ABSTRACT
Bovine viral diarrhoea-mucosal disease caused by bovine viral diarrhoea virus (BVDV) is a major infectious disease that affects the cattle industry. The nonstructural protein Npro of BVDV antagonizes the type I interferon (IFN-I) pathway, thereby escaping the host immune response. The exact mechanism by which Npro uses host proteins to inhibit IFN-I production is unclear. The host protein CALCOCO2 was identified as a binding partner of Npro using a yeast two-hybrid screen. The interaction between Npro and CALCOCO2 was confirmed by yeast co-transformation, co-immunoprecipitation assays, and GST pull-down assays. The stable overexpression of CALCOCO2 markedly promoted BVDV propagation, while the knockdown of CALCOCO2 significantly inhibited BVDV replication in MDBK cells. Interestingly, CALCOCO2 inhibited IFN-I and IFN-stimulated gene production in BVDV-infected cells. Ectopic expression of CALCOCO2 effectively reduced IRF3 protein levels via the proteasome pathway. CALCOCO2 was found to promote Npro-mediated ubiquitination degradation of IRF3 by interacting with IRF3. Our results demonstrate the molecular mechanism by which Npro recruits the CALCOCO2 protein to regulate IRF3 degradation to inhibit IFN-I production.
Introduction
Type I interferon (IFN-I) is an important component of antiviral innate immune responses. Upon viral infection, pattern recognition receptors (PRRs), including Toll-like receptors, retinoic acid-inducible gene-1-like receptors, NOD-like receptors, and some intracellular nucleic acid sensors, initiate antiviral immune responses [Citation1–3]. After recognizing pathogen-associated molecular patterns produced by RNA viruses, PRRs sequentially activate RIG-I, MAVS, TBK1, IRF3/7, and NF-κB, producing IFN-I [Citation4,Citation5]. IRF3 is a transcription factor responsible for IFN-I production [Citation6]. IFN-I can induce the expression of interferon-stimulated genes (ISGs) [Citation7]. ISGs can resist viral infection by inhibiting viral adsorption and entry, degrading viral proteins and nucleic acids.
Bovine viral diarrhoea-mucosal disease (BVD-MD) is a contact infection caused by bovine viral diarrhoea virus (BVDV) infection, which leads to host immunosuppression, affects animal performance, and causes great economic losses to the livestock industry [Citation8]. BVDV is responsible for many clinical signs in infected animals, from subclinical infections to severe disease and even death. The virus is spread through contact with infected animals and contaminated feed, water, and equipment [Citation9]. BVDV is a single-stranded positive-stranded RNA virus belonging to the genus Pestivirus in the family Flaviviridae [Citation10]. The structural protein Erns, nonstructural proteins Npro, and NS4B of BVDV play important roles in suppressing the innate immune response. The BVDV Erns protein could compete with TLRs to bind viral double-stranded RNA, interfering with the TLR-mediated innate immune response to inhibit IFN-β production [Citation11]. NS4B interacts with the CARD domains of MDA5 to negatively regulate the RLR-mediated antiviral response [Citation12]. Npro could interact with IRF3 and induce ubiquitination of IRF3 for its degradation via the proteasomal pathway [Citation13,Citation14]. Npro negatively regulates IFN-β expression by decreasing the activity of the S100A9 protein [Citation15]. However, the specific mechanism by which Npro uses host proteins to inhibit IFN-β production still lacks in-depth study.
CALCOCO2, also known as NDP52, is a member of the nuclear dot protein family that plays an important role in mitochondrial autophagy and allogeneic autophagy interacts with LC3, thereby anchoring the substrate to the autophagosome membrane and subsequently attaching the autophagosome to myosin VI to facilitate the fusion of autophagosomes with lysosomes [Citation16,Citation17]. Previous studies have identified the involvement of CALCOCO2 in the replication of various viruses. For example, CALCOCO2 could interact with the porcine epidemic diarrhoea virus (PEDV) nucleocapsid protein after ubiquitination by the E3 ubiquitin ligase MARCHF8 to influence the viral life cycle [Citation18]. CALCOCO2 could interact with the NSP2 protein of the chikungunya virus (CHIKV) to influence NSP2-induced apoptosis and nuclear localization [Citation19]. The expression of IFN-α and TNF-α was higher in CALCOCO2-silenced PK15 cells induced by classical swine fever virus (CSFV) than in control cells, thereby inhibiting CSFV replication [Citation20].
The present study found that BVDV Npro could interact with the host protein CALCOCO2 by screening the yeast library. Importantly, overexpression of CALCOCO2 significantly increased the titre of BVDV, while silencing of CALCOCO2 inhibited viral replication. CALCOCO2 suppressed the expression of IFN-β and ISGs. Further studies revealed an interaction between CALCOCO2 and IRF3 and that CALCOCO2 enhanced Npro-mediated ubiquitination levels of IRF3, which led to the degradation of IRF3. Identifying CALCOCO2 as a host factor that interacts with BVDV Npro and regulates the host’s antiviral response provides a new avenue for understanding host-virus interactions and could lead to the development of new strategies for controlling BVDV infections.
Materials and methods
Cell lines
Madin-Darby bovine kidney (MDBK) cells kept in our research group as BVDV-free were grown in DMEM supplemented with 10% horse serum (16050122, Lot: 2284918, New Zealand origin, Gibco), 1% penicillin, and streptomycin. CALCOCO2 overexpression or knockdown MDBK cell lines were constructed according to the previously described methods [Citation21]. Briefly, the CALCOCO2 CDS of bovine was inserted into the pLVX-IRES-puro vector to construct MDBK cell lines stably expressing CALCOCO2. The primer sequences for CALCOCO2 amplification are listed in . Small interfering RNA sequences targeting the CALCOCO2 gene (1: 5”-GCGAGTACTACACCTTCATGT-3,‘ 2: 5’-GCAGACCCTTAGTTTGAATTG-3”) were inserted into the pYr-Lvsh vector to construct CALCOCO2 knockdown cell lines. The generation of lentiviral particles was performed in HEK293T cells. The plasmids pLVX-CALCOCO2 or pYr-Lvsh-CALCOCO2 were co-transfected into HEK293T cells with the plasmids of pLP1, pLP2, and pLP/VSVG using the polyfast transfection reagent (HY-K1014, MedChemExpress, Monmouth Junction, NJ, USA) according to the manufacturer’s protocol. The virus liquid within 72 h after transfection was collected and used to infect the MDBK cells. The stably-transfected MDBK cells were selected with puromycin (5 μg/ml). CALCOCO2 overexpression and knockdown cells were identified by RT-qPCR and immunoblotting with an anti-CALCOCO2 mouse monoclonal antibody (ab124372, Abcam).
Table 1. List of primers used in this study.
Virus
The BVDV NADL strain, which belongs to the cytopathic biotype of BVDV genotype 1a, was provided by the China Veterinary Culture Collection Center. MDBK cells grown to 80% confluence in 96-well microplates were washed three times with cold PBS. The collected BVDV virus liquid was serially diluted 10-fold (10−1 to 10−9) and inoculated into MDBK cells, and each dilution factor was set to 8 replicate wells. Cells were incubated at 37°C in 5% CO2 for 2 h and then cultured in DMEM containing 2% horse serum. The cytopathic effect was observed under a microscope, and the TCID50 was calculated by the Reed-Muench method.
Viral infection
To determine the effects of CALCOCO2 on viral replication, CALCOCO2 overexpression and knockdown MDBK cells were infected with BVDV (MOI = 0.1 or 1) for 48 h. The cells were collected to detect Npro mRNA levels and viral titres. To determine the effects of CALCOCO2 on the mRNA levels of IFN-β and ISGs, CALCOCO2-overexpressing and knockdown cells were infected with BVDV (MOI = 0.1 or 5) for 24 h. To determine the effects of CALCOCO2 on IRF3 protein expression, CALCOCO2 overexpression MDBK or control cells pretreated with the proteasome inhibitor MG132 (0.5 μM) (HY-13259, MedChemExpress) for 4 h followed by infection with BVDV (MOI = 5) for 24 h were used to detect the protein level of IRF3. The cells were collected for western blotting analysis.
Yeast two-hybrid assay
Yeast two-hybrid constructs were built using the Clontech Matchmaker Gold Yeast Two-Hybrid System (TaKaRa). Yeast two-hybrid analysis was performed as described [Citation22]. The plasmids pGADT7 (AD), pGBKT7 (BD), pGBKT7-p53 (BD-P53), pGADT7-T (AD-T) and pGBKT7-Lambda (BD-Lam) were gifts from Dr. Wei Pan (Shandong Normal University). The bovine CALCOCO2 gene was amplified using the primers 5’-gccatggaggccagtgaattcATGGAAGAGACAGTAGACGATCCC-3” (forward) and 5”-cagctcgagctcgatggatccTTAGAGAGTGTGGCAGAACACATGG-3’ (reverse), which contained EcoR I and BamH I restriction sites (underlined), and cloned into the pGADT7 vector to generate the pGADT7-CALCOCO2 (AD-CLCOCO2) plasmid by using a ClonExpress II One Step Cloning Kit (C112, Vazyme). Similarly, the Npro of the BVDV gene was inserted into the pGBKT7 vector to generate the pGBKT7-Npro (BD-Npro) by using the ClonExpress II One Step Cloning Kit, and the primers were designated as EcoR I and BamH I restriction sites, respectively (the forward primer atggccatggaggccgaattcATGGAGTTGATCACAAATGAACTTTT and the reverse primer ccgctgcaggtcgacggatccGCAACTTGTGACCCATAGAGGG). DNA sequencing was used to verify successful plasmid construction, and these plasmids were transformed into the yeast strain AH109 and grown in a defined medium lacking leucine and tryptophan (SD/-Leu/-Trp). Selected colonies were transferred to quadruple dropout supplement plates (SD/-Ade/-His/-Leu/-Trp) and incubated at 30°C. The AH109 strain co-transformed with BD-P53 and AD-T served as a positive control, and the yeast strain co-transformed with Lam/T and BD/AD was used as the negative control.
GST pull-down
The sequences encoding Npro were cloned and inserted into the pGEX4T–1 and pCMV-HA-C vectors, respectively. The sequences encoding CALCOCO2 were cloned and inserted into the pGEX4T–1, pCMV-Flag-N, or pEGFP-N1 vector. E. coli BL21 (DE3) was transformed into the plasmids pGEX4T-CALCOCO2, pGEX4T-Npro, or pGEX4T–1. GST fusion protein expression was stimulated by adding 0.8 mM isopropyl 1-β-D-thioglycoside to the bacterial medium at 16°C. After incubation of the soluble GST-CALCOCO2 or GST-Npro protein with glutathione beads (70601–5, Beaver Biotechnology) on a rotating incubator at 4°C for 3 h, the beads were washed three times. HEK293T cells were transfected with pCMV-HA-Npro or pCMV-Flag-CALCOCO2 plasmids encoding HA-tagged Npro or Flag-tagged CALCOCO2 protein using the poly-fast transfection reagent (HY-K1014, MedChemExpress). The glutathione beads were incubated overnight with HA-Npro or Flag-CALCOCO2 protein expression in HEK293T cells. The targeted proteins were separated with 10% SDS and identified using western blotting and an anti-HA mAb (3724, CST) or anti-Flag mAb (14793, CST).
RNA extraction
MDBK cells were infected with BVDV for 24 h, washed with sterilized PBS and lysed in TRIzol for 5 minutes. After the end of lysis, 200 μl of chloroform was added, vigorously shaken for 60 s, and centrifuged at 13,000 × g for 10 min. The upper water sample was aspirated, 800 μl of isopropanol was added, mixed upside down, allowed to stand for 15 min, and centrifuged at 13,000 × g for 15 min. After discarding the liquid, 85% ethanol was added, and the sample was centrifuged at 13,000 × g for 3 min. The supernatant was discarded, and 20 μl of DEPC water was added. After the concentration was determined, aliquots were frozen at −80°C.
RT-qPCR
cDNA was prepared from the extracted RNA according to the instructions for HiScript II Q RT SuperMix (R223–01, Vazyme). Primers were designed using GenBank reference sequences and the primer design tool Primer Express 3.0.1 software. Specific primers for qPCR were verified by the NCBI primer-BLAST web tool and melt curve analysis [Citation23,Citation24]. GAPDH was used as an endogenous reference gene. The qPCR primers synthesized by Tsingke Biotechnology Company are shown in . Perform the qPCR test according to SYBR Green’s instructions.
Western blot
Cells were washed with sterilized PBS, lysed with 200 μl RIPA (PC101, EpiZyme Biotechnology) for 40 min, and centrifuged at 13,000 × g for 5 min, and the supernatant was collected. Protein concentrations were quantified by a bicinchoninic acid (BCA) protein assay (BCA Protein Assay Kit 23,225, ThermoScientific). After determining the protein concentration, 50 μl of 5× loading buffer was added and placed in boiling water for 10 min. A 25 μg protein sample was subjected to SDS‒PAGE electrophoresis, after which the protein was transferred to a 0.22 μm PVDF membrane and blocked with skim milk powder for 2 h, and the primary antibody was incubated at 4°C for 6 h. After the primary antibody incubation, the secondary antibody was incubated for 45 min after washing five times with TBST. After incubation, the cells were washed three times with TBST and exposed to the following antibodies: anti-Flag (14793, CST), anti-CALCOCO2 (ab124372, Abcam), anti-GFP (AB0047, Abways Technology), anti-GAPDH (AB0036, Abways Technology), anti-GST (2625, CST), anti-HA (3724, CST), anti-Flag (14793, CST), anti-IRF3 (4302, CST) and anti-β-actin (P68133, Abways Technology).
Co-immunoprecipitation assay
The pCMV-Flag-CALCOCO2 and pCMV-HA-Npro plasmids were co-transfected into HEK293T cells for 48 h. Cells were lysed on ice for 1 h in lysis buffer (protease inhibitor cocktail, 1 mM EDTA, 25 mM Tris-HCl pH 7.4, 1% Triton X-100, 150 mM NaCl, 1 mM PMSF). The lysate was then centrifuged at 13,000 × g and 4°C for 10 min. Subsequently, magnetic beads coupled with anti-Flag or HA tag mAb were incubated with the supernatants at 4°C for 4 h, followed by magnetic separation and washing with lysis buffer. Proteins bound to the beads were eluted from the beads in 1×SDS‒PAGE buffer, boiled for 10 min, and then subjected to immunoblot analysis.
Statistical analysis
All data are presented as the mean ± standard deviation and were analysed using GraphPad Prism software (version 8.0). Differences between two groups of normally distributed variables were analysed using Student’s t test, while differences among three groups were evaluated with one-way ANOVA and subsequent Tukey’s post hoc test (*, P < 0.05, **, P < 0.01, ns indicates nonsignificant).
Results
Identification of CALCOCO2 as a novel BVDV Npro protein-interacting protein
To search for host cellular proteins that interact with the BVDV Npro protein, the yeast two-hybrid system was used to screen a cDNA library generated from the MDBK cells, and the full-length BVDV Npro was used as bait. The positive clones screened from yeast transformants were isolated, sequenced, and aligned with references in the NCBI database. One specific clone from this screen contained a partial open reading frame for CALCOCO2 (GenBank accession no. NM_174400.2). To exclude the self-activation of CALCOCO2, a yeast cotransformation assay was performed in AH109 yeast, with a combination of SV40 large T antigen (AD-SV40-T) and murine p53 (BD-p53) fusion constructs as a positive control. As shown in , only AD-CALCOCO2/BD-Npro and the positive control could grow on SD/-4 medium, indicating that CALCOCO2 might be a potential binding partner for BVDV Npro.
Figure 1. Npro interacts with CALCOCO2. (a) the Npro-CALCOCO2 interaction was detected using the yeast two-hybrid system. The yeast strain co-transformed with BD-P53 and AD-T (P53/T) served as a positive control. BD-Npro/AD (Npro/AD) and BD/AD-CALCOCO2 (BD/CALCOCO2) were used to exclude self-activation. Cotransformation of Lam/T and BD/AD was used as a negative control. All yeasts co-transformed with the described constructs were screened on SD/-2 (SD/-Leu/-Trp) and SD/-4 (SD/-ade/-his/-Leu/-Trp) plates. (b, c) GST pull-down assay of CALCOCO2 and Npro. Purified GST, GST-Npro, or GST-CALCOCO2 proteins were bound to magnetic beads. The beads were then incubated with recombinant Flag or HA-tagged proteins expressed in HEK293T cells. The bound proteins were washed completely and subjected to western blotting using a rabbit anti-Flag mAb, a rabbit anti-HA mAb, or a rabbit anti-GST pAb. (d) HEK293T cells were co-transfected with HA-Npro and Flag-CALCOCO2 or Flag-EV. At 48 h post-transfection, HA-Npro immunoprecipitation was performed using the anti-Flag-tag mAb-magnetic beads, followed by immunoblot analysis with anti-Flag and anti-HA antibodies.
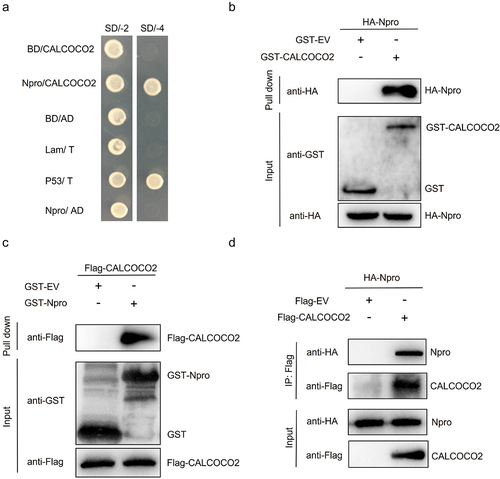
A GST pull-down assay was performed to further verify the CALCOCO2/Npro interaction. The GST-CALCOCO2 fusion protein was generated in E. coli BL21, and the HA-Npro protein was expressed in HEK293T cells. The results showed that GST-CALCOCO2 could pull down HA-Npro but not by GST alone (). Similarly, Flag-CALCOCO2 was only abundantly pulled down by GST-Npro (). The interaction between CALCOCO2 and Npro was also confirmed by co-IP experiments. When HA-Npro and Flag-CALCOCO2 were coexpressed in HEK293T cells, HA-Npro was coimmunoprecipitated with Flag-CALCOCO2 (), suggesting that CALCOCO2 interacts with Npro in mammalian cells. These data indicated that CALCOCO2 was an interacting partner of the BVDV Npro protein.
CALCOCO2 promotes BVDV proliferation
To investigate whether CALCOCO2 was involved in the replication of BVDV, MDBK cells were infected with recombinant retrovirus encoding CALCOCO2 to establish the CALCOCO2-overexpressing stable cell lines. RT-qPCR and western blotting results showed that CALCOCO2 expression at both the mRNA and protein levels was increased in CALCOCO2-overexpressing cell lines compared with the negative control cell lines, indicating that CALCOCO2 overexpression cell lines were successfully constructed (). Next, the cell lines were infected with BVDV (MOI = 0.1 or 1), and BVDV Npro mRNA and virus titres in CALCOCO2-overexpressing cells were significantly higher than those in control cells at 48 h post-infection (), indicating that overexpression of CALCOCO2 promoted viral replication in MDBK cells. To verify the effect of CALCOCO2 knockdown on BVDV replication, stable CALCOCO2 gene silencing in MDBK cells was established by lentivirus-delivered shRNAs. The knockdown efficiency of shRNAs on CALCOCO2 was determined by RT-qPCR and western blotting (). CCK8 assay analysis revealed that the viability of MDBK cells was not affected by CALCOCO2 overexpression or silencing (data not shown). As shown in , knockdown of CALCOCO2 significantly reduced the proliferation of BVDV, as determined by measuring viral titres and Npro mRNA levels. Overall, these data demonstrated that CALCOCO2 promoted BVDV progeny production.
Figure 2. CALCOCO2 promotes BVDV replication. (a) immunoblot analysis of CALCOCO2 protein expression in the MDBK cell line stably overexpressing CALCOCO2 using anti-CALCOCO2 and anti-GAPDH antibodies. (b) the mRNA levels of CALCOCO2 were analyzed through qPCR in cells that had stable overexpression of CALCOCO2. (c, d) CALCOCO2-overexpressing cell lines were infected with BVDV (MOI = 0.1 or 1) and harvested at 48 h post-infection. (c) the mRNA levels of BVDV Npro were analyzed through qPCR in CALCOCO2-overexpressing cells. (d) the TCID50 assay was used to determine the virus titers of BVDV in the cell cultures. (e) CALCOCO2 protein levels were analyzed through immunoblotting in stable CALCOCO2-knockdown cell lines. (f) the knockdown efficiencies of CALCOCO2-silenced MDBK cells were examined by immunoblot analysis. (g) the mRNA levels of the Npro gene were analyzed through qPCR in stable CALCOCO2-knockdown cell lines at 48 h post-infection. (h) the viral titers of BVDV-infected CALCOCO2-knockdown or control cells were determined by TCID50 at 48 h post-infection. The means and standard deviation from three independent experiments are shown. P values were calculated by Student’s t test in B,C and D and by one-way ANOVA in F, G and H: *, P < 0.05; **, P < 0.01.
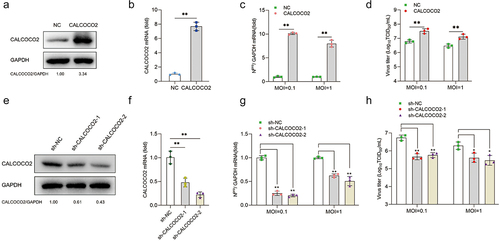
CALCOCO2 suppresses IFN-β and ISG expression during BVDV infection
Previous studies have shown the critical role of IFN-I in resistance to BVDV replication [Citation25,Citation26]. The effect of CALCOCO2 on IFN-β and ISG production in CALCOCO2-overexpressing stable cell lines during BVDV infection (MOI = 0.1 or 5) was first examined. The results revealed that CALCOCO2 overexpression significantly suppressed the expression of IFN-β, OAS1, ISG15, and M×1at the mRNA level (). To further confirm the impact of CALCOCO2 in regulating the production of IFN-β and ISGs, the transcript levels of these genes were examined by RT-qPCR in CALCOCO2 knockdown cells infected with BVDV. The results indicated that CALCOCO2 silencing could promote IFN-β, OAS1, ISG15, and M×1mRNA levels during BVDV infection (). These results showed that CALCOCO2 could suppress the IFN-I-mediated antiviral innate immune response in MDBK cells infected with BVDV.
Figure 3. CALCOCO2 restrains the expression of IFN-β and ISGs during BVDV infection. (a, b) a and b represent the qPCR analysis of IFN-β, MX1, ISG15, and OAS1 mRNA levels in CALCOCO2-overexpressing cells infected with BVDV (MOI = 0.1 or 5). c and d show the IFN-β, OAS1, MX1, and ISG15 mRNA levels in CALCOCO2 knockdown cells and control cells at 24 h after BVDV infection (MOI = 0.1 or 5). The relative mRNA levels of IFN-β and ISGs were normalized to GAPDH. The mean and SD values were determined from three independent experiments. P values were calculated by Student’s t test in a and B and by one-way ANOVA in c and d: **, P < 0.01; and ns indicates insignificant.
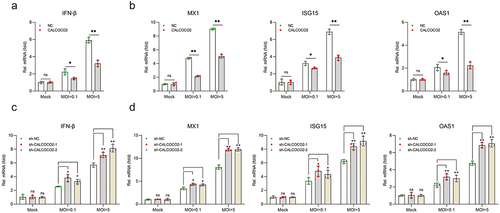
CALCOCO2 promotes the degradation of IRF3 via the ubiquitin-proteasome system
Given evidence showing that BVDV Npro antagonizes IFN-β production by facilitating the ubiquitination and degradation of IRF3 [Citation27], we focused on detecting the effect of CALCOCO2 interacting with Npro on IRF3 expression. Subsequently, we detected the protein expression of IRF3 after overexpression of CALCOCO2 in MDBK cells infected with BVDV. The images in clearly show that the protein levels of IRF3 in CALCOCO2-overexpressing cell lines were lower than those in control cell lines. Next, we determined whether CALCOCO2 overexpression could negatively regulate IRF3 transcription. The results showed that CALCOCO2 did not inhibit IRF3 transcription in MDBK cells (). To investigate whether CALCOCO2 facilitates the degradation of IRF3 via the ubiquitin-proteasome pathway, we subjected CALCOCO2-overexpressing cells to treatment with MG132 and subsequently assessed the protein expression levels of IRF3. The results indicated that the effect of CALCOCO2 overexpression on IRF3 degradation could be reversed using the proteasome inhibitor MG132 (). These results suggested that CALCOCO2 promoted the degradation of IRF3 through the ubiquitin-proteasome system.
Figure 4. CALCOCO2 promotes the degradation of IRF3. (a) the protein levels of IRF3 in CALCOCO2-overexpressing cells infected with BVDV were detected at 24 and 48 hpi. (b) at 24 and 48 h post-infection, the mRNA levels of IRF3 were analysed through qPCR in both CALCOCO2-overexpressing MDBK cells and control cells. GAPDH mRNA levels served as an internal reference control. Data are shown as the mean ± SD (ns, not significant). (c). Immunoblot analysis of IRF3 protein levels in CALCOCO2-overexpressing and control cells treated with the proteasome inhibitor MG132 (0.5 μM) or DMSO for 4 h followed by infection with BVDV (MOI = 5) for 24 h.
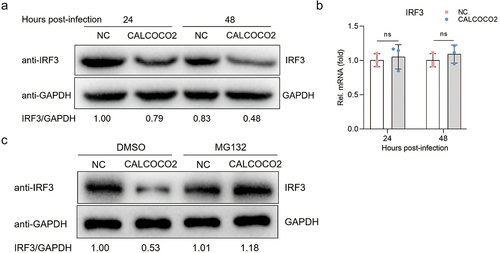
CALCOCO2 interacts with IRF3 and promotes Npro-mediated ubiquitination of IRF3
To determine whether CALCOCO2 regulates IRF3 degradation by facilitating Npro-induced IRF3 ubiquitination, HEK293T cells were co-transfected with HA-Npro, Flag-IRF3, and GFP-CALCOCO2 to detect the ubiquitination of IRF3. The higher level of IRF3 ubiquitination in cells transfected with CALCOCO2 and the lower expression of IRF3 in cell lysates also suggested that CALCOCO2 promoted the ubiquitination and degradation of IRF3 (). Moreover, we found that CALCOCO2 appeared to promote the interaction between Npro and IRF3 (). We speculated that CALCOCO2 might interact with IRF3, thereby enhancing the interaction between Npro and IRF3. Therefore, we constructed Flag-IRF3 expression vectors for co-IP experiments to verify whether IRF3 interacts with CALCOCO2. IRF3 was found to co-immuno-precipitate with CALCOCO2 when immunoprecipitates were detected using anti-Flag or anti-HA antibodies (). These data indicated that CALCOCO2 could interact with IRF3 and Npro, respectively, thereby promoting Npro-induced IRF3 ubiquitination and, ultimately, IRF3 degradation.
Figure 5. CALCOCO2 promotes Npro-mediated ubiquitination of IRF3 by interacting with IRF3. (a) HEK293T cells were co-transfected with HA-Npro, Flag-IRF3, Ub-myc, GFP-CALCOCO2, or GFP-EV and treated with 0.50 μM MG132. Anti-Flag-tag mAb-magnetic beads were used to perform IP on the cell lysates, which were subsequently analysed through immunoblotting using anti-Flag, anti-HA, anti-GFP, and anti-Ub antibodies. (b) HEK293T cells were co-transfected with Flag-IRF3 and HA-CALCOCO2 or HA-EV, followed by co-IP and immunoblot analysis of the interactions of Flag-IRF3 with HA-CALCOCO2 using the anti-HA-tag mAb-magnetic beads, anti-Flag, and anti-HA antibodies. (c) co-IP and immunoblot analysis using anti-Flag-tag mAb-magnetic beads on extracts of HEK293T cells transfected with HA-CALCOCO2 and Flag-IRF3 or Flag-EV.
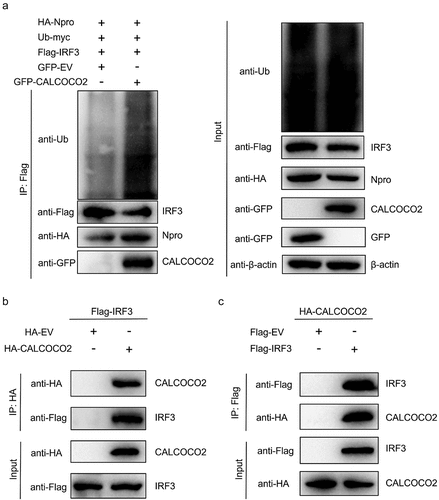
Discussion
Innate immune responses are the first line of host defence against viral infection, and IFN-I plays a crucial role in antiviral innate immunity [Citation28]. IFN-I, which mainly includes IFN-α and IFN-β, induces ISGs production by activating the JAK/STAT signalling pathway [Citation29]. ISGs are important in limiting viral replication and transmission [Citation30]. Many viruses have evolved strategies to inhibit the production of IFN-I from evading the innate immune response. BVDV is an RNA virus that causes serious economic losses in the cattle industry. The BVDV nonstructural protein Npro inhibits the production of IFN-I and enhances viral replication ability [Citation31,Citation32].
It has been reported that BVDV potentially induces immunosuppression by interacting with the BVDV Npro protein with the host protein S100A9 [Citation15]. Nevertheless, their study did not address the specific regulatory mechanisms of the interaction between Npro and S100A9 on IFN production. More recently, it has been reported that the atypical porcine pestivirus (APPV) Npro could inhibit the transmission of the IFN-I pathway by affecting the phosphorylation of IRF3 through interaction with IRF3 [Citation33]. CSFV Npro inhibits the IFN pathway and suppresses dsRNA-mediated mitochondrial apoptosis [Citation34]. The Npro of these viruses plays an important role in the viral infection process through cooperation with host proteins. However, it is still unclear which host proteins BVDV Npro can cooperate with to inhibit the IFN-I pathway. Thus, the yeast two-hybrid assay was performed using BVDV Npro as bait.
The autophagy receptor protein CALCOCO2 was identified as a candidate target protein for Npro. The interaction of CALCOCO2 and Npro was further verified by GST pull-down and co-IP assays (). One limitation of the study is that we did not perform immunoprecipitation assays to detect the interaction between Npro and CALCOCO2 in the lysates of MDBK cells infected with BVDV, because we were unable to obtain commercially available specific antibodies against the BVDV Npro protein, and no research group has successfully prepared this antibody. This study used yeast two-hybrid, GST pull-down, and co-IP experiments to verify the interaction between Npro and CALCOCO2. These results can also support existing conclusions.
Previous research has reported that CALCOCO2 plays an important role in viral replication. The PEDV nucleocapsid protein is ubiquitinated by the E3 ubiquitin ligase, recognized by CALCOCO2 and transported to the lysosome for degradation [Citation18]. More recently, it has been reported that the ubiquitinated nucleocapsid protein of SARS-CoV-2, SARS-CoV, and MERS is degraded after being bound by CALCOCO2 [Citation35]. The interaction of CALCOCO2 with the influenza A virus PB1-F2 protein inhibits TBK1-mediated ISRE activity but promotes the NF-κB signalling pathway [Citation36]. To investigate the relationship between CALCOCO2 and BVDV replication, we examined the BVDV mRNA levels and viral titres in CALCOCO2-overexpressing or CALCOCO2 knockdown stable cell lines and found that CALCOCO2 could promote BVDV replication in MDBK cells (), which is the first report on CALCOCO2 facilitating BVDV progeny production.
It has been reported that CALCOCO2 promotes MAVS degradation through selective autophagy to inhibit IFN-I production, thereby promoting viral replication during CVB3 infection [Citation37]. Therefore, we investigated the innate antiviral response of CALCOCO2-overexpressing or CALCOCO2-silenced stable MDBK cells infected with BVDV. The results show that CALCOCO2 suppressed IFN-β, MX1, ISG15, and OAS1 mRNA levels in MDBK cells (). Considering the degradation effect of BVDV Npro on IRF3, the effect of CALCOCO2, which interacts with Npro, on IRF3 protein and mRNA expression was investigated. IRF3 is the most important adapter protein downstream of the RIG-I signalling pathway, so we focused on the effect of CALCOCO2 on IRF3 expression. Our data revealed that CALCOCO2 could promote IRF3 degradation at the protein level but not at the mRNA level during BVDV infection. Subsequently, we found that rescue of IRF3 protein degradation occurred in CALCOCO2-overexpressing cells treated with MG132, indicating that CALCOCO2 promotes IRF3 degradation via the proteasomal pathway (). Then, the effect of CALCOCO2 on Npro-mediated ubiquitination of IRF3 was examined. This analysis shows that CALCOCO2 significantly promoted the ubiquitination of IRF3 and facilitated the interaction between Npro and IRF3 (). Moreover, co-IP assays showed that IRF3 and CALCOCO2 also interacted with each other. Hence, we speculate that CALCOCO2 promotes the ubiquitinated degradation of IRF3 by interacting with IRF3 and Npro, forming a complex that increases the frequency of interaction between Npro and IRF3.
In this study, we demonstrated that the host protein CALCOCO2 interacts with the BVDV nonstructural protein Npro and plays a key role in regulating IRF3 protein levels. Our findings provide novel insights into the mechanisms by which BVDV evades innate antiviral responses, highlighting the importance of the host protein CALCOCO2. Identifying the interaction between CALCOCO2 and BVDV Npro may open new possibilities for developing antiviral therapies targeting this host protein.
Author contributions
W.S, L.FX, W.R, G.J, and M.XM performed most of the experiments. W.R, W.J, and M.Z participated in the discussion. H.HB. and W.HM supervised the work and revised the final version of the manuscript. All the authors have read and approved the final manuscript.
Disclosure statement
The authors declare that the research was conducted without any commercial or financial relationships that could be construed as a potential conflict of interest.
Data Availability statement
The data supporting this study’s findings are available from the corresponding author.
Additional information
Funding
References
- Wu J, Chen ZJ. Innate immune sensing and signaling of cytosolic nucleic acids. Annu Rev Immunol. 2014;32(1):461–11. doi: 10.1146/annurev-immunol-032713-120156
- Cai C, Tang YD, Xu G, et al. The crosstalk between viral RNA- and DNA-sensing mechanisms. Cell Mol Life Sci. 2021;78(23):7427–7434. doi: 10.1007/s00018-021-04001-7
- Kong F, You H, Zheng K, et al. The crosstalk between pattern-recognition receptor signaling and calcium signaling. Int j biol macromol. 2021;192:745–756. doi: 10.1016/j.ijbiomac.2021.10.014
- Loo YM, Gale M Jr. Immune signaling by RIG-I-like receptors. Immunity. 2011;34(5):680–692. doi: 10.1016/j.immuni.2011.05.003
- Su C, Tang YD, Zheng C. DExD/H-box helicases: multifunctional regulators in antiviral innate immunity. Cell Mol Life Sci. 2021;79(1):2. doi: 10.1007/s00018-021-04072-6
- Taylor KE, Mossman KL. Recent advances in understanding viral evasion of type I interferon. Immunology. 2013;138(3):190–197. doi: 10.1111/imm.12038
- Santhakumar D, Rubbenstroth D, Martinez-Sobrido L, et al. Avian interferons and their antiviral effectors. Front Immunol. 2017;8:49. doi: 10.3389/fimmu.2017.00049
- Givens MD, Newcomer BW. Perspective on BVDV control programs. Anim Health Res Rev. 2015;16(1):78–82. doi: 10.1017/S1466252315000043
- Oguejiofor CF, Thomas C, Cheng Z, et al. Mechanisms linking bovine viral diarrhea virus (BVDV) infection with infertility in cattle. Anim Health Res Rev. 2019;20(1):72–85. doi: 10.1017/S1466252319000057
- Simmonds P, Becher P, Bukh J, et al. ICTV virus taxonomy profile: flaviviridae. J Gen Virol. 2017;98(1):2–3. doi: 10.1099/jgv.0.000672
- Zurcher C, Sauter KS, Schweizer M. Pestiviral E(rns) blocks TLR-3-dependent IFN synthesis by LL37 complexed RNA. Vet Microbiol. 2014;174(3–4):399–408. doi: 10.1016/j.vetmic.2014.09.028
- Shan Y, Tong Z, Jinzhu M, et al. Bovine viral diarrhea virus NS4B protein interacts with 2CARD of MDA5 domain and negatively regulates the RLR-mediated IFN-beta production. Virus Res. 2021;302:198471. doi: 10.1016/j.virusres.2021.198471
- Hilton L, Moganeradj K, Zhang G, et al. The NPro product of bovine viral diarrhea virus inhibits DNA binding by interferon regulatory factor 3 and targets it for proteasomal degradation. J Virol. 2006;80(23):11723–11732. doi: 10.1128/JVI.01145-06
- Chen Z, Rijnbrand R, Jangra RK, et al. Ubiquitination and proteasomal degradation of interferon regulatory factor-3 induced by Npro from a cytopathic bovine viral diarrhea virus. Virology. 2007;366(2):277–292. doi: 10.1016/j.virol.2007.04.023
- Darweesh MF, Rajput MKS, Braun LJ, et al. BVDV Npro protein mediates the BVDV induced immunosuppression through interaction with cellular S100A9 protein. Microb Pathog. 2018;121:341–349. doi: 10.1016/j.micpath.2018.05.047
- Viret C, Rozieres A, Faure M. Novel insights into NDP52 autophagy receptor functioning. Trends Cell Biol. 2018;28(4):255–257. doi: 10.1016/j.tcb.2018.01.003
- Di Rita A, Angelini DF, Maiorino T, et al. Characterization of a natural variant of human NDP52 and its functional consequences on mitophagy. Cell Death Differ. 2021;28:2499–2516. doi: 10.1038/s41418-021-00766-3
- Kong N, Shan T, Wang H, et al. BST2 suppresses porcine epidemic diarrhea virus replication by targeting and degrading virus nucleocapsid protein with selective autophagy. Autophagy. 2020;16(10):1737–1752. doi: 10.1080/15548627.2019.1707487
- Judith D, Mostowy S, Bourai M, et al. Species-specific impact of the autophagy machinery on Chikungunya virus infection. EMBO Rep. 2013;14(6):534–44. doi: 10.1038/embor.2013.51
- Fan S, Wu K, Luo C, et al. Dual NDP52 function in persistent CSFV infection. Front Microbiol. 2019;10:2962. doi: 10.3389/fmicb.2019.02962
- Wang S, Ma X, Guo J, et al. DDIT3 antagonizes innate immune response to promote bovine alphaherpesvirus 1 replication via the DDIT3-SQSTM1-STING pathway. Virulence. 2022;13(1):514–529. doi: 10.1080/21505594.2022.2044667
- Pan W, Song D, He W, et al. EIF3i affects vesicular stomatitis virus growth by interacting with matrix protein. Vet Microbiol. 2017;212:59–66. doi: 10.1016/j.vetmic.2017.10.021
- Bustin S, Huggett J. qPCR primer design revisited. Biomol Detect Quantif. 2017;14:19–28. doi: 10.1016/j.bdq.2017.11.001
- Thornton B, Basu C. Real-time PCR (qPCR) primer design using free online software. Biochem Mol Biol Educ. 2011;39(2):145–154. doi: 10.1002/bmb.20461
- Wang S, Hou P, Pan W, et al. DDIT3 targets innate immunity via the DDIT3-OTUD1-MAVS pathway to promote bovine viral diarrhea virus replication. J Virol. 2021;95(6). doi: 10.1128/JVI.02351-20
- Song Q, Zhao X, Cao C, et al. Research advances on interferon (IFN) response during BVDV infection. Res Vet Sci. 2022;149:151–158. doi: 10.1016/j.rvsc.2022.04.011
- Jefferson M, Whelband M, Mohorianu I, et al. The pestivirus N terminal protease N(pro) redistributes to mitochondria and peroxisomes suggesting new sites for regulation of IRF3 by N(pro.). PLoS One. 2014;9(2):e88838. doi: 10.1371/journal.pone.0088838
- Levy DE, Garcia-Sastre A. The virus battles: IFN induction of the antiviral state and mechanisms of viral evasion. Cytokine Growth Factor Rev. 2001;12(2–3):143–56. doi: 10.1016/S1359-6101(00)00027-7
- Shuai K, Liu B. Regulation of JAK–STAT signalling in the immune system. Nat Rev Immunol. 2003;3(11):900–11. doi: 10.1038/nri1226
- McDougal MB, Boys IN, De La Cruz-Rivera P, et al. Evolution of the interferon response: lessons from ISGs of diverse mammals. Curr Opin Virol. 2022;53:101202. doi: 10.1016/j.coviro.2022.101202
- Kozasa T, Abe Y, Mitsuhashi K, et al. Analysis of a pair of END+ and END− viruses derived from the same bovine viral diarrhea virus stock reveals the amino acid determinants in Npro responsible for inhibition of type I interferon production. J Vet Med Sci. 2015;77(5):511–8. doi: 10.1292/jvms.14-0420
- Chi S, Chen S, Jia W, et al. Non-structural proteins of bovine viral diarrhea virus. Vir Gen. 2022;58(6):491–500. doi: 10.1007/s11262-022-01914-8
- Mou C, Pan S, Wu H, et al. Disruption of interferon-β production by the N pro of atypical porcine pestivirus. Virulence. 2021;12(1):654–665. doi: 10.1080/21505594.2021.1880773
- Hardy S, Jackson B, Goodbourn S, et al. Classical swine fever virus N pro antagonizes IRF3 to prevent interferon-independent TLR3- and RIG-I-Mediated apoptosis. J Virol. 2020;95(5). doi: 10.1128/JVI.01136-20
- Jiao Y, Kong N, Wang H, et al. PABPC4 broadly inhibits coronavirus replication by degrading nucleocapsid protein through selective autophagy. Microbiol Spectr. 2021;9(2):e0090821. doi: 10.1128/Spectrum.00908-21
- Leymarie O, Meyer L, Tafforeau L, et al. Influenza virus protein PB1-F2 interacts with CALCOCO2 (NDP52) to modulate innate immune response. J Gen Virol. 2017;98(6):1196–1208. doi: 10.1099/jgv.0.000782
- Mohamud Y, Qu J, Xue YC, et al. CALCOCO2/NDP52 and SQSTM1/p62 differentially regulate coxsackievirus B3 propagation. Cell Death Differ. 2019;26(6):1062–1076. doi: 10.1038/s41418-018-0185-5