ABSTRACT
Moraxella catarrhalis is a major cause of chronic obstructive pulmonary disease. Toll-like receptor 2 (TLR2) plays an important role in the inflammatory response in host respiratory epithelial cells. M. catarrhalis induces an inflammatory immune response in respiratory epithelial cells that is mostly dependent on TLR2. However, the mechanisms by which this pathogen adheres to and invades the respiratory epithelium are not well understood. The present study aimed to reveal the role of TLR2 in M. catarrhalis adhesion to and invasion into alveolar epithelial cells, using molecular techniques. Pretreatment with the TLR2 inhibitor TLR2-IN-C29 enhanced M. catarrhalis adhesion to A549 cells but reduced its invasion, whereas the agonist Pam3CSK4 reduced both M. catarrhalis adhesion and invasion into A549 cells. Similarly, M. catarrhalis 73-OR strain adhesion and invasion were significantly reduced in TLR2−/− A549 cells. Moreover, the lung clearance rate of the 73-OR strain was significantly higher in TLR2−/− C57/BL6J mice than in wild-type (WT) mice. Histological analysis showed that inflammatory responses were milder in TLR2−/− C57/BL6J mice than in WT mice, which was confirmed by a decrease in cytokine levels in TLR2−/− C57/BL6J mice. Overall, these results indicate that TLR2 promoted M. catarrhalis adhesion and invasion of A549 cells and lung tissues and mediated inflammatory responses in infected lungs. This study provides important insights into the development of potential therapeutic strategies against M. catarrhalis and TLR2-induced inflammatory responses.
Introduction
Moraxella catarrhalis is a major cause of chronic obstructive pulmonary disease (COPD) [Citation1], which is characterized by chronic cough and shortness of breath. The essential first step in bacterial colonization and pathogenic processes is attachment to the human respiratory epithelium [Citation2]. Several M. catarrhalis-specific outer membrane proteins possess adhesive functions [Citation3–6]; however, information on the mechanism of M. catarrhalis colonization and infection of the respiratory tract is limited.
Toll-like receptors (TLRs), a family of pattern recognition receptors (PRRs) of the innate immune system, are responsible for the early recognition of invading pathogens [Citation7]. Although all 10 TLRs expressed in humans can respond to pathogen-associated molecular patterns (PAMPs), TLR2 is the most promising receptor because it recognizes a vast repertoire of ligands derived from several pathogens. TLR2 is expressed in several cell types, including immune, endothelial, and epithelial cells [Citation8]. The rapid initiation of pro-inflammatory responses by TLR2 is critical for controlling infection; however, dysregulation of this signalling pathway can lead to excessive release of pro-inflammatory cytokines, playing a role in the pathology of several diseases. Therefore, inhibiting TLR2 activation can help suppress overactive immune responses and prevent inflammatory damage. In addition, studies have shown that M. catarrhalis induces an inflammatory immune response in respiratory epithelial cells that is mostly dependent on TLR2 [Citation9,Citation10]. However, the mechanisms by which this pathogen adheres to and invades the respiratory epithelium are not well understood.
The present study aimed to reveal the mechanism underlying the adhesion and invasion of the highly virulent M. catarrhalis strain 73-OR, which was clinically isolated in a previous study [Citation11] focusing on the role of TLR2. This study provides important insights into the development of potential therapeutic strategies against M. catarrhalis and TLR2-induced inflammatory responses.
Materials and methods
Cell lines and culture conditions
A549 cells (type II alveolar cells) were obtained from the American Type Culture Collection and cultured in Ham’s F12 medium (Gibco, Thermo Fisher Scientific, Waltham, MA, USA) supplemented with 10% heat-inactivated foetal calf serum (Gibco), 100 U/mL penicillin, and 0.1 mg/mL streptomycin (Solarbio, Beijing, China). Cells were cultured in 25-cm2 flasks until 95–100% confluency, trypsinized, and transferred to 24-well plates (Thermo Fisher Scientific) for adhesion and invasion assays. TLR2−/− A549 cells (YKO-HS715) were purchased from Ubigene Biosciences (Guangzhou, China) and cultured in Ham’s F12 medium. A549 and TLR2−/− A549 cells were cultured at 37 ℃ in a humidified 5% CO2 incubator.
M. catarrhalis strains and reagents
M. catarrhalis 73-OR strain, belonging to a macrolide-susceptible clone CC446 (without the A2330T mutation) with weak adhesion to and strong invasion abilities into A549 cells [Citation11], was obtained from the sputum of a child diagnosed with lower respiratory tract infection [Citation11]. The TLR2 inhibitor TLR2-IN-C29 (CAT: 27029) and TLR2 agonist Pam3CSK4 (CAT: tlrl-pms) were purchased from Cayman Chemical and InvivoGen, respectively.
Adhesion and invasion assay
The adhesion assay was performed with A549 cells as described in our previous study [Citation9]. Briefly, the A549 cells were plated and incubated with an appropriate multiplicity of infection (MOI, 10:1) of M. catarrhalis for 4 h. After removing non-adherent bacteria with phosphate-buffered saline (PBS), cells were lysed with 200 µL of 0.25% trypsin (Sigma-Aldrich) at 37 ℃ for 10 min and 300 µL of 0.1% saponin (Sigma-Aldrich) for 10 min. The lysates were transferred to 1.5 mL Eppendorf tubes and mixed vigorously for 30 s. Aliquots of the samples were serially diluted (1 × 10−5 and 1 × 10−6) and plated onto blood agar plates (100 µL/plate) containing 5% sheep blood to determine the number of adherent bacteria. All assays were performed with 4–6 replicates, and at least three independent experiments were conducted. Results of representative experiments are presented as mean [colony-forming unit (CFU)/per well] ± standard deviation (SD).
The procedure of the invasion assay was similar to that of the adhesion assay as described in our previous study [Citation11]. Briefly, A549 cells were plated and incubated with M. catarrhalis as mentioned above. After removing non-adherent bacteria, 300 µg/mL of gentamycin was added for 1 h to kill any remaining adherent extracellular bacteria. Thereafter, the cells were washed with PBS again to remove residual gentamycin, followed by cell lysis. Finally, the number of invasive bacteria was calculated as previously described [Citation11].
Quantitative reverse transcription-polymerase chain reaction (qRT-PCR)
Total RNA was extracted from the cells using TRIzol reagent (15596026; Thermo Fisher Scientific), and the RNA concentration of each sample was calculated using a spectrophotometer. RNA was dissolved in diethyl pyrocarbonate (DEPC) water, and then cDNA was synthesized using the SuperScript III First-Strand Synthesis Super Mix Kit (18080400; Thermo Fisher Scientific). cDNA was amplified using Luna Universal qPCR Master Mix (M3003L; New England Biolabs). The relative expression of target genes was normalized to that of gapdh (internal reference), and multiple mRNA changes were calculated. Primer sequences were prepared by Sino Biological (Beijing, China) ().
Table 1. Gene primer sequences.
RNA-mediated interference (RNAi)
Scrambled RNAi oligonucleotides and small interfering RNAs (siRNAs) targeting TLR2 (Sigma-Aldrich) were transfected into A549 cells for 72 h using Lipofectamine RNAiMAX (Thermo Fisher Scientific), according to the manufacturer’s protocol. After the designated treatments, cell lysates were prepared for PCR analysis. The siRNA sequences are listed in .
Table 2. Small interfering RNA sequences.
Transmission electron microscopy (TEM)
The observation of M. catarrhalis adhesion to and invasion into A549 cells was performed as described previously with TEM [Citation11]. Briefly, A549 cells were plated and infected with M. catarrhalis strain 73-OR for 4 h. Non-adherent bacteria were removed by washing with PBS. Thereafter, the cells were lysed with 200 µL of 0.25% trypsin at 37 ℃ for 10 min, followed by centrifugation and washing with PBS. Infected A549 cells were fixed in 2.5% glutaraldehyde in PBS (0.06 M) for up to two days (pH 7.3). Cells were prepared for TEM as previously described [Citation12]. The distribution of M. catarrhalis isolates outside and inside A549 cells was determined at a magnification of × 3000, and lamellipodia enclosing the isolates and different phases of macropinocytotic ingestion were observed at a magnification of × 15000.
Mouse pulmonary clearance model
Male WT C57Black (BL)/6J mice and male TLR2–/– mice (C001263), aged 6–8 weeks, were obtained from Cyagen Biosciences, Inc (Suzhou, China). The mice were housed under standard pathogen-free conditions in an animal facility at the Institute of Microbiology of the Chinese Academy of Sciences. As described previously, the mouse pulmonary clearance model was established [Citation13–16]. Both the WT C57BL/6J mice and TLR2–/– mice were divided into control and infected groups, which were intratracheally injected with PBS and 108 CFU of 73-OR M. catarrhalis, respectively. At 3 h post-challenge, the mice were sacrificed via CO2 exposure, and blood and lung tissue samples were collected. The number of white blood cells (WBCs) in the blood was measured using an automated haematological analyser (Sysmex Xs-800i; Sysmex Corporation, Kobe, Japan). The lungs were homogenized on ice in 1 mL PBS (pH 7.2–7.4) using a tissue homogenizer. Thereafter, the samples were serially diluted and plated onto blood agar plates (100 µL/plate) containing 5% sheep blood (two replicates). After incubation overnight at 37℃ in a 5% CO2 atmosphere, CFU was determined by manual counting.
Histological and cytokine profile analysis
Histological analysis was performed as previously described [Citation11]. In short, histological changes in the lung tissue sections of each group mentioned above were compared at × 100 and × 400 magnifications.
Lung tissues and plasma were collected from mice after challenge with PBS or 108 CFU of M. catarrhalis (73-OR). The levels of interferon-gamma (IFN-γ), interleukin (IL)-12p70, IL-13, IL-1β, IL-2, IL-4, IL-5, IL-6, tumour necrosis factor-alpha (TNF-α), granulocyte-macrophage colony stimulating factor (GM-CSF), IL-18, IL-10, IL-17A, IL-22, IL-23, IL-27, and IL-9 were measured using the T helper cell (Th)1/Th2/Th9/Th17/Th22/Regulatory T cell (Treg) Cytokine 17-Plex Mouse ProcartaPlex™ Panel (Thermo Fisher Scientific).
Statistical analysis
Statistical analyses were performed using the GraphPad Prism 8 software. Significant differences between groups were determined using one-way analysis of variance (ANOVA). Differences between means were considered significant at *p < 0.05, **p < 0.01, ***p < 0.001.
Ethical statement
This study was approved by the Human Research Ethics Committee of the Peking Union Medical College Hospital (No. JS-2304 and No. JS-2904).
Results
TLR2 inhibitor and agonist act differently in the adhesion and invasion of M. catarrhalis into A549 cells
A549 cells were pretreated with 25 μM of TLR2 inhibitor TLR2-IN-C29 and 10 ng/mL of TLR2 synthetic analog ligand Pam3Cys-Ser-Lys4 (Pam3CSK4) for 1 h, followed by infection with the high-virulence M. catarrhalis strain 73-OR. Pretreatment with TLR2-IN-C29 enhanced the adhesion of the 73-OR strain to A549 cells but reduced its invasion (). In contrast, treatment with the agonist Pam3CSK4 reduced both M. catarrhalis adhesion to and invasion into A549 cells ().
Figure 1. TLR2 inhibitor and agonist act differently in the process of Moraxella catarrhalis adhesion to and invasion into alveolar epithelial A549 cells. Adhesion and invasion analysis of M. catarrhalis 73-OR strain infecting A549 cells pretreated with PBS, (a) TLR2 inhibitor TLR2-IN-C29 (25 μM), or (b) TLR2 agonist Pam3CSK4 (10 ng/mL). Data are from one of three essentially identical experiments; differences were considered significant at p < 0.05.
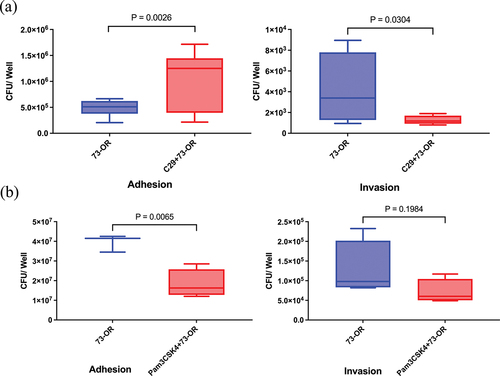
TLR2 is required for the adhesion and invasion of M. catarrhalis
To confirm the regulatory function of TLR2 in M. catarrhalis infection of A549 cells, we performed RNA-mediated interference experiments. RT-PCR confirmed that three tlr2-specific siRNA constructs (No. 34, 35, and 36) caused a decrease in TLR2 mRNA and IL-6 and IL-8 expression in A549 cells () compared to the control siRNA. Therefore, we selected one siRNA construct (No. 34) that had the best interference effect for subsequent experiments. Transfection with tlr2-specific siRNA significantly suppressed 73-OR strain-induced increase in IL-6 and IL-8 secretion by A549 cells (). However, tlr2 RNAi did not significantly decrease the 73-OR strain adhesion to and invasion into A549 cells (data not shown).
Figure 2. TLR2 plays a role in M. catarrhalis adhesion to and invasion into A549 cells. (a) RT-PCR analysis of gene-silencing efficiency in A549 cells transfected with control siRNA (NC) or tlr2-specific siRNA (34, 35, or 36) on days 1, 2, and 3. (b) Expression of TLR2, IL-6, and IL-8 in A549 cells transfected with tlr2-specific siRNA (34). (c) Adhesion and invasion analysis of 73-OR infecting WT A549 cells or TLR2−/− A549 cells constructed via CRISPR-Cas9 gene editing technology. (d) Transmission electron microscopy analysis of 73-OR infecting WT A549 cells or TLR2−/− A549 cells. Scale bars: 2 µm (A1, B1, and C1), 1 µm (B2), or 500 nm (A2 and C2).
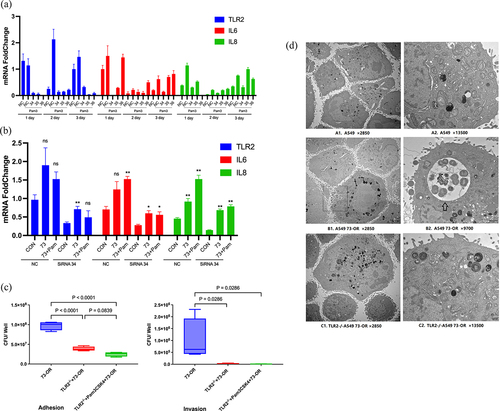
Additionally, TLR2−/− A549 cells constructed using the Clustered regularly interspaced short palindromic repeats (CRISPR)-Cas9 gene editing technology were used to further explore the role of TLR2 in the adhesion and invasion of M. catarrhalis in pulmonary epithelial cells. The adhesion of M. catarrhalis (73-OR strain) and invasion into TLR2−/− A549 cells were significantly reduced (). Moreover, TEM showed that the number of 73-OR strains adhering to and invading TLR2−/− A549 cells was lower than those adhering to and invading WT A549 cells (). The exact process of 73-OR adherence to and invasion into A549 cells is shown in Figure 2d-B1. Overall, these data show that TLR2 participates in M. catarrhalis adhesion to and invasion into pulmonary epithelial cells.
TLR knockout enhances lung M. catarrhalis clearance in vivo
Furthermore, we examined whether TLR2 regulates the clearance of M. catarrhalis from the lungs. WT and TLR2−/− mice were infected by intratracheal injection of either PBS (control group) or M. catarrhalis 73-OR strain, and lung bacterial clearance was determined 3 h later. There was no significant difference in bacterial clearance in PBS-treated WT and TLR2−/− mice. In contrast, lungs from TLR2−/− mice had a significantly lower number of adhering M. catarrhalis compared to those of WT mice after 3 h of infection with the 73-OR strain, indicating a higher bacterial clearance in M. catarrhalis-treated TLR2−/− mice than in M. catarrhalis-treated WT mice (). However, there was no significant difference in WBC count between WT and TLR2−/− mice after M. catarrhalis infection ().
Figure 3. Bacterial counts in the lung tissue and white blood cell (WBC) counts in the blood of WT and TLR2−/− mice after Moraxella catarrhalis infection. Pulmonary clearance and WBC count changes in mice (n = 5–7 per group) challenged with phosphate-buffered saline (PBS), or 108 CFU of M. catarrhalis 73-OR. (a) Clearance of 73-OR from the lungs in TLR2−/− mice and the WT group (P = 0.00222). (b) Comparison of WBC counts in WT and TLR2−/− groups. Pulmonary clearance and WBC count in the WT and TLR2−/− mice were compared using t-tests. Differences were considered significant at p < 0.05.
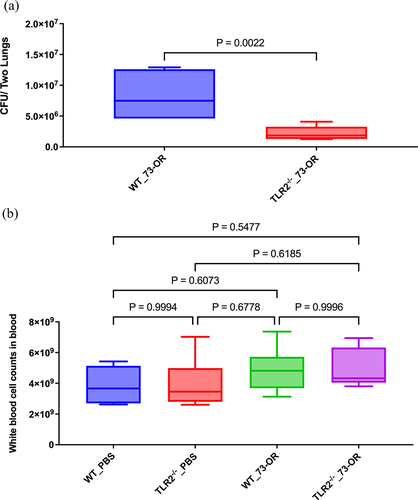
TLR2 contributes to inflammatory responses to M. catarrhalis in vivo
Consistent with the above findings, histological analysis revealed decreased inflammation in the lungs of M. catarrhalis-infected TLR2−/− mouse compared to that in the lungs of WT mouse, with a decrease in neutrophil and monocyte infiltration and loss of normal alveolar architecture (). Additionally, there was a decreasing trend in the concentration of cytokines in the lungs and blood (decreases in IL-18 and IL-23 in the blood were significant) of TLR2−/− C57/BL6J mice compared to the WT mice after 73-OR infection ().
Discussion
TLR2, one of the most important TLRs, is expressed on the surface of epithelial cells. TLR2 receptor plays an important role in the inflammatory response in host respiratory epithelial cells following M. catarrhalis infection [Citation12,Citation17]. However, the role of TLR2 in the adhesion to and invasion into host respiratory epithelial cells by M. catarrhalis has not been investigated. In the present study, the role of TLR2 in M. catarrhalis adhesion and invasion of A549 cells and inflammatory response in pulmonary tissues was revealed; TLR2 was involved in M. catarrhalis adhesion to and invasion into A549 cells in vitro and mouse lungs in vivo and mediated inflammatory responses.
In the present study, to determine the effects of TLR2 in M. catarrhalis adhesion and invasion of A549 cells, the cells were pretreated with the TLR2 inhibitor C29 or the agonist Pam3CSK4 and then infected with M. catarrhalis. Pretreatment with Pam3CSK4, which is a synthetic analog of bacterial lipopeptides and can be specifically recognized by TLR2 () [Citation18,Citation19], reduced M. catarrhalis adhesion to and invasion into A549 cells due to increased competition between the TLR2 agonist and TLR2 ligand in M. catarrhalis [Citation20]. C29, whose main function is to inhibit TLR signalling pathways () [Citation21], enhanced the ability of the 73-OR strain to adhere to A549 cells but reduced its invasion, indicating that the adhesion and invasion of M. catarrhalis may be mediated by different pathways. Overall, these results provide a theoretical basis for further studies on the TLR2 network regulatory system and the identification of effective regulatory factors.
There are two types of TLR signalling pathways: myeloid differentiation factor 88 (MyD88)-dependent pathways and TIR-domain-containing adapter-inducing interferon-β (TRIF)-dependent (MyD88-independent) pathways [Citation22]. TLR2 activates mitogen-activated protein kinases (MAPKs) and nuclear factor kappa-light-chain-enhancer of activated B cells (NF-κB) through a MyD88-dependent signalling pathway [Citation23], leading to the expression of pro-inflammatory cytokines, such as IL-1, IL-6, IL-8, and TNF-α [Citation24]. In the present study, decreased adhesion and invasion of M. catarrhalis as well as attenuated inflammatory responses were observed in TLR2−/− A549 cells and TLR2−/− C57/BL6J mice. TEM analysis confirmed a decrease in M. catarrhalis adhesion and invasion in the TLR2−/− A549 cells compared to WT A549 cells. Moreover, significantly higher bacterial clearance rates were observed in the lungs of TLR2−/− C57/BL6J mice than in those of WT mice. Decreased expression of cytokines was observed in TLR2−/− mice after 73-OR infection compared to that in WT mice, with significant differences observed in IL-18 and IL-23 levels between the groups. Overall, these results indicate that TLR2 is involved in M. catarrhalis adhesion to and invasion into A549 cells and host lung tissues, mediating inflammatory responses. Although the underlying mechanisms revealed in the present study require further investigation, our results suggest TLR2 as a promising therapeutic target for the treatment of human M. catarrhalis infections.
The present study had some limitations. First, our animal sample size was small, which limited the number and the type of analyses that we were able to perform. Moreover, the exact pathway of how M. catarrhalis interacts with the airway epithelial TLR2 is not clear, which represents an interesting topic for subsequent research to provide more mechanistic insights. Additionally, due to our technical limitations, TLR2-overexpressing A549 cells were unavailable to assess bacterial adhesion and invasion results in the present study. Finally, this study only focused on one strain, 73-OR. Elucidating the mechanisms of other strains will help to further understand the role of this microbe in airway health.
In conclusion, this study showed that TLR2 mediates the adhesion and invasion of respiratory epithelial cells by M. catarrhalis both in vitro and in vivo. This study provides important insights into the development of potential therapeutic strategies against M. catarrhalis and TLR2-induced inflammatory responses.
Author contributions
Y.L. and Y.X. designed the study. R.D. and Y.L. wrote the paper. J.Y. and W.K. performed and analysed most of the experiments. L.D. and S.H. helped with the animals’ experiments.
Disclosure statement
The authors declare that the research was conducted in the absence of any commercial or financial relationships that could be construed as a potential conflict of interest.
Data Availability statement
The data that support the findings of this study are available from the corresponding author upon reasonable request.
Correction Statement
This article has been corrected with minor changes. These changes do not impact the academic content of the article.
Additional information
Funding
References
- Verduin CM, Hol C, Fleer A, et al. M. catarrhalis: from emerging to established pathogen. Clin Microbiol Rev. 2002;15(1):125–10. doi: 10.1128/CMR.15.1.125-144.2002
- Murphy TF, Sethi S, Niederman MS. The role of bacteria in exacerbations of COPD. A constructive view. Chest. 2000;118(1):204–209. doi: 10.1378/chest.118.1.204
- Aebi C, Lafontaine ER, Cope LD, et al. Phenotypic effect of isogenic uspA1 and uspA2 mutations on moraxella catarrhalis 035E. Infect Immun. 1998;66(7):3113–3119. doi: 10.1128/IAI.66.7.3113-3119.1998
- Lafontaine ER, Cope LD, Aebi C, et al. The UspA1 protein and a second type of UspA2 protein mediate adherence of moraxella catarrhalis to human epithelial cells in vitro. J Bacteriol. 2000;182(5):1364–1373. doi: 10.1128/JB.182.5.1364-1373.2000
- Hu WG, Chen J, McMichael JC, et al. Functional characteristics of a protective monoclonal antibody against serotype a and C lipooligosaccharides from Moraxella catarrhalis. Infect Immun. 2001;69(3):1358–1363. doi: 10.1128/IAI.69.3.1358-1363.2001
- Forsgren A, Brant M, Karamehmedovic M, et al. The immunoglobulin D-binding protein MID from Moraxella catarrhalis is also an adhesin. Infect Immun. 2003;71(6):3302–3309. doi: 10.1128/IAI.71.6.3302-3309.2003
- Akira S, Uematsu S, Takeuchi O. Pathogen recognition and innate immunity. Cell. 2006 Feb 24;124(4):783–801. doi: 10.1016/j.cell.2006.02.015
- Simpson ME, WA P Jr. TLR2 as a therapeutic target in bacterial infection. Trends Mol Med. 2020;26(8):715–717. doi: 10.1016/j.molmed.2020.05.006
- Schaar V, de Vries SP, Perez Vidakovics ML, et al. Multicomponent moraxella catarrhalis outer membrane vesicles induce an inflammatory response and are internalized by human epithelial cells. Cell Microbiol. 2011;13(3):432–449. doi: 10.1111/j.1462-5822.2010.01546.x
- Augustyniak D, Seredyński R, McClean S, et al. Virulence factors of moraxella catarrhalis outer membrane vesicles are major targets for cross-reactive antibodies and have adapted during evolution. Sci Rep. 2018;8(1):4955. doi: 10.1038/s41598-018-23029-7
- Liu YL, Ding R, Jia XM, et al. Correlation of moraxella catarrhalis macrolide susceptibility with the ability to adhere and invade human respiratory epithelial cells. Emerg Microbes Infect. 2022;11(1):2055–2068. doi: 10.1080/22221751.2022.2108341
- Slevogt H, Seybold J, Tiwari KN, et al. Moraxella catarrhalis is internalized in respiratory epithelial cells by a trigger-like mechanism and initiates a TLR2- and partly NOD1-dependent inflammatory immune response. Cell Microbiol. 2007;9(3):694–707. doi: 10.1111/j.1462-5822.2006.00821.x
- Inoue S, Nakamura H, Otake K, et al. Impaired pulmonary inflammatory responses are a prominent feature of streptococcal pneumonia in mice with experimental emphysema. Am J Respir Crit Care Med. 2003;167(5):764–770. doi: 10.1164/rccm.2105111
- Jones MM, Johnson A, Koszelak-Rosenblum M, et al. Role of the oligopeptide permease ABC transporter of Moraxella catarrhalis in nutrient acquisition and persistence in the respiratory tract. Infect Immun. 2014;82(11):4758–4766. doi: 10.1128/IAI.02185-14
- Murphy TF, Brauer AL, Johnson A, et al. ATP-Binding cassette (ABC) transporters of the human respiratory tract pathogen, moraxella catarrhalis: role in virulence. PLoS One. 2016;11(7):e0158689. doi: 10.1371/journal.pone.0158689
- Singh B, Alvarado-Kristensson M, Johansson M, et al. The respiratory pathogen moraxella catarrhalis targets collagen for maximal adherence to host tissues. mBio. 2016;7(2):e00066. doi: 10.1128/mBio.00066-16
- Slevogt H, Schmeck B, Jonatat C, et al. Moraxella catarrhalis induces inflammatory response of bronchial epithelial cells via MAPK and NF-κB activation and histone deacetylase activity reduction. Am J Physiol Lung Cell Mol Physiol. 2006;290(5):L818–26. doi: 10.1152/ajplung.00428.2005
- Jin MS, Kim SE, Heo JY, et al. Crystal structure of the TLR1-TLR2 heterodimer induced by binding of a tri-acylated lipopeptide. Cell. 2007;130(6):1071–1082. doi: 10.1016/j.cell.2007.09.008
- Kang JY, Nan X, Jin MS, et al. Recognition of lipopeptide patterns by Toll-like receptor 2-Toll-like receptor 6 heterodimer. Immunity. 2009;31(6):873–84. doi: 10.1016/j.immuni.2009.09.018
- Su YC, Singh B, Riesbeck KM. Moraxella Catarrhalis: from interactions with the host immune system to vaccine development. Future Microbiol. 2012;7(9):1073–1100. doi: 10.2217/fmb.12.80
- Mistry P, Laird MH, Schwarz RS, et al. Inhibition of TLR2 signaling by small molecule inhibitors targeting a pocket within the TLR2 TIR domain. Proc Natl Acad Sci U S A. 2015;112(17):5455–5460. doi: 10.1073/pnas.1422576112
- Hamanaka J, Hara H. Involvement of toll-like receptors in ischemia-induced neuronal damage. Cent Nerv Syst Agents Med Chem. 2011;11(2):107–113. doi: 10.2174/187152411796011312
- Nagyőszi P, Wilhelm I, Farkas AE, et al. Expression and regulation of toll-like receptors in cerebral endothelial cells. Neurochem Int. 2010;57:556–564. doi: 10.1016/j.neuint.2010.07.002
- Sethi S, Wrona C, Eschberger K, et al. Inflammatory profile of new bacterial strain exacerbations of chronic obstructive pulmonary disease. Am J Respir Crit Care Med. 2008;177(5):491–497. doi: 10.1164/rccm.200708-1234OC