci USA. 2013 Feb 12;110(7):2623–2628. doi:10.1073/pnas.1222783110.
- Miyata ST, Kitaoka M, Brooks TM, McAuley SB, Pukatzki S. Vibrio cholerae requires the type VI secretion system virulence factor VasX to kill Dictyostelium discoideum. Infect Immun. 2011 Jul;79(7):2941–2949. doi:10.1128/IAI.01266-10.
- Basler M, Ho BT, Mekalanos JJ. Tit-for-tat: type VI secretion system counterattack during bacterial cell-cell interactions. Cell. 2013 Feb 14;152(4):884–894. doi:10.1016/j.cell.2013.01.042.
- Taylor RK, Miller VL, Furlong DB, Mekalanos JJ. Use of phoA gene fusions to identify a pilus colonization factor coordinately regulated with cholera toxin. Proc Natl Acad Sci USA. 1987 May;84(9):2833–2837. doi:10.1073/pnas.84.9.2833.
- Wang P, Dong JF, Li RQ, Li L, Zou Q-H. Roles of the Hcp family proteins in the pathogenicity of Salmonella typhimurium 14028s. Virulence. 2020 Dec;11(1):1716–1726. doi:10.1080/21505594.2020.1854538.
- Li Y, Chen L, Zhang P, Bhagirath AY, Duan K. ClpV3 of the H3-Type VI secretion system (H3-T6SS) affects multiple virulence factors in Pseudomonas aeruginosa. Front Microbiol. 2020;11:1096. doi:10.3389/fmicb.2020.01096.
- Zhang LQ, Xu JS, Xu J, Zhang H, He L, Feng J. TssB is essential for virulence and required for Type VI secretion system in Ralstonia solanacearum. Microb Pathog. 2014 Sep;74:1–7. doi:10.1016/j.micpath.2014.06.006.
- Klose KE, Mekalanos JJ. Differential regulation of multiple flagellins in Vibrio cholerae. J Bacteriol. 1998 Jan;180(2):303–316. doi:10.1128/JB.180.2.303-316.1998.
- Prouty MG, Correa NE, Klose KE. The novel sigma54- and sigma28-dependent flagellar gene transcription hierarchy of Vibrio cholerae. Mol Microbiol. 2001 Mar;39(6):1595–1609. doi:10.1046/j.1365-2958.2001.02348.x.
- Echazarreta MA, Klose KE. Vibrio flagellar synthesis. Front Cell Infect Microbiol. 2019;9:131. doi:10.3389/fcimb.2019.00131.
- Echazarreta MA, Kepple JL, Yen LH, Chen Y, Klose KE. A critical region in the FlaA flagellin facilitates filament formation of the Vibrio cholerae flagellum. J Bacteriol. 2018 Aug 1;200(15). doi:10.1128/JB.00029-18.
- Song L, Pan J, Yang Y, Zhang Z, Cui R, Jia S, Wang Z, Yang C, Xu L, Dong TG, et al. Contact-independent killing mediated by a T6SS effector with intrinsic cell-entry properties. Nat Commun. 2021 Jan 18;12(1):423. doi:10.1038/s41467-020-20726-8.
- Sana TG, Flaugnatti N, Lugo KA, Lam LH, Jacobson A, Baylot V, Durand E, Journet L, Cascales E, Monack DM. Salmonella Typhimurium utilizes a T6SS-mediated antibacterial weapon to establish in the host gut. Proc Natl Acad Sci USA. 2016 Aug 23;113(34):E5044–51. doi:10.1073/pnas.1608858113.
- LaCourse KD, Peterson SB, Kulasekara HD, Radey MC, Kim J, Mougous JD. Conditional toxicity and synergy drive diversity among antibacterial effectors. Nature microbiol. 2018 Apr;3(4):440–446. doi:10.1038/s41564-018-0113-y.
- Liu L, Ye M, Li X, Li J, Deng Z, Yao Y-F, Ou H-Y. Identification and characterization of an antibacterial Type VI secretion system in the carbapenem-resistant strain Klebsiella pneumoniae HS11286. Front Cell Infect Microbiol. 2017;7:442. doi:10.3389/fcimb.2017.00442.
- Lery LM, Frangeul L, Tomas A, Passet V, Almeida AS, Bialek-Davenet S, Barbe V, Bengoechea JA, Sansonetti P, Brisse S, et al. Comparative analysis of Klebsiella pneumoniae genomes identifies a phospholipase D family protein as a novel virulence factor. BMC Biol. 2014 May 29;12(1):41. doi:10.1186/1741-7007-12-41.
- Tang MX, Pei TT, Xiang Q, Wang Z-H, Luo H, Wang X-Y, Fu Y, Dong T. Abiotic factors modulate interspecies competition mediated by the type VI secretion system effectors in Vibrio cholerae. Isme J. 2022 Jul;16(7):1765–1775. doi:10.1038/s41396-022-01228-5.
- Kostiuk B, Santoriello FJ, Diaz-Satizabal L, Bisaro F, Lee K-J, Dhody AN, Provenzano D, Unterweger D, Pukatzki S. Author Correction: Type VI secretion system mutations reduced competitive fitness of classical Vibrio cholerae biotype. Nat Commun. 2022 Feb 11;13(1):916. doi:10.1038/s41467-022-28572-6.
- Sha J, Rosenzweig JA, Kozlova EV, Wang S, Erova TE, Kirtley ML, van Lier CJ, Chopra AK. Evaluation of the roles played by Hcp and VgrG type 6 secretion system effectors in Aeromonas hydrophila SSU pathogenesis. Microbiology. 2013 Jun;159(Pt 6):1120–1135. doi:10.1099/mic.0.063495-0.
- Ho BT, Fu Y, Dong TG, Mekalanos JJ. Vibrio cholerae type 6 secretion system effector trafficking in target bacterial cells. Proc Natl Acad Sci USA. 2017 Aug 29;114(35):9427–9432. doi:10.1073/pnas.1711219114.
- Sporing I, Felgner S, Preusse M, Eckweiler D, Rohde M, Häussler S, Weiss S, Erhardt M, et al. Regulation of flagellum biosynthesis in response to cell envelope stress in Salmonella enterica Serovar Typhimurium. mBio. 2018 May 1;9 (3).10.1128/mBio.00736-17
- Palmer AD, Slauch JM, O’Toole G. Envelope stress and regulation of the Salmonella pathogenicity Island 1 Type III secretion system. J Bacteriol. 2020 Aug 10;202(17). doi:10.1128/JB.00272-20.
- Nishino K, Honda T, Yamaguchi A. Genome-wide analyses of Escherichia coli gene expression responsive to the BaeSR two-component regulatory system. J Bacteriol. 2005 Mar;187(5):1763–1772. doi:10.1128/JB.187.5.1763-1772.2005.
- Hersch SJ, Watanabe N, Stietz MS, Manera K, Kamal F, Burkinshaw B, Lam L, Pun A, Li M, Savchenko A, et al. Envelope stress responses defend against type six secretion system attacks independently of immunity proteins. Nature microbiol. 2020 May;5(5):706–714. doi:10.1038/s41564-020-0672-6.
- Logan SL, Thomas J, Yan JY, Baker RP, Shields DS, Xavier JB, Hammer BK, Parthasarathy R. The Vibrio cholerae type VI secretion system can modulate host intestinal mechanics to displace gut bacterial symbionts. Proc Natl Acad Sci USA. 2018 Apr 17;115(16):E3779–E3787. doi:10.1073/pnas.1720133115.