ABSTRACT
Newcastle disease virus (NDV) typically induces severe illness in poultry and results in significant economic losses for the worldwide poultry sector. NDV, an RNA virus with a single-stranded negative-sense genome, is susceptible to mutation and immune evasion during viral transmission, thus imposing enormous challenges to avian health and poultry production. NDV is composed of six structural proteins and two nonstructural proteins that exert pivotal roles in viral infection and antiviral responses by interacting with host proteins. Nowadays, there is a particular focus on the mechanisms of virus-host protein interactions in NDV research, yet a comprehensive overview of such research is still lacking. Herein, we briefly summarize the mechanisms regarding the effects of virus-host protein interaction on viral infection, pathogenesis, and host immune responses. This review can not only enhance the present comprehension of the mechanism underlying NDV and host interplay, but also furnish a point of reference for the advancement of antiviral measures.
Introduction
As avian paramyxovirus type 1 (APMV-1), Newcastle disease virus (NDV) belongs to the genus Orthoavulavirus within the Paramyxoviridae family [Citation1]. The NDV strains can be categorized into three primary pathogenicity groups: avirulent, low pathogenic (lentogenic), intermediate pathogenic (mesogenic), and highly pathogenic (velogenic) strains [Citation2,Citation3]. The virulent NDV can usually cause a highly contagious and economically catastrophic viral ailment [Citation4]. In the most recent phylogenetic classification system, NDVs can be subdivided into two categories known as class I and class II [Citation5]. Class I comprises a unique genotype 1, accompanied by the sub-genotype 1.1.1, 1.1.2, and 1.2. Its genome length is 15,198 nucleotides (nt). On the other hand, class II comprises more than 20 genotypes and their genome lengths are either 15,186 or 15,192 nt [Citation6]. Class I NDV is typically avirulent, whereas class II NDV exhibits various virulence [Citation7].
Six open reading frames (ORFs) encode six structural proteins and two nonstructural proteins of NDV [Citation8,Citation9]. The structural proteins include the NP (nucleoprotein), P (phosphoprotein), M (matrix), F (fusion), HN (haemagglutinin-neuraminidase), and L (RNA-dependent RNA polymerase) protein [Citation10]. The W and V proteins, both nonstructural proteins, result from a specific RNA editing method by nucleotide shifting of the P gene during transcription [Citation11]. These viral proteins pose significant effects on multiple stages of the NDV infection cycle. In the initial phase of viral infection, the HN protein can attach to the surface of host cells by targeting sialic acid receptors. This can facilitate the adsorption of the virus and the host cells [Citation12,Citation13]. Meanwhile, the HN protein promotes the viral envelope and host cell membrane fusion mediated by the F protein [Citation14]. When membrane fusion occurs, viral nucleic acids are released into the cytoplasm, initiating a fresh cycle of viral replication [Citation15]. The ribonucleoprotein (RNP) complex of NDV is generated and released into the cytoplasm by hijacking the cell, whereby the RNA polymerase further promotes the production of the mRNA required for viral protein synthesis [Citation16]. Subsequently, viral mRNAs are translated into a distinct set of viral proteins [Citation17]. The M protein promotes NDV protein assembly, enabling virion release through budding [Citation18]. Finally, the sialic acid residues are eliminated by the HN protein through its neuraminidase (NA) activity, thereby enhancing virus release [Citation19].
Generally, certain host restriction factors can be utilized to specifically counter viral infection by targeting viral proteins [Citation20,Citation21]. However, NDV, like other paramyxoviruses, has evolved into exploiting the host machinery in order to carry out its replication cycle [Citation22,Citation23]. Currently, numerous studies have demonstrated the interactions between NDV proteins and host proteins, thereby playing a pivotal role in the modulation of NDV infection. However, a systematic summary of these studies is lacking. Herein, we aim to investigate the association between NDV and host from the perspective of protein interaction. The amassed knowledge of such interactions will not only enhance the present comprehension of the mechanism underlying NDV pathogenesis but also provide valuable reference for virus prevention and control.
Main text
NP protein
As a head structural protein, NDV NP protein is highly abundant and conserved within virus particles [Citation24]. The different spacer sequences of noncoding regions and base insertion lead to different lengths of the NP full-length gene. The coding segment of NP spans 1467 nt long and translates into a NP protein consisting of 489 amino acids (aa) [Citation25]. The NP protein can be further divided into two primary regions: the NCORE (called amino-terminal) and NTAIL (called carboxy-terminal) domains [Citation26]. The NP protein can achieve this by wrapping around the RNA and form a protective shield, thereby protecting the viral genomic RNA from degradation by cellular nucleases [Citation27]. During the viral assembly stage, the NP protein interacts with the M protein to aid in the budding of new virions. This interaction facilitates the successful packaging of viral genetic material into the budding virions [Citation28,Citation29]. Notably, the NP protein is required for the synthesis of viral genomes together with P and L proteins [Citation2]. Meanwhile, it actively participates in facilitating the mRNA translation of NDV through the activation of host cascades. Zhan et al. [Citation30] have elucidated the relevant molecular mechanism by which NDV NP protein activates host signalling pathways. Briefly, NDV triggers both signalling pathways targeting PI3K/Akt/mTOR and p38 MAPK/Mnk1 to enhance the cap-dependent translation of host. Of note, NDV NP protein plays a crucial role in mediating viral selective cap-dependent translation through interacting with the eukaryotic translation initiation factor eIF4E. This phenomenon represents the primary stage in the initiation of cap-dependent translation [Citation31]. Similarly, Li et al. [Citation32] have also demonstrated that the NP- eIF4E interaction during NDV infection. They observed that the NP-eIF4E interaction could be further modulated by mutant methyltransferase motifs (K-D-K-E) of NDV L protein, thereby enhancing viral replication, transcription, and translation. These findings underscore a new strategy through which NDV exploits the transcription and translation machinery of host for viral genome synthesis. Upon infection, NDV has the capability to trigger an interferon (IFN) response [Citation33]. 14–3–3ε, known for its interactions with retinoic acid-inducible gene I (RIG-I) and mitochondrial antiviral signalling protein (MAVS), serves as a crucial component within IFN-related host cascades [Citation34]. Xu et al. [Citation35] investigated the role of 14–3–3ε in NDV infection and observed that viral replication was promoted via the enhanced degradation of 14–3–3ε. Meanwhile, activation of the IFN pathway necessitated the presence of 14-3-3ε, which facilitated the interaction between MDA5 and MAVS during NDV infection. Importantly, the NP protein promoted the degradation of 14-3-3ε by facilitating its colocalization within lysosomes. Therefore, the NP-14-3-3ε interaction allows NDV to evade the IFN response and boost viral replication capabilities.
P protein
NDV P gene can be divided into 1463 nt of class I and 1451 nt of class II, which further encodes P proteins of 395 aa and 399 aa, respectively [Citation36,Citation37]. The P protein is an important structural protein and contains three parts: the NTD (called N-terminal), OD (called central oligomerization), and XD (called C-terminal X) domains [Citation38,Citation39]. The P protein, an indispensable element of RNA-dependent RNA polymerase (RdRp), plays a vital role in the synthesis of viral RNA [Citation11]. It together with NP, L proteins, and RNA genome forms the ribonucleoprotein complex (RNP) [Citation40]. Moreover, it effectively inhibits the illicit encapsidation of viral and non-viral mRNA by interacting with the NP protein [Citation41,Citation42]. Therefore, the replication and transcription of the viral genome depend on NDV P protein. Caspase recruitment domain-containing protein 11 (CARD11) is widely present within animal tissues and regulates signalling pathways following activation of the immune cell receptor [Citation43–45]. However, several studies have explored the precise role of CARD11 during NDV infection. Wang et al. [Citation46] have reported a direct interaction between the P and CARD11 proteins that involves in the RNP synthesis in NDV-infected neurons. They observed that both CARD11 and L competitively could bind to the XD domain of NDV P protein, thereby inhibiting the P-L protein interaction within the viral RNP complex. Consequently, this disruption led to a decrease in the polymerase activity and replication levels of NDV in neurons. Furthermore, a similar phenomenon was also observed in chicken fibroblasts, a non-neural cell model [Citation47]. The overexpression of CARD11 in DF-1 cells resulted in reduced NDV replication, whereas the knockdown of CARD11 in DF-1 cells enhanced viral replication. Notably, NDV P protein could co-localize with CARD11, thereby forming aggregates around the nucleus of the fibroblast. This suggests a strong interaction between the P and CARD11 proteins. Moreover, they revealed that CARD11 could effectively reduce syncytia formation through the CARD11-Bcl10-MALT1 pathway, and then inhibited viral replication in fibroblasts. Therefore, the P-host protein interactions, specifically the P-CARD11 interaction, pose significant effects on the replication and transcription of viral genome.
V and W proteins
The P gene encodes two nonstructural proteins, V and W, using specialized RNA editing methods [Citation48]. The mRNA encoding these proteins is distributed as follows: 68% for the P protein, 29% for the V protein, and 2% for the W protein, respectively [Citation49]. These nonstructural proteins share identical NTD but possess distinct CTD in comparison to the P protein [Citation50]. Significantly, NDV infection can be enhanced by the V and W proteins, which counteract the host immune system [Citation51,Citation52].
A non-templated guanine (G) residue can be inserted at a specific site of the P gene to generate the V protein via an RNA editing mechanism [Citation53]. The V protein is widely involved in suppressing the host innate immunity due to its a cysteine-rich zinc-binding motif [Citation49,Citation54]. Given its vital function in controlling the host immune response, the interactions between the V protein and host proteins, which modulate host immune responses, have been extensively studied in the context of NDV research. SOCS3, belonging to the suppressor of cytokine signalling (SOCS) protein family, is essential for the evasion of various viruses from the host immune system [Citation55,Citation56]. Wang et al. [Citation57] conducted a screening and identification process, revealing that the SOCS3 expression may be increased by exposure to NDV or the V protein. Subsequent assays provided evidence that the regulation of SOCS3 expression by V protein transfection or NDV infection could be influenced via the MEK/ERK signalling pathway. Moreover, U0126, a targeted inhibitor, could efficiently inhibit the MEK/ERK signalling pathway and consequently reduce SOCS3 expression. This inhibition, in turn, promoted the expression of IFN-I, OASL, and M×1and resulted in the suppression of viral replication. Thus, the increased expression of SOCS3 induced by exposure to NDV or the V protein can enhance viral replication, relying on the MEK/ERK signalling pathway. Generally, MAVS is important for the production of those cytokines with antiviral and proinflammatory properties in response to viral infections [Citation58]. Sun et al. [Citation59] have unveiled a new function of the V protein in directing MAVS to suppress the production of IFN. They revealed the role of the V protein in MAVS degradation by recruiting the E3 ubiquitin ligase RNF5, thereby inhibiting IFN production during the late stages of infection. Meanwhile, this observation holds true for multiple paramyxoviruses as well, such as Nipah virus (NiV) and Sendai virus (SeV) [Citation60,Citation61]. The V proteins of these paramyxoviruses exhibit functions similar to those of NDV V protein, including degradation of MAVS and inhibition of IFN production. Therefore, the essential role of the V protein in suppressing cellular IFN production is necessary for facilitating successful virus infection. IFN-α or IFN-β can induce the antiviral effects via the JAK/STAT signalling pathway, while the V protein induces degradation of phosphorylated STAT1 through ubiquitination and inhibits IFN-related pathway [Citation62,Citation63]. Meanwhile, the V protein has the ability to attach itself to the preserved helicase structure of melanoma differentiation-associated protein 5 (MDA5), thereby leading to the suppression of IFN-β [Citation64]. Nan et al. [Citation65] reported that NDV V protein could efficiently inhibit IFN responses by degrading p-STAT1 and MDA5. This inhibition of IFN responses ultimately induced less apoptosis. From this, NDV V protein can function as an inhibitor of cell apoptosis. As a member of the thioredoxin family, thioredoxin-like protein 1 (TXNL1) has been documented to trigger apoptosis in some cell lines [Citation66,Citation67]. Wang et al. [Citation68] elucidated the individual impacts of TXNL1 on cell apoptosis and NDV replication in DF-1 cells, a chicken embryo fibroblast cell line. They successfully identified the V-TXNL1 interaction in DF-1 cells and further demonstrated its inhibition of viral replication by inducing apoptosis via a Bcl-2/Bax and Caspase-3 pathway. As an important RNA-binding protein, Musashi RNA binding protein 1 (MSI1) plays a significant role in various phases of the cell cycle [Citation69]. Yang et al. [Citation70] successfully verified the V-MSI1 interaction, which contributed to blocking NDV release through the inhibition of apoptosis. This report represents the initial comprehensive analysis of the V-MSI1 interaction, demonstrating its ability to suppress NDV replication. CacyBP/SIP is originally identified in mouse tumour cells and poses significant effects on cell apoptosis. However, whether CacyBP/SIP promotes or suppresses cancer cell apoptosis may vary depending on the specific cell type [Citation71,Citation72]. Chu et al. [Citation73] elucidated that CacyBP/SIP could regulate NDV replication effectively through controlling apoptosis within DF-1 cells. They demonstrated the V and CacyBP/SIP interaction in DF-1 cells and further found the significant role of CacyBP/SIP in cell apoptosis and NDV replication. Meanwhile, NDV V protein had the ability to suppress the cell apoptosis activated by CacyBP/SIP. Additionally, Tong et al. [Citation74] reported an interaction between the V and hnRNP H1 proteins in yeast and DF-1 cells. Subsequent assays demonstrated that hnRNP H1 knockdown reduced viral replication, whereas its overexpression, conversely, enhanced viral replication. Interestingly, the V protein significantly regulated the expression of hnRNP H1 at the protein level, not at the transcriptional level. Notably, NDV V protein controlled the hnRNP H1 expression, thereby influencing cell growth. Hence, the V protein can regulate cell proliferation and enhance NDV replication through the interaction with hnRNP H1.
The W protein is another frameshift product of the P gene through RNA editing. The presence of two non-templated G residues is regarded as the insertion point, leading to the generation of the W protein [Citation75]. Much like the V protein, the W protein possesses the capability to neutralize the host cell interferon response, thereby promoting NDV replication [Citation76]. Nevertheless, the W-host protein interactions remain limited owing to its low expression level. As of now, the significant effects of the W protein on viral infection by interacting with host proteins has been demonstrated through its subcellular localization. The transportation of numerous proteins between the nucleus and cytoplasm is controlled by nuclear localization signals (NLS), which facilitate nuclear import, and nuclear export signals (NES), which facilitate nuclear export [Citation77]. As a member of the importin superfamily importin-α, Karyopherin α (KPNA) has the ability to regulate the transport of proteins containing an NLS signal into the nucleus [Citation78]. Yang et al. [Citation79] reported that the W protein was transported into the nucleus in an NLS-dependent manner by interacting with KPNA1/2/6. Conversely, if the W protein harboured an NES signal, it would undergo cytoplasmic transport in a manner independent of chromosomal maintenance 1 (CRM1). CRM1 has been previously proved to recognize the NES motif and control nuclear export of proteins [Citation80]. Further assays showed that the IFN-β expression induced by NDV was reduced due to the nuclear localization of the W protein, thereby enhancing viral infection and pathogenicity. Thus, the W and host protein interaction can influence the protein localization in different cellular compartments, ultimately contributing to viral replication and pathogenicity. Considering the limited research on NDV W protein, it is imperative that greater emphasis be placed on investigating it in the future.
M protein
NDV M gene spans a length of 1241 nt and codes for a M protein consisting of 364 aa. It is situated on the inner envelope surface of NDV and can shuttle between nucleus and cytoplasm. This is important for the connection between the viral envelope and the nucleocapsid [Citation81]. The M protein comprises two crucial functional domains: one resides beneath the viral envelope, while the other facilitates interaction with the RNP complex, enabling communication with both viral glycoproteins and RNP complexes. Generally, the nuclear entry of the M protein is contingent upon its NLS, which can suppress the transcription and translation of host genes at the early stage of infection. Subsequently, the M protein can translocate into the cytoplasm via its NES, where it modulates viral assembly and release at the late stage of infection [Citation82,Citation83].
As a membrane-associated protein, the M protein involves in multiple infection processes, such as nuclear localization, transcription and replication of viral genomes, and assembly and budding of viral particles. Thus, the research on the M and host protein interaction is more extensive compared to studies on other viral proteins. Firstly, this interaction can facilitate NDV infection by controlling the M protein’s localization. Duan et al. [Citation84] successfully elucidated the M- importin β1 interaction and demonstrated the crucial effects on facilitating the nuclear import of the M protein. Importantly, the M-importin α5 interaction depended on importin β1, whereas importin α5 exerted diverse effects on NDV infection and M protein transfer in comparison to importin β1. Briefly, importin α5 negatively regulated the nuclear import of the M protein mediated by importin β1, further impacting viral replication and pathogenicity. Meanwhile, they also identified a nucleolar phosphoprotein B23 as an interaction protein with the M protein. The interaction region encompassed the 30th to 60th aa of the M protein and the 188th to 245th aa of B23. This interaction contributed to the nucleolar localization, but the M protein could disrupt the structure and function of B23 in the nucleolus, thereby augmenting NDV replication and pathogenicity [Citation85]. The nuclear localization of the M protein during infection primarily serves to control the transcription and replication of both host cell and viral genes. Duan et al. [Citation86] demonstrated that the M and chicken bromodomain-containing protein 2 (chBRD2) interaction in the nucleus could promote NDV replication. Generally, the BRD2 protein resides within the nucleus and poses significant effects on modulating the gene transcription and host immune responses [Citation87]. Subsequent research revealed an interaction between both C-terminus domains of the M and chBRD2 proteins. This interaction between M and chBRD2 proteins led to the downregulation of chBRD2 expression and facilitated viral RNA synthesis and transcription. Furthermore, due to the M protein’s ability to independently facilitate NDV budding, its interactions with host proteins in viral budding have attracted increasing interest [Citation88–91]. Actin is now widely acknowledged as a cytoskeletal protein found within the cells, playing significant roles during viral infections [Citation92]. Previous studies have shown the interactions between actin and NDV NP protein [Citation93]. This interaction is required for the accurate assembly and subsequent release of NDV particles [Citation94]. NDV M protein possesses a late-stage FPIV domain akin to M proteins found in other paramyxoviruses, like PIV5, which enables the budding and replication of the M protein. Simultaneously, the budding of the M protein necessitates the involvement of the ESCRT machinery, an endosomal sorting complex impacting virus budding. The multivesicular body protein (CHMP) serves as the central component of the ESCRT complex. Li et al. [Citation95] have discovered the interaction between the M protein and members of the CHMP family, specifically CHMP4B and CHMP4C, which had a significant impact on the replication of NDV. Notably, the F23A mutation within the FPIV L-domain of the M protein can impede this interaction, resulting in impaired budding and subsequent growth abnormalities of NDV.
Moreover, it has been reported that M protein poses significant effects on the regulation of host immune responses in various paramyxovirus studies. ANP32B, the acidic leucine-rich nuclear phosphoprotein 32 family member B, plays essential roles in host immune and antiviral responses. It has been demonstrated to interact with the M protein of some non-segmented negative-strand RNA viruses, such as Hendra and Nipah virus [Citation96]. Günther et al. [Citation97] found that ANP32B interacted with NDV M protein and further determined their intracellular localization. The AND32B-M protein interaction exerted a significant role in host manipulation and immune regulation in infected hosts. Of note, the presence of ANP32B facilitated the accumulation of the M protein near the nucleus, whereas in the absence of ANP32B, the M protein was localized in both the cytoplasm and nucleus. Viperin, a conserved member of the interferon-stimulated genes (ISG) family, can pose great impacts on the innate immune responses of the host. Manisha et al. [Citation98] have determined the M-Viperin interaction that effectively inhibited NDV replication. Briefly, they found that the chicken viperin gene significantly weakened viral pathogenicity by inserting itself into the NDV genome. Further bioinformatics analysis and immunoprecipitation assays successfully substantiated the M-viperin interaction. Hence, NDV M protein contributes to enabling the virus to escape the host immune response by interacting with host proteins.
Additionally, as a promising class of oncolytic viruses, NDV exhibits a robust capacity to selectively target and eradicate cancer cells [Citation99,Citation100]. In numerous cells, apoptosis is triggered through transcriptional regulation of the BH3 domain-encoding gene [Citation101]. Interestingly, NDV M protein encompasses the BH3-like region, which further influences cancer cell death. Meanwhile, the M protein can facilitate cancer cell apoptosis by directly interacting with Bax through its BH3 domain (23—27 aa). This interaction contributes to the rearrangement of Bax in mitochondria, therefore facilitating tumour cell apoptosis [Citation102]. Therefore, the M and host protein interactions exert significant effects on NDV infection and host immune responses.
F and HN protein
Both F and HN proteins are classified as membrane glycoproteins of NDV and play crucial roles in viral infection, encompassing viral attachment, entry, fusion, and release processes [Citation103]. The F gene has a length of 1972 nt, which encodes a protein consisting of 533 aa. Serving as the type I envelope glycoprotein, the F protein contains three main functional regions, including the FP (called fusion peptide), two HR (called hydrophobic heptad repeat), and TM (called transmembrane) domains [Citation104,Citation105]. Furthermore, it can be classified into three subunits: F0, F1, and F2. The inactive precursor F0 initially synthesizes as the F protein and can be triggered through the proteolytic cleavage, subsequently resulting in the formation of the disulphide-linked F1–F2 complex. The F protein enables the fusion of the virus envelope with the cell membrane, thereby enabling the viral genome to be released into the cytoplasm and initiating virus replication [Citation106]. Additionally, the cleavage sites of NDV F protein are crucial for viral virulence, with 112(G/E)(K/R)Q(G/E)RL117 of avirulent strains and 112(R/K)RQ(R/K)RF117 of mesogenic and velogenic strains [Citation107,Citation108]. Till now, extensive research has been conducted on its aetiology, but limited attention can be given to the F-host protein interaction. Only Bu et al. [Citation105] have reported the interaction between host adaptor protein (AP) complexes and the F protein. AP complexes are a group of hetero-tetrameric proteins responsible for orchestrating the vesicle generation, subsequently facilitating their transport via various signalling pathways [Citation109]. These complexes can identify abundant cargo proteins based on the common classification motifs found in the cytoplasmic tail (CT) of transmembrane proteins [Citation110]. Bu et al. used a small interfering RNA system to confirm AP complexes involving in NDV infection. Their findings successfully elucidated the F-AP1M1/AP2M1 interactions. Notably, the YLMY motif played a crucial role in modulating this interaction. Simultaneously, it governed the AP-induced trafficking of the F protein from the cytoplasm to the cell surface, consequently impacting NDV replication. Thus, the essential YLMY motif can recruit AP complexes to aid in the surface localization of the F protein. This recruitment plays a crucial role in the F protein’s ability to impact virus budding.
The HN protein, a type II envelope glycoprotein, exhibits different aa lengths due to its inconsistent open reading frame (ORF) lengths and termination codon positions. This envelope protein generally consists of a maximum of 616 aa in the majority of non-virulent strains, whereas the HN protein of the virulent strains only contains 571 aa [Citation111]. As a multifunctional protein, the HN protein comprises the CT, TM, S (called stalk), and GH (called globular head) domains [Citation112]. The GH domain is responsible for neuraminidase and receptor-binding activities. The stalk domain, consisting of four parallel helix bundles, can enhance the fusogenic activity. Furthermore, the CT domain plays a crucial role in the incorporation of virions and the fusion of cell membrane [Citation13,Citation113]. Thus, NDV HN protein acts as an important factor during various infection stages. Importantly, the interactions between HN and host proteins have been extensively investigated as a pivotal factor in the adsorption of NDV, given its significant role as a key protein influencing virus attachment. Galectin-1, recognized as an immune regulatory molecule, facilitates interactions between hosts and pathogens by binding to glycans on viral surfaces. Sun et al. [Citation114] have demonstrated that the tracheal infection of NDV resulted in increased expression of chicken galectin (CG)-1B. They further revealed the inhibitory effects of CG-1B on viral haemagglutination (HA) activity [Citation115]. Of note, CG-1B effectively bound to the virus through its interaction with the HN protein, with specific G4 N-glycans playing a significant role in enhancing this interaction. This interaction ultimately resulted in a reduction in viral adsorption and replication levels. Therefore, this finding suggests an important role of the CG-1B-HN protein interaction in antiviral defence against NDV by inhibiting viral adsorption. Furthermore, our previous study successfully identified a host skeleton protein, named vimentin, that differentially interacted with mesogenic and velogenic HN proteins. In short, the mesogenic HN protein mediated a strong interaction with vimentin, whereas the velogenic HN protein showed no interaction with vimentin. Vimentin is widely recognized for its significant involvement in multiple stages of viral infection [Citation116]. Here, the diverse interaction resulted in differential effects of vimentin on multiple infection stages of NDV. Briefly, vimentin was essential for viral internalization, fusion, and release of velogenic NDV, whereas it mediated no significant effects on those infection processes of mesogenic NDV. Therefore, the HN-vimentin interaction acts as an inhibitor in regulating vimentin’s role in NDV infection [Citation117]. β-Carboline alkaloids can be widely found in diverse plant sources and demonstrate activity against a range of viruses [Citation118–120]. Three potent inhibitors of various NDV genotypes, namely compounds 6, 7, and 9, were identified as 1-formyl-β-carboline derivatives [Citation121]. Three derivatives primarily inhibited viral adsorption and other entry stages. Further assays demonstrated the direct interactions between NDV HN protein and these three derivatives, which inhibited the PI3K/Akt pathway and subsequently hindered viral entry. Therefore, these discoveries can provide promising scaffolds for the design of novel HN inhibitors. Moreover, NDV HN protein, due to the immunotherapeutic potential and remarkable sialic acid binding abilities, holds promise as a cancer adjuvant [Citation122]. The indirect interaction between the HN protein and the host promotes the oncolytic effects of NDV. As Baradaran et al. [Citation123] shown, the bacterial cells containing the HN-AcmA protein engaged in interactions with the MDA-MB-231 human breast cancer cells. Further assays verified the adhesion of L. lactis and Lb. plantarum, both displaying the recombinant HN, to the MDA-MB-231 cancer cells. This finding demonstrates HN’s potential ability to target cancer cells. Additionally, the HN protein is regarded as the primary antigen for controlling NDV owing to its good immunogenicity. As the smallest naturally occurring antibodies derived from camelid heavy-chain antibodies, the variable domains of the heavy chain of the heavy-chain antibodies (VHH) represent excellent candidates for numerous therapeutic and biotechnological applications [Citation124]. Seven VHHs targeting the HN protein were identified and characterized for the first time by Gao et al. [Citation125]. This significant finding will hold immense promise for advancing NDV diagnosis, understanding its pathogenesis, and developing therapeutic interventions.
L protein
The L protein comprises 2204 aa and has a molecular weight of 250 kDa, which is the largest protein of NDV [Citation32]. It can be categorized into four distinct functional domains, including the N-terminal RdRp, PRNTase (called polyribonucleotidyl transferase), CD (called connector), MTD (called methyltransferase), and CTD domains [Citation38]. The collaboration of L and P proteins can assemble RNA polymerase and mediates a crucial component involved in the NDV cycle [Citation126]. So far, research on the L-host protein interactions has primarily focused on protein stability and its impact on virus proliferation. As the naturally occurring and synthetic compounds, the β-carboline alkaloids have demonstrated exceptional antiviral efficacy [Citation127,Citation128]. Wang et al. [Citation129] successfully identified 9-butyl-harmo, a β-carboline derivative, as a potent antiviral agent against NDV. They identified numerous differentially expressed genes (DEGs) related to protein folding in NDV-infected groups after treatment with 9-butyl-harmol by deep sequencing analysis. Consequently, the HSP90β protein was successfully screened and participated in protein folding. Interestingly, the L protein has been recognized as a client protein of HSP90β among several viral proteins. Meanwhile, 9-butyl-harmol could disrupt the HSP90β-L interaction through the direct interaction with HSP90β. This disruption ultimately suppressed viral growth. The researchers further showcased that the compound 9-butyl-harmol employed the ubiquitin-proteasome system to interfere with and degrade the L protein. Therefore, this discovery clarifies an underlying mechanism of 9-butyl-harmol against NDV. The microRNAs (miRNAs) have been shown to regulate viral replication by interacting with various host mRNAs [Citation130]. Based on this, Chen et al. [Citation131] further investigated the interaction between miRNAs and NDV genes associated with viral replication. They revealed that miRNAs could regulate the infection of NDV through interacting with the L gene. Here, gga-miR-1603 and 1794 were recognized as specific regulators of the L gene. These miRNAs facilitated the degradation of the L gene through direct interaction, thereby inhibiting viral replication. Furthermore, host proteins can modulate the interactions between NDV proteins through specific mechanisms. As we previously introduced earlier in the section on P protein, the CARD11 protein can mediate the interaction of NDV L protein with NDV P protein via its domain. Briefly, the L and CARD11 proteins can competitively bind to the X domain of the P protein, consequently obstructing the interaction between L and P within RNP complexes of NDV. This interference can induce a decrease in virus replication and polymerase activities [Citation46]. Taken together, these interactions between the L protein and host proteins are essential for the protein stability and virus replication.
Conclusion
NDV encodes six structural proteins, designated NP, P, M, F, HN, and L, along with two non-structural proteins, known as V and W (). The virus-host protein interactions have been a prominent topic in the field of NDV research for a considerable period. As basic research on NDV has progressed, numerous host proteins or other molecules that interact with NDV proteins have been identified and continue to be discovered. The interactions between host proteins and NDV proteins exhibit significant effects on viral infection () and host antiviral responses (). The virus-host protein interplay generally contributes to the regulation of NDV infection and virulence by influencing various aspects, including transcription, translation, the synthesis of viral RNA, nuclear localization, viral infection processes, protein stability, host immune responses, and the regulation of signalling pathways, among others (). Research on the interactions between NDV proteins and host proteins is abundant, but there is a noticeable imbalance in studies focused on each viral protein. For instance, research into the interactions between M or V proteins and host proteins has notably surpasses that of other NDV proteins. Certain virulence-related proteins, especially two envelope glycoproteins F and HN, have been recognized as crucial factors influencing NDV infection and pathogenicity. However, investigations into their interactions with host proteins have remained comparatively limited. Thus, more attention should be paid to mechanisms underlying such protein interactions, which will yield significant implications in elucidating the pathogenesis of NDV.
Figure 1. Schematic representation of NDV proteins. The primary structural domains for NP, P (V/W), M, F, HN, and L proteins of NDV are displayed. NCORE, a conserved core domain; NTAIL, a variable tail domain; NTD, N-terminal domain; OD, central oligomerization domain; XD, C-terminal X domain; NES, nuclear export signals; NLS, nuclear localization signals; FP, fusion peptide; HRA, fusion peptide domain A; HRB, fusion peptide domain B; TM, transmembrane domain; CT, cytoplasmic tail; S, stalk; GH, globular head; RdRp, RNA-dependent RNA polymerase; PRNTase, polyribonucleotidyl transferase; CD, connector domain; MTD, methyltransferase domain; CTD, C-terminal domain.

Figure 2. Schematic representation illustrating the interaction between host cellular proteins and NDV proteins, crucial for viral infection. The diagram lists host proteins that have been extensively studied for their roles in various NDV infection processes. The schematic representation is created with Biorender.com.
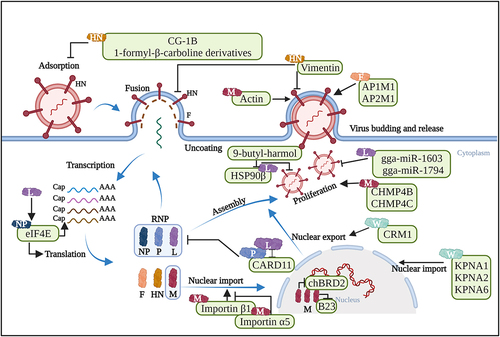
Figure 3. Schematic representation illustrating the interactions between host cellular proteins and NDV proteins, which regulate host antiviral responses. Host proteins that have been well explored for their roles in host antiviral responses during NDV infection are listed. The schematic representation is created with Biorender.com.
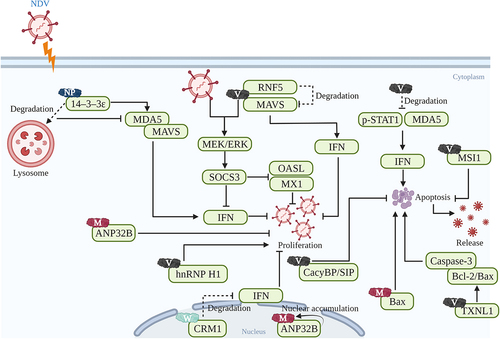
Table 1. The functions of virus-host protein/compound interactions during NDV infection.
The Paramyxoviridae family comprises a collection of most destructive pathogens affecting both humans and animals [Citation132]. Different paramyxoviruses exhibit significant similarities in terms of their viral proteins. Consequently, the interactions between the NDV protein and host proteins can be inferred from other paramyxovirus models, such as Measles virus (MeV), SeV, and parainfluenza virus 5 (PIV5). For MeV, its haemagglutinin (H) protein bears a striking resemblance to the HN protein of NDV and poses great effects on the initial infection phase by binding with the CD46 receptor. The researchers have identified the specific mutations in MeV H protein that allow MeV to utilize CD46 as a receptor [Citation133]. As for SeV, its V protein is also modified through a unique RNA method involving nucleotide shifting of the P gene during transcription, akin to the process observed in NDV V protein [Citation134]. The V-NLRP3 interaction can impede the self-oligomerization of NLRP3 and the recruitment of ASC, thereby obstructing the NLRP3-dependent ASC oligomerization. Conversely, this can inhibit the secretion of IL-1β by deactivating the NLRP3 inflammasome [Citation135]. Regarding PIV5, its M protein harbours structural domains similar to those found in NDV M protein, such as FPIV-like sequences near their amino-terminal ends [Citation136]. PIV5 M protein can interact with angiomotin-like 1 (AmotL1) in yeast, transfected mammalian cells, and virus-infected cells. Moreover, the PIV5 M and AmotL1 interaction is beneficial to viral infection through regulating viral budding [Citation137]. The number of published studies on this topic is substantial; however, we regret that we cannot cite all pertinent studies related to other paramyxoviruses due to space constraints.
Most notably, research on the virus-host protein interactions can be advantageous for the advancement of vaccines or antiviral drugs. This will be of great significance for the well-being of both animals and humans. Vaccine immunization, as the primary approach for preventing and controlling ND, has consistently posed challenges for researchers. The insights gained from studying the virus-host protein interactions can offer valuable information for the development of NDV vaccines. According to the hypothesis proposed by Jiang et al. [Citation138], the site modification regarding the virus-host protein interactions has the potential to boost the growth of the vaccine seed virus and enhance the protective antigen levels. Additionally, antiviral drugs are also a crucial intervention for the prevention and control of ND, such as 9-butyl-harmol [Citation129] and rhein [Citation139]. Analyzing virus-host protein interactions contributes to identify the suitable host proteins for the development of effective antiviral drugs. In conclusion, we have comprehensively reviewed the interactions between NDV proteins and host proteins, along with their effects on viral infection. While scientific prevention and control measures have effectively managed NDV in China, it remains a persistent concern in various countries and regions around the world, leading to substantial economic losses. Nevertheless, the existing research on the virus-host protein interaction is inadequate, leaving numerous unanswered inquiries. Hence, there is an urgent need for further exploration into the interaction mechanisms between viral and host proteins.
Disclosure statement
No potential conflict of interest was reported by the author(s).
Data availability statement
The data that support the findings of this study are openly available in the National Library of Medicine at https://www.ncbi.nlm.nih.gov/.
Additional information
Funding
References
- Amarasinghe GK, Ayllon MA, Bao Y, et al. Taxonomy of the order mononegavirales: update 2019. Arch Virol. 2019;164(7):1967–15. doi: 10.1007/s00705-019-04247-4
- Alexander DJ. Newcastle disease and other avian paramyxoviruses. Rev Sci Tech. 2000;19(2):443–62. doi: 10.20506/rst.19.2.1231
- de Leeuw OS, Koch G, Hartog L, et al. Virulence of Newcastle disease virus is determined by the cleavage site of the fusion protein and by both the stem region and globular head of the haemagglutinin–neuraminidase protein. J Gen Virol. 2005;86(6):1759–1769. doi: 10.1099/vir.0.80822-0
- Habib M, Yaqub T, Nazir J, et al. Genomic and biological characterization of Newcastle disease viruses isolated from migratory mallards (Anas platyrhynchos). Arch Virol. 2018;163(8):2179–2188. doi: 10.1007/s00705-018-3840-8
- Dimitrov KM, Abolnik C, Afonso CL, et al. Updated unified phylogenetic classification system and revised nomenclature for Newcastle disease virus. Infect Genet Evol. 2019;74:103917. doi: 10.1016/j.meegid.2019.103917
- Samuel A, Nayak B, Paldurai A, et al. Phylogenetic and pathotypic characterization of Newcastle disease viruses circulating in west Africa and efficacy of a current vaccine. J Clin Microbiol. 2013;51(3):771–81. doi: 10.1128/JCM.02750-12
- Dimitrov KM, Ramey AM, Qiu X, et al. Temporal, geographic, and host distribution of avian paramyxovirus 1 (Newcastle disease virus). Infect Genet Evol. 2016;39:22–34. doi: 10.1016/j.meegid.2016.01.008
- Morrison TG. Structure and function of a paramyxovirus fusion protein. Biochim Biophys Acta. 2003;1614(1):73–84. doi: 10.1016/S0005-2736(03)00164-0
- Alexander DJ. Newcastle disease and other avian paramyxovirus. Rev Sci Tech. 2000;19(2):443–462. doi: 10.20506/rst.19.2.1231
- Chen L, Song J, Liu H, et al. Phylodynamic analyses of class I Newcastle disease virus isolated in China. Transbound Emerg Dis. 2021;68(3):1294–1304. doi: 10.1111/tbed.13785
- Jadhav A, Zhao L, Ledda A, et al. Patterns of RNA editing in Newcastle disease virus infections. Viruses. 2020;12(11):1249. doi: 10.3390/v12111249
- Jin J, Zhao J, Ren Y, et al. Contribution of HN protein length diversity to Newcastle disease virus virulence, replication and biological activities. Sci Rep. 2016;6(1):36890. doi: 10.1038/srep36890
- Porotto M, Salah Z, DeVito I, et al. The second receptor binding site of the globular head of the Newcastle disease virus hemagglutinin-neuraminidase activates the stalk of multiple paramyxovirus receptor binding proteins to trigger fusion. J Virol. 2012;86(10):5730–41. doi: 10.1128/JVI.06793-11
- Chi M, Xie W, Liu Y, et al. Mutations in the DI-DII linker of the NDV fusion protein conferred hemagglutinin-neuraminidase-independent cell fusion promotion. J Gen Virol. 2019;100(6):958–967. doi: 10.1099/jgv.0.001278
- Ji Y, Liu T, Jia Y, et al. Two single mutations in the fusion protein of Newcastle disease virus confer hemagglutinin-neuraminidase independent fusion promotion and attenuate the pathogenicity in chickens. Virology. 2017;509:146–151. doi: 10.1016/j.virol.2017.06.021
- Molouki A, Peeters B. Rescue of recombinant Newcastle disease virus: a short history of how it all started. Arch Virol. 2017;162(7):1845–1854. doi: 10.1007/s00705-017-3308-2
- Fearns R, Plemper RK. Polymerases of paramyxoviruses and pneumoviruses. Virus Res. 2017;234:87–102. doi: 10.1016/j.virusres.2017.01.008
- Kong L, You R, Zhang D, et al. Infectious bronchitis virus infection increases pathogenicity of H9N2 avian influenza virus by inducing severe inflammatory response. Front Vet Sci. 2021;8:824179. doi: 10.3389/fvets.2021.824179
- Iorio RM, Field GM, Sauvron JM, et al. Structural and functional relationship between the receptor recognition and neuraminidase activities of the Newcastle disease virus hemagglutinin-neuraminidase protein: receptor recognition is dependent on neuraminidase activity. J Virol. 2001;75(4):1918–27. doi: 10.1128/JVI.75.4.1918-1927.2001
- Knott GJ, Bond CS, Fox AH. The DBHS proteins SFPQ, NONO and PSPC1: a multipurpose molecular scaffold. Nucleic Acids Res. 2016;44(9):3989–4004. doi: 10.1093/nar/gkw271
- Perreira JM, Chin CR, Feeley EM, et al. Ifitms restrict the replication of multiple pathogenic viruses. J Mol Biol. 2013;425(24):4937–55. doi: 10.1016/j.jmb.2013.09.024
- Liu P, Tang N, Meng C, et al. SLC1A3 facilitates Newcastle disease virus replication by regulating glutamine catabolism. Virulence. 2022;13(1):1407–1422. doi: 10.1080/21505594.2022.2112821
- Gong Y, Tang N, Liu P, et al. Newcastle disease virus degrades SIRT3 via PINK1-PRKN-dependent mitophagy to reprogram energy metabolism in infected cells. Autophagy. 2022;18(7):1503–1521. doi: 10.1080/15548627.2021.1990515
- Kho CL, Tan WS, Tey BT, et al. Regions on nucleocapsid protein of Newcastle disease virus that interact with its phosphoprotein. Arch Virol. 2004;149(5):997–1005. doi: 10.1007/s00705-003-0273-8
- Makkay AM, Krell PJ, Nagy E. Antibody detection-based differential ELISA for NDV-infected or vaccinated chickens versus NDV HN-subunit vaccinated chickens. Vet Microbiol. 1999;66(3):209–222. doi: 10.1016/S0378-1135(99)00016-4
- Zhang G, Xue J, Li X. The influence of Newcastle disease virus major proteins on virulence. Vet Sci Res. 2021;3(02). doi: 10.30564/vsr.v3i2.4098
- El Najjar F, Schmitt AP, Dutch RE. Paramyxovirus glycoprotein incorporation, assembly and budding: a three way dance for infectious particle production. Viruses. 2014;6(8):3019–54. doi: 10.3390/v6083019
- Krishnamurthy S, Samal SK. Nucleotide sequences of the trailer, nucleocapsid protein gene and intergenic regions of Newcastle disease virus strain Beaudette C and completion of the entire genome sequence. J Gen Virol. 1998;79(Pt 10):2419–2424. doi: 10.1099/0022-1317-79-10-2419
- Pantua HD, Mcginnes LW, Peeples ME, et al. Requirements for the assembly and release of Newcastle disease virus-like particles. J Virol. 2006;80(22):11062–11073. doi: 10.1128/JVI.00726-06
- Zhan Y, Yu S, Yang S, et al. Newcastle disease virus infection activates PI3K/Akt/mTOR and p38 MAPK/Mnk1 pathways to benefit viral mRNA translation via interaction of the viral NP protein and host eIF4E. PLOS Pathog. 2020;16(6):e1008610. doi: 10.1371/journal.ppat.1008610
- Penney J, Tsurudome K, Liao EH, et al. LRRK2 regulates retrograde synaptic compensation at the Drosophila neuromuscular junction. Nat Commun. 2016;7(1):12188. doi: 10.1038/ncomms12188
- Li X, Sun L, Zhao J, et al. Mutations in the methyltransferase motifs of L protein attenuate Newcastle disease virus by regulating viral translation and cell-to-cell spread. Microbiol Spectr. 2021;9(2):e0131221. doi: 10.1128/Spectrum.01312-21
- Shizuo A, Uematsu S, Takeuchi O, et al. Pathogen recognition and innate immunity. Cell. 2006;124(4):783–801. doi: 10.1016/j.cell.2006.02.015
- Gack MU, Albrecht RA, Urano T, et al. Influenza a virus NS1 targets the ubiquitin ligase TRIM25 to evade recognition by the host viral RNA sensor RIG-I. Cell Host Microbe. 2009;5(5):439–49. doi: 10.1016/j.chom.2009.04.006
- Xu Q, Liang J, Jin J, et al. Newcastle disease virus nucleocapsid protein mediates the degradation of 14-3-3ε to antagonize the interferon response and promote viral replication. Vet Microbiol. 2023;284:109851. doi: 10.1016/j.vetmic.2023.109851
- Paldurai A Determination of Genetic Factors Involved in the Virulence of Newcastle Disease Virus. Doctoral dissertation. 2012.
- Kumar R, Tiwari AK, Chaturvedi U, et al. Cloning and expression analysis of multiple proteins encoding P gene of Newcastle disease virus. Indian J Exp Biol. 2013;51(2):116–23.
- Cong J, Feng X, Kang H, et al. Structure of the Newcastle disease virus L protein in complex with tetrameric phosphoprotein. Nat Commun. 2023;14(1):1324. doi: 10.1038/s41467-023-37012-y
- Gerard FC, Ribeiro Ede A Jr., Leyrat C, et al. Modular organization of rabies virus phosphoprotein. J Mol Biol. 2009;388(5):978–996. doi: 10.1016/j.jmb.2009.03.061
- Romer-Oberdorfer A, Mundt E, Mebatsion T, et al. Generation of recombinant lentogenic Newcastle disease virus from cDNA. J Gen Virol. 1999;80(Pt 11):2987–2995. doi: 10.1099/0022-1317-80-11-2987
- Yu X, Cheng J, He Z, et al. The glutamic residue at position 402 in the C-terminus of Newcastle disease virus nucleoprotein is critical for the virus. Sci Rep. 2017;7(1):17471. doi: 10.1038/s41598-017-17803-2
- Errington W, Emmerson PT. Assembly of recombinant Newcastle disease virus nucleocapsid protein into nucleocapsid-like structures is inhibited by the phosphoprotein. J Gen Virol. 1997;78(Pt 9):2335–2339. doi: 10.1099/0022-1317-78-9-2335
- Fagerberg L, Hallström BM, Oksvold P, et al. Analysis of the human tissue-specific expression by genome-wide integration of transcriptomics and antibody-based proteomics. Mol & Cell Proteomics. 2014;13(2):397–406. doi: 10.1074/mcp.M113.035600
- Roche MI, Ramadas RA, Medoff BD. The role of CARMA1 in T cells. Crit Rev Immunol. 2013;33(3):219–43. doi: 10.1615/CritRevImmunol.2013007056
- Juilland M, Thome M. Role of the CARMA1/BCL10/MALT1 complex in lymphoid malignancies. Curr Opin Hematol. 2016;23(4):402–9. doi: 10.1097/MOH.0000000000000257
- Wang W, Chang X, Yao W, et al. Host CARD11 inhibits Newcastle disease virus replication by suppressing viral polymerase activity in neurons. J Virol. 2019;93(24):e01499–19. doi: 10.1128/JVI.01499-19
- Wang W, Wei Q, Hao Q, et al. Cellular CARD11 inhibits the fusogenic activity of Newcastle disease virus via CBM signalosome-mediated furin reduction in chicken fibroblasts. Front Microbiol. 2021;12:607451. doi: 10.3389/fmicb.2021.607451
- Cheng X, Wang W, Xu Q, et al. Genetic modification of oncolytic Newcastle disease virus for cancer therapy. J Virol. 2016;90(11):5343–5352. doi: 10.1128/JVI.00136-16
- Park MS, Shaw ML, Munoz-Jordan J, et al. Newcastle disease virus (NDV)-based assay demonstrates interferon-antagonist activity for the NDV V protein and the Nipah virus V, W, and C proteins. J Virol. 2003;77(2):1501–1511. doi: 10.1128/JVI.77.2.1501-1511.2003
- Ganar K, Das M, Sinha S, et al. Newcastle disease virus: current status and our understanding. Virus Res. 2014;184:71–81. doi: 10.1016/j.virusres.2014.02.016
- Hagmaier K, Stock N, Precious B, et al. Mapuera virus, a rubulavirus that inhibits interferon signalling in a wide variety of mammalian cells without degrading STATs. J Gen Virol. 2007;88(Pt 3):956–966. doi: 10.1099/vir.0.82579-0
- Qiu X, Fu Q, Meng C, et al. Kinetic analysis of RNA editing of Newcastle disease virus P gene in the early period of infection. Acta Virol. 2016;60(1):71–77. doi: 10.4149/av_2016_01_71
- Samson AC, Levesley I, Russell PH. The 36K polypeptide synthesized in Newcastle disease virus-infected cells possesses properties predicted for the hypothesized ‘V’ protein. J Gen Virol. 1991;72(Pt 7):1709–13. doi: 10.1099/0022-1317-72-7-1709
- Nishio M, Tsurudome M, Garcin D, et al. Human parainfluenza virus type 2 L protein regions required for interaction with other viral proteins and mRNA capping. J Virol. 2011;85(2):725–32. doi: 10.1128/JVI.01226-10
- Jia D, Rahbar R, Chan RW, et al. Influenza virus non-structural protein 1 (NS1) disrupts interferon signaling. PLoS One. 2010;5(11):e13927. doi: 10.1371/journal.pone.0013927
- Pauli EK, Schmolke M, Wolff T, et al. Influenza A Virus Inhibits Type I IFN Signaling via NF-κB-Dependent Induction of SOCS-3 Expression. PLOS Pathog. 2008;4(11):e1000196. doi: 10.1371/journal.ppat.1000196
- Wang X, Jia Y, Ren J, et al. Newcastle disease virus nonstructural v protein upregulates SOCS3 expression to facilitate viral replication depending on the MEK/ERK Pathway. Front Cell Infect Microbiol. 2019;9:317. doi: 10.3389/fcimb.2019.00317
- Bhoj VG, Sun Q, Bhoj EJ, et al. MAVS and MyD88 are essential for innate immunity but not cytotoxic T lymphocyte response against respiratory syncytial virus. P Natl Acad Sci USA. 2008;105(37):14046–51. doi: 10.1073/pnas.0804717105
- Sun Y, Zheng H, Yu S, et al. Newcastle disease virus v protein degrades mitochondrial antiviral signaling protein to inhibit host type I interferon production via E3 ubiquitin ligase RNF5. J Virol. 2019;93(18):e00322–19. doi: 10.1128/JVI.00322-19
- Morita N, Tanaka Y, Odkhuu E, et al. Sendai virus V protein decreases nitric oxide production by inhibiting RIG-I signaling in infected RAW264.7 macrophages. Microbes Infect. 2020;22(8):322–330. doi: 10.1016/j.micinf.2020.01.005
- Uchida S, Horie R, Sato H, et al. Possible role of the Nipah virus V protein in the regulation of the interferon beta induction by interacting with UBX domain-containing protein1. Sci Rep. 2018;8(1):7682. doi: 10.1038/s41598-018-25815-9
- Civas A, Island ML, Génin P, et al. Regulation of virus-induced interferon-A genes. Biochimie. 2002;84(7):643–54. doi: 10.1016/S0300-9084(02)01431-1
- Qiu X, Fu Q, Meng C, et al. Newcastle disease virus V Protein Targets Phosphorylated STAT1 to Block IFN-I Signaling. PLoS One. 2016;11(2):e0148560. doi: 10.1371/journal.pone.0148560
- Motz C, Schuhmann KM, Kirchhofer A, et al. Paramyxovirus V proteins disrupt the fold of the RNA sensor MDA5 to inhibit antiviral signaling. Science. 2013;339(6120):690–3. doi: 10.1126/science.1230949
- Nan FL, Zhang H, Nan WL, et al. Lentogenic NDV V protein inhibits IFN responses and represses cell apoptosis. Vet Microbiol. 2021;261:109181. doi: 10.1016/j.vetmic.2021.109181
- Xu W, Wang S, Chen Q, et al. TXNL1-XRCC1 pathway regulates cisplatin-induced cell death and contributes to resistance in human gastric cancer. Cell Death Dis. 2014;5(2):e1055. doi: 10.1038/cddis.2014.27
- Ni P, Xu W, Zhang Y, et al. TXNL1 induces apoptosis in cisplatin resistant human gastric cancer cell lines. Curr Cancer Drug Tar. 2015;14(9):850–9. doi: 10.2174/1568009614666141028094612
- Wang C, Chu Z, Liu W, et al. Newcastle disease virus V protein inhibits apoptosis in DF-1 cells by downregulating TXNL1. Vet Res. 2018;49(1):102. doi: 10.1186/s13567-018-0599-6
- Bish R, Vogel C. RNA binding protein-mediated post-transcriptional gene regulation in medulloblastoma. Mol Cells. 2014;37(5):357–64. doi: 10.14348/molcells.2014.0008
- Yang M, Ma J, Chu Z, et al. Musashi1 inhibit the release of Newcastle disease viruses through preventing apoptosis of DF-1 cells. Poult Sci. 2021;100(7):101105. doi: 10.1016/j.psj.2021.101105
- Tang Y, Zhan W, Cao T, et al. CacyBP/SIP inhibits Doxourbicin-induced apoptosis of glioma cells due to activation of ERK1/2. IUBMB Life. 2016;68(3):211–9. doi: 10.1002/iub.1477
- Fu C, Wan Y, Shi H, et al. Expression and regulation of CacyBP/SIP in chronic lymphocytic leukemia cell balances of cell proliferation with apoptosis. J Cancer Res Clin Oncol. 2016;142(4):741–8. doi: 10.1007/s00432-015-2077-0
- Chu Z, Wang C, Tang Q, et al. Newcastle Disease Virus V Protein Inhibits Cell Apoptosis and Promotes Viral Replication by Targeting CacyBP/SIP. Front Cell Infect Microbiol. 2018;8:304. doi: 10.3389/fcimb.2018.00304
- Tong L, Chu Z, Gao X, et al. Newcastle disease virus V protein interacts with hnRNP H1 to promote viral replication. Vet Microbiol. 2021;260:109093. doi: 10.1016/j.vetmic.2021.109093
- Karsunke J, Heiden S, Murr M, et al. W protein expression by Newcastle disease virus. Virus Res. 2019;263:207–216. doi: 10.1016/j.virusres.2019.02.003
- Park, MS, Shaw, ML, Munoz-Jordan J, et al. Newcastle Disease Virus (NDV)-Based Assay Demonstrates Interferon-Antagonist Activity for the NDV V Protein and the Nipah Virus V, W, and C Proteins. J Virol. 2003;77(2):1501–1511. doi: 10.1128/JVI.77.2.1501-1511.2003
- Fu X, Liang C, Li F, et al. The rules and functions of nucleocytoplasmic shuttling proteins. Int J Mol Sci. 2018;19(5):1445. doi: 10.3390/ijms19051445
- Miyamoto Y, Yamada K, Yoneda Y. Importin α: a key molecule in nuclear transport and non-transport functions. J Biochem. 2016;160(2):69–75. doi: 10.1093/jb/mvw036
- Yang Y, Xue J, Teng Q, et al. Mechanisms and consequences of Newcastle disease virus W protein subcellular localization in the nucleus or mitochondria. J Virol. 2021;95(7):e02087–20. doi: 10.1128/JVI.02087-20
- Kim YH, Han ME, Oh SO. The molecular mechanism for nuclear transport and its application. Anat Cell Biol. 2017;50(2):77–85. doi: 10.5115/acb.2017.50.2.77
- Battisti AJ, Meng G, Winkler DC, et al. Structure and assembly of a paramyxovirus matrix protein. P Natl Acad Sci USA. 2012;109(35):13996–4000. doi: 10.1073/pnas.1210275109
- Duan Z, Deng S, Ji X, et al. Nuclear localization of Newcastle disease virus matrix protein promotes virus replication by affecting viral RNA synthesis and transcription and inhibiting host cell transcription. Vet Res. 2019;50(1):22. doi: 10.1186/s13567-019-0640-4
- Duan Z, Song Q, Wang Y, et al. Characterization of signal sequences determining the nuclear export of Newcastle disease virus matrix protein. Arch Virol. 2013;158(12):2589–95. doi: 10.1007/s00705-013-1769-5
- Duan Z, Xu H, Ji X, et al. Importin alpha5 negatively regulates importin beta1-mediated nuclear import of Newcastle disease virus matrix protein and viral replication and pathogenicity in chicken fibroblasts. Virulence. 2018;9(1):783–803. doi: 10.1080/21505594.2018.1449507
- Duan Z, Chen J, Xu H, et al. The nucleolar phosphoprotein B23 targets Newcastle disease virus matrix protein to the nucleoli and facilitates viral replication. Virology. 2014;452-453:212–22. doi: 10.1016/j.virol.2014.01.011
- Duan Z, Han Y, Zhou L, et al. Chicken bromodomain-containing protein 2 interacts with the Newcastle disease virus matrix protein and promotes viral replication. Vet Res. 2020;51(1):120. doi: 10.1186/s13567-020-00846-1
- Zhou L, Han YF, Yuan C, et al. Screening and bioinformatics analysis of cellular proteins interacting with chicken bromodomain-containing protein 2 in DF-1 cells. Br Poultry Sci. 2021;62(6):810–819. doi: 10.1080/00071668.2021.1943311
- Pantua HD, McGinnes LW, Peeples ME, et al. Requirements for the assembly and release of Newcastle disease virus-like particles. J Virol. 2006;80(22):11062–73. doi: 10.1128/JVI.00726-06
- Duan Z, Hu Z, Zhu J, et al. Mutations in the FPIV motif of Newcastle disease virus matrix protein attenuate virus replication and reduce virus budding. Arch Virol. 2014;159(7):1813–9. doi: 10.1007/s00705-014-1998-2
- Peng T, Qiu X, Tan L, et al. Ubiquitination on lysine 247 of Newcastle disease virus matrix protein enhances viral replication and virulence by Driving Nuclear-Cytoplasmic Trafficking. J Virol. 2022;96(2):e0162921. doi: 10.1128/JVI.01629-21
- Duan Z, Zhang Q, Liu M, et al. Multifunctionality of matrix protein in the replication and pathogenesis of Newcastle disease virus: A review. Int j biol macromol. 2023;249:126089. doi: 10.1016/j.ijbiomac.2023.126089
- Cibulka J, Fraiberk M, Forstova J. Nuclear actin and lamins in viral infections. Viruses. 2012;4(3):325–47. doi: 10.3390/v4030325
- Giuffre RM, Tovell DR, Kay CM, et al. Evidence for an interaction between the membrane protein of a paramyxovirus and actin. J Virol. 1982;42(3):963–8. doi: 10.1128/jvi.42.3.963-968.1982
- Laliberte JP, McGinnes LW, Peeples ME, et al. Integrity of membrane lipid rafts is necessary for the ordered assembly and release of infectious Newcastle disease virus particles. J Virol. 2006;80(21):10652–62. doi: 10.1128/JVI.01183-06
- Li X, Li X, Cao H, et al. Engagement of new castle disease virus (NDV) matrix (M) protein with charged multivesicular body protein (CHMP) 4 facilitates viral replication. Virus Res. 2013;171(1):80–8. doi: 10.1016/j.virusres.2012.10.033
- Bauer A, Neumann S, Karger A, et al. ANP32B is a nuclear target of henipavirus M proteins. PLoS One. 2014;9(5):e97233. doi: 10.1371/journal.pone.0097233
- Gunther M, Bauer A, Muller M, et al. Interaction of host cellular factor ANP32B with matrix proteins of different paramyxoviruses. J Gen Virol. 2020;101(1):44–58. doi: 10.1099/jgv.0.001362
- Shah M, Bharadwaj MSK, Gupta A, et al. Chicken viperin inhibits Newcastle disease virus infection in vitro: A possible interaction with the viral matrix protein. Cytokine. 2019;120:28–40. doi: 10.1016/j.cyto.2019.04.007
- Ren S, Ur Rehman Z, Gao B, et al. ATM-mediated DNA double-strand break response facilitated oncolytic Newcastle disease virus replication and promoted syncytium formation in tumor cells. PLOS Pathog. 2020;16(6):e1008514. doi: 10.1371/journal.ppat.1008514
- Wang C, Wang T, Hu R, et al. Cyclooxygenase-2 Facilitates Newcastle Disease Virus Proliferation and is as a Target for Canthin-6-One Antiviral Activity. Front Microbiol. 2020;11:987. doi: 10.3389/fmicb.2020.00987
- Winn J, Carter M, Avery L, et al. Hox and a newly identified E2F co-repress cell death in Caenorhabditis elegans. Genetics. 2011;188(4):897–905. doi: 10.1534/genetics.111.128421
- Molouki A, Hsu YT, Jahanshiri F, et al. The matrix (M) protein of Newcastle disease virus binds to human Bax through its BH3 domain. Virol J. 2011;8(3):385. doi: 10.1186/1743-422X-8-385
- Rangaswamy US, Wang W, Cheng X, et al. Newcastle Disease Virus Establishes Persistent Infection in Tumor Cells in Vitro: Contribution of the Cleavage Site of Fusion Protein and Second Sialic Acid Binding Site of Hemagglutinin-Neuraminidase. J Virol. 2017;91(16):e00770–17. doi: 10.1128/JVI.00770-17
- Baker KA, Dutch RE, Lamb RA, et al. Structural basis for paramyxovirus-mediated membrane fusion. Mol Cell. 1999;3(3):309–19. doi: 10.1016/S1097-2765(00)80458-X
- Bu Y, Teng Q, Feng D, et al. Adaptor complex-mediated trafficking of Newcastle disease virus fusion protein is regulated by the YLMY motif of its cytoplasmic tail. Virulence. 2022;13(1):1849–1867. doi: 10.1080/21505594.2022.2136433
- Kim SH, Subbiah M, Samuel AS, et al. Roles of the fusion and hemagglutinin-neuraminidase proteins in replication, tropism, and pathogenicity of avian paramyxoviruses. J Virol. 2011;85(17):8582–96. doi: 10.1128/JVI.00652-11
- Matsuoka Y, Matsumae H, Katoh M, et al. A comprehensive map of the influenza a virus replication cycle. BMC Syst Biol. 2013;7(1):97. doi: 10.1186/1752-0509-7-97
- Yan C, Liu H, Jia Y, et al. Screening and mechanistic study of key sites of the hemagglutinin-neuraminidase protein related to the virulence of Newcastle disease virus. Poult Sci. 2020;99(7):3374–3384. doi: 10.1016/j.psj.2020.04.014
- Owen DJ, Collins BM, Evans PR. Adaptors for clathrin coats: structure and function. Annu Rev Cell Dev Bi. 2004;20(1):153–91. doi: 10.1146/annurev.cellbio.20.010403.104543
- Traub LM. Tickets to ride: selecting cargo for clathrin-regulated internalization. Nat Rev Mol Cell Bio. 2009;10(9):583–96. doi: 10.1038/nrm2751
- Berhanu A, Ideris A, Omar AR, et al. Molecular characterization of partial fusion gene and C-terminus extension length of haemagglutinin-neuraminidase gene of recently isolated Newcastle disease virus isolates in Malaysia. Virol J. 2010;7(1):183. doi: 10.1186/1743-422X-7-183
- Jin Z, Wei Q, Bi Y, et al. Identification of a potential neutralizing linear epitope of hemagglutinin-neuraminidase in Newcastle disease virus. Virol J. 2021;18(1):8. doi: 10.1186/s12985-020-01483-y
- Yuan P, Swanson KA, Leser GP, et al. Structure of the Newcastle disease virus hemagglutinin-neuraminidase (HN) ectodomain reveals a four-helix bundle stalk. P Natl Acad Sci USA. 2011;108(36):14920–5. doi: 10.1073/pnas.1111691108
- Sun J, Han Z, Shao Y, et al. Comparative proteome analysis of tracheal tissues in response to infectious bronchitis coronavirus, Newcastle disease virus, and avian influenza virus H9 subtype virus infection. Proteomics. 2014;14(11):1403–23. doi: 10.1002/pmic.201300404
- Sun J, Han Z, Qi T, et al. Chicken galectin-1B inhibits Newcastle disease virus adsorption and replication through binding to hemagglutinin-neuraminidase (HN) glycoprotein. J Biol Chem. 2017;292(49):20141–20161. doi: 10.1074/jbc.M116.772897
- Ramos I, Stamatakis K, Oeste CL, et al. Vimentin as a multifaceted player and potential therapeutic target in viral infections. Int J Mol Sci. 2020;21(13):4675. doi: 10.3390/ijms21134675
- Lu X, Liu K, Chen Y, et al. Cellular vimentin regulates the infectivity of Newcastle disease virus through targeting of the HN protein. Vet Res. 2023;54(1):92. doi: 10.1186/s13567-023-01230-5
- Dai J, Dan W, Schneider U, et al. β-Carboline alkaloid monomers and dimers: Occurrence, structural diversity, and biological activities. Eur J Med Chem. 2018;157:622–656. doi: 10.1016/j.ejmech.2018.08.027
- Chen D, Su A, Fu Y, et al. Harmine blocks herpes simplex virus infection through downregulating cellular NF-κB and MAPK pathways induced by oxidative stress. Antivir Res. 2015;123:27–38. doi: 10.1016/j.antiviral.2015.09.003
- Chen D, Tian X, Zou X, et al. Harmine, a small molecule derived from natural sources, inhibits enterovirus 71 replication by targeting NF-κB pathway. Int Immunopharmacol. 2018;60:111–120. doi: 10.1016/j.intimp.2018.04.050
- Wang C, Wang T, Dai J, et al. 1-Formyl-beta-carboline Derivatives Block Newcastle Disease Virus Proliferation through Suppressing Viral Adsorption and Entry Processes. Biomolecules. 2021;11(11):1687. doi: 10.3390/biom11111687
- Chen S, Zhang Q, Xu D, et al. Antitumor effect of the Newcastle disease viral hemagglutinin-neuraminidase gene is expressed through an oncolytic adenovirus effect in osteosarcoma cells. Anti-Cancer Drug. 2018;29(3):197–207. doi: 10.1097/CAD.0000000000000575
- Baradaran A, Yusoff K, Shafee N, et al. Newcastle disease virus hemagglutinin neuraminidase as a potential Cancer targeting agent. J Cancer. 2016;7(4):462–6. doi: 10.7150/jca.13566
- Vanlandschoot P, Stortelers C, Beirnaert E, et al. Nanobodies®: new ammunition to battle viruses. Antivir Res. 2011;92(3):389–407. doi: 10.1016/j.antiviral.2011.09.002
- Gao X, Hu X, Tong L, et al. Construction of a camelid VHH yeast two-hybrid library and the selection of VHH against haemagglutinin-neuraminidase protein of the Newcastle disease virus. BMC Vet Res. 2016;12(1):39. doi: 10.1186/s12917-016-0664-1
- Sourimant J, Rameix-Welti MA, Gaillard AL, et al. Fine mapping and characterization of the L-polymerase-binding domain of the respiratory syncytial virus phosphoprotein. J Virol. 2015;89(8):4421–33. doi: 10.1128/JVI.03619-14
- Wang P, Kong F, Wei J, et al. Alkaloids from the mangrove-derived actinomycete jishengella endophytica 161111. Mar Drug. 2014;12(1):477–90. doi: 10.3390/md12010477
- Wang YH, Tang JG, Wang RR, et al. Flazinamide, a novel beta-carboline compound with anti-HIV actions. Biochem Biophys Res Commun. 2007;355(4):1091–1095. doi: 10.1016/j.bbrc.2007.02.081
- Wang C, Wang T, Hu R, et al. 9-butyl-harmol exerts antiviral activity against Newcastle disease virus through targeting GSK-3β and HSP90β. J Virol. 2023;97(3):e0198422. doi: 10.1128/jvi.01984-22
- Chen Y, Liu W, Xu H, et al. MicroRNA Expression Profiling in Newcastle Disease Virus-Infected DF-1 Cells by Deep Sequencing. Front Microbiol. 2019;10:1659. doi: 10.3389/fmicb.2019.01659
- Chen Y, Zhu S, Hu J, et al. Gga-miR-1603 and gga-miR-1794 directly target viral L gene and function as a broad-spectrum antiviral factor against NDV replication. Virulence. 2021;12(1):45–56. doi: 10.1080/21505594.2020.1864136
- Liu YC, Grusovin J, Adams TE, et al. Electrostatic Interactions between Hendra Virus Matrix Proteins are Required for Efficient Virus-Like-Particle Assembly. J Virol. 2018;92(13):e00143–18. doi: 10.1128/JVI.00143-18
- Lin LT, Richardson CD. The host cell receptors for measles virus and their interaction with the viral hemagglutinin (H) protein. Viruses. 2016;8(9):250. doi: 10.3390/v8090250
- Irie T, Kiyotani K, Igarashi T, et al. Inhibition of interferon regulatory factor 3 activation by paramyxovirus V protein. J Virol. 2012;86(13):7136–45. doi: 10.1128/JVI.06705-11
- Komatsu T, Tanaka Y, Kitagawa Y, et al. Sendai Virus V Protein Inhibits the Secretion of Interleukin-1β by Preventing NLRP3 Inflammasome Assembly. J Virol. 2018;92(19):e00842–18. doi: 10.1128/JVI.00842-18
- Li M, Schmitt PT, Li Z, et al. Mumps virus matrix, fusion, and nucleocapsid proteins cooperate for efficient production of virus-like particles. J Virol. 2009;83(14):7261–72. doi: 10.1128/JVI.00421-09
- Pei Z, Bai Y, Schmitt AP. PIV5 M protein interaction with host protein angiomotin-like 1. Virology. 2010;397(1):155–66. doi: 10.1016/j.virol.2009.11.002
- Jiang L, Chen H, Li C. Advances in deciphering the interactions between viral proteins of influenza a virus and host cellular proteins. Cell Insight. 2023;2(2):100079. doi: 10.1016/j.cellin.2023.100079
- Hu Y, Okyere SK, Xu R, et al. Assessment of antiviral activity and mechanism of rhein on Newcastle disease virus (La Sota strain IV) in vitro. Nat Prod Res. 2022;36(5):1400–1404. doi: 10.1080/14786419.2021.1878515